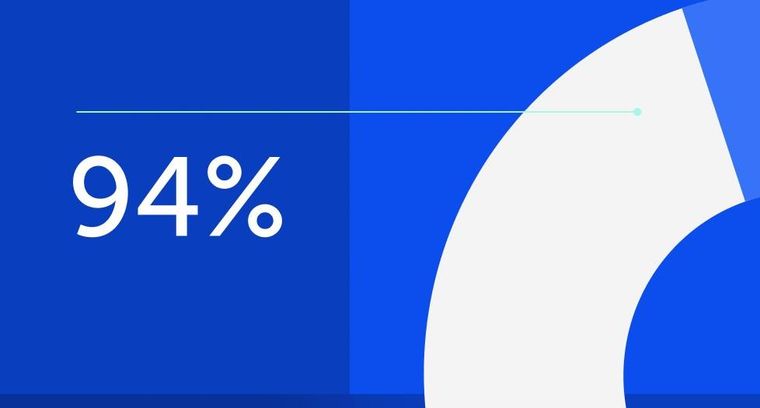
94% of researchers rate our articles as excellent or good
Learn more about the work of our research integrity team to safeguard the quality of each article we publish.
Find out more
ORIGINAL RESEARCH article
Front. Built Environ., 26 April 2024
Sec. Sustainable Design and Construction
Volume 10 - 2024 | https://doi.org/10.3389/fbuil.2024.1333146
This article is part of the Research TopicClimate Change and Urban ResilienceView all 7 articles
Introduction: As the world is engulfed with the growing impacts of climate change, the integration of climate adaptation measures into building performance requirements is essential. In the era of the fourth industrial revolution, smart buildings are expected to be the next frontier in the realm of building rating systems after sustainability-based one. Smart buildings can play a pivotal role in addressing the evolving challenges of changing climate due to their temporal and spatial cross-scale nature.
Methods: This study assesses the integration of climate hazard adaptation options within four prominent smart building rating systems (SBRS). Using a sectoral analysis approach and a 4-point Likert scale, we systematically evaluate the extent to which these rating systems incorporate climate adaptation measures directly or indirectly across multiple building sectors. We identify strengths and weaknesses in each system’s approach, highlighting areas where adaptation options are more profoundly addressed and sectors that require further attention.
Results: The evaluation results reveal variations in the comprehensiveness of climate adaptation integration among the smart building rating systems. The SRBS show a high level of integration of climate adaptation measures in the urban sectors intrinsically tied to the smart building paradigm, such as communication sector, and the human wellbeing and organization sector. Nevertheless, the study also revealed that SBRS almost universally fall short in covering other vital domains such as building envelope and structure, water and sanitation, and blue and green infrastructure.
Discussions: Complementing the SBRS with sustainability rating systems (GBRS) can effectively address the limitations in climate adaptation integration within SBRS. Moreover, the inherent interconnectedness of smart buildings with their surrounding infrastructure and the broader urban environment underscores the importance of the cross-scale consideration in the building rating domain in general and in climate related topics in particular, this interconnectedness also highlights a smart building’s reliance on its surrounding context for optimal functionality and the interdependency between the building and urban scale.
Human induced climate change is a global concern for the past 50 years. The scientific community has voiced the importance of collective action to fend off this threat (Leggett, 2020). Today, it seems that the climate change is an unavoidable challenge and a certain reality (Portner et al., 2022; UNEP, 2022). This is reflected in the latest report of the Intergovernmental Panel on Climate Change (IPCC) that concluded that the mean global temperature is likely to increase beyond the 1.5 C mark by 2040 even if all the promised GHG reductions of the 2015 Paris agreement are meet by 2030 (Legg, 2021). As a consequence, this alarming fact led the United Nations experts to rank climate change as the greatest existential threat to humanity (Christel, 2022).
One of the reasons for this grim prediction is related to the fact that over 60% of cities are already at risk to one or more natural disasters, and the number is constantly growing (Gu, 2019). This is particularly significant given the shift of human habitation from rural to urban areas over the last century (United Nations, Department of Economic and Social Affairs, Population Division, 2019). As a result, human settlements, urban environments, and cities are at the heart of this threat (Legg, 2021). Indeed, the built environment and cities occupy a unique position in climate challenge, being both a major contributor to the causes of climate change (Grubler and Fisk, 2012; Seto et al., 2014) and uniquely vulnerable to its impacts. Hence, the urban environment is placed at the forefront of the world’s efforts to combat and adapt to the looming changes in the Earth’s climate (Oroschakoff, 2019).
Local climate has historically been, and will continue to be, a central driving force in shaping the built environment. It significantly influences the design of our buildings and cities, and largely dictates their performance requirements. The building performance requirements and rating systems have evolved over thousands of years in response to technological, social, economic, and environmental factors into six distinct generations, starting from the historical generations such as 0.0 shelter,1.0 Safe and 2.0 Sanitary into the contemporary generations that appeared post-1970s −3.0 energy-efficient, 4.0 sustainable and 5.0 smart - each of which developed its unique path in handling and adapting to surrounding climate and requirements posed by different actors (Khoja and Danylenko, 2020).
Currently, the global building sector in general, and European one in particular, is in the midst of a complex transition from energy efficient and sustainable (3.0 and 4.0 generations) towards a sustainable and smart (4.0 and 5.0 generation) performance requirements. This transition is driven by a combination of various policies, regional and national energy and environmental goals, financial constraints, societal shifts, and technological changes. Moreover, the advancements of the 4.0 revolution, and fallout of the COVID-19 pandemic are shifting the building user expectations towards a more empathic and interactive buildings and put forward the immense potential of utilizing big data to enhance building performance as means to combat climate change (Khoja and Danylenko, 2020; Pishdad-Bozorgi and Zeng, 2022).
This transition towards sustainable and smart building stock is also observed at the EU policy level. The newly adopted amendments to Energy Performance of Buildings Directive (EPBD) in 2023 (EC, 2021; EP, 2023) and the EU taxonomy of 2020 (EU, 2020) are leading this transition. Beside that the existence of over 600 green building rating systems (GBRS) in the EU real estate market (Ebert et al., 2010) and the introduction of an EU-level rating system to standardize the EU GBRS (Sánchez Cordero et al., 2019) indicate that both the EU construction market and its policies are laying the groundwork for making sustainable fourth generation buildings a standard norm. At the other end, the recast of EPBD defines its objective §3 as to aid the EU transition towards smart cities. Therefore, it is noticed that since the EPBD recast of 2018, the EPBD acknowledges in many of its amendment articles the crucial role of “smart “technologies to support and complement the EU 2030 and 2050 objectives. The transition towards a “smart” built environment is even more evident in EPBD of 2023, as it expands requirements for buildings to include smart meters, smart girds, smart charging, automation and explicitly mentions ‘the Commission’s Smart Finance for Smart Buildings’ (§47) (EP, 2023). Moreover, it calls for the use of an EU-wide rating scheme - “smart readiness indicator” - which shall foster the transition to smarter buildings and “raise awareness amongst building owners and occupants of the value behind building automation” (EC, 2021).
While there is a growing recognition of the role of smart buildings in enhancing building resilience and the dual relationship between mitigation and adaptation offered by smart buildings, several critical aspects remain unclear. Notably, the specific building sectors (such as energy, water, envelope, etc.) that experience the most significant enhancement in adaptation through the integration of smart building performance requirements are not fully understood to date. Likewise, there is a lack of clear mapping regarding the types of climatic hazards (floods, storms, heatwaves, droughts, etc.) to which a smart-rated building might be better prepared to respond or accommodate.
Considering the significant political and market-driven shift towards integrating smart building features into the existing infrastructure, and building upon the research findings of Bai et al. (2020) and Felicioni et al. (2023) and the above marioneted researched gap in understanding the role of smart building rating systems in improving the building climate resilience. This understanding is needed to ensure that the next-generation of building performance requirements are not only environmentally friendly but also enhance resilience against climate-related challenges.
Therefore, this paper is investigating following research question: To what extent do smart building rating systems incorporate (directly or indirectly) measures that address and facilitate the adaptation of buildings to the emerging risks of climate change?
To answer this research question, this paper represents a descriptive based analysis aided by a numerical evaluation that assess the degree of integration of climate change adaptation options within four smart building rating systems (SBRS): the Smart Readiness Indicator (SRI), SmartScore, WiredScore, and the Ready2Services (R2S) label.
The indicators for each of the previously mentioned Smart Building Rating Systems (SBRS are organized based on the urban building sector (energy systems, water and wastewater systems, communication systems, etc.) and the climate hazards (heatwave, flood, hail, etc.) they address. The integration level of climate adaptation measures is evaluated for each sector-hazard combination using a simple 4-point Likert scale. This scale ranges from zero (0), indicating poor integration, to three (3), which signifies advanced integration of climate adaptation requirements in the system for a specific sector and hazard. A more detailed explanation of the assessment methodology is provided in Section 2.2.
The Smart Readiness Indicator (SRI) was first introduced in the 2018 revision EPBD 2018/844/EU to complement energy efficiency certification as a voluntary undefined EU scheme for rating the smart readiness of buildings. According to the EU Final report on the technical support to the development of a SRI, this option serves to ‘assess the technological readiness of buildings to interact with their occupants, to interact with connected energy grids and to operate more efficiently for buildings’ (EC, 2020; EC et al., 2020). The SRI is currently in its final phases of active testing and is expected to become a part of the new amendment of the EPBD. As of 2022 the SRI is being tested by the 6 member states (EC, 2022). Using the SRI rating, a building’s ability to exploit temporal and spatial cross-scaling synergies is assessed across nine technical domains and seven impact criteria. These domains encompass heating, domestic hot water (DHW), cooling, ventilation, lighting, dynamic building envelope, electricity, electric vehicle charging, and maintenance and control. Each domain in the SRI system is evaluated for its impact on energy efficiency, maintenance, comfort, convenience, health and wellbeing, information to occupants, and energy flexibility. The resulting assessment and score gained in each domain are then aggregated and presented as a weighted SRI score. This score reflects the building’s smart readiness compared to the maximum achievable smart readiness (EC et al., 2020).
The SmartScore rating system, introduced in 2013 by the New York-based company WiredScore, serves as an assessment tool for evaluating buildings based on the integration of smart systems that aim to enhance user experience, reduce costs, and promote sustainability. The current version, V2.1, is specifically designed to assess the “smartness” of office buildings. It evaluates the “smartness” through three main categories:
• User Functionality (UF): This category includes six subcategories and 33 indicators that assess how smart systems improve the building’s functionality for occupants.
• Technical Foundation (TF): TF consists of 22 indicators spread across six subcategories, evaluating the foundational technical elements that support smart systems.
• Innovation (IN): IN assesses the level of innovation in the building’s smart solutions.1
WiredScore defines itself as a digital connectivity certification system that rates the digital and the physical elements of the building to ensure that it is future-proof (Reeves et al., 2022). WiredScore offers a range of rating systems that can be used to assess new development of office buildings as well as existing ones. For the sake of consistency, only the WiredScore criteria for newly developed building is going to be examined in this paper. The WiredScore is composed of 7 domains hosting a total of 27 indicators, that are divided as follows: mobile and wireless connectivity with 6 indicators, building infrastructure-point of entry containing 3 indicators, building infrastructure—telecommunication room with 6 indicators, Building infrastructure-risers covered by 7 indicators, electrical resiliency represented with 2 indicators, access readiness assessed via 2 indicators and lastly digital connectivity innovation with 1 indicator2.
Lastly, the Ready2Services (R2S) label developed by Certivéa and the Smart Buildings Alliance (SBA) with 35 certified buildings is claimed to be the most widely used smart building rating system in Europe3. The R2S label is close in its objective to the WiredScore system. The R2S label focuses on the readiness of a building to accommodate a multitude of digital services, to make it adaptive, pleasant to live in and able to interact with its environment. The R2S label presents itself as preparing the building to complement a greater smart city or smart district development.
The comparison of the four SBRS presented in Table 1, provides insights about the focus area and evaluation topics of each system.
SBRS rating systems, much like Green Building Rating Systems (GRBS), were not initially designed with climate adaptation in mind. However, as both topics are inherently interlinked, this study aim to extend beyond aiding the understanding about the current state of climate adaptation in SBRS by identifying within each SBRS the urban sectors (such as energy, communication, and water) and climatic hazards where adaptation consideration are either well-integrated or in need of further development.
In this paper the extent to which each of the four investigated SBRS incorporate climate adaptation measures directly or indirectly across multiple urban sectors and climate hazards is assessed using a simple 4-point Likert scale. The 4-point scale ranges from zero (0) denoting a poor integration to three (3) points signifying advanced integration of climate adaptation requirement in the analyzed system for a certain sector and climate hazard. A more detailed explanation about the assessment methodology is provided further in Section 2.2.
By mapping the landscape of climate adaptation integration within smart building ratings, this research contributes to the ongoing discourse on climate-responsive building design and serves as a foundation for future advancements in sustainable and resilient construction practices.
The buildings performance requirements are put forward by multiple actors, staring from users (owners, occupants and public), economic actors and market participants (contractors, insurance companies, investors etc.) as well as the regulator (government, authorities, regulatory frameworks). Regulators in a growing number of countries are integrating performance-based regulations in their building codes (Lützkendorf et al., 2005). Throughout history the regulator bodies of cities drafted written rules or agreed on social norms that address the organization and functionality of the buildings in the city. The formalization process of the performance requirements has evolved over time into an adaptive process that integrates several cross-cutting topics from safety and security to sanitary, comfort, sustainability. Figure 1 illustrates the evolution of building performance requirements from historical to current generations. It is to be noted that this evolution has resulted in the formulation of six distinct generations of building performance requirements, with each subsequent generation advancing upon the foundation established by its predecessor in an additive and hierarchical manner (Khoja and Danylenko, 2020). These generations of performance requirements can be generally divided into two main groups: historical and current. The historical group encompasses the generations of building performance requirements that prevailed until about 1970 A.D.
Figure 1. The evolution of buildings. Adapted from (Khoja and Danylenko, 2020).
This journey begins with the 0.0 generation known as ‘shelter,’ where the primary design performance criterion was the provision of a shelter in its broader sense. As cities were established and written laws introduced, the 1.0 generation ‘safe’ emerged, expanding the original shelter functions by incorporating design requirements that addressed structural integrity and, subsequently, fire safety in buildings.
With the onset of the industrial revolution and increasing recognition within medical spheres regarding the impact of sanitary and ventilation systems on occupant health, a new era emerged. Sanitary requirements began to emerge in conjunction with the introduction of formal building codes, giving rise to the 2.0 generation of building requirements - a “sanitary building”. The energy crises of 1970s led to the inclusion of formal energy requirement supplemented with rating systems, consequently resulting into the creation of the 3.0 energy-efficient generation.
By the 1990s with the introduction of BREEAM system in the United Kingdom and the global recognition of urgent need for a sustainable development path, the 4.0 generation (sustainable) was born. Today it can be safe to say that sustainability budling requirements are becoming the new mainstream building generation in the EU with over 600 GRBS in the marketplace (Ebert et al., 2010), integration of the sustainability certification requirements in many national building codes (Khoja et al., 2021) and their partial integration in the EU EPBD of 2018 and 2023.
The 4.0 industrial revolution combined with the experience gained from the COVID –19 pandemic accelerated the formal establishment of the 5.0 generation of buildings—a “smart” building as illustrated in Figure 1.
A deeper dive into this 5.0 generation of buildings reveals that modern smart buildings trace their origins to the intelligent buildings of the 1980s (Buckman et al., 2014). While the intelligent buildings of the 1980s were generally reactive in nature, the new smart buildings are proactive. They harness extensive internal and external data collected through sensors and internet connectivity to anticipate and prepare for events in the immediate or foreseeable future (Buckman et al., 2014). At the same time, a building intelligence should not be confused merely with a building automation as the “smart” buildings offer much wider benefits besides safety, time and cost saving, expanding to health and inhabitants comfort (Batov, 2015). Moreover, smart buildings are capable of leveraging temporal and spatial scales that extends well beyond their physical boundaries to optimize the performance of both individual and surrounding buildings (Khoja, 2022). With the implementation of automation technologies and utilization of Information and Communication Technologies (ICT), it becomes feasible to harness the advantages of automated decision-making almost instantly, which can improve buildings’ sustainability performance. The continuous influx of data from smart-enabled active building systems, such as lighting, heating, ventilation, and cooling (HVAC), as well as renewable energy systems, enables real-time adjustments to the performance parameters of each system. Smart buildings have been proven to be an economically viable climate mitigation option due to their ability to take advantage of temporal and spatial scales that extend far beyond the building user and the building physical boundary (Janhunen et al., 2020; Khoja, 2022). However, it is important to note that bypassing the integration of passive and holistic sustainability elements—essentially leaping from the third generation focus on energy efficiency directly to fifth generation smart building—can paradoxically lead to poorer energy and economic outcomes. This is due to the high energy demands of smart building sensors and their potential for malfunction (Amin et al., 2017). Therefore, the appropriateness of labeling such buildings as “smart” is questionable when they overlook holistic sustainable design principles. Smart buildings, as we understand them, should build upon the principles of sustainability, and improve the overall building performance and user satisfaction. The benefits of such approach are confirmed in the study of Pannier et al. (2022) which showed that the inclusion of both passive and active elements can achieve a superior energy saving over the full life cycle of the building than just by including the passive elements alone. This can be explained by the building’s ability to integrate various temporal scales, adjusting its performance accordingly as illustrated in Figure 2.
The studies mentioned above primarily address the contributions of smart buildings to general climate mitigation efforts. However, when it comes to enhancing climate adaptation, there appears to be a limited understanding of the extent to which Smart Building Rating Systems (SBRS) integrate climate adaptation considerations into their frameworks. As we stand on the brink of the smart building era, it is essential to understand the degree to which existing SBRS integrate the climate adaptation in order to ensure that the next-generation of building performance requirements are not only environmentally friendly but also enhance resilience against climate-related challenges.
The unique problem that climate change impacts bring with it, is that they will affect a broader spectrum of the built environment physical and social sectors that are highly interrelated (Revi et al., 2017). Thus, for the adaptation measures to be truly successful they need to be equally holistic incorporating many sectoral, spatial, and temporal scales. This holistic perspective allows the built environment to function as a unified and interconnected system (Khoja et al., 2022). Interestingly, this cross-scale and cross-sectoral consideration is also a critical and distinctive enabling factor of “smart” buildings. This broader context is essential for both end-users and policymakers to fully leverage the advantages of smart buildings. In essence, a smart building’s intelligence is closely tied to its ability to take advantage of the cross physical and cross temporal exchange of information. Thus, a smart building must be always viewed within the greater urban context it interacts with.
In terms of urban climate adaptation, the IPCC AR5 report highlighted seven sectors in which adaptation measures are a priority for the urban areas. Nearly all key urban sectors identified by the IPCC as susceptible to climate change impacts are either located within or intricately linked to the building part thud being an integral part the built environment. Buildings, in fact, often serve as either origins or destinations for various sectors including water, energy, communication and transportation networks.
Accordingly, the assessment of the integration of the climate adaptation in the SRBS is made for the following sectors seven: Structures (envelope), Water, Wastewater and Sanitation Systems, Green and Blue Infrastructure, Energy Systems, Transportation and Mobility, Communication Systems, Human Wellbeing, and Organization. The rational behind selections of these sectors is twofold:
Firstly, it allow to align the building with the key urban sectors identified by the IPCC as vulnerable to climate impacts (Revi et al., 2017). Secondly, in recognition to the fact that the smart building is to be part of greater smart urban context, the chosen sectors represent elements of urban infrastructure that are interconnected and shared, either physically or virtually, across urban scales (building, neighbourhood, and city). These include energy systems, water and wastewater systems, communication systems, transportation, and aspects of human wellbeing. Even if the traditional planning views these sectors at each scale separately, in reality however, that these sectors do not operate in isolation but are part of an integrated urban system. Thus, the essence of this sectoral approach is to contribute to more holistic consideration of climate resilient urban environment and allow for the urban environment to be viewed as an interlocked entity.
For each of the seven sectors, the assessment of the incorporation of climate adaptation into each of the four smart building rating systems is measured using a simple 4-point ordinal scale, ranging from zero (0) to three (3) points as illustrated in Table 2. The justification for the assignment of the rating score is made based on the available peer reviewed publications as demonstrated in the example provided in Table 4.
Table 2. Scoring methodology to assess the degree of adaptation to climate change in the four investigated SRBS.
The choice of using a 4-point scale assessment is three-fold:
I. Simplicity and Comprehensive Overview: The objective of the study is to provide a simple, yet comprehensive overview about the degree of inclusion of climate adaptation in each system. By employing this numerical scale, we establish a balanced framework that enables easy and meaningful comparisons between different smart building rating systems (SRBS). This facilitates a deeper understanding of how various climate risks are considered by each system.
II. Detailed Comparison: The choice of a 4-point scale deemed well suited to capture subtle differences in the level of climate adaptation integration within each SBRS allowing to go beyond highlighting the mere presence or absence of climate adaptation strategies but the extent and manner of their incorporation.
III. Flexibility for Future Updates: As the field of knowledge and technology continues to evolve, so will the criteria and indicators for SRBS. The 4-point scale assessment enables accommodating such changes and allowing future adjustments or refinements of the results based on emerging information or revised criteria in smart building rating systems.
As the adaptation reactions overlap and can serve to enhance the reliance against one or more hazard, the assessment is conducted separately for each hazard taking into consideration either individual or grouped performance requirements that address the hazard. Hence, double counting for the same hazard is not permitted and is only possible if the indicator or group of indicators address two separate hazards. For the indicators that address the same hazard, the results will be based on the indicator that is assigned the highest score, i.e. (3) three points without aggregation. For the sectors of in which there is no clear relation between the sector and a hazard, such as the lack of relation between the climate impact of drought and transport sector, this point is excluded from the total score and is denoted with n/a. Finally, for each climate hazard, the assessment is made for the key urban sector that such energy, water, communication systems, etc. that is addressed by a single or a group of performance requirements.
Accordingly, the scoring matrix for each hazard and sector is represented in Table 3.
The example provided in Table 4 and illustrated in Figure 3 shows the application of the rating methodology to evaluate a degree of inclusion of adaptation requirements to the climate impact of drought in the SmartScore rating system.
Table 4. Evaluation of the inclusion of adaptation requirements in the SmartScore rating system to the impact of drought.
Figure 3. A schematic showing an example application of the methodology for assessing the integration of drought adaptation in the SmartScore rating system across the sectors.
The evaluation of the SRI indicators reveals a clear focus on optimizing energy consumption through a mix of energy monitoring, fault detection, energy demand forecasting, and communication with the external grid. Consequently, the SRI heavily relies on active system adaptation, which has its own inherent vulnerabilities in terms of climate adaptation and most of achieved points can be attributed to the dual benefit effect. This is more apparent in the system’s good performance against the hazard of heatwaves and in the energy sector, owing to the SRI system’s focus on renewable energy generation and smart grid integration. However, these considerations fall short in providing meaningful requirements that addresses other the climate change impact areas and key urban sectors, such as drought, transport, and blue and green infrastructure sectors where the system failed to collect any points. In total the SRI system collected 20 out of the possible 90 points. The detailed assessment of the system is provided in the attached Supplementary Material.
Like the SRI system, the SmartScore shows a tendency towards the use of active systems to enhance building adaptation and resiliency. Nevertheless, the evaluation of SmartScore system shows a balanced performance of the system across almost all sectors and hazards. However, both the transportation and mobility sector and the blue and green infrastructure are not addressed. The evaluation of all other sectors and climatic hazards yielded at least a single point. The main driver behind this result can be attributed to the system’s original focus on the communication sector and on the interaction between the building system and the end user. In total, the SmartScore system was assigned 27 out of a possible 90 points.
The WiredScore system focuses primarily on the communication and energy sectors, which may be justified given the nature of the rating system. This might explain the high similarity in the points achieved by both the WiredScore and SRI system. According to the conducted evaluation both systems achieved the exact same number of points in all sectors except for the drought, where the WiredScore was awarded a single point. This single point was awarded due to a dual benefit effect: as system focuses on ensuring reliable reception of mobile signals across the building, users can theoretically receive an early warning about possible drought, which is a strategy that is widely used today in many African countries (Calvel et al., 2020; Gwimbi and Siimane, 2021). However, when viewing the evaluation from the sectors point of view, the more differences become apparent between the WiredScore and SRI as well as other reviewed systems. The WiredScore was awarded points in only three out of the total seven sectors, namely the communication systems sector, the energy sector and the human wellbeing and organization sector. In total the WiredScore achieved 21 of the possible 90 points.
The stated goal of the R2S label is to equip certified buildings to be adaptive, pleasant to inhabit, and capable of interacting with their wider environment as an integral part of a Smart City. The R2S system covers six themes: one, titled ‘Services,’ is dedicated to the occupants and the building and contains a single category; two themes address governance, namely ‘Digital Security’ and ‘Responsible Management,’ the latter of which contains nine categories. The final three themes - ‘Connectivity,’ ‘Network Architecture,’ and ‘Equipment and Interfaces’ - focus on technical principles and collectively comprise eleven categories.
Despite the extensive catalogue of indicators of the R2S system, was awarded only 13 points out of the total possible 90 points, which represents the fewest points among all the examined rating systems. The R2S system heavily focuses on the communication sector, similarly to the WiredScore. However, the system neglects the impact of heatwaves on communication systems and lacks the effective combination of passive and active strategies to reduce vulnerability to climate change-related impacts.
The comparison of results among the four smart building rating systems are summarized in Table 5. The table shows the hazards and sectors in which each system either showed a strong or weak inclusion of climate adaptation topics. The detailed evaluation of the four SBRS across sectors, hazards along with the justification for each attained score can be found in Supplementary Material.
In a summary and from a sectoral perspective, as illustrated in Figure 4, all investigated systems incorporate performance requirements that address climate adaptation in the communication and human wellbeing and organization sectors. Notably, none of the systems scored less than 4 out of the possible 12 points in both sectors. WiredScore stands out as the only system that achieved the maximum points in the communication sector, while SmartScore earned the highest score of 9 points in the human wellbeing and organization sector. Regarding the energy sector, the SRI, SmartScore, and WiredScore systems demonstrated overall good incorporation of climate adaptation measures, with SRI leading the group. In contrast, R2S received 0 points in this sector, indicating a lack of consideration.
This brings us to the shortcomings shared between all the four systems. Firstly, it is observed that none of the systems has indicators in place addresses the climate adaptation of the green and blue infrastructure sector, as well as the transportation and mobility sector. Similarly, the water and sanitation sector has been barely addressed in the SmartScore system and is absent in all other systems. In regard to the structure and building envelope sector, the picture is rather mixed, with SmartScore and SRI showing good integration of adaptation provisions, while the other two systems illustrating poor results.
When viewed from a climate hazard perspective, as summarized in Figure 5, an analysis of the four smart building rating systems reveals noteworthy strengths and shared deficiencies. It is fair to say that all four systems showed relatively consistent though relatively low integration of provisions addressing the climatic hazards of flooding, heavy precipitation, storm and wind hazards, and heatwaves, with scores ranging from 3 to 6 points. SmartScore, being the best performing among the 4. Scoring consistently 6 points in all the aforementioned hazards.
In contrast, when it comes to addressing the climatic impact of drought, all systems showed a lower level of integration, with scores ranging from 0 to 3 points. SmartScore scored the highest in this category, followed by WiredScore and R2S, each receiving 1 point, while SRI received no points.
In summary, each of the four systems exhibits strengths aligned with its initial mission such as in the areas of connectivity, energy, or end-user, the comparison showed also significant gaps in addressing the full spectrum of climate hazards or urban sectors. In our view several factors contribute to this neglect of climate adaption into the existing SBRS. For one, the relatively recent emergence and inherent complexity of climate change adaptation and resilience issues, compared to mitigation measures. This is often compounded by the localized nature of these measures in contrast to the global mitigation action. Moreover, most SRBS are very specialized and focus on niche areas that are not yet widely implemented in the mainstream buildings. Lastly, the existing building planning process still views a building as a separate entity within the greater urban context. At the same time, smart buildings are not isolated and are designed to take advantage of the cross-scale information exchange between the building and its context, which can ultimately improve the resilience of the individual buildings as well as the collective urban system. Realizing this requires a fundamental shift in the planning paradigm (Khoja, 2022).
In the face of the escalating global climate crisis and the growing vulnerability of urban areas to climate-related hazards, there is an urgent need to assess the integration of climate adaptation measures within the existing building performance rating systems. Buildings and urban environments are expected to operate under new climate conditions they might not be well prepared against. This task has become even more pressing due to the limited availability of an operational climate resiliency rating system in the market (Felicioni et al., 2023). Against this backdrop and the clear shift in both policy and market domains towards incorporating smart building rating systems, this paper has undertaken the crucial task of examining the extent to which four prominent smart building rating systems—Smart Readiness Indicator (SRI), SmartScore, WiredScore, and Ready2Services (R2S)—incorporate climate adaptation provisions.
The simple numerical assessment made in this paper has addressed a critical research gap about the degree of integration of climate adaptation measures within smart building rating systems. It has also brought to the forefront the strengths and weaknesses inherent in each system’s approach. Additionally, it has pinpointed sectors where adaptation options are effectively addressed and those that demand further attention.
The findings reveal that the urban sectors intrinsically tied to the smart building paradigm, such as communication sector, and the human wellbeing and organization sector showed a good level of adaptation integration. Significantly, none of these systems scored less than 4 out of a possible 12 points in these sectors. WiredScore particularly distinguishes itself by achieving the maximum points in the communication sector, while SmartScore excels with the highest score of 9 points in the human wellbeing and organization sector. Nevertheless, the study also revealed that SBRS almost universally fall short in covering other vital domains such as building envelope and structure, water and sanitation, transportation, and mobility, as well as blue and green infrastructure. This can be attributed to the fact that these sectors are typically part of the conventional and not “smart” aspects of buildings. This reveals the shortcoming of a narrow focus and definition of SBRS. This underscores the need for urban planners and policymakers to broaden the SBRS scope to include these traditional sectors, ensuring a comprehensive urban strategy.
Moreover, it is intriguing to note that, from a climatic hazard perspective, the climatic impact of drought is scarcely addressed by all the reviewed systems. This deficiency may be attributed to the origins of these systems as they stem from regions historically unaffected by water shortages. These observations point out the limited geographical and thematic scope of existing smart building rating systems. This highlights the opportunity for policy reforms that mandate the inclusion of drought resilience measures in all building rating systems, regardless of their geographical origins:
Moreover, overcoming these shortcomings can be achieved when sustainability rating systems are applied in conjunction with smart building rating systems. The findings of Felicioni et al. (2023) has showed that sustainable rating systems explicitly address among other the water efficiency, site ecology as well as the transportation system. These are those sectors where the reviewed smart building rating systems failed. This fact demonstrates the possible advantage of combining more generation of building systems with each other and raises a fundamental question about the extent to which a building can genuinely be labeled as “smart” if it fails to meet the basic prerequisites of sustainable building requirements.
It is observed that current building rating assessment methods are effective in delivering high-performance buildings within specific domains, such as energy efficiency, smart technology integration, and sustainability. However, they often lack clarity in illustrating how a high rating in one domain might impact performance in other areas. This situation highlights the need for an umbrella rating system. Such a system would integrate these rating systems, allowing for a more comprehensive understanding and translation of performance scores from one domain to another. This holistic approach would provide a clearer picture of a building’s overall performance across various dimensions. Nevertheless, enforcing such rating system must carefully weigh the trade-offs between enhancing building performance and the potential increase in construction costs and design complexity (Academies et al., 2012). However, in terms of the climate adaptation, the American National Institute of Building Sciences’, has showed that for every $1 spent improving on building climate resilience beyond the provisions outlined in the 2015 International Codes (I-Codes), $4 can be saved in return (Council, 2018).
The interconnectedness of smart buildings with their surrounding infrastructure and the broader urban context emphasizes the significant potential of a smart building for adapting to climate impacts. However, it also reveals the dependence of a smart building on the surrounding context for its true functionality. A smart building may be as smart and sustainable as the surrounding environment permits. Thus, the availability of the fundamental prerequisites of a smart city plays a pivotal role in realizing the full potential of smart buildings in climate adaptation efforts. This also raises the importance of the cross-scale consideration in the building rating domain in general and in climate related topics in particular. This is particularly important because mitigation measures and adaptation actions can be complementary, leading to improved building performance across both areas (Munasinghe, 2001; IPCC, 2007; Raven et al., 2018; Schünemann et al., 2020). However, these dual benefits are not always fully realized. In fact, if not carefully considered, they can result in maladaptation or underperforming mitigation efforts (Scheraga and Grambsch, 1998; Rosenzweig et al., 2018). It can be argued that the complexity, differing objectives, the local vs. the global nature of mitigation approach restrict the full realization of their combined benefits (Metz et al., 2007). The complexity of accurately assessing building resilience is aggravated by the lack of a standardized evaluation framework. This absence renders the interpretation of resilience scores ambiguous, particularly when contrasted with the well-established methods used for measuring progress toward climate mitigation goals (Khoja et al., 2022). This argues the importance of developing a standardised framework for assessing the building vulnerability and resilience to climate risk. The (ISO 14091:2021 Adaptation to climate change) offers a good starting point for creating such a framework. This standard, being part of the ISO 14000 series, could be adapted and tailored specifically for urban environments (Khoja, 2024). Beyond the necessity of a framework, there is an urgent need to shift towards a holistic, multidimensional, and circular planning process that can bridge the temporal and spatial gaps inherent in current design practices. By doing so, planners and users can fully exploit the temporal and spatial cross-scale benefits offered by sustainable building design and smart building systems (Khoja, 2022). Such a shift would not only help enhance the resilience and sustainability of buildings but also ensure they are acting as interlocked, connected urban system.
Our perspective argues that a smart building, as the next evolutionary and complementary step in the building requitements that builds upon and expand beyond the energy-efficient and sustainable buildings, offer temporal and spatial advantages that allow the built environment to work as a holistic system. This approach provides policymakers and urban planners with effective tools to address climate change through multilevel governance and to treat the whole urban environment as a single entity. New research goals could focus on the standardization of SRBS, drawing from successful international models such as Germany’s Quality Seal for Sustainable Buildings (QNG), and exploring the financial mechanisms that could support such initiatives. Additionally, funding programs and financial incentives could accelerate the renovation of existing buildings with such smart technologies. While smart homes offer environmental, economic, and social benefits, it is crucial to address the significant security and data protection risks associated with these systems. The research by scholars like Ali and Awad (2018) and Brooks et al. (2020) discus these concerns in depth and suggests measures to mitigate them. The Smart building systems’ overreliance on active systems that require a constant information and energy feed make them also very vulnerable single system failures that could trigger catastrophic cascading risks. Balancing these active systems with passive ones is essential to overcome vulnerabilities. This advocates researching how the smart buildings can be completed with a nature-integrated architectural practices, in which our communities act “with” rather than “despite” it. The “Vernomimicry” approach suggested by Khoja and Waheeb (2020) offers a promising concept for developing contemporary urban adaptations that harmoniously align with nature’s principles.
The original contributions presented in the study are included in the article/Supplementary Material, further inquiries can be directed to the corresponding author.
AK: Visualization, Writing–original draft, Methodology, Investigation, Data curation, Conceptualization. OD: Writing–review and editing, Methodology.
The author(s) declare that financial support was received for the research, authorship, and/or publication of this article. This work was financially supported by the Munich University of Applied Sciences HM and the Deutsche Forschungsgemeinschaft (DFG, German Research Foundation)–Projectnumber 512819356.
The authors declare that the research was conducted in the absence of any commercial or financial relationships that could be construed as a potential conflict of interest.
All claims expressed in this article are solely those of the authors and do not necessarily represent those of their affiliated organizations, or those of the publisher, the editors and the reviewers. Any product that may be evaluated in this article, or claim that may be made by its manufacturer, is not guaranteed or endorsed by the publisher.
The Supplementary Material for this article can be found online at: https://www.frontiersin.org/articles/10.3389/fbuil.2024.1333146/full#supplementary-material
1https://wiredscore.com/certify-a-building/wiredscore/wiredscore-home/.
2https://wiredscore.com/certify-a-building/smartscore/.
3https://certivea.fr/certifications/label-r2s/.
Ali, B., and Awad, A. I. (2018). Cyber and physical security vulnerability assessment for IoT-based smart homes. sensors 18, 817. doi:10.3390/s18030817
Amin, U., Hossain, M., Lu, J., and Fernandez, E. (2017). Performance analysis of an experimental smart building: expectations and outcomes. Energy 135, 740–753. doi:10.1016/j.energy.2017.06.149
Bai, C., Dallasega, P., Orzes, G., and Sarkis, J. (2020). Industry 4.0 technologies assessment: a sustainability perspective. Int. J. Prod. Econ. 229, 107776–107815. doi:10.1016/j.ijpe.2020.107776
Batov, E. I. (2015). The distinctive features of “smart” buildings. Procedia Eng. 111, 103–107. doi:10.1016/j.proeng.2015.07.061
Brooks, D. J., Coole, M., and Haskell-Dowland, P. (2020). Intelligent building systems: security and facility professionals’ understanding of system threats, vulnerabilities and mitigation practice. Secur. J. 33, 244–265. doi:10.1057/s41284-019-00183-9
Buckman, A. H., Mayfield, M. & B. M., and Beck, S. (2014). What is a smart building? Smart Sustain. Built Environ. 3, 92–109. doi:10.1108/sasbe-01-2014-0003
Calvel, A., Werner, M., van Den Homberg, M., Cabrera Flamini, A., Streefkerk, I., Mittal, N., et al. (2020). Communication structures and decision making cues and criteria to support effective drought warning in Central Malawi. Front. Clim. 2, 578327. doi:10.3389/fclim.2020.578327
Christel, M. (2022). Climate change the greatest threat the world has ever faced, UN expert warns. Office High Comm. Hum. Rights. Available at: https://www.ohchr.org/en/press-releases/2022/10/climate-change-greatest-threat-world-has-ever-faced-un-expert-warns.
Ebert, T., EßIG, N., and Hauser, G. (2010). Zertifizierungssysteme für Gebäude: Nachhaltigkeit bewerten-Internationaler Systemvergleich-Zertifizierung und Ökonomie, KG. Germany: DETAIL-Institut für internationale Architektur-Dokumentation GmbH & Co. doi:10.11129/detail.9783955530143
EC (2020). “Commission Implementing Regulation (Eu) detailing the technical modalities for the effective implementation of an optional common Union scheme for rating the smart readiness of buildings C/2020/6929,” in EC.
EC Verbeke, S., Aerts, D., Reynders, G., Ma, Y., and Waide, P. (2020). Final report on the technical support to the development of a smart readiness indicator for buildings – final report. Brussels: Publications Office.
EC (2021). Proposal for a DIRECTIVE OF THE EUROPEAN PARLIAMENT AND OF THE COUNCIL on the energy performance of buildings (recast) COM/2021/802 final.
EP (2023). Amendments adopted by the European Parliament on 14 March 2023 on the proposal for a directive of the European Parliament and of the Council on the energy performance of buildings (recast). COM(2021)0802 – C9-0469/2021 – 2021/0426(COD)).
EU (2020). Regulation (EU) 2020/852 of the European Parliament and of the Council of 18 June 2020 on the establishment of a framework to facilitate sustainable investment, and amending Regulation (EU) 2019/2088.
Felicioni, L., Lupíšek, A., and Gaspari, J. (2023). Exploring the common ground of sustainability and resilience in the building sector: a systematic literature review and analysis of building rating systems. Sustainability 15, 884. doi:10.3390/su15010884
Grubler, A., and Fisk, D. (2012). Energizing sustainable cities: assessing urban energy. abingdon, United Kingdom: Routledge.
Gwimbi, P., and Siimane, T. A. M. (2021). Public health early warning system responses to extreme weather events in Lesotho: experiences, lessons and the future. in Handbook of climate change management: research, leadership, transformation. Springer.
IPCC (2007). “Summary for policymakers,” in Climate change 2007: mitigation. Contribution of working group III to the fourth assessment report of the intergovernmental Panel on climate change. Editors METZ B., DAVIDSON O. R., BOSCH P. R., DAVE R., and MEYER L. A. (Cambridge, United Kingdom and New York, NY, USA: Cambridge University Press).
IPCC (2021). Climate Change 2021-the Physical Science Basis. Contribution of Working Group I to the Sixth Assessment Report of the Intergovernmental Panel on Climate Change. Editor V. P. Masson-Delmotte, A. Zhai, S. L. Pirani, C. Connors, S. Péan, N. Bergeret al. (Cambridge University Press, In Press). Accessed 9 August 2021
Janhunen, E., Leskinen, N., and Junnila, S. (2020). The economic viability of a progressive smart building system with power storage. Sustainability 12, 5998. doi:10.3390/su12155998
Khoja, A., Essig, N., Erber, S., Kristan, M., Hass, F., and Daivs, A. (2020). Assessment scheme including KPI for the assessment and benchmarking the sustainability of historic buildings. ATLAS. Available at: http://rgdoi.net/10.13140/RG.2.2.34213.73445. doi:10.13140/RG.2.2.34213.73445
Khoja, A. (2022). “Scale matters: exploiting cross-scale interactions for a smart and sustainable built environment,” in Intelligent environments: advanced systems for a healthy planet. Editor P. DROEGE (Amsterdam, Netherlands: North Holland).
Khoja, A. (2024). “Towards bridging the climate resilience gap in building assessment systems: an integrated framework for the German,” in Built environment. [Unpublished doctoral dissertation] (Germany: Technische Universität München). (TUM).
Khoja, A., and Danylenko, O. (2020). Real estate 5.0: synthetizing the next generation of buildings. Switzerland: ON RESEARCH, 50–61.
Khoja, A., Moro, A., Borgaro, P., Bazzan, E., Quinlivan, L., Dunphy, N., et al. (2021). EUB SuperHub project. D1.1—Quality, usability and visibility of energy and sustainability certificates in the real estate market—deliverable D1.1. September 2021. Available at: https://eubsuperhub.eu/assets/content/Deliverables/EUBSuperHub_D1.1.pdf.
Khoja, A., Moro, A., and Essig, N. Q. (2022). An integrated cross scale urban resilience assessment framework. in IOP conference series: Earth and environmental science. United Kingdom: IOP Publishing, 012015.
Khoja, A., and Waheeb, S. (2020). Vernomimicry: bridging the gap between nature and sustainable architecture. J. Sustain. Dev. 13, 33. doi:10.5539/jsd.v13n1p33
Kitazawa, K., and Hale, S. A. (2021). Social media and early warning systems for natural disasters: a case study of Typhoon Etau in Japan. Int. J. Disaster Risk Reduct. 52, 101926. doi:10.1016/j.ijdrr.2020.101926
Lariu, P., Schwarzak, S., Eichstädt, R., Wifling, M., Joneck, M., and Verbraucherschutz, B. B. S. F. U. U. (2021). Klimaanpassung in Bayern: Handbuch zur Umsetzung, Bayerisches Staatsministerium für Umwelt und Verbraucherschutz (StMUV).
Leggett, J. A. (2020). The united Nations framework convention on climate change, the kyoto protocol, and the Paris agreement: a summary. Independently Published.
Lützkendorf, T., Speer, T., Szigeti, F., Davis, G., le Roux, P., Kato, A., et al. (2005). A comparison of international classifications for performance requirements and building performance categories used in evaluation methods. Helsinki (Finland): Performance based building, 61–80.
Metz, B., Davidson, O., Bosch, P., Dave, R., and Meyer, L. (2007). Mitigation of climate change. Contribution of working group II to the fourth assessment Report of the intergovernmental. United States: Panel on Climate Change.
Munasinghe, M. (2001). Interactions between climate change and sustainable development–an introduction. Int. J. Glob. Environ. Issues 1, 123.
National Research Council (2012). Disaster Resilience: A National Imperative. Washington, DC: The National Academies Press. doi:10.17226/13457
Pannier, M.-L., Remoué, T., and Bigaud, D. (2022). Stochastic comparative LCA of smart buildings. E3S Web Conf. 349, 04012. doi:10.1051/e3sconf/202234904012
Petersen, J. E., Shunturov, V., Janda, K., Platt, G., and Weinberger, K. (2007). Dormitory residents reduce electricity consumption when exposed to real-time visual feedback and incentives. Int. J. Sustain. High. Educ. 8, 16–33. doi:10.1108/14676370710717562
Pishdad-Bozorgi, P., and Zeng, Q. (2022). Conscious intelligent buildings: envisioning the next generation of smart buildings. EPiC Ser. Built Environ. 3, 470–478. doi:10.29007/q151
Portner, H. O., Roberts, D. C., Adams, H., Adler, C., Aldunce, P., Ali, E., et al. (2022). Climate change 2022: impacts, adaptation and vulnerability.
Raven, J., Stone, B., Mills, G., Towers, J., Katzschner, L., Leone, M. F., et al. (2018). Urban planning and urban design. in Climate change and cities: second assessment report of the urban climate change research network. Cambridge University Press.
Reeves, K., Ul, M. L., and Fitzgerald, H. (2022). WP3-D1: review of the SRI and other appropriate systems for measuring the ‘smartness’ of a building and requirements for data monitoring and DIY toolkits as a result.
Revi, A., Satterthwaite, D. E., Aragón-Durand, F., Corfee-Morlot, J., Kiunsi, R. B., Pelling, M., et al. (2017). Urban areas in climate change 2014: impacts, adaptation, and vulnerability. Part A: global and sectoral aspects. in Contribution of working group II to the fifth assessment report of the intergovernmental Panel on climate change.
Rosenzweig, C., Solecki, W. D., Romero-Lankao, P., Mehrotra, S., Dhakal, S., and Ibrahim, S. A. (2018). Climate change and cities: second assessment report of the urban climate change research network. Cambridge University Press.
Sánchez Cordero, A., Gómez Melgar, S., and Andújar Márquez, J. M. (2019). Green building rating systems and the new framework level (s): a critical review of sustainability certification within Europe. Energies 13, 66. doi:10.3390/en13010066
Scheraga, J. D., and Grambsch, A. E. (1998). Risks, opportunities, and adaptation to climate change. Clim. Res. 11, 85–95. doi:10.3354/cr011085
Schünemann, C., Olfert, A., Schiela, D., Gruhler, K., and Ortlepp, R. (2020). Mitigation and adaptation in multifamily housing: overheating and climate justice. Build. Cities 1, 36–55. doi:10.5334/bc.12
Sertyesilisik, B. (2017). Building information modeling as tool for enhancing disaster resilience of the construction industry.
Seto, K. C., Dhakal, S., Bigio, A., Blanco, H., Delgado, G. C., Dewar, D., et al. (2014). Human settlements, infrastructure and spatial planning.
United Nations, Department of Economic and Social Affairs, Population Division (2019). World Urbanization Prospects: The 2018 Revision (ST/ESA/SER.A/420). NY, United States: United Nations.
Keywords: smart building, climate resilience, climate adaptation, sustainability, building rating systems
Citation: Khoja A and Danylenko O (2024) Charting climate adaptation integration in smart building rating systems: a comparative study. Front. Built Environ. 10:1333146. doi: 10.3389/fbuil.2024.1333146
Received: 07 November 2023; Accepted: 08 April 2024;
Published: 26 April 2024.
Edited by:
Yukun Shi, University of Glasgow, United KingdomReviewed by:
Marta Maria Sesana, University of Brescia, ItalyCopyright © 2024 Khoja and Danylenko. This is an open-access article distributed under the terms of the Creative Commons Attribution License (CC BY). The use, distribution or reproduction in other forums is permitted, provided the original author(s) and the copyright owner(s) are credited and that the original publication in this journal is cited, in accordance with accepted academic practice. No use, distribution or reproduction is permitted which does not comply with these terms.
*Correspondence: Ahmed Khoja, S2hqb2FAaG0uZWR1
Disclaimer: All claims expressed in this article are solely those of the authors and do not necessarily represent those of their affiliated organizations, or those of the publisher, the editors and the reviewers. Any product that may be evaluated in this article or claim that may be made by its manufacturer is not guaranteed or endorsed by the publisher.
Research integrity at Frontiers
Learn more about the work of our research integrity team to safeguard the quality of each article we publish.