- 1Department of Mechanical Engineering, Universidad Tecnológica de Panamá, Panama City, Panama
- 2Research Group—Iniciativa de Integración de Tecnologías para el Desarrollo de Soluciones Ingenieriles (I2TEDSI), Panama, Panama
- 3Sistema Nacional de Investigación (SNI), Clayton, Panama
Construction materials derived from agro-industrial waste are increasingly attractive in the building sector, due to their sustainability and lower environmental impact. Hence, in recent years worldwide the amount of research and publications tending to the development of materials that take advantage of residues from agro-industrial activities has increased. The role of thermal insultation materials in the building envelope is significant, especially in hot-humid region. This study presents the manufacturing and evaluation of a cellulose and rice husk-based insulation material, as a proposal for the reuse of materials considered as value-added waste, such as recycled paper and rice husks. Boards and test specimens were elaborated, as well as mechanical and thermal tests. The material was evaluated by means of thermal tests, in accordance with ASTM C177, to measure the thermal conductivity. Tensile and compressive strength tests were performed, based on ASTM C209 and ASTM C39 Standard, respectively. According to the results obtained, the material shows a thermal coefficient of 0.04 W/m∙K which corresponds to a material with the potential to thermally insulate an enclosure. Maximum stresses were obtained for the 3 compositions in average for a range between 1.31 and 1.76 MPa. Ultimate compressive strength obtained was between 20.19 and 21.23 MPa. The proposed material is presented as a sustainable alternative, which can be used in the field of environmentally friendly buildings, which contribute to reducing the carbon footprint, by energy savings.
1 Introduction
Building is the second largest sector in plastic consumption, remaining in range of 16% and 23%. Also, this sector consumes around 30% and 40% of energy consumption, responsible for more than 33% of energy related greenhouse gases emission in the world (Lopez Hurtado et al., 2016; Kumar et al., 2020; Pal et al., 2021; Zhao et al., 2022). Traditional insulation material used in building applications are mostly manufactured from petrochemical sources (Carlos Javier et al., 2023). Production and manufacturing processes of construction materials account a higher carbon footprint, polluting air, land, and water (Maraveas, 2020). Construction materials derived from agro-industrial waste are increasingly attractive in the construction sector, due to their sustainability and lower environmental impact. Hence, in recent years the amount of research and publications tending to the development of materials that take advantage of residues from agro-industrial activities has increased (Chabannes et al., 2014; Viegas et al., 2014; Lopez Hurtado et al., 2016; Liu et al., 2017; Antunes et al., 2019; Dixit and Yadav, 2019; Muthuraj et al., 2019; Maraveas, 2020; Moreno et al., 2021; Nandy et al., 2021; Zou et al., 2021; Ali et al., 2022; Amantino et al., 2022; Carlos Javier et al., 2023), to reduce the environmental impact generated by commercial materials such as fiberglass, rock wool, expanded polystyrene, polyurethane foam, polystyrene, phenolics, among other non-biodegradable materials. Thus, in the scientific literature studies have been reported with insulating materials based on cane fibers, coconut tow, cassava, rice, wood, coffee husks, cork, sheep’s wool, recycled newsprint, textile fibers, trays egg, and others.
One of the main characteristics of the use of natural fibers, in addition to their thermal resistance, is that they have low energy (gray energy or embodied energy) in their production process, transportation and to get the final product (Dixit et al., 2010; Pal et al., 2021). Normally the natural fibers used as insulation are the result of other processes, as in the case of rice husks and sugar cane, agricultural by-products are grown in large proportions.
Cellulose, which is obtained from recycled and shredded newspaper, is the most widely used thermal insulator, thanks to its thermoacoustic properties. Cellulose fiber is obtained mainly from the separation of newsprint by physical methods; material that is then processed and shredded (Lopez Hurtado et al., 2016; Garzón Bernal and Cuba Córdoba, 2020). Cellulose insulation can come as a pre-cast panel, in which cellulose fibers are molded with polyester or a similar binder, or as loose fibers, which are applied by hand or specialized equipment, in attics, ceilings, or walls. The first use of cellulose fiber as an insulating material date back to 1919 in Canada, but it was not until 1950 that commercial products were developed in the US. Pulp application gained popularity in the 1970s due to the US oil embargo (Lopez Hurtado et al., 2016).
The rice husk constitutes a residue, when the grain is separated from the husk when going through the piling process and represents around 20% of the gross weight of harvested rice. Rice hulls are mainly made up of carbon, cellulose, and silica (Dixit and Yadav, 2019). Silica represents 20% of rice husk, which makes it resistant to water penetration, thus protecting the rice grain when it is inside. Also, this material has been studied due to its thermal insulation properties when mixed with different types of binders and other materials, such as newsprint cellulose (Buratti et al., 2018). Rice husk has also been used as an aggregate material in concrete called “bio-aggregate concrete”, and in another study it replaces the use of aggregates such as sand in percentage, thus achieving a lighter material, with excellent performance as thermal insulator (Amantino et al., 2022). There are also studies on the potential as a thermal insulator of carbonized rice husks (Dixit and Yadav, 2019) and ash (Antunes et al., 2019; Muthukrishnan et al., 2019; Zou and Yang, 2019; Maraveas, 2020).
Globally, the construction sector represents between 30% and 40% of energy consumption (Lopez Hurtado et al., 2016; Das and Tiwari, 2018; Carlos Javier et al., 2023). During the design or conditioning of buildings, from the point of view of heat transfer, it is necessary to consider all the materials used during construction (specifically the thicknesses and thermal conductivity coefficient of each material), as well as outdoor and indoor temperatures. On the other hand, about 20% of the total energy consumption in buildings worldwide corresponds to the use of air conditioners (Hu et al., 2019; Bienvenido-Huertas et al., 2020). In this case, the role of thermal insultation materials in the building envelope is significant, especially in hot-humid region. The envelope is recognized as a key part for enhancing their thermal efficiency since it expends between 50% and 60% of the whole heat transfer (Kumar et al., 2020; Zhao et al., 2022).
According to the reviewed research, to establish sustainable houses and buildings or “green buildings and neighborhoods” (Zhang et al., 2019), as strategies to reduce energy consumption, it is necessary to verify the possibility of implementing new insulating materials, lighting changes and the implementation of systems that include renewable energy, prior to a more detailed feasibility study.
As a contribution in the generation of new materials from waste materials, this work presents the methodology that was carried out to develop an alternative insulation material based on newspaper (cellulose) and rice husk. Mechanical and thermal tests were performed. Moreover, with the results obtained, it is expected to contribute as a basis for future research in the field of construction materials derived from agro-industrial waste, as an alternative to be used in the field of environmentally friendly buildings.
2 Literature review: Cellulose and rice husk-based material
Cellulose, which is obtained from recycled and shredded newspaper, is the most widely used thermal insulator. Cellulose fiber insulation has lower value of embodied energy and environmental impact compared to conventional insultation materials (Pal et al., 2021). Commercially it can be found in the form of a semi-rigid mat (boards) or blown insulation (loose-fill cellulose insulation). Cellulose boards as a thermal insulating material are usually used as fillers in false ceilings, partitions, doors, and furniture (Moreno et al., 2021). It can also be found as loose fibers, those that are applied manually or with specialized equipment, in attics, ceilings or walls. In recent years, there have been important efforts to improve the energy efficiency of buildings, through the application of thermal insulation in the building envelope, which includes the reduction of the thermal bridge effect, that involves the loss of energy at different points of the envelope (Lopez Hurtado et al., 2016).
Cellulose has been compared with thermal insulators made from mineral fibers, finding that its insulation capacity (R value) remains constant under sudden temperature changes. Likewise, the cellulose-based insulation offers low embodied energy, an important factor when selecting a material. Energy incorporated per kilogram of cellulose is between 0.94 and 3.3 MJ/kg, a reduced range of values compared to commercial thermal insulation that is between 10.8 and up to 45 MJ/kg (Lopez Hurtado et al., 2016; Pal et al., 2021).
In boards made from recycled paper, textile fibers and glue, values of the thermal conductivity coefficient (k) between 0.034 and 0.039 W/m∙K have been reported (Ricciardi et al., 2014; Pal et al., 2021) and 0.040 W/m∙K, when it is applied by blowing technics (Carlos Javier et al., 2023). Similarly, foamed cellulose has reported k of 0.039 W/m∙K (Yeon et al., 2014). In general, typical values of the thermal conductivity coefficients of cellulose fibers are around 0.040 W/m∙K (Lopez Hurtado et al., 2016). This can be explained by the microstructure of cellulose-based products involving voids, some of them connected and others not (Aksogan et al., 2018), depending on the arrangement of the components combined with the cellulose.
It should be noted that the values of k may vary depending on the manufacturing process, installation method, as well as the origin of the raw material. There is an inversely proportional relationship between conductive heat transfer and relative humidity (González-Serrud et al., 2022). The higher the relative humidity is present in the boards, the heat transfer with the environment decrease. In the case of cellulose fibers, due to their hygroscopic nature, moisture absorption can affect the final values of thermal conductivity (Lopez Hurtado et al., 2016). Hygroscopic cellulose insulation can be used to control indoors air humidity, increasing indoor thermal confort (Pal et al., 2021).
Fiber board from cellulose and without the use of additional bonding, using biodegradable, renewable and recyclable materials is also reported with a Young’s modulus of 17 GPa and a strength of 120 MPa (Arévalo and Peijs, 2016).
Likewise, when mixing cellulose with other materials, such as perlite and gypsum, k values between 0.083 and 0.12 W/m∙K are obtained (Aksogan et al., 2018), which, although not so low, correspond to studies that seek to improve other characteristics in cellulose, such as fire retardant and fungicide.
Rice husk insulation is made from the outer protective covering of rice grains, which is typically discarded as waste during the rice milling process. Rice husks are abundant, renewable, and readily available in many rice-producing regions, making them an attractive choice for sustainable insulation. Rice husk insulation is an emerging and innovative insulation material to create a sustainable and efficient thermal barrier. Rice husk insulation has been studied for its thermal properties, and it has been found that the addition of rice husk fibers to thermal insulation wallboards can decrease their thermal conductivity and increase their insulation performance. A k value of 0.047 W/m∙K was reported for a rice husk particle. Rice husk-earth based composite present k values between 0.102 and 0.197 W/m∙K, with different rice husk content (above 15%). Also, increase on rice husk content in defined proportion could produce decreases in thermal conductivity due to bulk density decrease (Antunes et al., 2019).
Studies that have been carried out on natural fibers materials have shown its good insulating characteristics when mixed with different types of binders. For example, in a study carried out on rice husks and cassava starch as a binder, a thermal conductivity of 0.0262 W/m∙K was obtained (Canto et al., 2018). In the work carried out in Universidad Tecnológica de Panamá, Azuero, thermal conductivity coefficient of 0.073 W/m°C and 0.049 W/m∙K, were obtained for rice husk and rice straw boards, respectively; these values are in the range of thermal insulators that goes from 0.020 to 0.080 W/m∙K (Gonçalves and Bergmann, 2007).
Material fibers that contain cellulose have a high resistance, as well as a low density, which is interesting when it comes to meeting thermal insulation needs in conjunction with lightweight construction systems. Regarding mechanical characterization results, specifically, compressive stress between 0.25 and 0.29 MPa have been reported in material based on newsprint, perlite, and plaster. The high compressive strengths of the samples added with zinc borax (between 0.35 and 0.45 MPa) could be due to the denser matrix formed by zinc borax compared to those formed by the other additives (Aksogan et al., 2018). In another material based on rice husk and geopolymer, a compressive strength between 0.26 and 7.24 MPa was obtained (Wang et al., 2020). A biocomposite made from rice husk, wheat husk, wood, and textile fibers, bonded with a biodegradable polymer, demonstrated compressive strength between 11 and 40 MPa (Muthuraj et al., 2019). Rice husk insultation material bonded with lime, presented compressive strength of 0.33 MPa and after 60 days of setting, and with a hemp insulation material the compressive strength was 0.48 MPa (Chabannes et al., 2014). In a carbonized wheat straw composite insultation cementing with a geopolymer, the compressive strength was between 0.49 and 0.82 MPa (Zou et al., 2021). Panels based on cardboard, cement, and sand reported values from 1.494 to 6.3 MPa in compressive strength, considering that strength increases with curing time. The tensile strength for a material based on cardboard, cement and sand is between 0.987 and 2.098 MPa. As in the case of compressive strength, it improves as the days of curing time (Ahmad et al., 2021).
Even though cellulose and rice husk based insultation material presents advantages to the environment, efforts must still be made in the optimization and development of these materials in terms of their properties, manufacturing, installation, and performance as a final product, also considering the origin of the raw material. Research has focused on the search for alternative raw materials that reduce or replace chemical additives with fungicidal and fire-retardant characteristics (Nagieb et al., 2011; Ricciardi et al., 2014; Yeon et al., 2014; Lopez Hurtado et al., 2016; Aksogan et al., 2018). In general, when it comes to cellulose and natural fibers, a percentage of boric acid and borax is added to give the material resistance to the appearance of fungi and give it fire retardant properties. The concentrations of borax and boric acid reported are between 15% and 20% of the total mass of cellulose-based fiber (Lopez Hurtado et al., 2016). Other authors indicate that the ideal concentration of fire-retardant materials to achieve good resistance to the flame is found to be 5% (Nagieb et al., 2011). Carbonized biomass partially and adopting fireproof inorganic cementing materials were proposed to enhance the flame retardancy in naturals materials as wheat straw insultation materials (Zou et al., 2021).
Mechanical, thermal, and acoustic study of polymer-based composite materials derived from expanded cork and rice husk by-products demonstrated that the incorporation of rice husk grains in composite formulations helps reduce thermal conductivity by 20% compared to composites without rice husk. Increasing rice husk content in composites can contribute to improved acoustic performance, with sound absorption up to 0.6 at low frequencies. Composite materials produced with expanded cork by-products and rice husk present a mechanical behavior suitable for use in construction systems such as walls and floors. An assessment of the suitability of these composite materials in building systems, such as walls and floors, was conducted, and the most suitable formulations were selected through a multi-criteria analysis. The findings suggest that construction solutions utilizing these composite materials can be applied in buildings, contributing to reduced energy consumption over the buildings’ operational lifetimes (Marques et al., 2020).
Bio composite made from recycled materials, specifically peanut shells, and rice husks, reinforced with polypropylene exhibited impressive tensile and flexural strength, as well as offering qualities such as thermal insulation, sound absorption, aqueous stability, and flame resistance. The thermal conductivity of these composites ranged from 0.156 to 0.270 W/m∙K. In summary, the article suggests that bio composites derived from agro-industrial waste can serve as a sustainable and environmentally friendly alternative to traditional building materials (Guna et al., 2020).
In other study, biocarbon was produced from hemp and switchgrass via pyrolysis at three different temperatures (450, 550, and 650°C). Composites were created with varying concentrations of biocarbon fillers and sizes. Biocarbon from both plants significantly reduces water absorptivity when compared to unfilled hemp fiber-reinforced composites. Tensile strength showed improvement with hemp biocarbon and switchgrass biocarbon, with the maximum tensile strength (10 wt% biocarbon of 50 microns, produced at 650°C). Other properties such as energy at tensile rupture, flexural strength, and impact strength varied based on particle size, filler loading, and pyrolysis temperature. Thermal conductivity was affected by filler-loading and particle size. The average thermal conductivity of the composite with hemp was 0.059 W/m∙K, while that of the switchgrass was 0.058 W/m∙K (Dahal, 2023).
Natural fibers are a renewable resource, making them a sustainable and environmentally friendly choice compared to synthetic fibers (Vidal et al., 2023). They also tend to have lower embodied energy and a smaller carbon footprint, further reducing the environmental impact of composite materials (Karimah et al., 2021). However, it is worth noting that natural fibers can exhibit more variability in properties compared to synthetic fibers, potentially affecting consistent performance in composite materials. Additionally, their susceptibility to moisture absorption can impact their mechanical properties and long-term durability (Dahal, 2023; Dahal et al., 2023; Martínez et al., 2023). Nonetheless, research is actively addressing these limitations through innovative processing techniques and the application of bio-based binders and coatings in order to improve the performance of natural fiber composites (Vidal et al., 2023).
Overall, both cellulose and rice husk insulation materials offer thermal insulation properties and can be used in construction. As insulation materials have their advantages and are considered environmentally friendly options. The choice between them may depend on factors such as availability, cost, and specific project requirements. Rice husk insulation, on the other hand, is derived from rice husks and offers the advantage of utilizing agricultural waste. It has been found to improve the insulation performance of wallboards.
3 Materials and methods
3.1 Materials and samples preparation
The insulating agro-waste-material, based in recycled newspaper and rice husk, were manufactured by wet process, mechanical and chemical treatments. Overall, it consists of 5 main steps: grinding, mixing, moulding, pressing, and drying. The cellulose was obtained from 100% recycled newspaper paper, which is mechanically separated. This material should be converted into slurry pulp. The rice husk was shredded by mechanical grinding, and particles around 0.6 mm were obtained. The rice husk and newspaper are mixed, then, borax is added. All the components were bonded with glue, then moulded and pressed with masses of 15 lbs (6.8 kg), which exert a constant pressure on the entire surface of the material. The glue used consisted of a mixture between white glue and water, in proportions defined according to previous experiences in 10 oz of glue with 1 oz of water. The pressure is maintained for at least 2 days to ensure bonding. The drying process is carried out at room temperature. This experience was performed in hot-humid region, in Panama. Due to the humid conditions, this process takes approximately 2 weeks in the sun. After this time has elapsed, the samples are ready to be tested.
An experimental program was carried out on trial-and-error basis, adopting three compositions with different percentages of rice husk and cellulose (Table 1). In each mixture, 15% borax and 62% glue were kept constant. Three units of each test were prepared, according to ASTM (American Society for Testing and Materials) standards: 3 boards for thermal conductivity coefficient measurements, 9 samples for tensile test (according to ASTM C209 Standard) (ASTM C209-20, 2020) and 9 samples for compression test (cylindrical test samples according to ASTM C39 Standard) (ASTM C39/C39M-21, 2021). The boards dimensions were 30 cm × 30 cm x 1.5 cm, according to the ASTM C177 Standard (ASTM C177-19, 2019). The compressive tests are carried out on a cylindrical sample of 4 inches depth and 2 inches diameter (ø). Tensile stress sample geometry is shown in Figure 1. Molds were manufactured, considering ASTM standards.
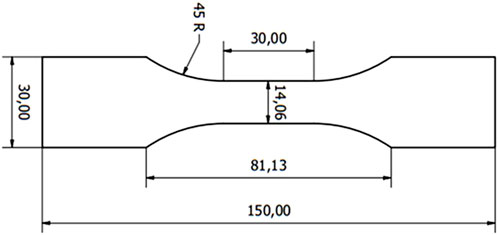
FIGURE 1. Tensile stress sample (ASTM C209-20, 2020).
3.2 Mechanical and thermal tests
The equipment used to carry out the tensile tests was Autograph AGS-J, for universal testing, available at Laboratorio de Análisis Industriales y Ciencias Ambientales (LABAICA) of the Universidad Tecnológica de Panamá (Figure 2). It works by applying a controlled force, then register the load (in Newtons) vs. elongation (in mm) curves.
The equipment used to carry out the compression tests is an ELE International Accu-Tek Compression Machine, available at Laboratorio de Suelos y Ensayo de Materiales de Azuero, Universidad Tecnológica de Panamá (Figure 3). During the test, an axial load is applied until either the material fails, or the piston fully disengages. A total of three tests were carried out for each of the defined compositions, as indicated by the standard as the minimum number of test tubes tested for the result to be considered valid. According to ASTM C39, the compressive stress is calculated to the nearest 0.1 MPa. The L/D ratio is obtained for each of the cylindrical specimens, where L corresponds to the length and D to the average diameter of the cylinder. The table 2 shows the correction factors according to the ASTM C39 Standard (ASTM C39/C39M-21, 2021).
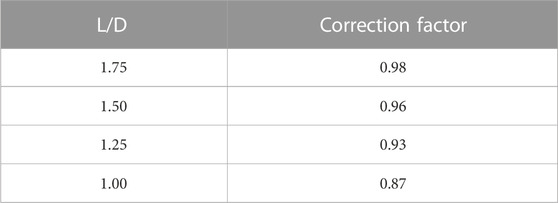
TABLE 2. Correction factors for the L/D ratio, according to ASTM C39 Standard (ASTM C39/C39M-21, 2021).
The thermal conductivity coefficient was measured with a “hot box” apparatus, according to ASTM-C177 standard (ASTM C177-19, 2019). The “hot box” device is based on Fourier’s Law of heat conduction. It consists of a box made of wood, with one side open. It contains a heat source inside (incandescent bulb of known power), installed on one of its sides and a DHT22 temperature sensor with Arduino (Figures 4A, B). The solid material to be evaluated is placed on the open side. The internal walls of the apparatus are well insulated, to minimize conduction losses and to produce and unidirectional heat flux through the solid material to be evaluated.
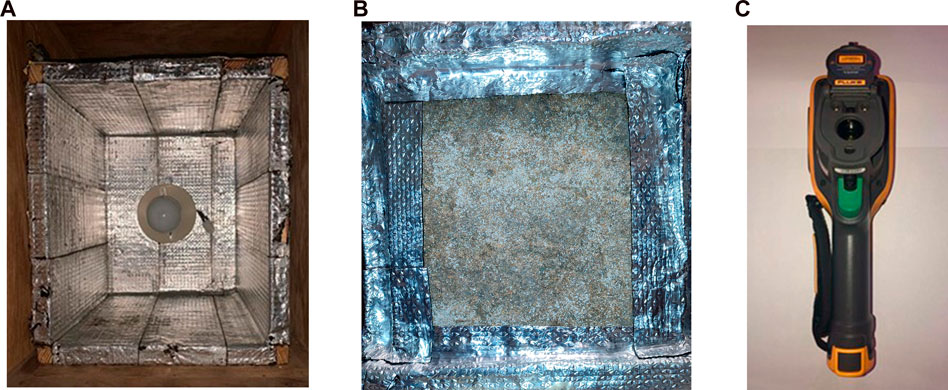
FIGURE 4. Thermal conductivity coefficient measurement device, (A) inside, (B) outside, and (C) thermographic camera Fluke Ti110.
The main objective of this device is to measure the temperature gradient, measuring the temperatures inside and outside the box. The outside temperature measurements are carried out using a thermographic camera (FLUKE Ti 110) (Figure 4C). To maintain a steady state, the system must be given sufficient time to complete the test or ensuring stationary test conditions.
The thermal conductivity coefficient (k) of the panel can be calculated by means of the following Eq. 1:
where L is the thickness of the panel (0.015 m), A is the area of the panel (0.09 m2),
The methodology is summarized in Figure 5.
4 Results and discussion
4.1 Tensile stress
Tensile strength tests were performed, based on ASTM C209. In this test, an increasing axial force was applied to the sample, until it fractured, this to know its resistance under stress conditions and the elongation produced. Three test samples were generated for each composition. In Figure 6 it is shown, the tensile stress (MPa) vs. deformation (mm/mm) curves obtained for 3 samples of 3 different compositions. The curves show a similar trend.
Table 3 shows the maximum stress of each of the tensile samples and the average stress obtained for each of the studied compositions.
Significance in the maximum tensile stress data differences was analyzed under the same conditions, using the one-way analysis of variance (ANOVA), by means of Minitab software, at 95% confidence level (Figure 7). For this case in which 3 compositions were analyzed, it is verified that the data tend to a straight line, so the assumption of normality is accepted.
Maximum stresses were obtained for the 3 compositions in average for a range between 1.31 and 1.76 MPa. Tensile strength values of 1.953 MPa have been reported for a material based on cardboard, cement, and sand, in 1:1:2 proportions, after 28 days of curing (Ahmad et al., 2021).
According to Ahmad et al., 2021, the tensile capacity of a cardboard pulp-based material is lower than that normally obtained in conventional unreinforced concrete (between 2.2 and 4.2 MPa). The addition of fibers could provide greater tensile strength, since they not only minimize tensile failure, but can also improve impact and break strength, compressive strength, impact strength, to name a few. For this reason, the addition of fibers was suggested to improve the tensile strength of the material developed from cardboard pulp (Ahmad et al., 2021). In this study, in the material developed, average tensile strength values of 1.71, 1.31, and 1.76 MPa were obtained for the three compositions studied, which is in accordance with what was previously stated. Materials based on cardboard, cement and sand have presented resistances comparable to that obtained in this study.
Despite the fact that the results are consistent with what has been reported in the scientific literature, it would be convenient to evaluate other configurations, such as, for example, the addition of long fibers in only one direction to reinforce the behavior of the material in tension, since an insulating material that it is placed as part of the envelope of a building does not have a great resistance to tension as an essential condition, it could be desirable in structural cases.
4.2 Compressive stress
In Figure 8 is shown the ultimate compressive stress in MPa, for average values of the 3 compositions.
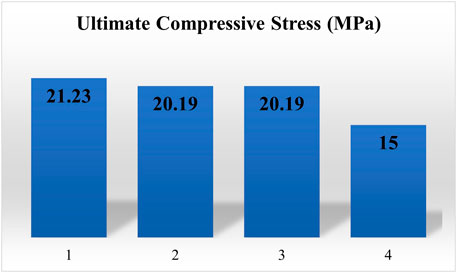
FIGURE 8. Ultimate compressive strength (average value of 3 compositions): 1) Sample 1; 2) Sample 2; 3) Sample 3; 4) Muthuraj et al., 2019.
The compressive strength of the developed material is comparable to that obtained by Muthuraj et al., 2019. The compressive strength value obtained in each of the tested specimens allows us to verify the possibility of using the developed material in construction applications such as case of insulating materials in building envelopes.
It should be noted that the load applied to the specimens does not correspond to the ultimate load that they can resist, but because the piston exceeded the allowable calibration limit, the compression machine stopped. In Figure 9, it is shown that the samples absorbed the force applied by the piston.
Regarding the compressive strength, the values obtained are between 20.19 and 21.23 MPa. In Muthuraj et al., 2019, for a material based on rice husk, a compressive strength of 15 MPa was obtained. In this sense, through the tests carried out, it was possible to verify that the resistance of the developed material presented values like those reported in the literature. It should be noted that this property allows verifying the integrity of the material and its behavior under compression, considering its performance when installed inside a building. Although it is true, it is not a material that must support structural loads, it must be capable of not fracturing during its installation or during its operation as an insulator.
Within the general analysis of the manufacturing process of the composite material, it was possible to determine very favorable results when verifying its behavior in tension and compression, even so, considering that the rice husk can be crushed to obtain particles smaller than 0.6 mm.
4.3 Thermal conductivity measurements
Thermal conductivity coefficient, obtained by “hot box” methodology, are reported in Table 4. Three tests were conducted for each sample. During measurements, lasted for about 2 h, considered as sufficient time to complete the test or ensuring stationary test conditions. Thermal conductivity is obtained with Eq. 1 and the data presented in Table 4. In Figure 10, it is shown the thermal photographs of the outer surface of the plates tested in the Thermal Conductivity “hot box”, for each sample.
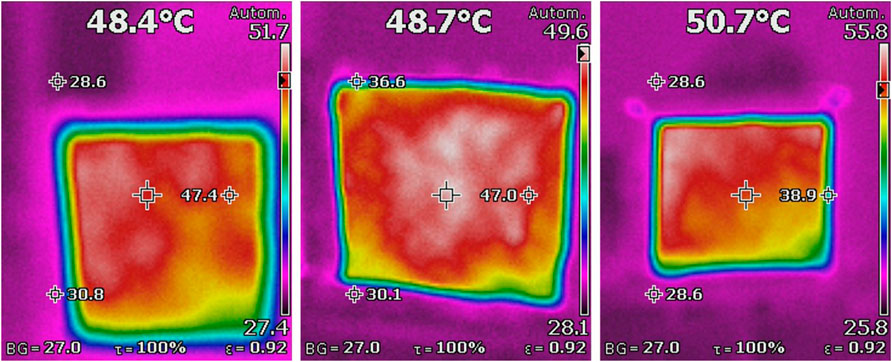
FIGURE 10. Thermal photographs of the outer surface of the plates tested in the Thermal Conductivity “hot box”. From left to right; (A) sample 1, (B) sample 2, (C) sample 3.
For traditional materials, the values range from 0.03 to 0.06 W/m∙K and for organic insulation materials, the values range from 0.03 to 0.1 W/m∙K (Janowska-renkas et al., 2022; Zhao et al., 2022; Carlos Javier et al., 2023). The choice of which material to use in any specific application will depend on a variety of factors, including but not limited to, material characteristics, binder in the mixtures, humidity, cost, ease of installation, and sustainability. Also, the thermal conductivity of thermal insulations materials depends on the density and humidity (Azzouzi et al., 2020; Ntimugura et al., 2020; Carlos Javier et al., 2023).
Rigid polyurethane, polyethylene foam, expanded and extruded polystyrene foam, and low-density polyethylene, have thermal conductivities ranging from 0.027 to 0.33 W/m∙K (Zabalza Bribián et al., 2011). This range indicates that thermal conductivity can vary considerably between different polymers. Rigid polyurethane and polystyrene foams are found to be particularly effective as thermal insulators, with thermal conductivity values of 0.032 and 0.031–0.0375 W/m∙K, respectively. Natural and organic fibers such as cotton, sheep wool, and various plant fibers (coconut, sisal, jute, banana, and pineapple) exhibit thermal conductivities in a similar range to polymers, with values between 0.034 and 0.07 W/m∙K (Faruk et al., 2012). These materials have the additional benefit of being renewable and biodegradable, which makes them attractive from a sustainability perspective. When comparing these with more traditional materials, such as pumice, cork, and cellulose, it is observed that their thermal conductivity is in a comparable range. However, the specific value for each material depends largely on its individual characteristics.
In the presented study, experimental results showed a good thermal behavior of all the investigated compositions: k-values vary in the 0.0409–0.04607 W/m∙K range. This range is in the same order of magnitude of many traditional insulating systems, resulting as innovative thermal insulation solution for building applications.
In research performed on cellulose fiber insulation, encompassing its manufacturing, installation, and performance aspects, unveiled that, while the typical thermal conductivity value for cellulose averages approximately 0.040 W/m∙K, its properties and performance may exhibit slight variations based on the manufacturing process and installation method (Lopez Hurtado et al., 2016). Also, the source of cellulose can impact the material’s thermal insulating efficiency (Yeon et al., 2014).
Similar studies to assess the thermal, acoustic, and environmental behavior of rice husk panels, presents thermal conductivity values of 0.070 W/m∙K (Buratti et al., 2018) and 0.080 W/m∙K (Muthuraj et al., 2019), respectively.
In other study, increase on rice husk content in defined proportion, could produce decreases in thermal conductivity due to bulk density decrease (Antunes et al., 2019). In our case, with rice husk content evaluated, the k-values remains almost constant.
One of the main characteristics of the developed material, in addition to their thermal resistance, is that they have low energy in its production process because, for example, in the case of rice husks, it is agricultural byproducts which is grown in large proportions in our country. The rice is one of the crops with the highest production in Panamanian agriculture, with a sown area of more than 95,890 ha and 7,416,500 quintals in husk, according to data published by National Institute of Statistics and Census in the 2018–2019 agricultural year (INEC arroz 2015 a 2020, 2021). This data only indicate he crop area and they do not specify the total amount of residues. In other hand, the rice husk is considered an agro-industrial waste, that is normally discard in municipal landfills or incinerate, becoming a significant environmental concern in developing nations. With the expansion of agricultural production and intensification of farming system, more agro-waste residues are produced in all the world (Maraveas, 2020), and in Panama it is not the exception.
The Sustainable Development Goals, better known as SDGs, promoted by the United Nations and signed by more than 190 countries, includes specific objectives dedicated to sustainable cities and communities (11), responsible production and consumption (12), action for the climate (13), industry and innovation and infrastructure (9). The use of natural fibers instead of mineral fibers is related to these objectives, from the use of resources to the development of innovative products for different applications, per example, as thermal insultation materials on building’s envelope, especially in hot-humid region.
Global reports such as the Global Risks Report (Markovitz and Heading, 2023) and the National Energy Plan 2015–2050 (Secretaría Nacional de Energía, 2016), underscore the pressing environmental risks, notably climate change and biodiversity loss, which rank among the most probable and impactful global challenges. The development of efficient thermal insulating materials holds promise for mitigating the energy consumption of buildings, thereby potentially reducing greenhouse gas emissions (Palomo, 2017). One of the strategies to address the climate crisis and resource depletion is the transition to a circular economy (Foster, 2020). By transforming agricultural waste materials like rice husks into valuable resources, we promote reuse and diminish reliance on non-renewable resources (Carlos Javier et al., 2023; Soto et al., 2023).
In terms of energy consumption, the developed material could reduce the residential energy consumption, specially, considered the high cost of energy for air conditioning in Panama. The local climate, including humidity and temperature, affects how much cooling is required and, consequently, the cost of running an air conditioner. Also, the energy efficiency plays a role in energy costs. Well-insulated and well-designed spaces may require less cooling. In this case, the presented research aims to promote the use of materials considered as agro-industrial waste in our country, demonstrating their applicability in different areas, specially, in thermal insulation of walls, floor or roof, looking for energy loses reduction.
Compared to cellulose traditional insulation material, the addition of rice husk, could give rot-proof resistance (Chabannes et al., 2014; Antunes et al., 2019) and seem to have better resistance to molds in comparison with others natural materials (Antunes et al., 2019). Also, rice husk is bioadsorbent, have a hard surface, high silicon content, low bulk density, and is difficult to decompose with bacteria. In nature, the rice husk protects seeds from physical damage and attacks by pathogens, insects, and pests (Zou and Yang, 2019); this last characteristic is interesting in terms of growth of fungi, that is a desirable property in thermal insultation materials, particularly in humid region.
Based on the literature analysis, the developed material has environmental advantage compared to conventional ones (plastic foams and inorganic fibers):
• considering it is based on recycled and residues from agro-industrial; cellulose insulation continued to be predominantly made from recycled newspaper and other paper waste, making it a sustainable and environmentally friendly choice.
• cellulose insulation was often considered a cost-effective choice for insulation projects due to its affordability and energy-saving benefits, natural fibers and recycled materials present a promising solution to the plastic pollution, posed by building insultation materials (Zhao et al., 2022),
• considering this type of material has a better performance in terms of embodied energy (Pal et al., 2021); between 0.31 and 1.83 kg CO2 eq./kg for cellulose ground from wastepaper, very low compared to Expanded and Extruded Polystyrene (6.3 and 7.55 kg CO2 eq./kg, respectively) (Chandhran and Elavenil, 2023) and
• contribute to reducing the carbon footprint and global warming, considering the demand for energy and CO2 emissions; important goals of saving resources, carbon sequestration and environmental protection (Ricciardi et al., 2014; Zou et al., 2021).
4.3.1 Limitations of the study and future developments
Limitations in the development of this project can include those imposed by the COVID-19 (between 2021 and 2022) and available equipment, both in terms of the manufacturing process of panels and specimens, as well as carrying out tests to obtain reference values. However, it is considered that the study carried out constitutes a reference in Panama, to promote the use of materials considered as agro-industrial waste in our country, demonstrating their applicability in different areas.
The proposed new material has the potential for thermal insulation, so, for its future commercialization, the research and development process must continue, providing additional improvements. Per example, the flame retardancy of the developed material should be improved before being applied in buildings.
There are certain considerations that must be considered during manufacturing process of organic based materials: cellulose-based insulators absorb water in large quantities which could reduce its properties in certain environmental condition, especially in hot-humid region. These conditions allow the growth of fungi and reduce their useful life.
For future developments, the authors are evaluating the degradation of developed material, under ambient controlled conditions. Also, it is necessary to optimize the manufacturing process, considering the size of ground rice husk particles and drying process. Among the recommendations for improvement are obtaining rice husk particles with dimensions less than 0.6 mm. This could be achieved with specialized equipment, as well as sieves that allow particles with smaller dimensions to be obtained. With this, a more homogeneous material could be achieved, leading to a process with commercialization potential.
5 Conclusion
New boards made of recycled newspaper and rice husk were developed and tested, as an alternative for insulation material. The studied material was fabricated and evaluated from three different compositions and tests, including tensile strength tests, compressive strength tests y thermal conductivity tests. The tensile, compressive, and thermal performance were evaluated to propose their application as insulting boards for the building sector, as an alternative for the reuse of materials considered as value-added waste, such as recycled paper and rice husks.
The maximum stresses in average tension are in a range of 1.31–1.76 MPa. Despite the fact that the results are consistent with what has been reported in the scientific literature, further research could include other configurations, such as the addition of long fibers in only one direction to reinforce the behavior of the material in tension, since a material insulation that is placed as part of the envelope of a building does not have a great resistance to tension as an essential condition, it could be desirable in structural cases.
Regarding the compressive strength, the values obtained are between 20.19 and 21.23 MPa. In Muthuraj et al., 2019, for a material based on rice husk, a compressive strength of 15 MPa was obtained. In this sense, through the tests carried out, it was possible to verify that the resistance of the developed material presented values like those reported in the literature.
The experimental results showed a good thermal behavior of all the investigated compositions: k-values vary in the 0.0409–0.04607 W/m∙K range. The developed material has competitive thermal conductivity compared to many natural and recycled insulation materials and may be a viable option for applications requiring efficient thermal insulation. However, there is still opportunity of improvement to meet or exceed the efficiency of the leading industrial insulators on the market.
Within the general analysis of the manufacturing process of the composite material, it was possible to determine very favorable results when verifying its behavior in tension and compression, even so, considering that the rice husk can be crushed to obtain particles smaller than 0.6 mm. As a result of this research, a material with good thermal insulation capacity and good compression performance.
As a result of the findings presented in this article, it can be concluded that the material developed could potentially have utility across various engineering domains, including the production of lightweight components, construction panels, sustainable packaging, energy dissipation, thermal insulation, among other. Nevertheless, further research and testing are imperative to assess its viability and performance in each specific application.
In general, the panels investigated could be a valid solution to replace the classic insulation systems, although the investigation remains open for carrying out additional tests on the developed material, such as bending, flammability, and degradability tests, among others. Also, the proposed material is presented as a sustainable alternative, which can be used in the field of environmentally friendly buildings, which contribute to reducing the carbon footprint. This research aims to promote the use of materials considered as agro-industrial waste in our country, demonstrating their applicability in different areas. Also is considered as a contribution in advances in the use of natural fibers and recycled paper as thermal insulation materials, which could accordingly help future development of sustainable green insulations. The obtained results indicates that recycling materials and agro-waste materials have the potential to replace conventional constructions materials and hence achieve environmental and economic sustainability.
Data availability statement
The raw data supporting the conclusions of this article will be made available by the authors, without undue reservation.
Author contributions
NM-C: Conceptualization, Formal Analysis, Investigation, Methodology, Project administration, Supervision, Validation, Writing–original draft, Writing–review and editing. SG-S: Investigation, Methodology, Supervision, Validation, Writing–original draft, Writing–review and editing. AJ-R: Original idea, Methodology and funding.
Funding
The authors declare financial support was received for the research, authorship, and/or publication of this article. This research was funded by Sistema Nacional de Investigación (SNI) as part of a Panamanian Institution Secretaria Nacional de Ciencia, Tecnología e Innovación (SENACYT, https://www.senacyt.gob.pa/, accessed on 26 March 2023).
Acknowledgments
The authors would like to thank the Research Group—Iniciativa de Integración de Tecnologías para el Desarrollo de Soluciones Ingenieriles (I2TEDSI), Faculty of Mechanical Engineering (https://fim.utp.ac.pa/, accessed on 26 April 2023), Centro Regional de Azuero, Universidad Tecnológica de Panamá (https://ls.utp.ac.pa/), Laboratorio de Suelos y Ensayo de Materiales de Azuero (LSEMA) (https://ls.utp.ac.pa/laboratorio-de-suelos-y-ensayo-de-materiales-de-azuero-lsema, accessed on 26 April 2023), Laboratorio de Análisis Industriales y Ciencias Ambientales (LABAICA) (https://cei.utp.ac.pa/contactenos-laboratorio-de-analisis-industriales-y-ciencias-ambientales, accessed on 26 April 2023) within the Universidad Tecnológica de Panamá (https://utp.ac.pa/, accessed on 26 April 2023), for their collaboration.
Conflict of interest
The authors declare that the research was conducted in the absence of any commercial or financial relationships that could be construed as a potential conflict of interest.
Publisher’s note
All claims expressed in this article are solely those of the authors and do not necessarily represent those of their affiliated organizations, or those of the publisher, the editors and the reviewers. Any product that may be evaluated in this article, or claim that may be made by its manufacturer, is not guaranteed or endorsed by the publisher.
References
Ahmad, A., Adil, M., Khalil, A., and Rahman, M. (2021). Mechanical properties and durability of boardcrete blocks prepared from recycled cardboard. J. Build. Eng. 33, 101644. doi:10.1016/j.jobe.2020.101644
Aksogan, O., Resatoglu, R., and Binici, H. (2018). An environment friendly new insulation material involving waste newsprint papers reinforced by cane stalks. J. Build. Eng. 15, 33–40. doi:10.1016/j.jobe.2017.10.011
Ali, M., Alabdulkarem, A., Nuhait, A., Al-Salem, K., Iannace, G., and Almuzaiqer, R. (2022). Characteristics of agro waste fibers as new thermal insulation and sound absorbing materials: hybrid of date palm tree leaves and wheat straw fibers. J. Nat. Fibers 19, 6576–6594. doi:10.1080/15440478.2021.1929647
Amantino, G. M., Hasparyk, N. P., Tiecher, F., and Toledo Filho, R. D. (2022). Assessment of bio-aggregate concretes’ properties with rice residue. J. Build. Eng. 52, 104348. doi:10.1016/j.jobe.2022.104348
Antunes, A., Faria, P., Silva, V., and Brás, A. (2019). Rice husk-earth based composites: a novel bio-based panel for buildings refurbishment. Constr. Build. Mater 221, 99–108. doi:10.1016/j.conbuildmat.2019.06.074
Arévalo, R., and Peijs, T. (2016). Binderless all-cellulose fibreboard from microfibrillated lignocellulosic natural fibres. Compos Part A Appl. Sci. Manuf. 83, 38–46. doi:10.1016/j.compositesa.2015.11.027
ASTM C177-19 (2019). American society for testing and materials-ASTM. Available at: https://www.astm.org/c0177-19.html (Accessed July 31, 2023).
ASTM C209-20 (2020). American society for testing and materials-ASTM. Available at: https://www.astm.org/c0209-20.html (Accessed July 31, 2023).
ASTM C39/C39M-21 (2021). American society for testing and materials-ASTM. Available at: https://www.astm.org/c0039_c0039m-21.html (Accessed July 31, 2023).
Azzouzi, D., Rabahi, W., Seddiri, F., and Hemis, M. (2020). Experimental study of the fibres content effect on the heat insulation capacity of new vegetable composite plaster-pea pod fibres. Sustain. Mater. Technol. 23, e00144. doi:10.1016/j.susmat.2019.e00144
Bienvenido-Huertas, D., Sánchez-García, D., Pérez-Fargallo, A., and Rubio-Bellido, C. (2020). Optimization of energy saving with adaptive setpoint temperatures by calculating the prevailing mean outdoor air temperature. Build. Environ. 170, 106612. doi:10.1016/j.buildenv.2019.106612
Buratti, C., Belloni, E., Lascaro, E., Merli, F., and Ricciardi, P. (2018). Rice husk panels for building applications: thermal, acoustic and environmental characterization and comparison with other innovative recycled waste materials. Constr. Build. Mater 171, 338–349. doi:10.1016/j.conbuildmat.2018.03.089
Canto, A., Batista, M., Sanchez, J., Moreno, M., and James, A. (2018). Aislante térmico a base de materiales orgánicos Development of a thermal-insulation based on organic materials 1. Ciudad Panamá: Revista De Iniciación Científica.
Carlos Javier, R. H., Karin, R. N., and Juan Pablo, C. R. (2023). Valorization of wheat crop waste in araucanía, Chile: development of prototype of thermal insulation material for blowing technique and geographical analysis. Buildings 13, 1152. doi:10.3390/buildings13051152
Chabannes, M., Bénézet, J. C., Clerc, L., and Garcia-Diaz, E. (2014). Use of raw rice husk as natural aggregate in a lightweight insulating concrete: an innovative application. Constr. Build. Mater 70, 428–438. doi:10.1016/j.conbuildmat.2014.07.025
Chandhran, K. S. D., and Elavenil, S. (2023). A comprehensive state-of-the-art review of sustainable thermal insulation system used in external walls for reduction in energy consumption in buildings. Int. J. Green Energy 20, 895–913. doi:10.1080/15435075.2022.2120769
Dahal, R. K., Acharya, B., and Dutta, A. (2023). The interaction effect of the design parameters on the water absorption of the hemp-reinforced biocarbon-filled bio-epoxy composites. Int. J. Mol. Sci. 24, 6093. doi:10.3390/ijms24076093
Dahal, R. K. (2023). Hemp-based biocarbon-filled biopolymer composites: its preparation, characterization, and optimization. Avaliable At: https://hdl.handle.net/10214/27551.
Das, P., and Tiwari, P. (2018). Valorization of packaging plastic waste by slow pyrolysis. Resour. Conserv. Recycl 128, 69–77. doi:10.1016/j.resconrec.2017.09.025
Dixit, M. K., Fernández-Solís, J. L., Lavy, S., and Culp, C. H. (2010). Identification of parameters for embodied energy measurement: a literature review. Energy Build. 42, 1238–1247. doi:10.1016/j.enbuild.2010.02.016
Dixit, S., and Yadav, L. (2019). Synthesis of green thermally resistant composite: a review. Indian J. Chem. Technol. 26, 494–503. doi:10.56042/ijct.v26i6.19150
Faruk, O., Bledzki, A. K., Fink, H. P., and Sain, M. (2012). Biocomposites reinforced with natural fibers: 2000-2010. Prog. Polym. Sci. 37, 1552–1596. doi:10.1016/j.progpolymsci.2012.04.003
Foster, G. (2020). Circular economy strategies for adaptive reuse of cultural heritage buildings to reduce environmental impacts. Resour. Conserv. Recycl 152, 104507. doi:10.1016/j.resconrec.2019.104507
Garzón Bernal, L. D., and Cuba Córdoba, A. (2020). Paneles tipo sándwich a base de celulosa reciclada para fachadas. Bogota: Universidad La Gran Colombia.
Gonçalves, M. R. F., and Bergmann, C. P. (2007). Thermal insulators made with rice husk ashes: production and correlation between properties and microstructure. Constr. Build. Mater 21, 2059–2065. doi:10.1016/j.conbuildmat.2006.05.057
González-Serrud, S., Bernal, A., Chung, A., and Marín, N. (2022). Fabricación de láminas construidas a base de fibras naturales que absorben humedad relativa del entorno. Rev. Iniciación Científica 8, 7–16. doi:10.33412/rev-ric.v8.2.3664
Guna, V., Ilangovan, M., Rather, M. H., Giridharan, B. V., Prajwal, B., Vamshi Krishna, K., et al. (2020). Groundnut shell/rice husk agro-waste reinforced polypropylene hybrid biocomposites. J. Build. Eng. 27, 100991. doi:10.1016/j.jobe.2019.100991
Hu, S., Yan, D., and Qian, M. (2019). Using bottom-up model to analyze cooling energy consumption in China’s urban residential building. Energy Build. 202, 109352. doi:10.1016/j.enbuild.2019.109352
INEC arroz 2015 a 2020 (2021). Superficie sembrada y cosecha de Arroz, maíz y fríjol de Bejuco. Panamá: INEC.
Janowska-renkas, E., Król, A., Pochwała, S., Pałubski, D., Adamska, M., and Klementowski, I. (2022). The fire resistance and heat conductivity of natural construction material based on straw and numerical simulation of building energy demand. Energies (Basel) 15, 1155. doi:10.3390/en15031155
Karimah, A., Ridho, M. R., Munawar, S. S., Amin, Y., and Damayanti, R. (2021). A comprehensive review on natural fibers: technological and socio-economical aspects. Polym. (Basel) 13, 4280. doi:10.3390/polym13244280
Kumar, D., Alam, M., Zou, P. X. W., Sanjayan, J. G., and Memon, R. A. (2020). Comparative analysis of building insulation material properties and performance. Renew. Sustain. Energy Rev. 131, 110038. doi:10.1016/j.rser.2020.110038
Liu, L. F., Li, H. Q., Lazzaretto, A., Manente, G., Tong, C. Y., Liu, Q. B., et al. (2017). The development history and prospects of biomass-based insulation materials for buildings. Renew. Sustain. Energy Rev. 69, 912–932. doi:10.1016/j.rser.2016.11.140
Lopez Hurtado, P., Rouilly, A., Vandenbossche, V., and Raynaud, C. (2016). A review on the properties of cellulose fibre insulation. Build. Environ. 96, 170–177. doi:10.1016/j.buildenv.2015.09.031
Maraveas, C. (2020). Production of sustainable construction materials using agro-wastes. Materials 13, 262. doi:10.3390/ma13020262
Markovitz, G., and Heading, S. (2023). Global Risks Report 2023: we know what the risks are-here’s what experts say we can do about it. Global Risks Report.
Marques, B., Tadeu, A., António, J., Almeida, J., and de Brito, J. (2020). Mechanical, thermal and acoustic behaviour of polymer-based composite materials produced with rice husk and expanded cork by-products. Constr. Build. Mater 239, 117851. doi:10.1016/j.conbuildmat.2019.117851
Martínez, B., Bernat-Maso, E., and Gil, L. (2023). Applications and properties of hemp stalk-based insulating biomaterials for buildings: review. Materials 16, 3245. doi:10.3390/ma16083245
Moreno, P., Villamizar, N., Perez, J., Bayona, A., Roman, J., Moreno, N., et al. (2021). Fire-resistant cellulose boards from waste newspaper, boric acid salts, and protein binders. Clean. Technol. Environ. Policy 23, 1537–1546. doi:10.1007/s10098-021-02046-7
Muthukrishnan, S., Gupta, S., and Kua, H. W. (2019). Application of rice husk biochar and thermally treated low silica rice husk ash to improve physical properties of cement mortar. Theor. Appl. Fract. Mech. 104, 102376. doi:10.1016/j.tafmec.2019.102376
Muthuraj, R., Lacoste, C., Lacroix, P., and Bergeret, A. (2019). Sustainable thermal insulation biocomposites from rice husk, wheat husk, wood fibers and textile waste fibers: elaboration and performances evaluation. Ind. Crops Prod. 135, 238–245. doi:10.1016/j.indcrop.2019.04.053
Nagieb, Z. A., Nassar, M. A., and El-Meligy, M. G. (2011). Effect of addition of boric acid and borax on fire-retardant and mechanical properties of urea formaldehyde saw dust composites. Int. J. Carbohydr. Chem. 2011, 1–6. doi:10.1155/2011/146763
Nandy, S., Goswami, S., Marques, A., Gaspar, D., Grey, P., Cunha, I., et al. (2021). Cellulose: a contribution for the zero e-waste challenge. Adv. Mater Technol. 6, 994. doi:10.1002/admt.202000994
Ntimugura, F., Vinai, R., Harper, A., and Walker, P. (2020). Mechanical, thermal, hygroscopic and acoustic properties of bio-aggregates – lime and alkali - activated insulating composite materials: a review of current status and prospects for miscanthus as an innovative resource in the South West of England. Sustain. Mater. Technol. 26, e00211. doi:10.1016/j.susmat.2020.e00211
Pal, R. K., Goyal, P., and Sehgal, S. (2021). “Effect of cellulose fibre based insulation on thermal performance of buildings,” in Materials today: proceedings (Elsevier; Second International Conference on Aspects of Materials Science and Engineering (ICAMSE 2021)), 5778–5781. doi:10.1016/j.matpr.2021.02.749
Palomo, M. (2017). Aislantes térmicos. Criterios de selección por requisitos energéticos. Madrid, España: Universidad Tecnológica de Panamá.
Ricciardi, P., Belloni, E., and Cotana, F. (2014). Innovative panels with recycled materials: thermal and acoustic performance and Life Cycle Assessment. Appl. Energy 134, 150–162. doi:10.1016/j.apenergy.2014.07.112
Secretaría Nacional de Energía (2016). Plan energético nacional 2015-2050. Available at: https://www.senacyt.gob.pa/wp-content/uploads/2018/12/3.-Plan-Energetico-Nacional-2015-2050-1.pdf (Accessed September 15, 2023).
Soto, M., Rojas, C., and Cárdenas-Ramírez, J. P. (2023). Characterization of a thermal insulating material based on a wheat straw and recycled paper cellulose to Be applied in buildings by blowing method. Sustain. Switz. 15, 58. doi:10.3390/su15010058
Vidal, J., Ponce, D., Mija, A., Rymarczyk, M., and Castell, P. (2023). Sustainable composites from nature to construction: hemp and linseed reinforced biocomposites based on bio-based epoxy resins. Materials 16, 1283. doi:10.3390/ma16031283
Viegas, G. M., Walsh, C., María, Y., and Barros, V. (2014). Evaluación cuali-cuantitativa dE aislacionEs térmicas altErnativas para viviEndas. El caso dE la agricultura familiar 1 QuantitativE Evaluation of altErnativE thErmal insulation. thE casE of family-basEd farming 1. Santiago - Chile: Revista INVI.
Wang, S., Li, H., Zou, S., and Zhang, G. (2020). Experimental research on a feasible rice husk/geopolymer foam building insulation material. Energy Build. 226, 110358. doi:10.1016/j.enbuild.2020.110358
Yeon, J. O., Kim, K. W., Yang, K. S., Kim, J. M., and Kim, M. J. (2014). Physical properties of cellulose sound absorbers produced using recycled paper. Constr. Build. Mater 70, 494–500. doi:10.1016/j.conbuildmat.2014.07.088
Zabalza Bribián, I., Valero Capilla, A., and Aranda Usón, A. (2011). Life cycle assessment of building materials: comparative analysis of energy and environmental impacts and evaluation of the eco-efficiency improvement potential. Build. Environ. 46, 1133–1140. doi:10.1016/j.buildenv.2010.12.002
Zhang, C., Cui, C., Zhang, Y., Yuan, J., Luo, Y., and Gang, W. (2019). A review of renewable energy assessment methods in green building and green neighborhood rating systems. Energy Build. 195, 68–81. doi:10.1016/j.enbuild.2019.04.040
Zhao, J. R., Zheng, R., Tang, J., Sun, H. J., and Wang, J. (2022). A mini-review on building insulation materials from perspective of plastic pollution: current issues and natural fibres as a possible solution. J. Hazard Mater 438, 129449. doi:10.1016/j.jhazmat.2022.129449
Zou, S., Li, H., Liu, L., Wang, S., Zhang, X., and Zhang, G. (2021). Experimental study on fire resistance improvement of wheat straw composite insulation materials for buildings. J. Build. Eng. 43, 103172. doi:10.1016/j.jobe.2021.103172
Keywords: insulation material, thermal conductivity, cellulose, waste rice husk, recycled waste materials, agro-industrial waste, mechanical properties, thermal properties
Citation: Marín-Calvo N, González-Serrud S and James-Rivas A (2023) Thermal insulation material produced from recycled materials for building applications: cellulose and rice husk-based material. Front. Built Environ. 9:1271317. doi: 10.3389/fbuil.2023.1271317
Received: 02 August 2023; Accepted: 09 October 2023;
Published: 30 November 2023.
Edited by:
Paulo Santos, University of Coimbra, PortugalReviewed by:
Francesco Asdrubali, Roma Tre University, ItalyFrancesco Barreca, Mediterranea University of Reggio Calabria, Italy
Copyright © 2023 Marín-Calvo, González-Serrud and James-Rivas. This is an open-access article distributed under the terms of the Creative Commons Attribution License (CC BY). The use, distribution or reproduction in other forums is permitted, provided the original author(s) and the copyright owner(s) are credited and that the original publication in this journal is cited, in accordance with accepted academic practice. No use, distribution or reproduction is permitted which does not comply with these terms.
*Correspondence: Nacarí Marín-Calvo, bmFjYXJpLm1hcmluQHV0cC5hYy5wYQ==