- 1School of Design, The Hong Kong Polytechnic University, Kowloon, Hong Kong SAR, China
- 2School of Computer Science and Engineering, Bangor University, Bangor, United Kingdom
The urgency of environmental sustainability has catalyzed a thorough reconsideration of traditional workspaces and their environmental impact, particularly concerning carbon footprint stemming from business-related travel and daily commutes. In response to these concerns, the innovative notion of the “Phygital Workspace” has arisen. Moreover, the advent of the COVID-19 pandemic has accelerated the proliferation of this technology. In a Phygital Workspace, physical and digital elements coexist and interact to enhance productivity, collaboration, and overall work experience. This integration is often facilitated using advanced digital technologies, such as Augmented Reality (AR), Virtual Reality (VR), Mixed Reality (MR), and other immersive or interactive tools. This conceptual framework sets the stage for the seamless integration of both physical and digital dimensions, empowered by the potential of Extended Reality (XR) technology. An integral part of this review involves the analysis of various workspace scenarios, and further examines the role of a Phygital Workspace plays in reducing carbon footprint within each context. The scenarios under examination include flexible workspace scenarios, field work scenarios, and hybrid work scenarios. XR technology’s contributions to reducing carbon footprint are evaluated by minimizing the need for physical travel, enabling remote collaboration, and enhancing workspace efficiency within these scenarios. Employing a systematic review methodology, this review considers the status of XR technology adoption in diverse business environments, pinpointing its strengths and limitations. The aim is to provide a solid foundation for the framework of a new typological work environment that leverages XR technology to effectively reduce the carbon footprint associated with travel. The framework comprises four key dimensions: XR Infrastructure, Collaborative Work Environment, Social Interaction Enhancement, and Environmental Impact Assessment. These dimensions collectively guide the development and implementation of sustainable Phygital Workspaces, catering to technological, social, and ecological considerations. It is an impassioned call for collaborative efforts among researchers, policymakers, and organizations to converge their expertise for a more environmentally conscious future of work.
1 Introduction
The evolution of workspaces has undergone a profound and far-reaching transformation, significantly driven by the relentless March of technological progress (Figure 1). We have traversed a path that extends from the traditional, tangible brick-and-mortar office environments of bygone eras to the sudden and dramatic rise of teleworking, a trend accelerated by the global COVID-19 pandemic (Figure 2). This evolution has intricately intertwined the spheres of work dynamics, environmental sustainability, and carbon footprint. The emergence of Extended Reality (XR) interactive technologies redefines not only the way we work but also the nature of our interactions, all while profoundly impacting our contributions to the environment.
In the post-COVID telework era, XR technologies have risen as powerful tools capable of bridging the physical divide, enabling immersive virtual interactions that hold the potential to reshape our professional lives. With telepresence meetings, virtual conferences, and collaborative digital workspaces, XR technologies have drastically reduced the necessity for business-related travel, thereby making significant strides towards mitigating carbon footprint associated with transportation. A “phygital” workspace, is where physical and digital elements intertwine seamlessly, became a reality, enabling remote employees to engage in immersive shared environments, reducing the demand for resource-intensive office spaces. This concept has gained prominence due to advancements in communication tools, collaboration platforms, and the increasing need for telework options.
However, while the integration of XR technologies into workspaces offers promising avenues for reducing carbon footprint, it is crucial to address their own environmental implications. The production, energy consumption, and eventual disposal of XR hardware require careful consideration.
In the exploration that follows, we will navigate the shifts that have taken place, transitioning from the conventional pre-COVID traditional workspaces to the emerging post-COVID telework paradigms. Simultaneously, we will carefully consider the transformative influence that XR technologies exert on the broader landscape of sustainability.
Specifically, we answer the following research questions in this article:
RQ1: The definition of Phygital Workspace, compared with traditional workspace and telework spaces.
RQ2: How XR enabled Phygital workspace reduce the carbon footprint and in which industries is it particularly needed?
RQ3: The future research framework of Pyhigital workspace.
1.1 Search methodology
As showed in Figure 3, the search was performed by combining keywords that relate to AR/VR/MR/XR (“augmented reality” OR “mixed reality” OR “virtual reality” OR “Extended Reality” OR"AR” OR “MR” OR “VR"OR"XR”) with keywords relating to specific environmental topics to form regular expressions. All possible combinations of keywords from the left column with keywords from the right column were tested. An initially short list of keywords was expanded by the findings of the search. Through this iterative procedure, the search finally led to the initial identification of 65 related publications. The literature search was performed between July and December 2023. The duration of post-COVID telework spaces spans from 2019 until the present.
1.2 Pre-COVID traditional workspaces: a historical perspective
In the era preceding the COVID-19 pandemic, the traditional workspace was characterized by bustling offices, daily commutes, and in-person interactions and business-related travel (Hook et al., 2020). Carbon footprint was a natural byproduct of these practices, as employees traversed urban centers, contributing significantly to transportation-related emissions.
According to a report by EPA (U.S. Environmental Protection Agency) in 2023, a typical passenger vehicle emits about 4.6 metric tons of carbon dioxide per year, about 400 g of CO2 per mile. This impact was exacerbated by factors such as traffic congestion and extended travel distances, resulting in commuting accounting for a substantial 40–60 percent of total carbon footprint within the transportation sector. In Beijing (Wang and Zeng, 2019), by 2014, the sheer volume of CO2 emissions attributable to commuting had surged to a staggering 553.68 × 104 tons, with car journeys being the predominant contributors, accounting for a substantial 75%–80% of emissions. In the context of Hong Kong (Xue et al., 2022), the conventional 5-day commute into the city center is associated with the most significant carbon footprint. In 2020, the transport sector accounted for a notable 19.7 percent of carbon footprint, positioning it as the second-largest contributor, second only to the electricity industry. The persistent growth in the number of licensed private cars, with over 70 percent of registered vehicles in Hong Kong being privately owned as of 2021, thus constituting a key factor in emissions within the transport sector.
Meanwhile, business travel encompasses non-commuting journeys associated with various work-related activities, such as off-site meetings and events, constituting another significant source of carbon footprint generation within the professional sphere. A report estimated that, before the COVID-19 pandemic, business travel constituted approximately 15–20 percent of global travel (McCain et al., 2021). The carbon footprint emanating from business travel primarily manifests as indirect emissions, representing a challenge that extends beyond immediate organizational control. Among these emissions, air travel emerges as the predominant contributor. Alternative modes of business travel, such as rail and passenger vehicles, exhibited an estimated 3.5 times smaller carbon footprint impact per passenger according to The Department for Business Energy & Industrial Strategy (BEIS), 2022 (Figure 4). This underscores the environmental implications of various transportation choices in the business context, emphasizing the importance of exploring and adopting sustainable alternatives to mitigate the carbon footprint associated with business travel.
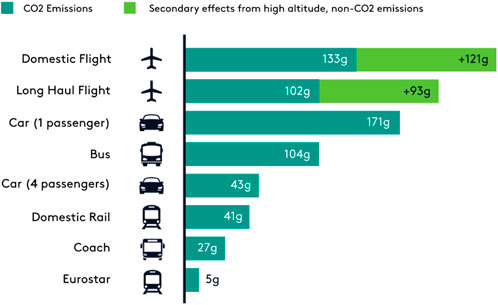
Figure 4. Emissions from different types of transport, per passenger per km travelled Source: The Department for Business Energy & Industrial Strategy (BEIS), 2022. This image is licensed under the terms of the Open Government Licence v3.0.
1.3 Post-COVID telework spaces: a new frontier
The concept of telework spaces had already been in existence before 2020 (Globally Cool, 2022). The necessity for physical office presence varies significantly depending on industry specifics and organizational demands. Certain sectors, particularly those dealing with sensitive information, require secure physical environments to ensure data protection. Roles that rely on specialized equipment and technology only available in office settings may find in-office work indispensable. Prior to the COVID-19 pandemic, only about 5% of Europeans were engaged in telework (Giovanis, 2018). The onset of the pandemic accelerated the demand for telework and led to significant technological advancements in this field. In 2022, the United Kingdom emerged as a leader in this transformative trend, experiencing a 20% reduction in the number of individuals commuting to work. This was closely followed by Ireland at 17% and Sweden at 13% (Elldér, 2020). By May 2021, 12.3% of people aged between 15 and 64 were working remotely, which increased 140% compared to figures before the pandemic (Remote Work Statistics Europe, 2023).
Based on our literature review and surveys on telework, the author concludes that compared with traditional workspace, telework significantly alter spatial settings, working hours and technology management, subsequently impacting employee psychology and team management, as illustrated in Figure 5. Traditional workspace design encompasses three main categories. Cubical offices cater to the era’s needs, offering privacy, concentration space, and concealed cable management. Conversely, the Hot Desk system is not assigned to a specific worker, eliminating fixed seating and allowing employees to choose spaces based on their work mode. The shift in open workspace design emphasizes psychological considerations, with designers analyzing human behavior, wellness, and cultural roles to enhance staff engagement, productivity, and create a healthier work environment. Compared to the spatial settings of traditional workspaces, homes for teleworkers serve multiple purposes, with personal and professional spheres overlapping in terms of spatial and temporal requirements (Maillot et al., 2022). Regarding working hours, teleworking individuals transition from place to place in an office setting, while at home, they remain in the same location despite engaging in various activities, thus presenting possibilities of work-family conflict (Hill et al., 2003; Vaziri et al., 2020).
From a technological standpoint, while Information and Communication Technologies (ICTs) play a central role in teleworking, they alone are insufficient for creating optimal remote working conditions (Santos and Pereira, 2023). The utilization of technologies requires training and adaptation time for all company members, necessitating technical and organizational support (Golden, 2009). Psychologically, the reliance on technology for maintaining professional relationships and performing work activities is crucial, as studies emphasize its importance in preserving team connections, circulating information, and fostering a unified work team (Maillot et al., 2022). The willingness of employees to adapt management styles to the telework context has resulted in increased operational leeway and the cultivation of close relationships, perceived as a supportive sign. However, this adaptability has a dual effect, as managers, in response, have intensified their control measures, at times resorting to awkward and intrusive practices, such as an excessive use of virtual meetings. This increased control not only limits employees’ autonomy and recovery but also contributes to the risk of burnout (Vullinghs et al., 2020). On an individual level, virtual tools fall short of fully compensating for face-to-face interactions, and the use of cameras and microphones during meetings may constrain the spontaneity of exchanges.
The adoption of telework significantly reduces commuting frequency and distances (Figure 6), thereby substantially curbing emissions stemming from transportation. Research indicates potential reductions in weekly vehicle distance traveled, with estimates reaching 20%, leading to fewer trips by up to 30% and time savings of 28% due to reduced congestion. A study conducted in London (Element Energy Limited, 2022) demonstrated that individuals who adopted more flexible work arrangements, splitting their work time between a city center office and local workspaces, experienced a remarkable 49 percent reduction in carbon footprint compared to the traditional 5-day commute. Similarly, those who divided their work hours between local workspaces and home witnessed a 43 percent reduction in carbon footprint (McCain et al., 2021). It is noteworthy that in countries where public transport is a common mode of commuting, teleworking practices will have less impact on energy use than in countries where the private car is the dominant mode (Hook et al., 2020). From business travel side, during the peak of the pandemic, there was a 68% reduction in jet fuel demand, resulting in a 10% decline in U.S. carbon footprint compared to 2019, significantly impacted by the downturn in business travel (McCain et al., 2021).
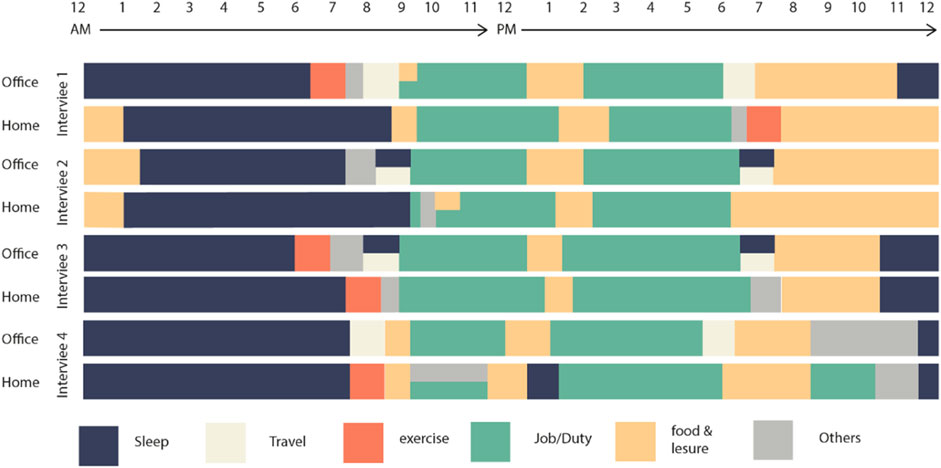
Figure 6. The mapping of daily routine with the comparison of working in office and at home of traditional workspace. Data collected by Tim Yu Lai.
However, the relationship between telework and travel patterns is more complex than initially perceived. It is suggest that the travel demand displaced by teleworking may be partly offset by induced travel demand in other areas (Hook et al., 2020). As shown by Elldér, 2020, teleworkers tend to travel farther than non-teleworkers on both teleworking and non-teleworking days. While non-teleworkers averaged 46 km per day, teleworkers covered 54 km on teleworking days and 64 km on non-teleworking days. The U.S. National Household Travel Surveys (NHTS) revealed that teleworkers took 10.8% more non-work trips per day compared to non-teleworkers (4.18 versus 3.77), with these trips being, on average, 15.7% longer (36 km versus 32 km). Teleworking may result in increased energy consumption at home, attributed to activities such as heating, cooking, and lighting, without corresponding reductions in energy usage at the workplace (Hook et al., 2020). In consequence, this ‘additive’ impact of teleworking could be further exacerbated if businesses do not transition to smaller offices, which generally have a reduced energy footprint, or if they choose not to close their offices in response to the growing prevalence of teleworking. Additionally, a survey (Opinium, 2022) found that a lack of planning and investment in teleworking is posing a threat to a successful return to the office on a large scale. Consequently, there could be an increase in office energy consumption, and possibly total carbon emissions, because of the surge in telecommuting juxtaposed with insufficient planning.
2 Development of XR-enabled phygital workspace (RQ1)
2.1 Challenges inherent in telework spaces: case study
In the contemporary landscape of collaborative telework spaces, the integration of diverse expertise and perspectives is essential. This case study examines POLAR* (Project Oriented Learning and Action Research), through which engineers, designers, environmental scientists, and psychologists collaborate in transdisciplinary teams on a project that addresses a complex challenge.
In POLAR* 2023-24 “Agricademy”, the focus of this study, students engaged with stakeholders in the Mekong Delta to understand and address the impact of saline intrusion, which is affecting livelihoods in agricultural communities. We focus on identifying challenges that are inherent to teleworking spaces, offer insights into the limitations of virtual collaboration, and propose a framework for XR-enabled Phygital Workspaces.
Professionals are required to collaborate in teams that span business functions and geographic boundaries, using teleworking software. Universities tend to organise around specialist schools of thought, which limits opportunities for students to work with others outside of their discipline. Addressing the disconnect between academic and professional realms, POLAR* is a platform for transdisciplinary teaching and learning that prepares graduates for the future of work.
Participating students work in transdisciplinary teams to address a complex, live challenge. They telework from different global locations, collaborating with stakeholders who are situated locally to the challenge, guided by academic coaches.
Teams complete a series of tasks, governed by the Double Diamond [Design Council] process; a series of divergent-convergent steps followed by a critical review. They map and analyse a complex ecosystem, identify specific opportunities, then propose interventions for consideration by the local stakeholders. Design methods used promote visual communication and help develop a shared modelling language, which facilitates discussion and accelerates decision making.
Applying methods from design research, tacit knowledge is shared and explicit knowledge is developed within each team. Framed by the SECI Model [Nonaka]: 1) Team formation tasks socialise knowledge, 2) divergent steps externalise knowledge, 3) convergent steps combine knowledge, and 4) critical reviews followed by reflection internalises knowledge.
Miro, an online collaborative visual workspace platform chosen to be used in Polar*, fosters free-form visual communication over type-constrained written communication.
For this case, four key points are analysed to examine the effects in translating collaborative efforts into practical, impactful solutions. Obstacles faced in the process are also summarised, prompting questions about the robustness and applicability of proposed solutions.
2.1.1 Quality and speed of communication
During Socialisation, where essential tacit knowledge is shared and relationships are formed, team members felt uncertain about common goals, strategies. This resulted in team instability and dissatisfaction.
In conducting research for this project, architects were unable to conduct an on-site exploration and psychologists were unable to interact with stakeholder directly. The absence of a direct experience of the site and stakeholder limited the contextual information available.
Despite input from local stakeholders, elements such as survey questionnaires, field investigations, and on-site trials during prototyping were limited. This is a common challenge in site-specific projects.
2.1.2 Familiarity with virtual collaboration tools
It is crucial to recognize that not all individuals possess the same level of familiarity with online collaboration tools and the research framework. “Lack of training” and “extended breaks in practice” are identified as potential causes of performance decay, both of which may raise concerns in the context of telework.
Beyond web-based training modules and virtual online meetings, participating employees face limited opportunities for practical training or the cultivation of professional networks to share interpersonal experiences (Badiru, 2012). Such disparities can create hurdles to effective collaboration, underscoring the need for comprehensive training and support to bridge these gaps.
2.1.3 Team building and relationships
From the perspective of social interaction, concerns regarding the disconnection experienced by team members due to isolation from team activities.
Asynchronous communication using messaging tools allowed flexibility, but weakened social connections that would formed more effectively during synchronous communication.
2.1.4 Knowledge management
In a project with a prolonged duration, learning curves (Badiru and Mertens, 2023) and forgetting curves (Badiru, 1994) exist. Researchers have suggested that extended disconnection from their normal work routine within a collective social presence can amplify the tendency to forget lessons learned about efficient ways of working (Badiru and Mertens, 2023).
From the perspective of collaborative efficiency, it became challenging to maintain consistency and continuity throughout the project.
2.2 XR framework (RQ1)
As Figure 7 illustrates, in the realm of XR, where “X" symbolizes the ever-evolving landscape of new realities (Rauschnabel et al., 2022), it encompasses a spectrum of technologies that merge the physical and digital worlds, including Virtual Reality (VR), Augmented Reality (AR), and Mixed Reality (MR). They played a pivotal role in revolutionizing the way of connecting and collaborating in telework scenarios. It fostered online workspaces and virtual platforms as promising avenues for reducing the environmental impact of traditional work practices.
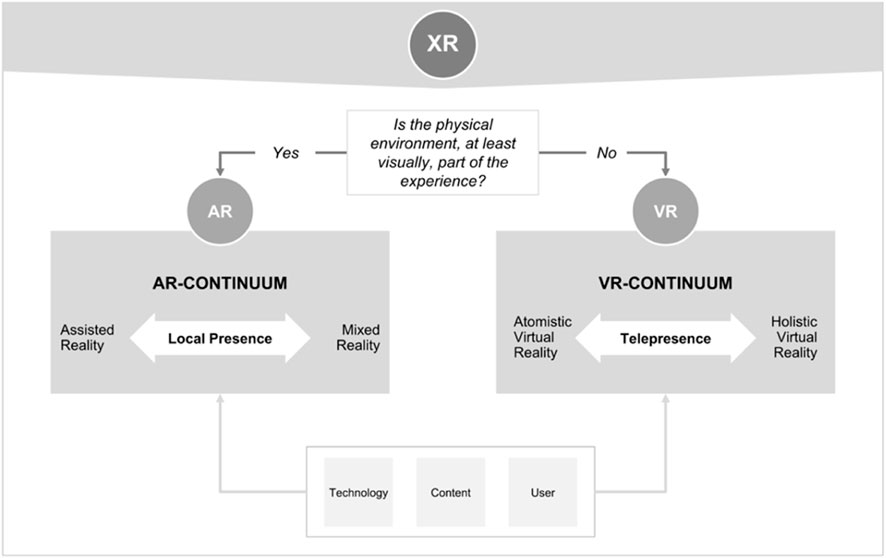
Figure 7. The XR framework of Augmented and Virtual Reality. Reproduced from Rauschnabel et al., 2022, licensed under CC-BY-4.0 DEED.
Augmented Reality (AR) seamlessly integrates virtual content into a user’s real-time perception of the physical environment through computational devices. Local presence, as defined by Rauschnabel et al., 2022 gauges the extent to which users perceive AR objects as truly present in their physical surroundings. In assisted reality, content appears distinctly artificial and overlaid, lacking a sense of actual presence. In contrast, Mixed Reality (MR) provides users with the experience of virtual content truly existing in their physical environment. The potential of AR lies in its ability to overlay digital information onto physical spaces, thereby facilitating real-time collaboration and remote assistance. While MR merges the physical and digital worlds together, seamlessly integrating tangible and digital elements to enrich interactive experiences.
Virtual Reality (VR) immersing users in an artificial, all-encompassing 3D space that visually separates them from the physical environment. VR, as explored in previous research (Rauschnabel et al., 2022), is closely linked to the concept of telepresence, which measures the extent to which a user feels present in the virtual realm rather than the physical environment. Applications of VR ranging from desk scale, room scale to immersive cave scale, characterized by lower levels of telepresence, to holistic VR, where telepresence is markedly high. VR’s immersive capabilities mainly transform workspaces by providing virtual meeting environments that enhance remote collaboration and reduce the need for physical presence. To enable social interaction, VR experiences rely on virtual representations, such as avatars or live capturing individuals as holograms into spaces. The former is created by the computer through specific software for animation, while the latter is achieved through volumetric capture technology, enabling real-time projection of a remote person.
From the preceding case analyses and literature reviews, we have identified that telework presents more opportunities for improvement in team management approaches, collaborative interaction methods, and the potential carbon footprint implications in specific industries. As the design of the workspace significantly influences employee satisfaction and productivity, XR (Extended Reality) enabled Phygital Workspaces could serve as potential tools to integrate into the new working environment. The design of Phygital Workspaces, enabling intuitive and gestural modifications of shared spaces, not only facilitates personalized environments but also plays a crucial role in environmental conservation by reducing the demand for resource-intensive office space. This, in turn, contributes to further diminishing the carbon footprint associated with telework.
For example, unlike traditional video conferencing like Zoom where participants are displayed on a flat screen, XR-enabled meetings utilize spatial presence features, such as spatial audio, to create a sense of shared physical space among participants. This enhances interaction, improves focus, and reduces external disruptions commonly encountered in traditional video conferences.
In XR meetings, participants often use avatars or virtual representations of themselves. These avatars can be customized to reflect the user’s appearance and, in some cases, convey non-verbal cues and body language, enhancing the sense of presence and personalization. Meanwhile, participants can manipulate virtual objects, share 3D models, and collaborate in real-time within the virtual environment. This fosters a more dynamic and engaging meeting experience (Figure 8).
As depicted in Figure 9, the framework is constructed based on the user feedback mechanism (left) in the utilization of Phygital Workspaces, aiming to reduce the carbon footprint (up). Simultaneously, limitations of this technology in achieving this goal are also taken into consideration. Leveraging XR technology as a means, the approach unfolds by fostering a collaborative work environment and enhancing social interaction (right).
“Phygital workspace" is a concept that combines physical and digital elements in a workspace, and its feedback mechanism is based on users' physical and perceptual inputs. Physical inputs from users may include tangible actions and interactions between individuals and interfaces, while perceptual inputs encompass spatial awareness and interactions with virtual content.
In this workspace, the system collects and analyzes users’ physical actions, such as touch, gestures, or other tangible behaviors. Simultaneously, it pays attention to interactions between users and interfaces, such as clicks, drags, or other operations on the screen. These physical inputs serve as part of the feedback to better understand users’ intentions and needs. On the other hand, perceptual inputs involve users’ awareness of space and interactions with virtual content. This may include information provided through virtual or augmented reality technologies and users’ perception of their location and orientation in the digital environment. This perceptual input helps create a more immersive working experience.
The output of the system is to integrate these inputs, creating immersive interactions and information mapping to provide context information. This could involve presenting virtual elements around the user, combining digital content with the physical environment to better support users’ workflows and tasks.
Essentially, compared with Figure 8, the Phygital workspace (Figure 10) developed according to the framework while leveraging spatial mapping and XR techniques. This innovative approach enables the dynamic transformation of the workspace, shifting effortlessly between 2D and 3D dimensions to immerse users in their environment and mitigate information overload and psychological isolation. Tailored to diverse work scenarios and XR technologies, the Phygital workspace systematically addresses key aspects such as team collaboration, office efficiency, and environmental sustainability. The culmination of these strategic efforts gives rise to the establishment of an XR-enabled Phygital workspace. Ongoing research endeavors will meticulously explore and validate the practicality and viability of this concept.
3 Carbon footprint calculation framework
The telework versus traditional workspace carbon footprint calculation framework (Figure 11) considers the reduction in travel-related emissions, varying with different work modes like co-working, fieldwork, and hybrid arrangements (Maillot et al., 2022). However, it also accounts for increased energy consumption associated with prolonged residential stays, encompassing additional annual hours spent at home and heightened energy use in areas like electricity, fossil-fuel usage for cooling, heating, refrigeration, and lighting (Hook et al., 2020).
The framework (Figure 12) for calculating the carbon footprint of the Phygital Workspace is built upon the telework spaces model. It involves subtracting the carbon footprint generated by hardware lifecycle and subsequently adding the reduction achieved through software enhancements in specific areas. This process aims to comprehensively assess the environmental impact of the phygital workspace, considering both the contributions of hardware and software to the overall carbon footprint.
Hardware lifecycle including the production, transport, use and end of life processing. Manufacturing XR devices (such as VR headsets, AR glasses, laptop, desktop and related components) involves extraction, production and transportation of raw materials, energy-intensive processes, and transportation. The carbon footprint associated with manufacturing contribute to the initial carbon footprint of XR utilities. Since 2021, Mata enables customers to purchase refurbished products for devices, including Quest 1 and Quest 2 (Meta, 2021). The introduction of refurbished products aligns with sustainability efforts, as refurbishing involves extending the lifespan of electronic devices, reducing the demand for new manufacturing, and lowering the overall environmental impact associated with production. The frequency and duration of using XR devices impact their overall energy consumption and, subsequently, the carbon footprint. For devices requiring regular charging, the energy source used contributes to the carbon footprint, but using renewable sources for charging can reduce this impact. Additionally, some XR devices consume power even in standby mode. Reducing standby power consumption is crucial for minimizing the overall energy footprint.
In the transportation category, we account for ground, air, and sea transportation, encompassing the journey from the manufacturing site to distribution hubs and ultimately reaching the customer.
Within the hardware usage, consumption is divided into two main components. Firstly, it includes the environmental impact generated by data centers and network infrastructure, along with the increased energy consumption resulting from the expansion of applications within our proposed phygital workspace. Processing and streaming content of XR applications rely on cloud-based services and data centers. Data centers’ energy consumption and carbon footprint are key factors affecting the overall carbon footprint of XR utilities. In the case of Mata, the data center buildings completed in 2022 have achieved a notable Power Usage Effectiveness (PUE) of 1.09. This PUE is impressive, being one-third lower than the industry average level. To achieving this, Mata employs an AI model to craft low-carbon mix designs for construction, utilizing byproducts like fly ash and slag as cement replacements (Meta, 2022). Additionally, recycled ground glass substitutes for cement in various concrete applications. Secondly, it considers the consumption associated with hardware itself, incorporating factors like power efficiency, annual usage hours, and the market share of each XR device. Energy-efficient hardware and optimized software can help reduce consumption. What’s more, developing XR content involves energy-intensive tasks like rendering and processing complex 3D graphics. High-quality content creation can lead to increased energy use, contributing to the carbon footprint.
4 Exploring the carbon footprint reduction mechanisms of XR-enabled workspaces (RQ2)
4.1 Reducing carbon footprint through XR in various work modes
4.1.1 Flexible workspace scenario
Co-Working: Some organizations use shared spaces, which bring together employees from various departments in a collaborative environment. Employees have flexibility in choosing their workspaces from various types of work areas, such as open spaces, quiet rooms, or meeting pods.
Shift Working: Some organizations, especially in manufacturing and healthcare, have employees working in shifts, utilizing the same workspace at different times.
Comparing with traditional workspace, these scenarios with more flexible work arrangements encourages telecommuting or selects energy-efficient co-working spaces can lead to considerable emissions reduction.
In co-working environments like retail, customer service, and education, VR facilitates a collaborative space for employees, customers, students, and teachers, transcending physical limitations. Through VR platforms, individuals can engage in virtual meetings, co-create in shared spaces, and foster teamwork, providing a seamless collaborative experience. Employees can virtually choose and personalize their workspaces, mirroring the flexibility offered by physical co-working environments. This not only promotes a sense of inclusivity but also accommodates diverse work preferences within a shared virtual space. XR technologies such as Horizon Workrooms seamlessly blend virtual reality (VR) content with real-world workspace elements through mixed-reality keyboard and desk tracking, improving workspace efficiency, reducing the need for physical paperwork and office space dedicated to file storage. Horizon Workrooms is accessible to a broad user base, while Spatial targets enterprises and offers features like lifelike avatars, immersive interactions, and seamless integrations with cross-platform tools, allowing users to join meetings from VR headsets, desktops, and smartphones (Figure 13). Integrations with tools like Slack and Google Drive further enhance workflow efficiency. These attributes synergistically contribute to an advanced collaborative ecosystem and serve as a foundation for achieving notable reductions in the carbon footprint linked to conventional workspaces and travel.
In shift working scenarios, particularly in industries like manufacturing and healthcare, VR can optimize workspace utilization. As employees work in shifts and share the same physical workspace at different times, VR offers a solution by creating a virtual representation of the workspace. This allows employees working in different shifts to access and interact with the same virtual workspace, maintaining continuity and collaboration. VR can also serve as a training tool for shift workers, offering immersive simulations and hands-on experiences to enhance their skills and adapt to varying work scenarios. In Horizon Workrooms, functions like the whiteboarding and idea pinning and note-taking within the VR environment empowers teams to brainstorm and collaborate visually, just as they would in physical spaces. Customizable avatars and spatial audio enhance the feeling of presence and personal connection, fostering more engaging virtual interactions.
In Design stage, Zaha Hadid Virtual Reality Group showcases a potential workflow of human designer-builders to create and exchange information seamlessly across both virtual and physical realities. By displaying user statistics, including total users, active users, sculpture volume, sculpting time elapsed, remaining time, and component count, highlights a groundbreaking design workflow. Each sculpting session accommodates up to four users in a virtual reality environment. They navigate freely, choosing, scaling, and placing components based on preferences and dynamic rules tied to the selected scale. This allows architects and designers to visualize and interact with 3D models in real-world environments. This reduces the need for physical prototypes, minimizing material waste and the environmental impact associated with traditional design iterations.
However, there are still limitations from user interaction, psychological, and collaboration perspectives related to this kind of VR workspaces. From the perspective of user interaction, users may face a cognitive load when transitioning between the virtual and physical world, especially when using traditional input devices like keyboards and mice. The need to visually confirm keystrokes or mouse positions in a virtual environment may disrupt the natural flow of work. The reliance on physical keyboards and mice can hinder the seamless integration of virtual and real-world interactions, potentially impacting user experience and productivity. What’s more, extended use of VR headsets may lead to discomfort, eye strain, and fatigue, impacting user wellbeing and concentration. This could result in decreased overall satisfaction and effectiveness of VR as a collaborative tool. Physical discomfort may deter users from engaging in collaborative activities, limiting the potential for extended and effective collaboration within virtual environments. VR headsets may also not be suitable for individuals with certain physical disabilities, leading to feelings of exclusion or frustration. Lack of accessibility features may impede the adoption of VR as a collaborative tool in diverse workplaces. Considerations for accessibility in the workplace are crucial to ensure that all team members can fully participate in collaborative efforts, promoting inclusivity and equal opportunities. The last but not least, VR applications and content may not seamlessly integrate with existing software and workflows, limiting interoperability. This can pose a challenge for collaborative projects that require a smooth transition between virtual and traditional tools. Difficulties in compatibility may disrupt the collaborative workflow, leading to delays, data transfer issues, or the need for additional training to bridge the gap between virtual and traditional work environments.
From the psychological side, the immersive nature of VR can create a sense of isolation from the physical environment, potentially impacting the psychological wellbeing of users. Lack of real-world interactions may lead to feelings of detachment or disconnection. In collaborative work environments, the isolation caused by VR may hinder spontaneous communication and impede the natural flow of teamwork, reducing the effectiveness of collaborative efforts.
4.1.2 Field work scenario
Employees, such as field technicians or sales representatives, are primarily working in the field, often traveling to different locations.
Field work often involve frequent travel to various locations, with air travel being a major contributor, which can lead to high carbon footprint. In contrast to Virtual Reality (VR), which immerses users in a wholly synthetic environment, Augmented Reality (AR) enriches the real world, keeping users in their context. This versatility makes AR applicable across various sectors, such as education, healthcare, military, retail, entertainment, and construction.
Within the retail sector, brands leverage AR to enhance online shopping, allowing consumers to visualize products in their real-world environments. In healthcare, AR aids in visualizing organs and simulating procedures, with surgeons using AR to project 3D representations of patient anatomy, improving accuracy and outcomes.
Furthermore, Augmented Reality (AR) presents a promising avenue for reducing carbon footprint in the construction industry. Its effectiveness lies in enabling remote collaboration and decision-making, thereby reducing the need for physical travel to construction sites. For instance, during the construction stage, an on-site job supervisor inspecting a heat duct can virtually bring a colleague into the scenario, viewable through a tablet or smartphone. Together, they can access a 3D model of an electrical duct scheduled for installation in the upcoming construction phase. Simultaneously, the avatars of the virtual teammate and the on-site supervisor can be used to mark up and annotate 3D models and existing structures as necessary. This application of AR not only enhances collaboration but also contributes to a more sustainable construction approach by minimizing travel-related carbon emissions.
Limitations arise in the scalability of AR solutions. While effective for small projects, their applicability diminishes in large-scale endeavors. For example, an AR system facilitating a small team in constructing a residential building may encounter difficulties when coordinating a multi-story commercial building project.
4.1.3 Hybrid work scenario
Teams are geographically dispersed, and members work together using digital communication tools without a central office. This scenario combines in-office and telework, allowing employees to split their time between the office and remote locations.
In the integrated XR workspace, incorporating both virtual and AI workflows, collaborative team environments are fostered. Team members engage in real-time interaction within a shared digital space, reducing the need for daily commutes and thereby decreasing the carbon footprint. This approach enables team members to work from their preferred locations, leading to a reduced demand for physical office space and associated energy consumption.
ShapesXR emerges as a transformative force in the realm of design and collaboration, particularly in the crucial early stages. It empowers diverse stakeholders, from scientific researchers to creative professionals, to shape their ideas in an immersive and efficient manner. By offering a virtual canvas where designers can conceptualize and iterate in a true-to-life scale, ShapesXR empowers teams to make informed decisions early on. This not only accelerates the product development cycle but also aids in ensuring that design choices are aligned with the desired outcomes. Furthermore, ShapesXR empowers Nano-Scale design and collaboration through spatial computing and 6-degree-of-freedom inputs. For instance, scientists could harness the platform’s capabilities to manipulate proteins in 3D space. With the ability to directly interact with and modify microscopic entities, ShapesXR bridges the gap between theory and practical application, minimizing the environmental impact of traditional laboratory work, promoting remote collaboration, and enhancing data analysis and modeling for sustainable solutions.
In comparison to ShapesXR, Omniverse stands out as a versatile platform with a broader scope of applications. Its integration with industries like architecture, engineering, construction, media and entertainment, manufacturing, supercomputing, and game development. While ShapesXR focuses on the early design phase and efficient collaboration, Omniverse extends its capabilities with AI-driven workflows (Figure 14) to encompass various stages of design, simulation, and creation across multiple sectors. This reduction in travel-related emissions aligns with sustainability goals, making both ShapesXR and Omniverse valuable tools to reduce the ecological impact of resource-intensive design, development, and creation.
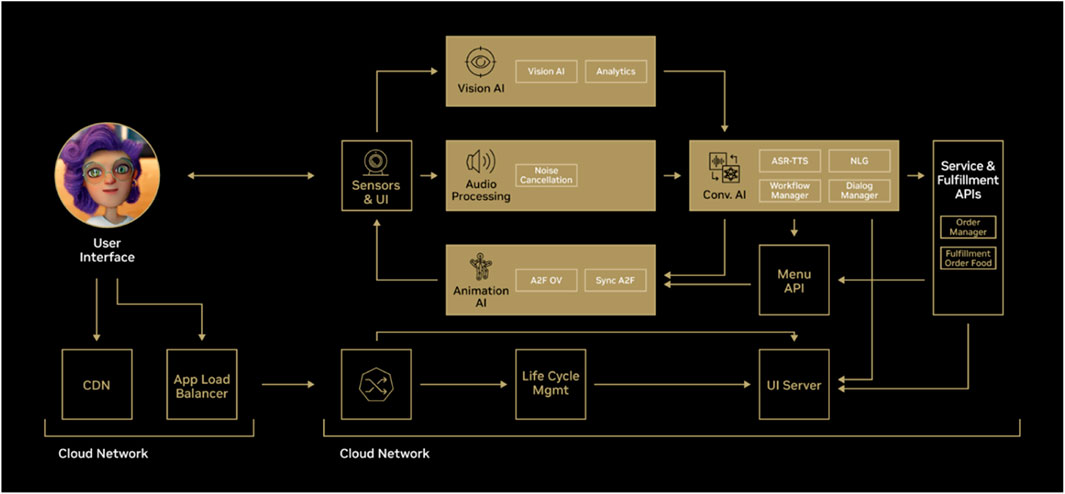
Figure 14. Example of an avatar AI workflow pipeline built with Omniverse ACE (Rubenstein and Qi, 2022). Reproduced with permission from Nvidia, original image: https://developer.nvidia.com/blog/building-cloud-native-ai-powered-avatars-with-nvidia-omniverse-ace/.
However, the effectiveness of the integrated XR system heavily relies on the quality and accuracy of AI algorithms. Inaccuracies or biases in these algorithms could impact the overall performance and decision-making capabilities of the system. Adhering to evolving regulations related to AI and XR technologies is crucial. Navigating compliance requirements, especially in industries with stringent regulations, may add complexity to the implementation and usage of integrated XR solutions.
4.2 Approaches: foster collaborative work environment
To foster a collaborative work environment, based on our previous case study analysis and literature research, we have identified three key directions within this approach. These include establishing clear communication channels within the team, implementing transparent decision-making processes, and facilitating knowledge sharing.
In the endeavor to establish transparent and efficient communication channels within a collaborative work environment, our system integrates three essential components: Task Tracker, Phygital Memo Board, and Mood Lighting. These elements are meticulously designed to enhance team communication, streamline task management, and create a dynamic and engaging workspace. The Task Tracker serves as the linchpin for effective task management. Its centralized platform enables team members to create, assign, and monitor tasks in real-time. This functionality fosters a shared understanding of project progress, ensuring that everyone is well-informed about the evolving dynamics of the tasks at hand. Furthermore, the Task Tracker acts as a communication hub, allowing team members to provide updates, share completion statuses, and relay pertinent information associated with each task. This transparency cultivates a collaborative atmosphere where the entire team is on the same page regarding project developments. Complementing the Task Tracker is the Phygital Memo Board, a digital canvas for collaborative notetaking and brainstorming. This feature enables team members to express ideas, draw diagrams, and share crucial information in a visually engaging manner. Real-time collaboration is facilitated, allowing multiple team members to contribute simultaneously. The Phygital Memo Board becomes a dynamic space for creative collaboration, enhancing the fluid exchange of ideas and fostering a culture of collective input. To add a subtle yet impactful layer to communication, Mood Lighting is incorporated into the system. Serving as a visual indicator, Mood Lighting reflects the team’s overall mood and workload. Different lighting modes can be associated with specific project statuses, providing an intuitive at-a-glance understanding of the team’s current situation. For instance, a green light may signify smooth progress, while a red light could indicate that urgent attention is required. This innovative status signaling system enhances communication efficiency without the need for constant notifications, creating a seamless and visually intuitive method of conveying information.
Transparent decision-making processes will be supported by AI-driven solutions integrated into the XR environment. AI algorithms are employed to analyze vast amounts of data generated by various facets of the collaborative workspace, ranging from project progress and team interactions to individual contributions. This data analysis process goes beyond human capabilities, identifying patterns, trends, and correlations that might not be immediately apparent to human decision-makers. Furthermore, the AI-driven solutions within the XR environment are designed to provide actionable insights. These insights act as decision support tools, offering valuable information to guide the decision-making process. For instance, the system might highlight potential bottlenecks, forecast project timelines, or identify areas where additional resources are needed. This augmentation of decision-makers’ capabilities with AI-driven insights ensures a more informed and effective decision-making process. Moreover, the XR environment provides a collaborative space where decision-makers can collectively explore and discuss the insights presented by AI-driven solutions. This shared virtual space promotes collaborative decision-making, allowing team members to contribute their perspectives, challenge assumptions, and collectively arrive at well-informed decisions.
In remote work scenarios, the online repository serves as a virtual meeting point for knowledge exchange, bridging communication gaps caused by limited face-to-face interactions. Whether team members operate in different time zones or prefer asynchronous communication, the repository ensures information availability when needed. This centralized hub for documents, information, and collaborative tools promotes accessibility and teamwork, particularly in remote work setups. In industries relying on 3D models, spatial computing, and 3D environments, integrating XR technologies into an online repository is crucial. Architects and construction professionals benefit from immersive experiences with 3D building designs. In engineering and manufacturing, precise visualization is achieved through interaction with 3D models, stored in the repository as detailed CAD models, schematics, and simulations. Healthcare professionals utilize XR-enhanced knowledge sharing for educational purposes, storing 3D models of anatomical structures and medical procedures. Real estate professionals leverage XR for immersive property walkthroughs, storing 3D models in the repository for remote collaboration and client presentations. In the gaming and entertainment industry, XR technologies play a pivotal role in creating and sharing 3D assets and virtual environments. The online repository becomes a central hub for collaborative work, enabling developers to collectively work in a shared 3D space.
By integrating gamification elements, such as regular updates and progress reports, into the system, we stimulate engagement and record participation. This approach motivates team members through the introduction of competitive or reward-based elements, enhancing the overall work experience and fostering a sense of accomplishment. Within the XR environment, a dedicated virtual space is designated to showcase professional achievements, completed projects, and milestones. Team members can seamlessly navigate this space, visually exploring and celebrating collective successes. Work-related challenges and competitions seamlessly become part of the XR environment, allowing teams to participate in virtual sprints or collaborative tasks within an immersive space. This integration enhances teamwork and boosts productivity. Additionally, XR-enhanced gamification introduces virtual certifications or badges for mastering new skills and completing training programs, adding a tangible dimension to professional development. Team members have the flexibility to personalize their XR workspaces with achievements, cultivating a motivating and visually appealing environment. This concise integration of gamification elements within the XR framework creates a holistic approach to stimulating engagement, fostering collaboration, and promoting continuous professional growth.
4.3 Approaches: enhance social interaction
In the pursuit of enhancing social interaction within XR environments, the strategy involves enriching interpersonal engagement through seamlessly integrated features. Avatars and other social elements heighten the sense of presence, while virtual team-building activities foster collaboration and camaraderie, enhancing overall team cohesion. The implementation of Virtual Office Hours further strengthens social dynamics by providing dedicated time for real-time communication, idea sharing, and questions. The approach extends to inclusive design elements like voice commands, gesture controls, and support for screen readers, ensuring accessibility for users with diverse needs. This multifaceted strategy creates a socially dynamic and inclusive environment within the XR framework, promoting seamless communication and interaction.
In a hybrid work setting, XR plays a pivotal role in enhancing virtual interactions, fostering a sense of presence and connection among employees. Virtual team-building activities overcome geographical barriers, fostering cohesion and strengthening relationships between remote and in-office team members.
In co-working environments, XR creates shared collaborative spaces where avatars and holograms contribute to a dynamic atmosphere, promoting creativity and innovation. Voice commands and gesture controls facilitate effortless interaction, fostering a sense of belonging among diverse co-working members.
For industries with shift work, XR-enabled virtual environments offer flexibility in collaboration, allowing shift workers to engage in virtual meetings and maintain social connections regardless of their working hours. Virtual office hours ensure accessibility and inclusivity, providing support to employees working different shifts.
In fieldwork scenarios, XR facilitates real-time collaboration, enabling fieldworkers in construction, research, or maintenance to connect virtually, share insights, and seek guidance through holographic representations. XR’s application in immersive training scenarios enhances efficiency and safety by preparing fieldworkers for diverse challenges.
4.4 Technical challenges and limitations
However, like any transformative innovation, XR introduces its set of limitations and challenges. Limitations inherent to the use of XR technology itself, which includes High Cost of Equipment, Maintenance Issues, Adoption Rates, and Content Development Challenges for effectively using this new technology, all of which can hinder productivity. The risk of data breaches and cyberattacks looms large, making it imperative to have robust security measures in place to safeguard valuable data, which is also related to the safety measures in social interaction stage.
The primary obstacle to the broad adoption of XR technologies is the considerable expense associated with the required equipment. VR headsets, AR glasses, and other hardware components can be financially burdensome, hindering accessibility. According to industry reports, the global AR and VR market size is expected to reach around 209 billion U.S. dollars by 2022, reflecting the substantial investment in these technologies (Thomas, 2023).
XR devices, being complex electronic equipment, are susceptible to wear and tear, technical glitches, and the need for regular updates or repairs. This necessitates ongoing maintenance efforts, adding to the overall cost. The global augmented reality market is projected to grow at a CAGR of 43.8% from 2021 to 2028, indicating both the challenges and opportunities in maintaining and upgrading XR devices (Mendoza-Ramírez et al., 2023).
The adoption of XR technologies is influenced by factors such as user familiarity, comfort, and acceptance of immersive experiences. Statistics reveal that in 2020, the number of XR devices shipped worldwide was around 60 million units (Thomas, 2023). However, achieving widespread adoption requires addressing user resistance and promoting the benefits of XR.
Creating engaging and immersive content for XR experiences demands specialized skills. The shortage of content developers proficient in XR technologies can be a bottleneck for growth. Reports suggest that the global market for AR and VR content is expected to reach over 50 billion U.S. dollars by 2026, highlighting the demand for quality XR content (marketsandmarkets, 2023).
To safeguard valuable data within XR systems, multiple security measures are employed. End-to-end encryption is implemented to secure the transmission of data between users and XR servers, ensuring that intercepted data remains unreadable without the decryption key. Strong user authentication mechanisms, such as multi-factor authentication (MFA), are incorporated to add an extra layer of protection by requiring users to verify their identity through multiple means. Secure storage practices are adopted for XR-related data, utilizing encrypted databases and secure file storage systems to protect stored data from unauthorized access. A comprehensive incident response plan is developed to effectively handle security incidents, encompassing procedures for detecting, reporting, and mitigating potential breaches. Regular backups of XR-related data are performed to prevent data loss in the event of a security incident, with secure and easily restorable backup processes. Additionally, strict adherence to relevant data protection regulations and industry standards, such as GDPR, HIPAA, or other applicable regulations, ensures a higher level of data protection.
5 Conclusion
In conclusion, this essay has delved into the pivotal role of Extended Reality (XR) technology in shaping sustainable work environments. The significance of XR technology lies not only in its capacity to enhance collaboration and productivity but also in its potential to significantly reduce the carbon footprint associated with traditional workspaces. The careful examination of factors such as energy consumption, hardware production, and end-of-life disposal has underscored the importance of a holistic perspective in the adoption of XR technologies. Balancing the benefits of enhanced virtual collaboration with the environmental implications is essential for responsible and sustainable implementation.
The research acknowledges certain limitations, primarily stemming from the novelty of the Phygital workspace concept emerging post-COVID-19, resulting in a scarcity of relevant literature and practical applications. Recognizing this constraint, we concede that a more comprehensive understanding of this emerging concept necessitates a wealth of practical data and experiential accumulation. In light of this, we have chosen to employ our own case study as the research subject for two principal reasons.
Firstly, our case study provides a tangible and controlled environment, allowing for an in-depth exploration of the practical application effects of the Phygital workspace within an authentic work setting. By observing and documenting the actual usage patterns within our team, we aim to gain a more holistic understanding of the advantages, challenges, and impacts across different dimensions of the Phygital workspace.
Secondly, opting for our own case study affords us the flexibility to design and adjust research methods to ensure a comprehensive examination of all facets of the Phygital workspace. Using our team as the research subject allows us to delve deeper into the nuances of Phygital workspace in actual work scenarios, providing more precise and specific data for the research. Therefore, while we acknowledge the inherent limitations, selecting our own case study is a strategic choice aimed at mitigating these constraints in practice and obtaining more substantive research outcomes.
In overcoming the limitations of the Phygital workspace, we are implementing a series of concrete methods and measures. Firstly, to validate the practical implementation and effects of each dimension, we will leverage existing case studies and conduct comprehensive evaluations by tracking real-world data, including the establishment of collaborative work environments and the enhancement of social interaction. This ensures the effectiveness of the Phygital workspace in actual work scenarios.
Secondly, to further validate the cultivation of collaborative work environments and the enhancement of social interaction, we will conduct a series of experiential workshops. These workshops will be designed based on different industry needs, team sizes, and durations to simulate authentic work environments. Through workshop implementation, we will collect participant feedback and observe actual effects, providing a more comprehensive understanding of the applicability of the Phygital workspace in various contexts.
Finally, to quantify the effects and gain deeper insights, we will conduct a survey. This survey will be divided into two parts, with the first part focusing on individual employees to gather their perspectives and experiences with the Phygital workspace. The second part will involve an evaluation by management, primarily concentrating on overall team performance and collaboration effectiveness. Through this series of methods and measures, we aim to comprehensively understand the practical application effects of the Phygital workspace and continually optimize its design and implementation. Specifically, we will focus on optimizing XR device usage policies, providing renewable energy solutions for data centers, and gaining a deeper understanding of the motivations and barriers for organizations adopting Phygital Workspaces throughout this process.
In the broader context, the adoption of Phygital Workspaces represents a paradigm shift towards a more environmentally conscious approach to work. As organizations increasingly seek to reduce their carbon footprint, the integration of XR technologies offers a promising avenue. By embracing the principles of sustainability and aligning them with the potential of XR, organizations can not only enhance their operational efficiency but also contribute to a more sustainable and eco-friendly future.
Author contributions
TLo: Funding acquisition, Supervision, Writing–review and editing. YC: Conceptualization, Methodology, Writing–original draft. TLa: Visualization, Writing–review and editing. AG: Writing–review and editing.
Funding
The author(s) declare that financial support was received for the research, authorship, and/or publication of this article. The work described in this paper was fully supported by a grant from the Hong Kong Polytechnic University (Project No. P0046262).
Conflict of interest
The authors declare that the research was conducted in the absence of any commercial or financial relationships that could be construed as a potential conflict of interest.
Publisher’s note
All claims expressed in this article are solely those of the authors and do not necessarily represent those of their affiliated organizations, or those of the publisher, the editors and the reviewers. Any product that may be evaluated in this article, or claim that may be made by its manufacturer, is not guaranteed or endorsed by the publisher.
References
Badiru, A., and Mertens, A. (2023). Poosible learning curve effects and the need for more analysis. Available at: https://www.dau.edu/sites/default/files/Migrate/DATLFiles/Mar-Apr_2023/Badiru_MarApr2023.pdf.
Badiru, A. B. (1994). Multifactor learning and forgetting models for productivity and performance analysis. Int. J. Hum. Factors Manuf. 4, 37–54. doi:10.1002/hfm.4530040105
Bobby Carlton (2020). Virtual work platform spatial now available free on all VR and AR devices. Available at: https://vrscout.com/news/virtual-work-place-spatial-free-oculus-quest/May 13, 2020).
Element Energy Limited (2022). Analysis of a net zero 2030 target for greater London. Available at: https://www.london.gov.uk/sites/default/files/nz2030_element_energy.pdf.
Elldér, E. (2020). Telework and daily travel: new evidence from Sweden. J. Transp. Geogr. 86, 102777. doi:10.1016/j.jtrangeo.2020.102777
Giovanis, E. (2018). The relationship between teleworking, traffic and air pollution. Atmos. Pollut. Res. 9, 1–14. doi:10.1016/J.APR.2017.06.004
Globally Cool, B. V., and Laszlo, K. (2022). The European market potential for hybrid workspace technology. October: CBI Ministry of Foreign Affairs. Available at: https://www.cbi.eu/market-information/outsourcing-itobpo/hybrid-workspace-technology/market-potential.
Golden, T. (2009). Applying technology to work: toward a better understanding of telework. Organ. Manag. J. 6, 241–250. doi:10.1057/omj.2009.33
Hill, E. J., Ferris, M., and Märtinson, V. (2003). Does it matter where you work? A comparison of how three work venues (traditional office, virtual office, and home office) influence aspects of work and personal/family life. J. Vocat. Behav. 63 (2), 220–241. doi:10.1016/S0001-8791(03)00042-3
Hook, A., Court, V., Sovacool, B. K., and Sorrell, S. (2020). A systematic review of the energy and climate impacts of teleworking. Environ. Res. Lett. 15, 093003. doi:10.1088/1748-9326/ab8a84
Maillot, A., Meyer, T., Prunier-Poulmaire, S., and Vayre, E. (2022) ‘A qualitative and longitudinal study on the impact of telework in times of COVID-19’, sustainability, null, p. null. doi:10.3390/su14148731
marketsandmarkets (2023). Augmented (AR) and virtual reality (VR) market size. Available at: https://www.marketsandmarkets.com/Market-Reports/augmented-reality-virtual-reality-market-1185.html.
McCain, M., Dowd, A., Salzer, D., Toothaker, E., and Xu, S. (2021). Business travel GHG emissions analysis: factors, tools, and cases for calculating GHG emissions and setting science-based targets for organizations. World Resour. Inst. doi:10.46830/wriwp.20.00086
Mendoza-Ramírez, C. E., Tudon-Martinez, J. C., Félix-Herrán, L. C., Lozoya-Santos, J. d. J., and Vargas-Martínez, A. (2023). Augmented reality: survey. Appl. Sci. 13 (18), 10491. doi:10.3390/app131810491
Nonaka, I., and Takeuchi, H. (1995). The knowledge-creating company: how japanese companies create the dynamics of innovation. New York: Oxford University Press.
Rafiq Elmansy (2021). The double diamond design thinking process and how to use i. Available at: https://www.designorate.com/the-double-diamond-design-thinking-process-and-how-to-use-it/.
Rauschnabel, P., Felix, R., Hinsch, C., Shahab, H., and Alt, F. (2022). What is XR? Towards a framework for augmented and virtual reality. Comput. Hum. Behav. 133, 107289. doi:10.1016/j.chb.2022.107289
Rubenstein, S., and Qi, A. (2022). Building cloud-native, AI-powered avatars with NVIDIA Omniverse ACE’, 21 september. Available at: https://developer.nvidia.com/blog/building-cloud-native-ai-powered-avatars-with-nvidia-omniverse-ace/.
Santos, R. S., and Pereira, S. D. S. (2023). For telework, please dial 7—qualitative study on the impacts of telework on the well-being of contact center employees during the COVID19 pandemic in Portugal. Adm. Sci. 13 (9), 207. doi:10.3390/admsci13090207
Sheryl, E. (2020). How to navigate the remote work learning curve during COVID-19’, March. Available at: https://www.hrdive.com/news/how-to-navigate-the-remote-work-learning-curve-during-covid-19/574389/.
The Department for Business Energy and Industrial Strategy (BEIS) (2022) ‘2022 government Greenhouse Gas conversion factors for company reporting’.
The U.S. National Household Travel Surveys (NHTS) (2024). The U.S. National Household travel surveys (NHTS). Available at: https://nhts.ornl.gov/.
Thomas, A. (2023). Extended reality (XR) market size worldwide from 2021 to 2026. Available at: https://www.statista.com/statistics/591181/global-augmented-virtual-reality-market-size/March 9, 2023).
Vaziri, H., Casper, W. J., Wayne, J. H., and Matthews, R. A. (2020). Changes to the work–family interface during the COVID-19 pandemic: examining predictors and implications using latent transition analysis. J. Appl. Psychol. 105 (10), 1073–1087. doi:10.1037/apl0000819
Vullinghs, J. T., De Hoogh, A. H. B., Den Hartog, D. N., and Boon, C. (2020). Ethical and passive leadership and their joint relationships with burnout via role clarity and role overload. J. Bus. Ethics 165 (4), 719–733. doi:10.1007/s10551-018-4084-y
Wang, H., and Zeng, W. (2019). Revealing urban carbon dioxide (CO2) emission characteristics and influencing mechanisms from the perspective of commuting. Sustainability 11 (2), 385. doi:10.3390/su11020385
Keywords: XR technologies, carbon footprint, work environment, commute, phygital workspace
Citation: Lo TTS, Chen Y, Lai TY and Goodman A (2024) Phygital workspace: a systematic review in developing a new typological work environment using XR technology to reduce the carbon footprint. Front. Built Environ. 10:1370423. doi: 10.3389/fbuil.2024.1370423
Received: 14 January 2024; Accepted: 12 April 2024;
Published: 19 July 2024.
Edited by:
Xi Deng, The University of Hong Kong, Hong Kong SAR, ChinaReviewed by:
Xiaoqiao Li, Hong Kong Metropolitan University, ChinaFangyu Cheng, Harbin Institute of Technology, China
Garvin Goepel, The University of Hong Kong, Hong Kong SAR, China
Copyright © 2024 Lo, Chen, Lai and Goodman. This is an open-access article distributed under the terms of the Creative Commons Attribution License (CC BY). The use, distribution or reproduction in other forums is permitted, provided the original author(s) and the copyright owner(s) are credited and that the original publication in this journal is cited, in accordance with accepted academic practice. No use, distribution or reproduction is permitted which does not comply with these terms.
*Correspondence: Yuting Chen, eXV0aW5nY2hlbjA0MDJAZ21haWwuY29t