- 1Eindhoven University of Technology, Eindhoven, Netherlands
- 2Delft University of Technology, Delft, Netherlands
- 3University of Twente, Enschede, Netherlands
How can we assess the ergonomic comfort of a sizeable spatial configuration such as the indoor space of a complex building or an urban landscape when we design, plan, and manage the space? Is there a fundamental difference between indoor [architectural] spatial configurations and outdoor [urban] spatial configurations with respect to ergonomics? Can we have a unified approach to the computational study of spatial ergonomics? This paper addresses these fundamental questions while providing a brief taxonomic review of the scholarly literature on these matters from a mathematical point of view, including a brief introduction to the modelling-based approaches to the computational ways of studying the fundamental effects of spatial configuration on human behaviours. Furthermore, the paper proposes a computational approach for ergonomic assessment of spatial configurations that explicitly allows for combined accessibility and visibility analyses in the built environment. The gist of this approach is the conceptualisation of spatial configurations as rasterised (voxelated) 2D manifold walkable terrains whose voxels have 3D vistas, unifying the simulations and analyses of accessibility and visibility. The paper elaborates on how such a representation of space can provide for conducting various sorts of computational queries, analyses, and simulation experiments for research in spatial ergonomics. The paper concludes with a mapping of the computational modelling approaches pertinent to the study and assessment of spatial ergonomics; and marks avenues of future research on various categories of exploratory, generative, and associative models for ex-ante and ex-post assessment of ergonomic matters at spatial scales.
1 Introduction
The importance of ergonomics and human factors in spatial design and interventions can be understood, unfortunately, by examination of the disastrous results of numerous contemporary building developments and interventions in cities around the planet that disregard ergonomics and human factors. Such interventions have resulted in the so-called ghost cities (Jin et al., 2017; DeLyser, 1999), dangerous crime neighbourhoods (Angel, 1968; Newman, 1996; Cozens et al., 2005; Jacobs, 2016), and depressive social environments (Wang R. et al., 2019; Galea et al., 2005). Systematically studying ergonomics and human factors based on the spatial configuration of the built environment can be beneficial for three primary purposes, in line with the three main activities of design processes as defined by Lawson (Lawson, 2006), all of which need to address fundamental questions regarding the relationship between environment and human behaviours:
• Evaluation: quality assurance of built environment, governance, standardization, certification through ex-post assessment of safety, operational efficiency, occupational comfort, and security;
• Analysis: spatial, social, behavioural sciences, and environmental psychology;
• Synthesis: scientific and evidence-based design methodology, design didactics, spatial-decision-support, and design research.
One of the pioneers of ergonomics research, Lillian Moller Gilbreth, formulated some of the oldest spatial questions in ergonomics about the comfort of kitchens and assembly lines for work and the relations between [spatial] movement, work efficiency, fatigue, and comfort (Perloff and Naman, 2012; Gilbreth, 1914). While the scale of a kitchen or the functional space for a stationary worker in an assembly line is too small to have non-trivial navigation challenges, the repetition of the work at scale (such as in hospitals or subway complexes) raises the importance of comfort of frequent human movements.
In an indoor environment, it is easy to observe that the configuration or layout of the environment can directly affect the spatial ergonomics of the building. However, the counterpart of configuration in urban land-use distributions, i.e., the functionalities assigned to spaces, has a rather subtle and yet substantial influence on the spatial ergonomics on the urban scale. The so-called cost of transport can show, for instance, that the expected walking travel time is dependent on the spatial configuration since the assignment of different functionalities to spaces is what initially motivates the movement of people between two spaces (q.v. (Nourian et al., 2021; Çubukçuoğlu et al., 2021)).
Notwithstanding the importance of morphological factors such as heights of ceilings, proportions of rooms, and alike, this article only addresses the ergonomic questions with regards to the syntactic relations between spaces rather than the morphological attributes of the spaces in isolation. We will address the discussion on the complex relationship between spatial configuration and spatial ergonomics, focusing on the potential comfort of pedestrians during way-finding and walking through the built environment. Our perspective is based on the assumption that accessibility and visibility are the main factors that are not only directly related to the holistic notion of human comfort and ergonomics in space, but also precisely in the purview of architectural designers and spatial decision-makers. This is because both of them are directly influenced by the design decisions concerning the configuration and shape of built environments.
Our proposed spatial modelling framework provides for combined accessibility and visibility simulations that can show the potential ergonomic comfort or discomfort of an environment with respect to walkability. This, however, does not mean that we are proposing a framework for simulations of actual walking behaviour. The framework, rather, paves the way for spatial ergonomics researchers to build models, test hypotheses, and collate their results in a standardised framework that is suitable for various approaches in modelling, ranging from mathematical models to models based on artificial intelligence. The key connective element, and thus the main advantage of the proposed framework, will be that it introduces graphs as natural representations of spaces, whether for accessibility analyses or visibility analyses. Furthermore, the fact that both kinds of graphs can be constructed out of the same superset of nodes (corresponding to voxels) means that the results of analyses obtained from the two types of studies can be easily collated and combined as desired. Moreover, the generality of the proposed approach is such that the distinction between indoor and outdoor environments will be rendered unnecessary, hence providing for a unified view towards analyses of architectural and urban configurations. Therefore, arguably, this approach opens up new avenues for rigorous research in spatial ergonomics.
2 Theoretical context
2.1 Design science, science of artificial, and generative science
A substantial part of Spatial Ergonomics as an interdisciplinary field of research is concerned with understanding the complex relations of pedestrians’ behaviour within complex spatial environments. Such systematic understandings could inform and underpin design activities by introducing a scientific approach to evaluating the impact of spatial designs and interventions on human comfort. Moreover, the paper argues that a knowledge-based approach for informing decision-making in design activities is necessary for guaranteeing human qualities usually referred to as the live-ability of buildings and cities. In this sense, Spatial Ergonomics can be seen as a design science as called for by H.A. Simon in (Simon, 1970). This is related to, yet different from the studies of environmental psychology and behaviour, which attempts to inductively understand the bi-directional relation of humans and their environment from a cognitive and physiological point of view (Golledge et al., 1993; McAndrew, 1993; Golledge, 1999). However, we also argue that the Problem-Oriented approach of environmental psychology (Proshansky, 1978) and the Design-Oriented approach of spatial ergonomics are approaching the essence of such a relation from a different angle.
This difference in approach allows us to consider spatial ergonomics as a subject matter of the Sciences of Artificial (Simon, 1970), i.e., the decision sciences aimed at the study of practices to improve the current state of systems towards better ones (non-verbatim, ibid). The main goal in the sciences of artificial is to extend the natural sciences by going beyond understanding phenomena and discovering the relation of human choices and their consequences, (Schelling, 1984). Within the scale of the city, understanding the complex relation of the urban environment and human behaviour requires explicit simulation models (Batty, 2007). To illustrate the specifics of scientific studies and their application in spatial ergonomics research, we find it important to distinguish three types of models as to their purposes:
• Exploratory Models are also known as the exploratory model analysis (EMA). These sorts of models do not aim to validate or refute a specific hypothesis, nor do they intend to predict a quantity precisely. Rather, they focus on the uncertainty in the open systems, exploring the implication of varied assumptions and hypotheses based on a series of computational experiments (Bankes, 1993; Agusdinata, 2008). Exploratory models benefit greatly from improved computational power in recent years, providing new knowledge for understanding the phenomena or system behaviours through the information gained from many computational experiments, acting as the base for further hypothesis building. Exploratory models could be driven by data, questions, or even models (Bankes, 1993). Network centrality analyses inspired by Social Network Analysis research are typical applications of exploratory models, as often seen in Space Syntax research (various exemplary reference provided further on).
• Associative Models are statistical models or neural networks that can be fitted/trained using data describing the dependent and independent variables. Such dependent variables can be numerical or categorical, making the problem either regression or classification. A predefined (classical) statistical model (such as multi-linear/logistic regression or a gravity model) can be fitted to the independent inputs to predict the dependant outputs of interest. Similarly, Artificial Neural Networks can be trained to find non-trivial (most likely non-linear) relations between independent input and dependent output variables to capture a complex association (see e.g., (Wang Z. et al., 2019)).
• Generative Models are also known as simulation models, which are mechanism replicators made based on a mathematical or computational understanding formalised in a theory or a simulation model capable of explaining “how things work.” Archetypal examples include an understanding or assumption of the local behaviour of a spatial agent (a virtual pedestrian) based on its surrounding environment and other agents in its vicinity; or an Agent-Based Model revealing the emergence of behavioural patterns from predefined situated patterns of behaviour (Crooks et al., 2015). Another example of such models is the simulation of mobility/accessibility through Random Walks (Nourian, 2016). Such models directly link to the idea of ergonomics and the so-called First Law of Geography, as formulated by Waldo Tobler: Everything is connected to everything else, but near things are more connected than distant things, (Tobler, 1970). Thus the easier it is to get somewhere, the higher the probability of going there: measuring distance in time, through stochastic paths (random walks) (Nourian, 2016).
To be able to understand and trace the effect of the spatial form (i.e., the geometry and topology of a built environment) on behavioural patterns, we need to move toward models that can explain and justify such complex relations. We should start from the fact that relationships between form factors and human behaviours are fundamentally complex not only on an individual/psychological level but also on a collective/social level and that they need to be studied through simulation models. If our only goal in studying ergonomics were to predict comfort or discomfort, then it would suffice to study the association of some spatial factors with various comfort criteria describing human perception or human behaviour within built environments. However, if we are to understand and explain the mechanisms underlying these [complex] associations, then we need to study the phenomena directly through simulation models (Epstein and Axtell, 1996; Epstein, 2008). Therefore, our perspective in spatial ergonomics is also to promote the notion of Generative Science which is the pursuit of explainable and reproducible knowledge through devising and experimenting with complex simulation models; introduced by Joshua Epstein (Epstein, 2012; Epstein, 1999). Thus, we suggest that we need to synthesise simulation models that can replicate the complex relations of causes/stimuli and effects/behaviours to understand the relation of the design decisions on the shape and configuration of built environments (choices) and social behaviours and human perceptions (consequences).
The relations between the form of the environment and human behaviour (e.g., movement) can be identified to have at least two types of complexities, one being the network complexity of navigable/walkable spatial environments and their effects on human behaviour; the other being the process complexity of the influences of the other actors on the behaviour of individual actors in an environment. In the face of such complexities, if the aim is to understand the mechanisms resulting in the emergence of specific behavioural patterns, it will be necessary to utilise generative simulation models that are capable of replicating the mechanisms of stimuli and responses and their inter-relations of multiple actors within a complex spatial environment (Epstein, 2012). Examples of such models are stochastic simulation models, Agent-Based Models, Cellular Automata, Game Theoretical Models, or network-based models such as Markov Chains (a.k.a. Random Walks). Furthermore, the simulation-driven approach has been widely applied to acquire insights and incorporate the complexity of the un-modelled uncertainties in the study of environment-agent interactions (DeLaurentis and Callaway, 2004; Macal and North, 2009; Heppenstall et al., 2011); a particular example of which is to assess how visibility influences path-finding (Gath-Morad et al., 2021).
However, developing such simulation models will introduce some complexities, as it is inevitable to step away from the principle of Ceteris Paribus (Schlicht, 2012). Considering this inherent complexity of the relations between the inputs and outputs of such morphology-behaviour models, we can distinguish the application of each category of models in the study of these inter-relations from two directions:
• Ex-Ante Assessment of environmental design decisions: given a design as a proposed new spatial configuration or intervention in an existing spatial configuration, ex-ante assessments focus on what will be the projected behaviours induced by the spatial configuration? Such questions can be best answered by building exploratory or generative models. These models can be validated and calibrated using exemplary data from the past.
• Ex-Post Assessment of environmental design decisions: given a building or a built environment configuration from the past and observed behavioural data (e.g., of pedestrian movement), ex-post assessments focus on how can the correlation between spatial configuration and human behaviour be made explicit through statistical models or neural networks? Considering the complexity of these associations, such questions can be best answered by building associative models through model-fitting or model-training using exemplary data (evidence) from the past.
Considering that all such models require behavioural data and spatial configurations from the past to be either trained, validated, or calibrated, it is clear that for moving towards an “evidence-based design” discipline, not only data needs to be gathered from the past, but it should also be structured properly by explicitly considering such analytic prospects. One key challenge or question of importance will be about the representation of a spatial configuration. It is important to have such a representation of a building or a built environment to assess and further analyse on, otherwise the notion of assessment becomes ill-defined.
2.2 Spatial morphology and space syntax
The terms morphology and syntax are historically borrowed from Linguistics, respectively referring to the study of the shape of the words in isolation and the grammatical structures connecting them. However, in the contemporary academic circles of architectural and urban studies, the term morphological colloquially refers to both morphological and syntactic studies on the configuration of space in built environments (Hillier, 1998; Marshall, 2004) and its relation to human behaviour; especially focusing on pedestrian movement in space (Hillier and Hanson, 1989; Hillier, 1999). Unequivocally, we can define spatial configurations as distributions of human activities (land-uses in geospatial scales and designated spatial functions in architectural scales) in non-trivial/non-Euclidean network spaces. In this sense, provided a network abstraction of the underlying space is available, a particular spatial configuration would be a coloured/labelled graph as an abstraction of the space in question.
Multiple scholars have proposed different lenses for the study of spatial morphology and, in particular, the “spatial configuration”. Conzen offered a categorisation of different elements of the urban landscape based on the time scale of their change: 1) the city layout, 2) building form, and 3) land use. Each of these elements represents different degrees of changes, i.e., land use changing most often, buildings’ physical form lasting longer, and the city layouts being the most permanent elements (Conzen, 1960). Vance ties the changes in the urban environment to human activity and claims that they can not be reduced to alteration of the physical structure (Vance, 1977). Frey relates the emergence of cities to the interaction of navigation, communication, and land values (Frey, 1999). Despite different formulations, what most of these lenses have in common is the emphasis on the relationship between the form of urban environments and the social structures that emerge within them (Stephenson, 2008); this perspective is widely known in the scientific literature as the morphological approach that is mainly concerned with the study of spatial form that can be defined as the geometry and topology (also referred to as the configuration or layout) of an environment and their effects on human behaviour. Based on this relationship, an urban form can be evaluated via activity patterns (primarily through the so-called pedestrian or natural movement (Hillier et al., 1993)), particularly human congregation that emerges within it, e.g., some studies have used activity/presence/movement density, and intensity as proxies for urban functionality (Chiu et al., 2020).
Hillier (Hillier and Hanson, 1989; Hillier et al., 1996) introduced the clear framework of Space Syntax to structure, measure, and understand the relation between the form of spatial structures and people’s behaviour within them. Space syntax offers an exploratory methodology to investigate the urban spatial structure and uncover the spatial qualities of urban areas and their correlations with various network centrality indicators as proxies for spatial activities related to pedestrian movement through spaces. These methods aim to quantify the configurational properties of the built environment through the study of the structural properties of spatial networks, mainly through network centrality indicators. At the architectural scale, syntactic measures have been shown to correlate with behavioural patterns in a variety of settings, including malls (Okamoto et al., 2014; Omer and Goldblatt, 2016; Koohsari et al., 2013); subway stations (van der Hoeven and van Nes, 2014), museums (Turner and Penn, 1999), and hospitals (Haq and Luo, 2012). At an urban scale, these centrality indicators (such as integration/closeness or choice/betweenness) have been investigated based on their potential for revealing various relations between urban structural elements and human activity patterns (Peponis et al., 1997a); the influence of spatial structure (as a network) on staying behaviour at certain Points of Interests (referred to as movement to spaces in the Space Syntax jargon), as well as the correlation of the frequency of pedestrian movement through spaces with the presence of socio-economic activities (such as retail).
While urban form (Pacione, 2009) is assumed to be the conductor of the pedestrian movement patterns (Hillier et al., 1993), the effect of land-use or the distribution of designated functions in urban or architectural scales cannot be ignored in shaping the pedestrian movement patterns; this is because the reasons people walk in an environment have to do with their social and economic needs (Algers et al., 2005; Meyer et al., 2001). However, human movement is arguably a focal point of attention for ergonomics research at a spatial level, especially because it is directly affected by the design decisions on the shape and layout of an environment. Consequently, these decisions on a larger scale can function as an attractor or a driving force of developments (Johnson and Fisher, 2013).
2.3 Visibility and accessibility analysis
A comprehensive understanding of the relation of the behavioural patterns and built environments (either in architectural scale or urban scale) pertains to two main ergonomic aspects: Accessibility and Visibility in space.
Within Hillier’s approach, the relation between urban structural elements and human activity patterns is not limited to the accessibility provided by the street network; It also includes concepts to denote the visual relations of spatial elements such as axial graph (Bentley, 1985; Moughtin, 2007; De Smith et al., 2018) and Turner-Penn’s topological model of space is derived from visibility graph (Turner, 2001; Penn, 2003). This model can be constructed, e.g., based on the intersections of visibility polygons (a.k.a. Isovists (Benedikt, 1979)) to quantify the inter-visibility and proximity of spatial elements (Turner, 2003; Turner et al., 2001). Penn argues that this approach (e.g., the use of axial lines of sight) contributes significantly to the understanding of environmental cognition by putting forward a topological space representing our cognitive model of space (Penn, 2003).
In his seminal book, “The Image of the City” (Lynch and for Urban Studies, 1960), Lynch argues that legibility facilitates the perception and understanding of the spatial structure, and consequently, there is a correlation of the sense of identity in the urban space and legibility. He specifies three elements that comprise legibility: clarity, visibility, and coherence (Lynch and for Urban Studies, 1960). Legibility is influential in way-finding (Weisman, 1981; Koseoglu and Onder, 2011), and this is not only in perceiving the space and constructing a cognitive map from it but also in utilising the cognitive map in way-finding (Belir and Onder, 2013). The two most important factors affecting legibility are simplicity of spatial configuration (2D) and saliency of landmarks (3D), which respectively correspond to accessibility and visibility of urban spaces (Lynch and for Urban Studies, 1960). Lynch ties the construction of the cognitive map of a city to five elements: nodes (2D), paths (2D), districts (2D), landmarks (3D), and edges (3D) (Lynch and for Urban Studies, 1960). He maintains that the 2D elements function as anchors of locations. Thus their role in the cognitive map of the user is topological, whereas the 3D elements, on the other hand, provide a sense of distance and direction for the user. Thus their role is more geometrical (Dalton et al., 2003). On a tangent line and from a descriptive point of view, O’Neill ties the representation of cognitive map to the concept of legibility by defining it as the potential of a space to facilitate the formation of a successful cognitive map (O’Neill, 1991).
People experience and understand space primarily through vision, mapping the relation of the visible features of the space, and identifying the navigation opportunities (Li et al., 2016). Landmarks or salient objects in particular play an important role in wayfinding (Daniel and Denis, 1998; Denis et al., 1999; Lovelace et al., 1999; Schroder et al., 2011). Sorrows et al. attribute the distinctiveness of landmarks to their visual (colour, shape, etc.), semantic (function, type, etc.), and structural salience (location as to other elements) (Sorrows et al., 1999). Golledge (Golledge et al., 1993) puts a relative perspective on the matter and ties the quality of being a landmark to the distinctiveness of an element compared to other elements in a local or global neighbourhood, a relative view towards salience that is fundamental to our decision-support perspective towards the computational study of ergonomics. Although characterisation and detection of potential elements as landmarks can be done without topological models (see an example in (Nothegger et al., 2004; Steiniger et al., 2008)), structural salience depends on the position of a landmark along a route (Klippel et al., 2005; Elias et al., 2023), thus requiring an implicit or explicit topological model. Specifically, visibility is proved to be influential for way-finding and navigation in complex space, even when such as space is a multi-level uncertain environment that has vertical dimension (Gath-Morad et al., 2021).
Although it is indisputable that the local and global structural properties of an urban environment (or the indoor space of a building) influence the cognitive representations of it (FREUNDSCHUH and EGENHOFER, 1997), the fact that legibility is a more subjective measure of the space rather than an objective property of it, has led to the complication of understanding of humans’ spatial perception processes in built environments (Zmudzinska-Nowak, 2003). To untangle this subjective-objective dichotomy, a few attempts have been oriented towards comparing the quantitative representation of a given built environment with humans’ perception of it. (Stamps, 2005; Gjerde, 2011; Long and Baran, 2011). In particular, the correlation of legibility (subjective) and intelligibility (objective) (Long and Baran, 2011), and modelling permeability using Isovists (Stamps, 2005). It is worth noting that there is a parallel field of research focused on way-finding and path-planning for robots, called Robot Motion Planning (cf. (Patle et al., 2019)) that is especially relevant to the discussion because of its history in developing environment maps as accessibility and visibility graphs for navigation.
Alternative models for studying accessibility from a cognitive/psychological point of view (and thus in relation to visibility) have the potential to take cognitive factors into account, e.g., by considering the simplicity or ease of navigation in path-finding (Turner, 2001; Duckham and Kulik, 2003; Nourian et al., 2015), or by utilising Random-Walk, Markov-Chains, or Spectral Models in analysing accessibility from a stochastic perspective as opposed to the inherent deterministic perspective associated with using centrality indicators based on geodesics or shortest paths (Nourian, 2016; Blanchard and Volchenkov, 2008; Nourian et al., 2016a). Moreover, the notions of accessibility and visibility lead to an interesting phenomenon: somewhere is considered accessible when it is eventually accessible under some conditions (a maximum time or distance before reaching the place), but somewhere is considered visible only if it is immediately visible. This semantic difference also becomes a challenge for unifying the analyses of accessibility and visibility.
In summary, studying accessibility from a cognitive and ergonomic point of view would eventually require studying visibility, especially for assessing the legibility of an environment for way-finding. This entails a need for a mathematical model of the relation between accessibility and visibility that can support exploratory and associative approaches, as well as generative simulations.
3 Proposed framework
3.1 Intertwined geometrical and topological models of space
Purely topological models of space are arguably inspired by the social network models studied in computational social science since the 1950/60s (Nourian, 2016; Bonacich, 1987). They are often considered for studying the configurational properties of the urban space (e.g., (Hillier, 1998)). Due to the historical prevalence of [urban] spatial network models consisting of lines (axial lines of sight in Space Syntax-oriented models or the street centre line in Transport Planning oriented models), much attention has been paid to the adequate representation of line-based networks for morphological studies. In retrospect, it can be seen that the orientation towards line-based networks has been mainly a pragmatic choice for keeping computational costs low. Multiple critiques of this approach have pointed to either the counter-examples for spaces that cannot be reduced to a line network or the failure of topological models in integrating the metric properties (Turner et al., 2001; Peponis et al., 1997b; Batty, 2001; Ratti, 2004a; Ratti, 2004b; Montello, 2007; Kostakos et al., 2010; Netto, 2015; Pafka et al., 2018). However, without dwelling much on the futile debates on the advantages or disadvantages of geometric or topological models of space, given that topological and geometric measures are not independent, we argue that models need to be constructed based on a close relation of geometry and topology rather than a supplementary attribution of metric weights.
We argue that the reduction of walkable or visible space into a few lines is overly simplistic and problematic. Conventionally, i.e., in Space Syntax studies, both accessibility and visibility are modelled through topological models made up of “lines of sight” dubbed Axial Lines (Penn, 2003). However, even apart from the reported problems in defining such axial-line networks ((Ratti, 2004b)), the connection (similarities and differences) between the two notions could be more explicit. Mathematically speaking, a walkable surface is essentially a 2D-Manifold and a visible prospect is fundamentally a 3D-Manifold. Studying accessibility requires working with geodesic/optimal-path algorithms on [1D] line-networks or [as we propose] on [2D] surface-meshes representing 2D manifold-surfaces of passages, possibly with many holes (mathematically known as surfaces of high genus/genera [holes]) and that studying visibility requires working with intersection algorithms within 3D manifold-solids of prospects (mathematically known as solids of high genus/genera [holes]). It suffices to say that from a mathematical point of view, a manifold surface or a manifold solid cannot be reduced into a line-network without losing the metric properties of space unless a relatively high density of graph vertices is produced to “sample” the space. Though accessibility and visibility analyses have different inherent characteristics, and thus modelling requirements, they have a similar constituent element, i.e., the spatial units. To make the connection more explicit for exploratory, associative, and generative modelling studies, we argue that the models be made separately by respecting the inherent differences of the two notions while using similar constituent elements to allow for collation and connection of their results.
We propose here to derive the topological model from a regular discretisation of the geometry of walkable surfaces and visible environments to provide for comprehensive ergonomic research (see Figure 1 for a complete picture). We argue that a regular spatial subdivision based on voxels can provide a uniform way of modelling 2-Manifold walkable spaces and 3-Manifold visible spaces for studying accessibility and visibility respectively. Space Syntax models were also later generalised by the models made by late Alasdair Turner for pixel-based visibility and accessibility analysis (called the Visibility Graph Analysis) integrated into Depth Map (Turner et al., 2001; Turner, 2007), which could be seen as consistent with the framework proposed in this paper. A few of the crucial concepts introduced in this section are explained in more detail in Table 1. The following sections elaborate on the steps introduced in Figure 1.
3.2 Spatial modelling based on topological voxelation
We propose to use the regular discretisation of spatial structures through voxels to construct a topological voxelization of 2D manifold surfaces and 3D manifold solids for accessibility and visibility analyses. Analysing both qualities on voxel models can be done by using graph/network representations constructed based on the topological relations between voxels, which includes (but is not necessarily limited to) the face-to-face connectivity between the voxels.
Both the walkable passages needed by accessibility analyses and visible prospect needed by visibility analyses could be modelled through topological voxelation on their original manifold. Afterwards, the voxelated model could be uniformly used to construct accessibility graphs and visibility graphs can be respectively constructed based on definitions of isochronal accessible neighbourhoods and isovist visible neighbourhoods. These local definitions of connectivity effectively result in a graph that encapsulates the connectivity structure of a spatial configuration in terms of immediate accessibility or immediate visibility. Figures 2, 3 show how the accessibility and visibility graphs could be constructed stepwise in a hypothetical voxelated space referenced with a 3D Morton curve, on a horizontal and vertical section, respectively. Both examples show the local neighbours of a separate voxel unit, demonstrating the edges and all connected nodes to this specific node in the graph to be constructed. After going through this process over all the nodes in the set, the corresponding graphs could be constructed (in the form of sparse matrices). In practice, interestingly enough, both such spatial models can be built by using regular volumetric cells or voxels with the help of software packages such as topoGenesis in a straightforward manner (Azadi and Nourian, 2021). It is also noteworthy that the visibility graph can be constructed within the raster through Fast Voxel Traversal algorithm (Amanatides and Woo, 1987).
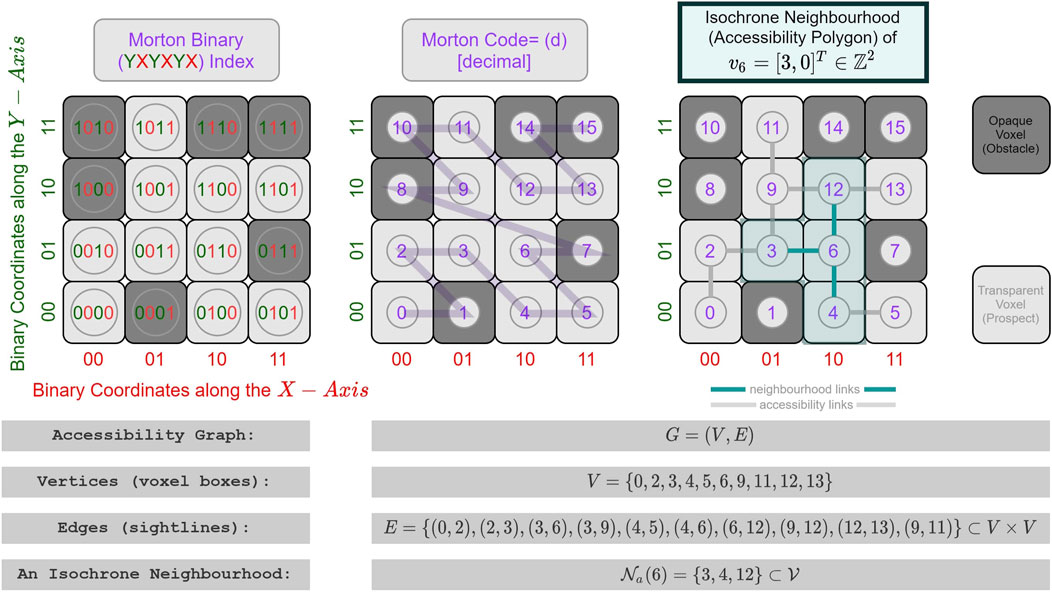
FIGURE 2. Accessibility graph construction on a hypothetical horizontal section of a voxelated passage space referenced with a 3D Morton curve.
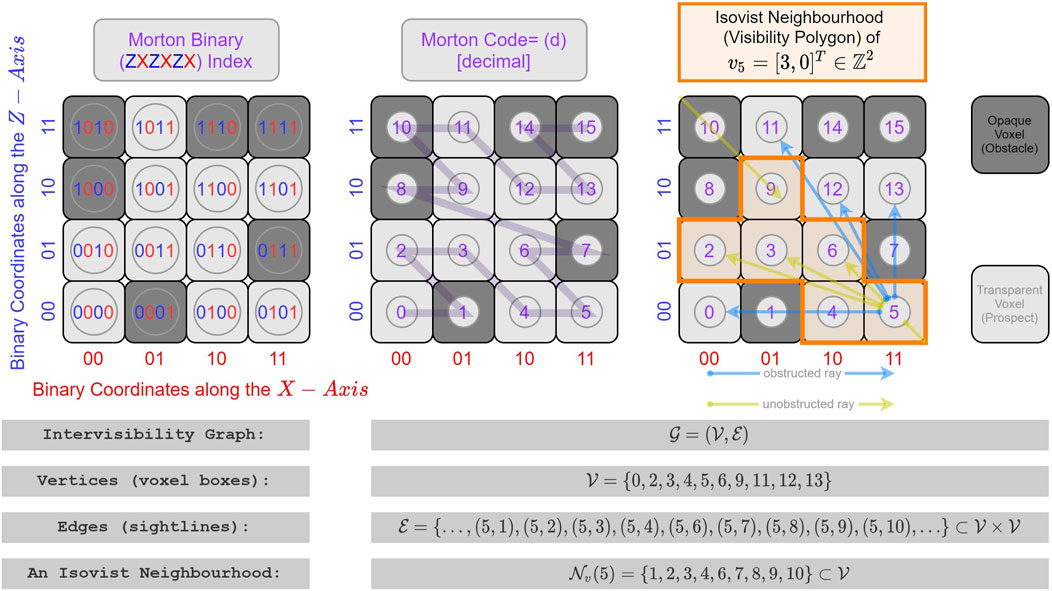
FIGURE 3. Visibility graph construction on a hypothetical vertical section of a voxelated prospect space referenced with a 3D Morton curve. Original mesh. Continuous 2D manifold. Accessibility Graph (Katz Centrality).
It is worth mentioning that the scale of the graphs generated from walkable surfaces and visible solids are usually not the same due to the difference in dimensionality. The latter would have far more nodes than the former, while in a graph with binary weights on edges, the former is usually a subset of the latter, as well, since all the [immediately] accessible locations are [immediately] visible for people in specific perspectives from the corresponding voxel. As shown with a rightward arrow in Figure 1, the entire voxel space of the accessibility graph can be elevated to an eyesight level and incorporated into an intervisibility graph. In this way, a bipartite graph of inter-visibility can be formed to distinguish the visibility stances and visibility targets. Note that the examples shown in Figures 2, 3 are based on immediate binary connections. We want to stress the semantic difference of accessibility and visibility mentioned in Section 2.3. In the framework proposed by this paper, the two approaches are further unified: direct/immediate spatial or visual connections (visible and accessible neighbourhoods of a certain location) could be modelled with the edges in the graph, while the locations that are eventually accessible or eventually visible by people could be represented with a path in the graph traversing the walkable nodes. As a result, a global picture of [eventual] accessible or [eventual] visible spaces can be constructed from a “connected-component” (an island in the graph, in a manner of speaking). Furthermore, as described in Table 1, the relations represented by the edges in a graph can be either binary for marking if a pair of nodes are interconnected/inter-visible or not, or in the real-number range to get the relations weighted with some Gaussian normalisation, based on the different proximity/similarity/connectivity of the nodes. Furthermore, as described in Table 1, the relations represented by the edges in a graph can be either binary for marking if a pair of nodes are interconnected/inter-visible or not, or in the real-number range to get the relations weighted with some Gaussian normalisation, based on the different proximity/similarity/connectivity of the nodes. For example, for accessibility, the existence of slopes would make the comfort (ease) of walking in different directions vary (Nourian, 2016; Nourian et al., 2015), and places farther than a certain area would be homogeneously considered as “too far” for being accessible by people; thus changing the concept of accessibility to a linguistic variable in the sense of Fuzzy Logics (Nourian et al., 2016a). Similarly, for visibility, the angles of viewing can be assumed to be different in comfort and ease of viewing and finding a salient object (e.g., in the context of way-finding). Evidently, all these aspects could be modelled with adjustable parameters in the voxel-based modelling framework.
Our proposed approach makes the distinction between the topology and geometry of the environment unnecessary (Morello and Ratti, 2009; Fisher-Gewirtzman et al., 2013; Nourian et al., 2016b; Gorte et al., 2019), as the metric distance between locations is preserved even after graph construction and can be inferred from the integer coordinates of voxels and could be easily demonstrated on the walkable manifold (Nourian, 2016; Gorte et al., 2019), which would be otherwise lost in purely topological models (Ratti, 2004b).
Additionally, it provides a uniform way of studying spatial ergonomic matters such as way-finding in architectural (indoor) and urban (outdoor) environments as it removes the need to distinguish streets and corridors from other walkable areas such as squares and rooms (as seen in Figure 4). Both indoor (Gorte et al., 2019) and outdoor (Nourian et al., 2016b; Morello and Ratti, 2009) spaces can be modelled in the same way either as voxelated approximations of walkable 2-manifold surfaces or as voxelated approximations of visible 3-manifold solids. Specifically, Topological Voxelization at a geographical scale (Nourian et al., 2016b) preserves the most important properties of spatial forms and provides a uniform level of resolution while removing noise and unnecessary details. In scenarios where a combination of models are needed, voxel-indices or vertex indices in the graph can be globally unique indices referring to a space-filling curve, such as the Morton curve (see Figures 2, 3), thus making it possible to adjoin graphs if necessary without confusion. In addition, the globally unique indexing will facilitate querying data on both the graph and voxelated model. Specifically, the binary coordinates of the voxelated model referenced with a Morton curve can show the clustering information of the nodes in the space, since it effectively forms a Quadtree (2D) or Octree (3D) in space.

FIGURE 4. The original mesh is representing a 2-manifold walkable surface of a hypothetical architectural configuration (Nourian et al., 2015). After voxelization, the voxelated model has been used as the basis of the accessibility graph (16,548 nodes and 31,958 links). The result of Katz centrality has been visualized on the accessibility graph using Houdini 18.5 (https://www.sidefx.com/). Katz centrality was performed using NetworkX-NumPy (2.6.3) (Hagberg et al., 2008) implementation with the attenuation factor set to 99.9% of the reciprocal of the largest eigenvalue of the adjacency matrix. The procedure took 43 s on a 6-Core Intel Core i9.
In summary, the voxelated framework provides a uniform way of constructing the base models on which both types of analyses/simulations (accessibility and visibility) can be performed.
3.3 Generality of voxel-based models
The accessibility and visibility graphs described above can facilitate spatial analysis in multiple ways: from providing for straightforward ways of converting questions to standard queries and property checks on graphs, at the simplest level, to facilitating problem-solving by utilizing graph traversal algorithms, centrality analyses on networks, and more importantly enabling the utilization of constructs from Spectral Graph Theory for simulating Random-Walks/Markov-Chains or solving Partial Differential Equations. Similarly, the same graphs can be used as “environments” for making Agent-Based Simulation Models. Understanding the value of graph data models is easier when they reveal patterns that could not be revealed [so easily] otherwise. Even the simplest uses of such graphs such as running the most routine queries, e.g., the neighbours of a visibility vertex will reveal unintuitive properties of the modelled space such as, for instance, all the points in a neighbourhood that can see the tip of a tower. The so-called “viewshed” of a heritage building can similarly be made by finding the union of the visibility neighbourhoods of the voxels associated with it. The advantage of such straightforward procedures must be clear for standardizing and streamlining assessment procedures, e.g., with respect to municipal regulations for view protection concerning architectural heritage (for planning heritage buffer zones). Furthermore, such graphs can also be used to answer generative design questions, e.g., what is the shape of the locus of points with a certain level of minimum view towards greenery in a neighbourhood or a hospital? Where should we place a sign with maximum view probability for pedestrians in a metro station? These questions refer back to the central question of ergonomics study in space: the comfort and discomfort of people in space and how a design or an intervention can change such comfort levels. In summary, there are various types of models (as previously mentioned in Section 2.1) that can be constructed based on the voxel-graph models to study spatial ergonomics questions as application scenarios:
• Exploratory Models: similar to social network analysis, the centrality-based analysis could be performed on the voxelated models. They are typically exploratory in the sense that a network centrality indicator is first computed and assumed to be an indicator of the spatial potential (or lack thereof) for human activities (e.g., pedestrian movement).
• Associative Models: we could collect data concerning urban spatial ergonomics, such as the real-world time needed for navigation, the felt comfort or discomfort and/or physiological parameters of comfort during walking, the pattern of cognitive maps formed in the space, the emotions, memories and other senses in space, the efficiency, effectiveness, and vitality of space (Nourian et al., 2021), and use the voxelated model as the common ground, attribute the aforementioned data to nodes or edges of the graph and perform associative analysis and predictions.
• Generative Models: we could also conduct various simulations on the voxelated model with different parametric configurations to come to an interpretable and explainable conclusion. For example, simulation models of pedestrian behaviour could be made by discretisation of a Partial Differential Equation describing universal rules of the pedestrian behaviour in a spatial domain (e.g., (Hoogendoorn et al., 2014)) or as an Agent-Based Simulation-Model (e.g., (Gath-Morad et al., 2021; Turner, 2007)).
4 Demonstration
To illustrate the potentials of the proposed framework, we have created a simple exploratory model of accessibility and visibility based on a portion of urban environment of Rotterdam, the Netherlands (see Figure 5). The Zomerhofkwartier (ZoHo) in Rotterdam, situated near Central Station, is undergoing redevelopment. The area is predominantly residential but it also includes some office spaces. On the boarder of the area lies “de Hofbogen,” a former elevated rail track from the 19th century that is not in use any more. Historically facing socio-economic challenges, including poverty and perceived insecurity, the predominantly migrant-populated area has seen recent initiatives like the Waterplein Benthemplein project to enhance social resilience. In 2020, the City of Rotterdam announced new development plans for ZoHo aiming to create new homes, work spaces, and rooftop green landscapes that will be connected to the future Hofbogenpark. The planning process involves consultation with the ZoHo district-level council and groups such as ZoHo Citizens, representing local entrepreneurs and organizations (Peinhardt, 2021).
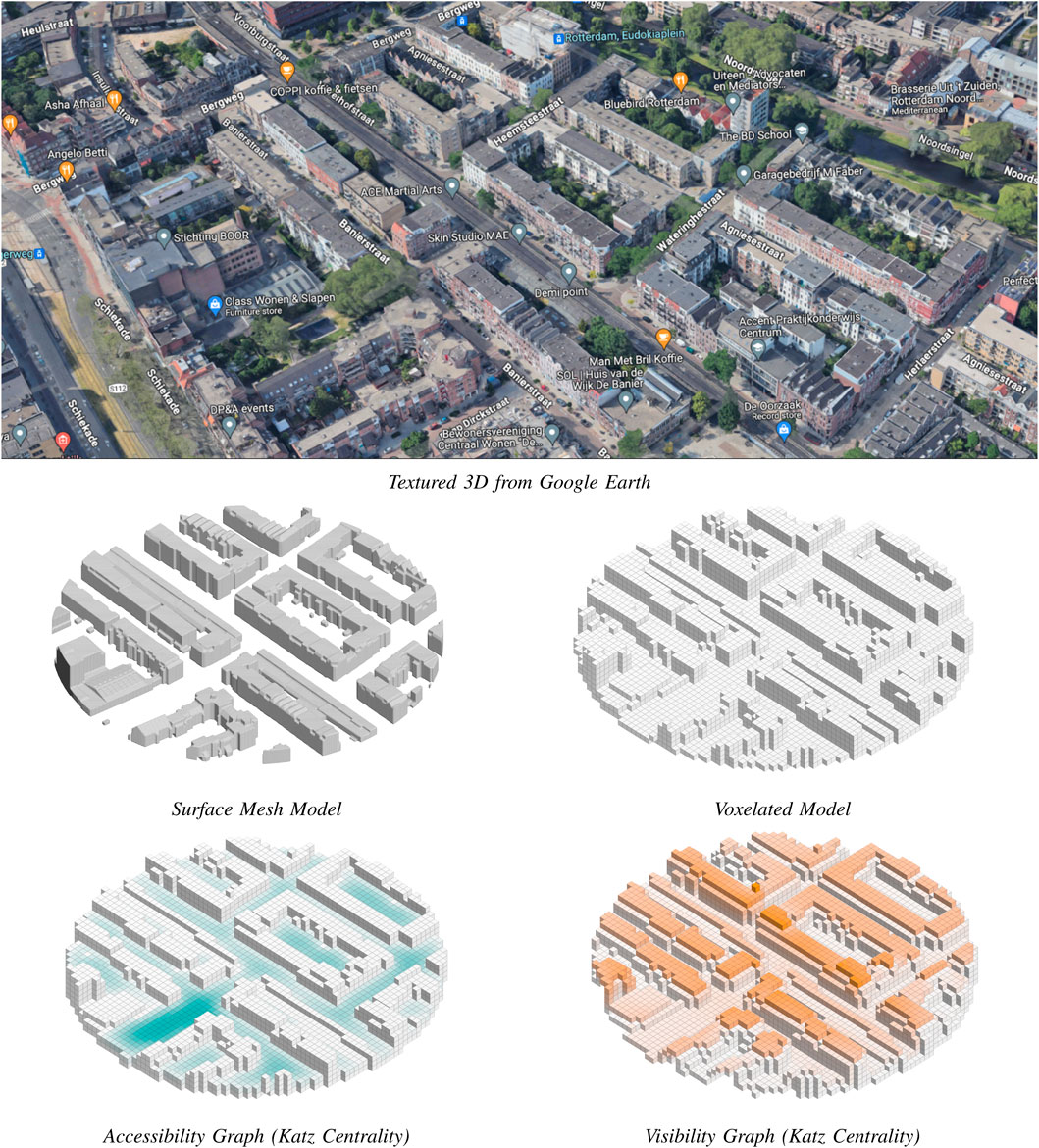
FIGURE 5. A portion of the ZoHo neighbourhood in Rotterdam: the surface mesh and voxelated models; Katz centrality for accessibility and visibility graphs; along with the view of the textured 3D model of the area from Google Earth to illustrate the urban context.
The surface mesh represents a portion of the ZoHo neighbourhood in Rotterdam. After voxelization, the voxelated model is used as the basis of the accessibility graph (1,631 nodes and 2,741 links) and the visibility graph (4,477 nodes and 960,523 links). The result of Katz centrality has been visualized on both graphs using Houdini 18.5. Katz centrality was performed using NetworkX-NumPy (2.6.3) (Hagberg et al., 2008) implementation with the attenuation factor set to 95.0% of the reciprocal of the largest eigenvalue of the adjacency matrix. The procedure took 0.1 and 4.5 s on a 6-Core Intel Core i9 for accessibility and visibility graph accordingly. The codes and models are open source and available at [redacted].
The models reveal the usefulness of accessibility and visibility graphs by visualizing a Katz Centrality indicator on both of them, which is a particular variant of Eigenvector Centrality proposed by Leo Katz in 1953 for revealing the relative importance of nodes in social networks as to the ‘status’ of actors (Katz, 1953). Eigenvector centrality indicators shows the relative centrality of the nodes in a network with respect to the relative centrality of their neighbours recursively, which can also be adjusted to be the final result of a Random-Walk Simulation. Intuitively, a node of high eigenvector centrality can be said to have a high influential position for diffusions or dispersion of information along the links of the network. As explained in (Nourian, 2016) (pp.228-236), such eigenvector centrality indicators are used in geographical analysis, social network analysis and webpage sorting (PageRank (Page et al., 1999)). The particular advantage of Katz centrality, as compared to PageRank and the ‘normal’ eigenvector centrality, is that it works for directed graphs and that it is not influenced by the degree regularity of the nodes that is a characteristic of accessibility graphs on voxelated manifolds. Katz centrality indicator has an attenuation parameter that defines the relative importance of longer walks as compared to short walks, which effectively allows for mitigating the adverse effects of the extreme degree-regularity of accessibility graphs. In particular, the results presented in Figure 5 highlight the utility of accessibility and visibility graphs by revealing the relative importance of some locations in a voxelated network. Interestingly, obtained with the same Katz centrality indicator, both results reveal meaningful patterns of influence with regards to accessibility and visibility. Theoretically, a place of high Katz centrality in the accessibility graph can be said to be highly accessible even for random walkers, thus beneficial for planning influential urban functions that need to be easily accessible; and a place of high Katz centrality in the visibility graph can be said to be highly visible from the highly visible places, thus of importance with respect to visibility, proper for, e.g., the construction of a new landmark. Note the difference between the two voxel models used for accessibility and visibility analyses: the base map in voxels used for visibility analysis represents a one-layer-thick voxel envelope around the urban objects while the base map in voxels used for accessibility analysis represents the walkable ground surfaces. For example, note that the spots of high altitude in the visibility graph are highlighted in the centrality analysis and that the highlighted spots in the accessibility graph are obviously connected to layers of well-accessible voxels within the scope of the model. Such indicators would also be useful in creating safe spaces, e.g., following the normative ideas of the theory of Defensible Space Theory of Newman (Newman, 1996) and the arguments of Jane Jacobs (Jacobs, 2016) (see, e.g., (Byon et al., 2010; Rosser et al., 2017)). Therefore, we could argue that such exploratory computation is beneficial for both ex-ante and ex-post assessment of built environments as mentioned in Section 2.1.
Another rationale for demonstrating preliminary results from Katz centrality is out of pragmatic considerations concerning the computation costs. Accessibility graphs are utilized nowadays on a daily basis on navigation platforms such as Google Maps and thus they are already commonplace. However, visibility graphs and their value in the study of ergonomics are less known, perhaps due to the prohibitive time and memory costs of making visibility graphs of substantial size. As can be seen in the provided examples from such graphs in an urban context, the visibility graphs constructed as such can be quite large for urban environments (both due to involving many nodes and many more links), orders of magnitude bigger than their accessibility graph counterparts. Even though conventional centrality analyses (such as closeness centrality and the notoriously expensive betweenness centrality) will be prohibitively expensive to run on such humongous graphs, stochastic analyses such as Katz centrality and PageRank analyses can be performed successfully on such graphs, as shown in the examples.
We have only demonstrated the potential of exploratory models in these exemplary settings. The application of associative and generative models will be the future focus of our work. It is important to note that we only discussed the construction of unweighted and undirected binary graphs but more interesting questions can be answered by weighting the adjacency links in directed graphs (both for accessibility and visibility graphs) according to the purpose of the model, which will be supported by the Katz centrality indicator as introduced, without loss of generality.
5 Conclusion and discussion
Accessibility and visibility analyses could be considered the two most crucial problems concerned within urban spatial ergonomics to discuss the relations between the built environment and human behaviours. Considering the advantages of the voxel models in the unification of geometry and topology, they facilitate the synthesis of an overarching representation model for spatial configurations (at both architectural and urban scales). This is essentially different from the kind of ergonomics discussed in the context of industrial design as that field operates on the object-scale and focuses more on the geometrical and physical properties of the human body, whereas in the spatial-scale of the built environment, the discussion of ergonomics focuses on cognitive and locomotive capabilities of humans that govern their behaviour within the space.
In a voxelated space, a spatial configuration can be defined as a coloured graph, dual to a voxelated domain, in which the voxel colours/labels indicate the designated use (functionality) of spaces. Such a model of a spatial configuration would encompass all the aforementioned aspects of importance previously considered in architectural/urban morphological research (geometry, topology, and land-use/function-allocation). However, as compared to irregular networks, voxelated networks can be rather dense and thus heavy for computational simulations of accessibility and visibility (as seen in Figure 5). Whilst this can be a pragmatic barrier in the computational aspects of research in spatial ergonomics, the uniformity arising out of the simplicity of the voxel-graph models makes them unrivalled for spatial ergonomics models, especially for developing simulation models. In addition to urban environments, this framework and methodology is also applicable to the case of an indoor space with pieces of furniture that is voxelated (with appropriate size) to analyze the visibility and accessibility within indoor environments. Particularly, the added value of this framework is in having an integrated approach to how indoor and outdoor environment can be modelled and analysed.
One point of attention that was not discussed throughout the article is the temporal dimension of simulation models: in the study of visibility and accessibility, time can be a factor that can be inherently present in simulation models (think of daylight and its effects on visibility or temporal patterns of movement in a city/building), but exploratory models or associative models are relatively indifferent to time. On the other hand, in setting impact-assessment models for decision-support, one is often interested in the long-term and average temporal effects of decisions on an environment. This entails that the results of the simulations often need to be aggregated at least in time to provide such a long-term perspective. It might also be desirable to simplify or generalise the spatial structure of a building or a city to a few nodes or even one node for assessment purposes, hence another reason to aggregate/integrate the simulation results in space for assessment purposes, which is also not deeply touched by this paper.
Providing a uniform representation model such as the one proposed here enables the discipline to evaluate a given spatial scenario as to spatial ergonomics. Furthermore, the discretisation provides a clear and navigable structure for a solution space and provides for adopting a decision-making approach to urban and architectural design and developing explainable and explicit knowledge about ergonomics and human factors of architectural and urban design. We sincerely hope that the theoretical framework proposed in the paper could initiate some discussions.
Long Abstract (600 words) How can we assess the ergonomic comfort of a sizeable spatial configuration such as the indoor space of a complex building or an urban landscape when we design, plan, and manage the space? Is there a fundamental difference between indoor [architectural] spatial configurations and outdoor [urban] spatial configurations? Can we have a unified approach to the computational study of spatial ergonomics? This paper addresses these fundamental questions by providing a brief taxonomic review of the scholarly literature on these matters from a mathematical point of view. It presents a brief introduction to the modelling-based approaches to the study of spatial complexity and the computational ways of understanding the fundamental effects of spatial configuration on human behaviours. The paper argues that a substantial part of Urban Spatial Ergonomics (USE) as an interdisciplinary field of scholarly research is concerned with understanding the complex relations of pedestrians’ behaviour with the configuration of complex spatial environments. Such systematic understandings could inform and underpin design activities by introducing a scientific approach to evaluating the impact of spatial designs and interventions on human comfort. Moreover, the paper argues that a knowledge-based approach for informing decision-making in design activities is necessary for guaranteeing human qualities usually referred to as the live-ability of buildings and cities, particularly by introducing ex-ante and ex-post assessment frameworks. In this sense, USE could be closely related to the notions of Design Sciences, the Sciences of Artificial, and Generative Sciences. The paper further introduces three types of models that could be beneficial for USE, namely, exploratory models, associative models, and generative models, suitable for application in the ex-ante and ex-post assessments of design decisions. Furthermore, the paper proposes a computational approach for ergonomic assessments of spatial configurations that explicitly allows for combined accessibility and visibility analyses in the built environment. The gist of this approach is the conceptualisation of spatial configurations as rasterised (voxelated) 2D manifold walkable terrains whose voxels have 3D vistas, unifying the simulations and analyses of accessibility and visibility with the mathematical representation of graphs. The paper elaborates on how such a representation of spatial configurations can provide for conducting various sorts of computational queries, analyses, and simulation experiments for research in spatial ergonomics. Specifically, a voxelated model of space (whether the original model of space is a 2D manifold surface or a 3D manifold solid) will have multiple advantageous properties, including the straightforwardness of constructing a topological model without losing metric distance information, and the unified representation on both indoor and outdoor spaces in both architectural and geographical scales. Additionally, the paper includes a few demonstrative examples illustrating how accessibility and visibility graphs could be constructed in both a hypothetical spatial configuration and a real-world urban district in Rotterdam, the Netherlands. Preliminary results of exploratory models using Katz centrality, a variant of eigenvector centrality originated in Social Network Analysis, in both graphs are presented, which show the potential of voxelated models of accessibility and visibility analyses in USE studies. The paper concludes with a mapping of the computational modelling approaches pertinent to the study and assessment of spatial ergonomics; and marks avenues of future research on various categories of exploratory, generative, and associative models for ex-ante and ex-post assessment of ergonomic matters at spatial scales.
Data availability statement
Original datasets are available in a publicly accessible repository: https//github.com/shervinazadi/Urban_Ergonomics
Author contributions
SA: Conceptualization, Data curation, Formal Analysis, Investigation, Methodology, Software, Visualization, Writing–original draft, Writing–review and editing. NB: Conceptualization, Formal Analysis, Investigation, Methodology, Resources, Writing–original draft, Writing–review and editing. PN: Conceptualization, Formal Analysis, Investigation, Methodology, Project administration, Supervision, Visualization, Writing–original draft, Writing–review and editing.
Funding
The author(s) declare that no financial support was received for the research, authorship, and/or publication of this article.
Conflict of interest
The authors declare that the research was conducted in the absence of any commercial or financial relationships that could be construed as a potential conflict of interest.
Publisher’s note
All claims expressed in this article are solely those of the authors and do not necessarily represent those of their affiliated organizations, or those of the publisher, the editors and the reviewers. Any product that may be evaluated in this article, or claim that may be made by its manufacturer, is not guaranteed or endorsed by the publisher.
References
Agusdinata, D. B. (2008). Exploratory modeling and analysis: a promising method to deal with deep uncertainty. Delft: TU Delft Open. \MakeLowercase []{ISBN} 9789071382260.
Algers, S., Eliasson, J., and Mattsson, L.-G. (2005). Is it time to use activity-based urban transport models? a discussion of planning needs and modelling possibilities. Ann. Regional Sci. 39 (4), 767–789. doi:10.1007/s00168-005-0016-8
Amanatides, J., and Woo, A. (1987). A fast voxel traversal algorithm for ray tracing. Eurographics 87 (3), 3–10. doi:10.2312/EGTP.19871000
Angel, S. (1968). Discouraging crime through city planning. University of California Institute of Urban and Regional Development.
Azadi, S., and Nourian, P. (2021). topoGenesis. original-date: 2020-05-31T13:29:16Z, Aug. 2021. Available: https://github.com/shervinazadi/topoGenesis(visitedon09/12/2021.
Bankes, S. (1993). Exploratory modeling for policy analysis. Operations Res. 41 (3), 435–449. \MakeLowercase []{ISSN} 0030-364X. doi:10.1287/opre.41.3.435
Batty, M. (2007). Cities and complexity: understanding cities with cellular Automata, agent-based models, and fractals. MIT Press. \MakeLowercase []{ISBN} 9780262524797.
Batty, M. (2001). Exploring isovist fields: space and shape in architectural and urban morphology. Environ. Plan. B Plan. Des. 28 (1), 123–150. 10.1068/b2725. Available: doi:10.1068/b2725
Belir, O., and Onder, D. (2013). Accessibility in public spaces: spatial legibility for visually impaired people.
Benedikt, M. L. (1979). To take hold of space: isovists and isovist fields. Environ. Plan. B Plan. Des. 6 (1), 47–65. doi:10.1068/b060047
Bentley, I. (1985). Responsive environments: a Manual for designers (butterworth architecture). London: Architectural Press. \MakeLowercase []{ISBN} 9780750605663.
Blanchard, P., and Volchenkov, D. (2008). Mathematical analysis of urban spatial networks. Springer Science and Business Media.
Bonacich, P. (1987). Power and centrality: a family of measures. Am. J. Sociol. 92 (5), 1170–1182. \MakeLowercase []{ISSN} 00029602, 15375390. doi:10.1086/228631
Byon, Y.-J., Abdulhai, B., and Shalaby, A. (2010). Incorporating scenic view, slope, and crime rate into route choices: emphasis on three-dimensional geographic information systems with digital elevation models and crime rate geospatial data. Transp. Res. Rec. 2183 (1), 94–102. doi:10.3141/2183-11
Chiu, Y.-H., Chuang, I.-T., and Tsai, C.-Y. (2020). Analyzing correlation of urban functionality and spatial configuration. Environ. Plan. B Urban Anal. City Sci. 48 (5), 1296–1313. doi:10.1177/2399808320924673
Conzen, M. R. G. (1960). Alnwick, northumberland: a study in town-plan analysis. Trans. Pap. 27, iii. doi:10.2307/621094
Cozens, P. M., Saville, G., and Hillier, D. (2005). Crime prevention through environmental design (cpted): a review and modern bibliography. Leeds: Property management.
Crooks, A., Croitoru, A., Lu, X., Wise, S., Irvine, J., and Stefanidis, A. (2015). Walk this way: improving pedestrian agent-based models through scene activity analysis. ISPRS Int. J. Geo-Information 4 (3), 1627–1656. doi:10.3390/ijgi4031627
Çubukçuoğlu, C., Nourian, P., Tasgetiren, M., Sariyildiz, I., and Azadi, S. (2021). Hospital layout design renovation as a Quadratic Assignment Problem with geodesic distances. J. Build. Eng. 44, 102952–952. doi:10.1016/j.jobe.2021.102952
Dalton, R., Bafna, S., Conroy Dalton, R., and Bafna, S. (2003). “The syntactical image of the city: a reciprocal definition of spatial elements and spatial syntaxes,” in 4th international space syntax symposium (London, UK), 17–19.
Daniel, M.-P., and Denis, M. (1998). Spatial descriptions as navigational aids: a cognitive analysis of route directions. Kognitionswissenschaft 7 (1), 45–52. 10.1007/bf03354963. Available:. doi:10.1007/bf03354963
DeLaurentis, D., and Callaway, R. K. (2004). A system-of-systems perspective for public policy decisions. Rev. Policy Res. 21 (6), 829–837. doi:10.1111/j.1541-1338.2004.00111.x
DeLyser, D. (1999). Authenticity on the ground: engaging the past in a California ghost town. Ann. Assoc. Am. Geogr. 89 (4), 602–632. doi:10.1111/0004-5608.00164
Denis, M., Pazzaglia, F., Cornoldi, C., and Bertolo, L. (1999). Spatial discourse and navigation: an analysis of route directions in the city of venice. Appl. Cogn. Psychol. 13 (2), 145–174. doi:10.1002/(sici)1099-0720(199904)13:2<145::aid-acp550>3.0.co;2-4
De Smith, M., Goodchild, M., Longley, P., and Longley, A. (2018). Geospatial analysis: a comprehensive guide to principles, techniques and software tools. London: Drumlin Security. \MakeLowercase []{ISBN} 9781912556045.
Duckham, M., and Kulik, L. (2003). ““simplest” paths: automated route selection for navigation,” in International conference on spatial information theory (Springer), 169–185.
Elias, B., Brenner, C., and Fisher, P., 2023 “Automatic generation and application of landmarks in navigation data sets. 469–480. doi:10.1007/3-540-26772-7_36
Epstein, J. M. (1999). Agent-based computational models and generative social science. Complexity 4 (5), 41–60. doi:10.1002/(sici)1099-0526(199905/06)4:5<41::aid-cplx9>3.3.co;2-6
Epstein, J. M., and Axtell, R. (1996). Growing artificial societies: social science from the bottom up. Cambridge, Massachusetts: Brookings Institution Press.
Fisher-Gewirtzman, D., Shashkov, A., and Doytsher, Y. (2013). Voxel based volumetric visibility analysis of urban environments. Surv. Rev. 45 (333), 451–461. \MakeLowercase []{ISSN} 00396265. doi:10.1179/1752270613Y.0000000059
Freundschuh, S. M., and Egenhofer, M. J. (1997). Human conceptions of spaces: implications for GIS. Trans. GIS 2 (4), 361–375. doi:10.1111/j.1467-9671.1997.tb00063.x
Frey, H. (1999). Designing the city: Towards a more sustainable urban form (designing the city: towards a more sustainable urban form). London: E and FN Spon. \MakeLowercase []{ISBN} 9780419221104.
Galea, S., Ahern, J., Rudenstine, S., Wallace, Z., and Vlahov, D. (2005). Urban built environment and depression: a multilevel analysis. J. Epidemiol. Community Health 59 (10), 822–827. doi:10.1136/jech.2005.033084
Gath-Morad, M., Thrash, T., Schicker, J., Hölscher, C., Helbing, D., and Aguilar Melgar, L. E. (2021). Visibility matters during wayfinding in the vertical. Sci. Rep. 11 (1), 1–15. doi:10.1038/s41598-021-98439-1
Gilbreth, L. M. (1914). The psychology of management: the function of the mind in determining, teaching and installing methods of least waste. New York: Sturgis and Walton Company.
Gjerde, M. (2011). Visual evaluation of urban streetscapes: how do public preferences reconcile with those held by experts? URBAN Des. Int. 16 (3), 153–161. doi:10.1057/udi.2011.10
Golledge, R. (1999). Wayfinding behavior: cognitive mapping and other spatial processes. Baltimore, London: Johns Hopkins University Press. \MakeLowercase []{ISBN} 9780801859939. Available: https://books.google.nl/books?id=TjzxpAWiamUC.
Golledge, R. G. (1993). “Chapter 2 geographical perspectives on spatial cognition,” in Behavior and environment, ser. Advances in psychology, T. Gärling, and R. G. Golledge 96, North-Holland, 16–46. doi:10.1016/S0166-4115(08)60038-2
Hagberg, A. A., Schult, D. A., and Swart, P. J. (2008). “Exploring network structure, dynamics, and function using networkx,” in Proceedings of the 7th Python in science conference. Editors G. Varoquaux, T. Vaught, and J. Millman (Pasadena, CA USA), 11–15.
Haq, S., and Luo, Y. (2012). Space syntax in healthcare facilities research: a review. HERD Health Environ. Res. Des. J. 5 (4), 98–117. 10.1177/193758671200500409. Available:. doi:10.1177/193758671200500409
Heppenstall, A. J., Crooks, A. T., See, L. M., and Batty, M. (2011). Agent-based models of geographical systems. Springer Science and Business Media.
Hillier, B. (1998). Space is the machine: a configurational theory of architecture. Cambridge University Press. \MakeLowercase []{ISBN} 9780521645287. Available: https://books.google.nl/books?id=8CygHAAACAAJ.
Hillier, B. (1999). The common language of space:a way of looking at the social,economic and environmental functioning of cities on a common basis. en 11 (3), 344–349. Available: http://www.jesc.ac.cn/jesc_en/ch/reader/view_abstract.aspx?file_no=19990316.
Hillier, B., and Hanson, J. (1989). The social logic of space. Cambridge University Press. \MakeLowercase []{ISBN} 9781139935685.
Hillier, B., Major, M., Desyllas, J., Karimi, K., Campos, B., and Stonor, T. (1996). Tate gallery, millbank: a study of the existing layout and new masterplan proposal.
Hillier, B., Penn, A., Hanson, J., Grajewski, T., and Xu, J. (1993). Natural movement: or, configuration and attraction in urban pedestrian movement. Environ. Plan. B Plan. Des. 20 (1), 29–66. doi:10.1068/b200029
Hoogendoorn, S. P., van Wageningen-Kessels, F. L., Daamen, W., and Duives, D. C. (2014). Continuum modelling of pedestrian flows: from microscopic principles to self-organised macroscopic phenomena. Phys. A Stat. Mech. its Appl. 416, 684–694. doi:10.1016/j.physa.2014.07.050
Jin, X., Long, Y., Sun, W., Lu, Y., Yang, X., and Tang, J. (2017). Evaluating cities’ vitality and identifying ghost cities in China with emerging geographical data. Cities 63, 98–109. doi:10.1016/j.cities.2017.01.002
Johnson, J., and Fisher, W. (2013). Urban geography: an introductory analysis (commonwealth and international library of science, technolog). Elsevier Science. \MakeLowercase []{ISBN} 9781483181547.
Katz, L. (1953). A new status index derived from sociometric analysis. Psychometrika 18 (1), 39–43. doi:10.1007/bf02289026
Klippel, A., and Winter, S. (2005). “Structural salience of landmarks for route directions,” in Spatial information theory. Editors A. G. Cohn, and D. M. Mark (Berlin, Heidelberg: Springer Berlin Heidelberg), 347–362.
Koohsari, M. J., Kaczynski, A. T., Giles-Corti, B., and Karakiewicz, J. A. (2013). Effects of access to public open spaces on walking: is proximity enough? Landsc. Urban Plan. 117, 92–99. doi:10.1016/j.landurbplan.2013.04.020
Koseoglu, E., and Onder, D. E. (2011). Subjective and objective dimensions of spatial legibility. Procedia - Soc. Behav. Sci. 30, 1191–1195. doi:10.1016/j.sbspro.2011.10.231
Kostakos, V. (2010). “Space syntax and pervasive systems,” in Geospatial analysis and modelling of urban structure and dynamics. Editors B. Jiang, and X. Yao (Dordrecht: Springer Netherlands), 31–52. doi:10.1007/978-90-481-8572-6_3
Li, X., Hijazi, I., Koenig, R., Lv, Z., Zhong, C., and Schmitt, G. (2016). Assessing essential qualities of urban space with emotional and visual data based on GIS technique. ISPRS Int. J. Geo-Information 5 (11), 218. 10.3390/ijgi5110218. Available:. doi:10.3390/ijgi5110218
Long, Y., and Baran, P. K. (2011). Does intelligibility affect place legibility? understanding the relationship between objective and subjective evaluations of the urban environment. Environ. Behav. 44 (5), 616–640. 10.1177/0013916511402059. Available:. doi:10.1177/0013916511402059
Lovelace, K. L., Hegarty, M., and Montello, D. R. (1999). “Elements of good route directions in familiar and unfamiliar environments,” in Spatial information theory. Cognitive and computational foundations of geographic information science. Editors C. Freksa, and D. M. Mark (Berlin, Heidelberg: Springer Berlin Heidelberg), 65–82.
Lynch, K., and for Urban Studies, J. C. (1960). The Image of the city (Harvard-MIT joint center for urban studies series). Harvard University Press. \MakeLowercase []{ISBN} 9780262620017.
Macal, C. M., and North, M. J. (2009). “Agent-based modeling and simulation,” in Proceedings of the 2009 winter simulation conference (WSC) (IEEE), 86–98.
McAndrew, F. (1993). Environmental psychology. Pacific Grove: Brooks/Cole. \MakeLowercase []{ISBN} 9780534193089. Available: https://books.google.nl/books?id=ip99AAAAMAAJ.
Meyer, M., Meyer, M., and Miller, E. (2001). Urban transportation planning: a decision-oriented approach (civil engineering series). McGraw-Hill. \MakeLowercase []{ISBN} 9780072423327.
Montello, D. R. (2007). “The contribution of space syntax to a comprehensive theory of environmental psychology,” in Proceedings of the 6th international space syntax symposium (Istanbul: Citeseer), 1–12.
Morello, E., and Ratti, C. (2009). A digital image of the city: 3D isovists in Lynch’s urban analysis. Environ. Plan. B Plan. Des. 36 (5), 837–853. \MakeLowercase []{ISSN} 14723417. doi:10.1068/b34144t
Moughtin, C. (2007). Urban design: street and square. London: Taylor and Francis. \MakeLowercase []{ISBN} 9781136350344.
Netto, V. M. (2015). “what is space syntax not?” reflections on space syntax as sociospatial theory. URBAN Des. Int. 21 (1), 25–40. doi:10.1057/udi.2015.21
Nothegger, C., Winter, S., and Martin, R. (2004). Selection of salient features for route directions. Spatial Cognition Comput. 4, 113–136. doi:10.1207/s15427633scc0402_1
Nourian, P. (2016). Configraphics: graph theoretical methods for design and analysis of spatial configurations. Delft: TU Delft. \MakeLowercase []{ISBN} 9789461867209.
Nourian, P., and Bai, N. (2021). “Reuse: configurational assessment of functionality of buildings before and after a change,” in Mastermind Crash: a method to reveal the impact of architectural redesign, ser. Mastermind Crash: a method to reveal the impact of architectural redesign. Editor A. Pereira Roders 3rd ed. (Delft: TU Delft Open), 23–73. Available: http://pure.tudelft.nl/ws/portalfiles/portal/88291501/CRASH_mastermind_2021_v3.pdf.
Nourian, P., Gonçalves, R., Zlatanova, S., Ohori, K. A., and Vo, A. V. (2016b). Voxelization algorithms for geospatial applications: computational methods for voxelating spatial datasets of 3d city models containing 3d surface, curve and point data models. MethodsX 3, 69–86. doi:10.1016/j.mex.2016.01.001
Nourian, P., Rezvani, S., Sariyildiz, I., and van der Hoeven, F. (2016a). “Spectral modelling for spatial network analysis,” in Proceedings of the symposium on simulation for architecture and urban design (simAUD 2016) (Delft: SimAUD).
Nourian, P., Van Der Hoeven, F., Rezvani, S., and Sariylidiz, S. (2015). Easiest paths for walking and cycling. London: 10TH INTERNATIONAL SPACE SYNTAX SYMPOSIUM.
Okamoto, K., Kaneda, T., Ota, A., and Meziani, R. (2014). Correlation analyses between underground spatial configuration and pedestrian flows by space syntax measures: a case study of underground mall complex in nagoya station. Tokyo: National Library of Australia Cataloguing-in-Publication entry, 116.
Omer, I., and Goldblatt, R. (2016). Using space syntax and q-analysis for investigating movement patterns in buildings: the case of shopping malls. Environ. Plan. B Urban Anal. City Sci. 44 (3), 504–530. 10.1177/0265813516647061. Available:. doi:10.1177/0265813516647061
ONeill, M. J. (1991). Evaluation of a conceptual model of architectural legibility. Environ. Behav. 23 (3), 259–284. 10.1177/0013916591233001. Available:. doi:10.1177/0013916591233001
Pacione, M. (2009). Urban geography: a global perspective. London: Taylor and Francis. \MakeLowercase []{ISBN} 9781134043095.
Pafka, E., Dovey, K., and Aschwanden, G. D. (2018). Limits of space syntax for urban design: axiality, scale and sinuosity. Environ. Plan. B Urban Anal. City Sci. 47 (3), 508–522. 10.1177/2399808318786512. Available:. doi:10.1177/2399808318786512
Page, L., Brin, S., Motwani, R., and Winograd, T. (1999). The pagerank citation ranking: bringing order to the web. Stanf. InfoLab, Tech. Rep.
Patle, B. K., Pandey, A., Parhi, D. R. K., and Jagadeesh, A. J. D. T. (2019). A review: on path planning strategies for navigation of mobile robot. Def. Technol. 15 (4), 582–606. doi:10.1016/j.dt.2019.04.011
Peinhardt, K. (2021). Resilience through placemaking: public spaces in rotterdam’s climate adaptation approach. Bonn: eng. Discussion Paper 1/2021, 2021. 10. 23661/dp1.2021. Available: http://hdl.handle.net/10419/228834.
Penn, A. (2003). Space syntax and spatial cognition. Environ. Behav. 35 (1), 30–65. 10.1177/0013916502238864. Available:. doi:10.1177/0013916502238864
Peponis, J., Ross, C., and Rashid, M. (1997a). The structure of urban space, movement and co-presence: the case of atlanta. Geoforum 28 (3-4), 341–358. doi:10.1016/s0016-7185(97)00016-x
Peponis, J., Wineman, J., Rashid, M., Kim, S. H., and Bafna, S. (1997b). On the description of shape and spatial configuration inside buildings: convex partitions and their local properties. Environ. Plan. B Plan. Des. 24 (5), 761–781. doi:10.1068/b240761
Perloff, R., and Naman, J. L. (2012). Lillian gilbreth: tireless advocate for a general psychology. Portraits Pioneers Psychol. Volume II 2, 107.
Proshansky, H. M. (1978). The city and self-identity. Environ. Behav. 10 (2), 147–169. 10 . 1177/0013916578102002. Available:. doi:10.1177/0013916578102002
Ratti, C. (2004a). Rejoinder to hillier and penn. Environ. Plan. B Plan. Des. 31 (4), 513–516. doi:10.1068/b3019b
Ratti, C. (2004b). Space syntax: some inconsistencies. Environ. Plan. B Plan. Des. 31 (4), 487–499. doi:10.1068/b3019
Rosser, G., Davies, T., Bowers, K. J., Johnson, S. D., and Cheng, T. (2017). Predictive crime mapping: arbitrary grids or street networks? J. quantitative Criminol. 33 (3), 569–594. doi:10.1007/s10940-016-9321-x
Schelling, T. (1984). Choice and consequence. Harvard University Press. \MakeLowercase []{ISBN} 9780674127715. Available: https://books.google.nl/books?id=MF08nRe6jQoC.
Schlicht, E. (2012). Isolation and aggregation in economics. Springer Berlin Heidelberg. \MakeLowercase []{ISBN} 9783642702983. Available: https://books.google.nl/books?id=o6%5C_tCAAAQBAJ.
Schroder, C. J., Mackaness, W. A., and Gittings, B. M. (2011). Giving the “right” route directions: the requirements for pedestrian navigation systems. Trans. GIS 15 (3), 419–438. doi:10.1111/j.1467-9671.2011.01266.x
Simon, H. (1970). The sciences of the artificial (karl taylor compton lectures). Cambridge: Tun Huang Book Company. \MakeLowercase []{ISBN} 9780262690232. Available at: https://books.google.nl/books?id=mjZgAAAAMAAJ.
Sorrows, M. E., and Hirtle, S. C. (1999). “The nature of landmarks for real and electronic spaces,” in Spatial information theory. Cognitive and computational foundations of geographic information science. Editors C. Freksa, and D. M. Mark (Berlin, Heidelberg: Springer Berlin Heidelberg), 37–50.
Stamps, A. E. (2005). Isovists, enclosure, and permeability theory. Environ. Plan. B Plan. Des. 32 (5), 735–762. 10.1068/b31138. Available:. doi:10.1068/b31138
Steiniger, S., Lange, T., Burghardt, D., and Weibel, R. (2008). An approach for the classification of urban building structures based on discriminant analysis techniques. Trans. GIS 12 (1), 31–59. doi:10.1111/j.1467-9671.2008.01085.x
Stephenson, J. (2008). The cultural values model: an integrated approach to values in landscapes. Landsc. Urban Plan. 84, 127–139. doi:10.1016/j.landurbplan.2007.07.003
Tobler, W. R. (1970). A computer movie simulating urban growth in the detroit region. Econ. Geogr. 46 (1), 234–240. doi:10.2307/143141
Turner, A. (2001). “Angular analysis,” in Proceedings of the 3rd international symposium on space syntax (Atlanta, GA: Georgia Institute of Technology), 30–31.
Turner, A. (2007). Ucl depthmap 7: from isovist analysis to generic spatial network analysis. Istanbul: New Developments in Space Syntax Software.
Turner, A., Doxa, M., O’Sullivan, D., and Penn, A. (2001). From isovists to visibility graphs: a methodology for the analysis of architectural space. Environ. Plan. B Plan. Des. 28 (1), 103–121. 10.1068/b2684. Available:. doi:10.1068/b2684
Turner, A. (2003). Analysing the visual dynamics of spatial morphology. Environ. Plan. B Plan. Des. 30 (5), 657–676. 10 . 1068/b12962. Available:. doi:10.1068/b12962
Turner, A., and Penn, A. (1999). “Making isovists syntactic: isovist integration analysis,” in Proceedings of of second international symposium on space syntax, 103–121.
Vance, J. (1977). This Scene of man: the Role and Structure of the City in the Geography of western civilization (harper and row series in geography). Harper’s College Press. \MakeLowercase []{ISBN} 9780061674075.
van der Hoeven, F., and van Nes, A. (2014). Improving the design of urban underground space in metro stations using the space syntax methodology. Tunn. Undergr. Space Technol. 40, 64–74. doi:10.1016/j.tust.2013.09.007
Wang, R., Liu, Y., Xue, D., and Helbich, M. (2019a). Depressive symptoms among Chinese residents: how are the natural, built, and social environments correlated? BMC public health 19 (1), 887–888. doi:10.1186/s12889-019-7171-9
Wang, Z., Liang, Q., Duarte, F., Zhang, F., Charron, L., Johnsen, L., et al. (2019b). Quantifying legibility of indoor spaces using deep convolutional neural networks: case studies in train stations. Build. Environ. 160, 106099–099. doi:10.1016/j.buildenv.2019.04.035
Weisman, J. (1981). Evaluating architectural legibility. Environ. Behav. 13 (2), 189–204. 10 . 1177/0013916581132004. Available:. doi:10.1177/0013916581132004
Keywords: spatial ergonomics, accessibility, visibility, architectural morphology, urban morphology, simulation models
Citation: Azadi S, Bai N and Nourian P (2024) Ergonomics of spatial configurations: a voxel-based modelling framework for accessibility and visibility simulations. Front. Built Environ. 9:1300843. doi: 10.3389/fbuil.2023.1300843
Received: 23 September 2023; Accepted: 22 December 2023;
Published: 29 January 2024.
Edited by:
Yongkui Li, Tongji University, ChinaReviewed by:
Hasim Altan, Prince Mohammad bin Fahd University, Saudi ArabiaSheila Walbe Ornstein, University of São Paulo, Brazil
Copyright © 2024 Azadi, Bai and Nourian. This is an open-access article distributed under the terms of the Creative Commons Attribution License (CC BY). The use, distribution or reproduction in other forums is permitted, provided the original author(s) and the copyright owner(s) are credited and that the original publication in this journal is cited, in accordance with accepted academic practice. No use, distribution or reproduction is permitted which does not comply with these terms.
*Correspondence: Pirouz Nourian, cC5ub3VyaWFuQHV0d2VudGUubmw=
†ORCID: Shervin Azadi, orcid.org/0000-0002-8610-2774; Nan Bai, orcid.org/0000-0001-7637-3629; Pirouz Nourian, orcid.org/0000-0002-3817-7931