- Department of Heat Engineering and Technology, Faculty of Civil Engineering, Riga Technical University, Riga, Latvia
Over the past decades there has been a strong evidence of a temperature rise across the world that has led to a growing concern of more extreme weather patterns and regular seasonal heat waves globally. As such, building occupants are at a continuously growing risk to overheating exposure inside the premises throughout the warm season of the year. This study investigates the utilization of cooling pad technology as a potential solution to enhance cooling efficiency. Compared to traditional cooling methods, the implementation of cooling pads leads to significant reductions in temperature and enhanced humidity control, while consuming relatively lower amounts of energy. The study contains a comprehensive analysis of the climatic conditions in Latvia, focusing on temperature and humidity variations throughout the year over the last decade in three cities–Riga, Daugavpils and Liepaja, that extensively represent the scope of climatic variations across Latvia, featuring coastal and continental climate patterns. This study aims to evaluate the effectiveness and suitability of cooling pad technology in Nordic climate, focusing on three Latvian cities. The novelty of the study lies in its analysis of cooling pad technology’s effectiveness in Nordic climatic conditions in addressing the increasing cooling demand. The paper examines the fundamental principles behind cooling pad technology, its impact on chiller performance, and its ability to optimize the cooling process. The utilization of cooling pad technology as an effective means to enhance cooling efficiency across the building stock to improve occupant comfort level and IEQ is highlighted. The results demonstrate 5.47% COP increase during average summer temperature conditions, and 17.78% COP increase in peak summer temperature conditions after implementation of cooling pads. This study contributes to the existing knowledge on cooling technologies, offering practical recommendations for the implementation of cooling pad systems use in Latvia and across the wider Nordic region, which is experiencing the gradual rise in summer temperature and humidity level.
1 Introduction
The global community is experiencing a warming at a magnitude that surpasses any observed fluctuations over the past tens of millions of years (Westerhold et al., 2020). Within the European region, the mean annual average temperature has risen by approximately 1.7°C–1.9°C compared to the pre-industrial era (Ji et al., 2023). Furthermore, the most recent decade spanning from 2013 to 2022 exhibits record-breaking statistics, with all 10 years ranking among the warmest years on record (EEA, 2023).
Over the past decades there has been a strong evidence of a temperature rise across the world that has led to a growing concern of more extreme weather patterns and regular seasonal heat waves globally. As such, building occupants are at a continuously growing risk to overheating exposure inside the premises throughout the summer season (CIBSE, 2017; Laouadi et al., 2020a; Qian et al., 2023). Overheating poses significant risks to building occupants, as prolonged exposure to high temperatures can lead to heat-related illnesses, including heat exhaustion and heat stroke, which can be life-threatening. The physiological impacts of overheating on occupants can result in reduced cognitive performance, decreased productivity, and an increased likelihood of accidents, particularly in work and educational environments (McLeod et al., 2022). Vulnerable groups, such as the elderly, children, and individuals with pre-existing health conditions, are particularly susceptible to the adverse effects of overheating, requiring careful consideration of strategies to mitigate these risks in building design and operation (Murtagh et al., 2019; Laouadi et al., 2020b; Ramallo-González et al., 2020; Attia et al., 2023).
While the Baltic region experiences a cool and temperate climate, the summers are characterized by ever increasing temperature averages and prolonged dry weather conditions with no or very little precipitation, which among other widespread economic challenges (in agricultural and industrial sectors) poses serious concerns for building occupants in terms of achieving comfortable indoor temperatures (Jung et al., 2022; Taylor et al., 2023). The Baltic region, known for its cool and temperate climate, has been experiencing a significant shift in weather patterns in recent years. The summers are becoming hotter, with ever-increasing temperatures, posing challenges for maintaining comfortable indoor conditions for building occupants. As the demand for cooling rises, energy efficiency becomes a crucial consideration, particularly in light of escalating electricity prices (Camacho et al., 2023; Sun et al., 2023; Yagi and Managi, 2023) and the need for sustainable practices (Prozuments et al., 2021).
The increase in summer temperatures in the Baltic region can be attributed to a variety of factors, including the global warming, which encompasses the broader concept of climate change. In line with the advent of higher seasonal temperatures, recent scientific investigations suggest that contemporary buildings face an increased susceptibility to overheating due to their architectural characteristics, including increased glazing surface area (Zou et al., 2023) and enhanced building airtightness levels (Laouadi et al., 2020a; Deshko et al., 2021). Glazed facades and windows compromise the thermal resistance of buildings during both cold and warm seasons. Additionally, window frames, as integral components of the building envelope, contribute to further heat loss (Baiburin et al., 2019), which, in conjunction with glazing, may account for up to 50% of the total building heat loss (Aburas et al., 2019; Tafakkori and Fattahi, 2021). Comparative analyses of building insulation materials conducted by Kumar et al. and Kapoor et al. (Kapoor et al., 2021; D; Kumar et al., 2020) have demonstrated that structures with higher thermal transmittance exhibit greater cost-effectiveness in regions with increased cooling demands, whereas those with lower thermal transmittance are more economically viable in regions with increased heating requirements. In addition to high temperatures, relative humidity (RH) also plays a vital role in occupants’ comfort. High humidity levels can exacerbate the discomfort experienced during hot weather, leading to feelings of stickiness and reduced thermal satisfaction (Williams et al., 2020). Therefore, an ideal cooling solution for the Baltic region should consider both temperature reduction and humidity control (Arundel et al., 1986; Psomas et al., 2021).
Achieving improved building energy efficiency requires a multidisciplinary approach encompassing various interrelated factors such as architectural features, building materials, the insulation quality of the building envelope, HVAC (heating, ventilation, and air conditioning) operation, and occupant behavior (Santos-Herrero et al., 2021). Mechanical ventilation systems serve as effective measures for diluting and removing indoor air contaminants, thereby mitigating health risks associated with inadequate ventilation (Godish, 1996; Seppänen, 1999; Engvall et al., 2005). While natural ventilation remains prevalent in many buildings worldwide in terms of fresh air supply and exchange, significant changes in outdoor environments and human behavior, driven by economic growth and global trends, have led to an increased implementation of mechanical ventilation systems in buildings (L. Zhao and Liu, 2020). Furthermore, to minimize heat loss and moisture accumulation in building structural elements, greater attention has been devoted to achieving building airtightness, which significantly contributes to the cost efficiency and feasibility of integrating mechanical ventilation systems into building infrastructure (Jiang and Chen, 2001; Salehi et al., 2017; Fernández-Agüera et al., 2019). HVAC systems account for approximately 40% of energy consumption in the building sector, and as indoor environmental quality requirements continue to become more stringent, this proportion is expected to rise in the future (Cao et al., 2016; Ana Picallo-Perez et al., 2021; Pan et al., 2021). The carbon optimization plan of the European Union sets forth an ambitious target of reducing carbon dioxide emissions from the building sector by 80% by 2050 compared to 1990 levels (Rootzen, 2012; European Commission, 2019). Reaching this target will require major changes in the design, operation and maintenance of buildings and the efficiency of their integrated heating, cooling and ventilation systems (Bardage, 2017; González-Lezcano, 2023). This leads to a growing interest in exploring alternative cooling technologies that can address these challenges while maintaining energy efficiency (Salata et al., 2022).
One promising technology that has gained attention in recent years is cooling pad technology, commonly used in evaporative cooling systems. Cooling pad technology, when implemented before the condenser unit of a chiller, offers several advantages. The use of cooling pads facilitates enhanced heat transfer and improved cooling efficiency by promoting evaporative cooling mechanisms (Laknizi et al., 2021a). This results in reduced energy consumption and enhanced operational performance of the chiller system, thereby contributing to overall energy efficiency and cost savings (Malli et al., 2011; Sohani et al., 2017a; Tejero-González and Franco-Salas, 2021a; Laknizi et al., 2021b). By leveraging the principles of evaporative cooling, these pads facilitate the cooling process by utilizing the heat exchange before the condenser coil in the chiller system that occurs when water evaporates (Abdullah et al., 2023; Pacak et al., 2023). This results in a reduction in both temperature and humidity, creating a more comfortable indoor environment (Sreeram et al., 2015; Sohani et al., 2017b). This is particularly important in the context of rising electricity prices, as cooling pads allows for cost savings while ensuring comfortable indoor conditions. Additionally, cooling pads have the potential to enhance sustainability by reducing carbon emissions associated with high-energy cooling systems (Laknizi et al., 2019; Tejero-González and Franco-Salas, 2021a; Kapilan et al., 2023; Ndukwu et al., 2023). Furthermore, cooling pad technology offer flexibility in their application, making them suitable for various climate conditions and settings such as residential, commercial and industrial building stock (Franco et al., 2014; Obando et al., 2020). They can be integrated into existing cooling systems or implemented as standalone units, providing adaptability in different contexts (Jain and Hindoliya, 2011; Malli et al., 2011).
Given the aforementioned challenges posed by increasing summer temperatures in the Baltic region, coupled with the need for energy efficiency and improved comfort, it becomes crucial to explore the potential of cooling pad technology to increase the efficiency of cooling systems and, thus, reduce the energy consumption attributed to the operation of these systems (Tejero-González and Franco-Salas, 2021a).
This study aims to investigate the effectiveness of cooling pads in enhancing cooling efficiency and occupants’ comfort in the context of the Baltic climate. By conducting experiments and evaluating the performance of cooling pads under controlled conditions, this study aims to provide valuable insights into the benefits and practical implications of adopting cooling pad technology as a viable solution for the region. In addition, this study examines the challenges posed by rising summer temperatures in the Baltic region by examining the potential of cooling pad technology. By considering the importance of temperature reduction, humidity control, and energy efficiency, this study aims to contribute to the existing knowledge on cooling technologies and provide valuable recommendations for the implementation of cooling pad systems in the Baltic climate.
2 Methodology
2.1 Compliance with local regulatory codes
As the summer outdoor temperatures are steadily rising, and there is a higher frequency of recorded heat wave events across Europe (Baldwin et al., 2019; Ramallo-González et al., 2020; Oliveira et al., 2022; Iacobucci, 2023), the building occupants are at an ever increasing risk to overheating exposure which is already emerging as an existing issue in many buildings where no cooling systems are present. With an advent of higher temperature patterns in the summer, the building cooling requirement will be continuously increasing with respect to cooling energy consumption per floor area.
Reg. No. 359 “Labour Protection Requirements in Workplaces” defines the permissible indoor air parameters within the work spaces based on the assigned category of the work environment (I-III), that employers have to comply with.
The category I work environment is not associated with physical efforts or requires very slight or slight physical efforts; Category II work environment is associated with medium or great physical efforts (for example, permanent lifting and movement of weights (up to 10 kg), welding, metal processing works); Category III work environment is associated with heavy work [for example, permanent lifting and movement of weights (greater than 10 kg)].
2.2 Evaporative cooling technology
In the context of the indoor cooling demand, the analysis of summer climate data provides an insight into the environmental conditions that buildings and occupants face. The rising temperatures and humidity levels highlight the urgency to address the increased cooling demand to maintain comfortable indoor environments while ensuring energy efficiency and sustainability (Tejero-González and Franco-Salas, 2021b; Rahman et al., 2023).
The thermal difference (ΔT) that can be obtained by an evaporative cooling pad system is the saturation efficiency (η):
where T1 is the dry temperature of the entering air (oC).
T2 is the dry temperature of the exiting air (oC).
Twb is the wet bulb temperature at entry (oC).
If the saturation efficiency of the pad η is known for, the thermal difference produced by the pad (ΔT) can be determined by a following equation:
The process of air cooling through the evaporation of water is known as adiabatic cooling and is considered an isenthalpic process. Consequently, the ability to cool a stream of air is determined by the difference between the air’s dry bulb temperature and the saturation temperature associated with its enthalpy. In practical terms, the saturation temperature is closely approximated by the wet bulb temperature. Therefore, the cooling potential of an air stream can be determined as the difference between the dry bulb temperature and the wet bulb temperature (Tdry–Twb).
In case of an indirect system the heat transfer from the wetted air mass to the room air supply can be determined by the following equation:
Eq. 1 and (2) provide a mathematical representation of the evaporative cooling process, allowing to determine the thermal difference (ΔT) achieved by an evaporative cooling pad system. It is important to note that the cooling potential of an air stream depends on factors such as the dry bulb temperature (Tdry) and the wet bulb temperature (Twb). The difference between these two temperatures reflects the extent of cooling that can be achieved in the system. By maintaining a lower wet bulb temperature or increasing the efficiency of the evaporative cooling pad, a greater thermal difference and improved cooling performance can be attained. In the case of an indirect system, where the heat transfer occurs between the wetted air mass and the room air supply, Eq. 3 provides a means to calculate the heat transfer (Q).
Understanding the equations and principles behind evaporative cooling systems enables to optimize system performance for the specific climate conditions. By adjusting factors like air flow rates, pad design, material, and wet bulb temperature control, cooling efficiency can be enhanced to effectively meet the growing indoor cooling demand (Jiansheng et al., 2022; Zhao et al., 2022). Moreover, this optimization not only prioritizes energy efficiency and sustainability but also ensures occupant comfort in the face of rising temperatures and humidity levels in the Latvian climate, as well as the regulatory codes to ensure occupant health and wellbeing.
3 Results
3.1 Summer climate data analysis
The analysis of summer climate data for three Latvian cities—Riga (representing a humid continental climate), Liepaja (representing a coastal climate), and Daugavpils (representing a continental climate). The three cities where selected as they broadly represent the scope of Latvian climatic pattern. The data was derived from the State meteorogical weather stations that are deployed to measure outdoor weather conditions. The analyzed climate data reveals an increase in average temperatures and humidity levels over the years, highlighting the increasing average summer temperatures and humidity observed from 2012 to 2022.
3.2 Average summer temperatures
The average summer temperatures in the studied cities demonstrate a clear upward trend, indicative of the warming climate. In Riga, which represents a humid continental climate, the average summer temperature ranged from 17.4°C in 2012°C to 19.5°C in 2022. This gradual increase over the analyzed period highlights the rising intensity of heat exposure faced by building occupants during the summer months. Similarly, in Liepaja, which represents a coastal climate, the average summer temperature ranged from 16.2°C to 18.4°C, showing a consistent rise. Daugavpils, with a continental climate, experienced average summer temperatures ranging from 16.4°C to 18.2°C, showcasing a similar dynamic (Figure 1).
The rising temperatures observed in these cities can be attributed to climate change, which is primarily driven by human-induced factors such as greenhouse gas emissions (Gi et al., 2018). As global temperatures rise, the impact on regional climate becomes evident, leading to hotter summers and therefore increased cooling demands (Salata et al., 2022).
3.3 Average summer humidity
The analysis of average summer humidity levels in the studied cities further emphasizes the changing climatic conditions. In Riga, the average summer humidity ranged from 8.6 g/m³ in 2012 to 9.9 g/m³ in 2022, signifying a considerable increase. Liepaja experienced average humidity levels ranging from 9.1 g/m³ to 10.17 g/m³, while Daugavpils witnessed averages ranging from 8.97 g/m³ to 9.83 g/m³ over the analyzed period (Figure 2.).
The rising humidity levels pose challenges in maintaining indoor comfort, as high humidity can lead to feelings of stickiness, reduced thermal satisfaction, and the potential for mold growth (Arundel et al., 1986; Hernberg et al., 2014; Attia et al., 2022). The combination of increasing temperatures and humidity levels underscores the heightened demand for indoor cooling solutions to ensure occupant comfort and wellbeing during the summer season. The need for effective cooling systems becomes more critical as the frequency and intensity of heatwaves continue to rise (Oliveira et al., 2022; Iacobucci, 2023).
3.4 Hourly-measured temperature analysis in the context of heat-wave occurrence
The climate data analysis for the three analyzed cities revealed that the frequency of heat-wave occurrence is increasing every summer, posing significant challenges to maintaining comfortable indoor environments, and necessitating effective cooling solutions. The analysis of hourly-measured temperature data to investigate the occurrence of heat waves over the past decade was conducted for the three cities. The increasing frequency of temperature recordings exceeding 25°C (deemed as heat-wave threshold) was examined in Daugavpils (continental climate) and Liepaja (coastal climate).
Analysis of the hourly-measured temperature data reveals ratehr similar pattern in both cities (Figure 3.). In Daugavpils, there has been a steady rise in the number of hourly temperature recordings exceeding 25°C since 2019. This indicates a significant increase in the occurrence of higher temperature episodes, potentially leading to prolonged heat waves. On the other hand, Liepaja exhibits fluctuating trends in the hourly count of temperature recordings above 25°C over the years. However, recent observations from 2021 to 2022 demonstrate the two highest count years on record (with almost 175 of recorded temperature hours above 25°C) and suggest a consistent upward trend, indicating a growing occurrence of elevated temperatures and the potential for more frequent heat waves in the future (Figure 3.).
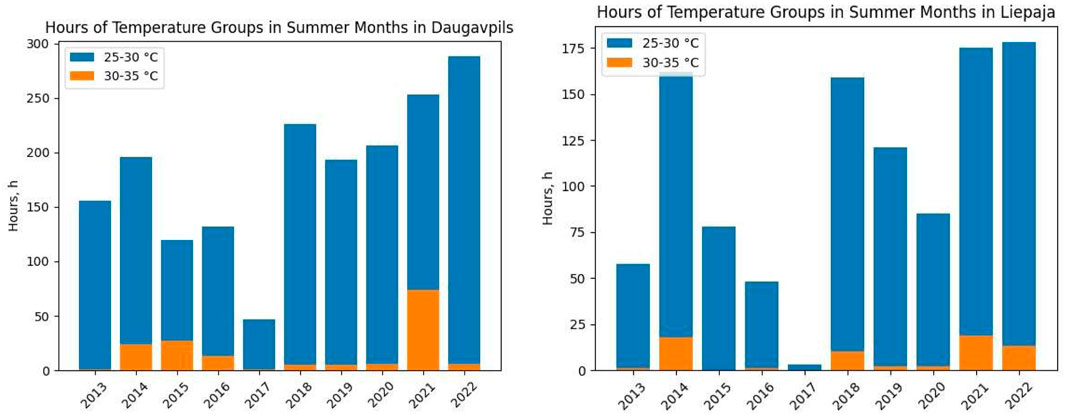
FIGURE 3. Hours of recorded temperature within 25–30 and 30–35°C range throughout July-August (2013–2022) in Daugavpils (left) and Liepaja (right).
The graphs below demonstrate the hourly recorded temperature variations throughout the summer months (July-August) in Riga in 2013 (left) and 2022 (right), (Figure 4.). The bar diagrams show that the occurrence of temperature recordings in 2022 exceeding 25°C have increased substantially compared to 2013. In the 2022, 280 recordings were detected of hourly temperature exceeding 25°C, while in 2013 this figure was much lower—147 h, suggesting more frequent heat wave occurrences and thus higher indoor overheating risk.
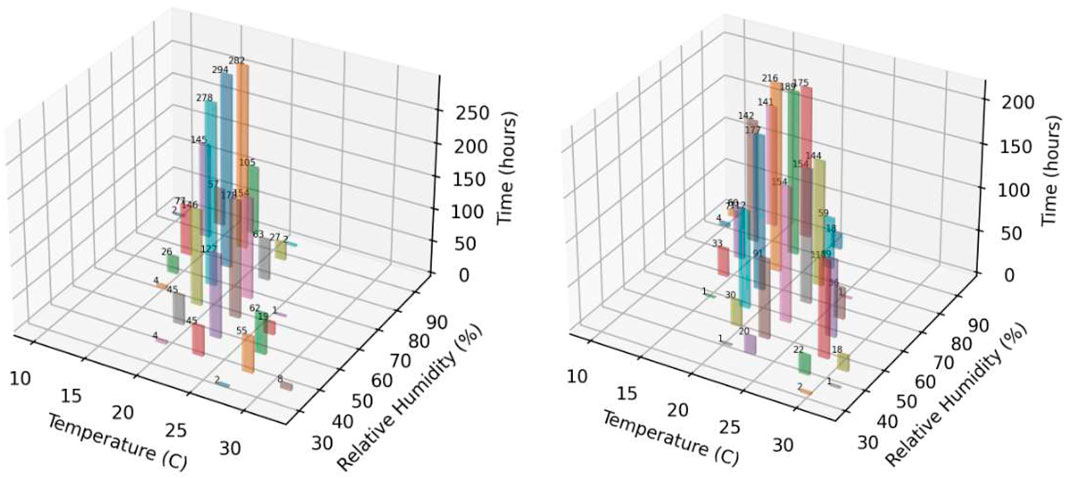
FIGURE 4. Comparative summary of hourly temperature recordings in Riga in 2013 (left) and 2022 (right).
The psychrometric chart below (Figure 5.) demonstrates the hourly summer temperature recordings over the last decade (2012–2022) which shows that while the average temperature and relative humidity values are concentrated within fairly wide range, the occurrence of recorded temperature over 25°C and RH over 65% is rather frequent, suggesting that building occupants are at risk of compromised indoor environmental quality if buildings are not equipped with proper cooling systems, or architectural passive design solutions to mitigate heat wave effects.
Given the increasing occurrence of high temperatures and the rising risk of indoor overheating, cooling pad technology emerges as a promising solution to mitigate these challenges. Cooling pad technology offers several advantages in the context of the increasingly warm climate, ensuring occupant comfort and wellbeing during the period of increased outdoor temperature and humidity levels.
The result analysis of hourly-measured temperature data highlights the increasing occurrence of high temperatures over the past decade, particularly exceeding 25°C. Daugavpils exhibits a consistent rise in temperature recordings above this threshold since 2019, while Liepaja and Riga shows fluctuating trends that have recently indicated a steady increase in summer temperature and humidity. In light of these changing climate conditions, cooling pad technology presents an effective solution to mitigate indoor overheating. By implementing cooling pads, an improved indoor comfort, reduced heat-related risks, and increased resilience to heat-waves can be attained.
The analyzed climate data show that throughout the summer months favourable conditions develop in the three examined cities in Latvia to employ evaporative cooling, as this technology is generally more efficient in conditions when the temperature is above 25°C, and RH levels between 40%–70%. RH levels below 70% are generally considered suitable for evaporative cooling–the lower the humidity, the better the cooling effect (Laknizi et al., 2019; Ndukwu et al., 2023; Rahman et al., 2023).
3.5 Chiller COP analysis
The chiller should run at its highest COP to produce the appropriate cooling capacity with a minimum amount of power consumption. Cooling loads, however, usually have a seasonal behaviour (especially in the Baltic climate region) and, in some buildings cooling loads, however, represent an important percentage of the overall energy demand (up to 44%) (Borodinecs et al., 2007; Gulotta et al., 2021; Krumins et al., 2022). Lower outdoor temperatures result in low cooling loads (little use of HVAC systems), so air-cooled chillers are more appropriate, since condensing refrigerant using cool outdoor air is quite efficient. In contrast, higher temperatures entail high cooling loads (strong use of HVAC systems) and, therefore, water-cooled chillers are more efficient because this type of chillers have better performance and higher nominal capacity (so a smaller number of chillers is required to cover high cooling loads (Chen Q. M. K. J. et al., 2022; Kumar et al., 2023).
Four reference air cooled chiller units were examined to determine the feasibility of adiabatic cooling pad use in air-cooled chillers in Latvian climate conditions. In order to determine the potential of cooling pad technology, the climate dataset of the last recorded summer (2022) in Riga was selected, which was based on average and peak summer temperature and RH data.
To understand the operation of each chiller with regard to a chiller load ratio or outdoor temperature, which are variables that affect the efficiency of cooling production systems, COP relation to ambient outdoor temperature graph was plotted. If the most recently recorded average summer temperatures are taken into consideration, a preliminary plot can be generated demonstrating the impact of cooling pads on air-cooled chiller performance. Taking the average air parameters in Riga over the summer of 2022 (t = 19°C and RH = 72%), as well as the average decrease in the inlet temperature by 2.5°C to after saturation to 90% (Figure 6), the COP value of each unit increases by a certain factor. Figure 6 demonstrates the COP increase after cooling pads application before the air-cooled chiller’s condenser unit for four selected chiller units.
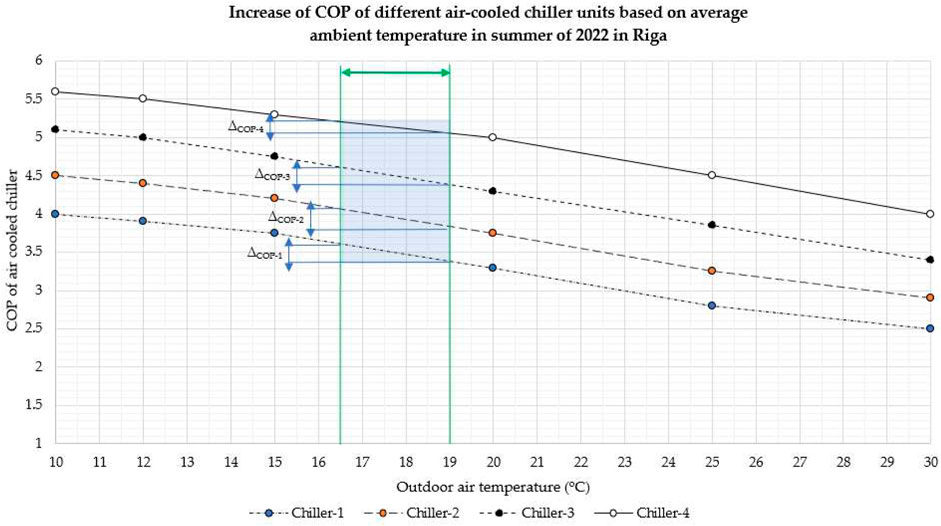
FIGURE 6. Increase in air-cooled chiller unit COP after application of evaporative cooling pad before the condenser during average summer temperature: case of Riga.
According to Figure 4 the peak temperature (32°C) in 2022 in Riga was recorded for 18 total hours with relative humidity around 55%. During adiabatic cooling process via cooling pads, the air mass will be saturated from RH 55% to RH 90%, resulting in temperature decrease to 25.9°C. Figure 7 shows the COP increase for the selected air cooled chiller units in an event of a peak temperature.
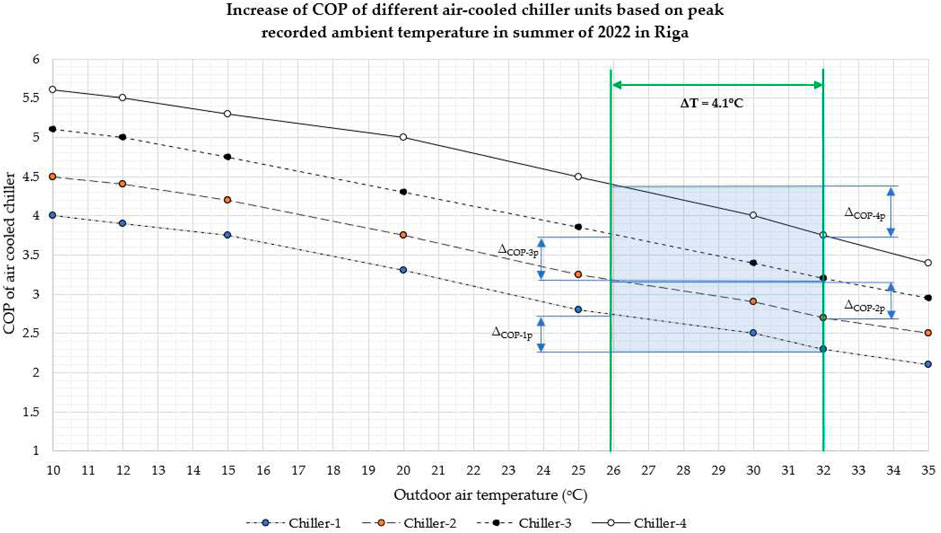
FIGURE 7. Increase in air-cooled chiller unit COP after application of evaporative cooling pad before the condenser during peak recorded temperature: case of Riga.
Table 1 compares the COP of the chillers during average and peak summer temperatures in Riga in 2022. ΔCOP% column shows the percentage increase in COP from due to the temperature decrease after cooling pad employment. It indicates the percentage improvement in efficiency for each chiller. During peak summer temperatures, the COP values are lower, as chillers are less efficient in hotter conditions. While the COP increases very slightly during the average temperature conditions (3.17%–5.47%), at peak conditions, the chiller COP increase is rather substantial (14.29%–17.78%), which implies that during prolonged hot weather conditions (T > 25°C) the use of adiabatic cooling pads may result in considerable energy savings attributed to chiller operation.

TABLE 1. Indoor parameters during warm period of the year in accordance with Republic of Latvia Cab. Reg. No. 359 “Labour Protection Requirements in Workplaces”.
4 Discussion
The increasing occurrence of heat waves, as evidenced by the analysis of hourly-measured temperature data, necessitates effective strategies to mitigate the impact of extreme heat on indoor environments. In the context of the increasingly warm climate in across the examined cities in Latvia, passive design solutions may offer promising approaches to mitigate the effects of heat waves and maintain comfortable indoor conditions. Therefore, the integration of architectural passive design solutions along with the highly efficient cooling pad technology, may result in a high combined effectiveness. Incorporating shading devices such as overhangs, louvers, or external blinds can effectively reduce solar heat gain during heat waves (Nikoofard et al., 2011). Designing buildings with proper cross-ventilation and operable windows allows for the influx of fresh air during cooler periods, promoting natural cooling and reducing reliance on mechanical cooling systems (Liu et al., 2023). Optimizing building orientation to minimize direct Sun exposure on the east and west facades can significantly reduce heat gain, while maximizing natural daylighting (De la Paz Pérez et al., 2023). Incorporating high thermal mass materials like concrete or stone into the building structure can help absorb and store excess heat during the day and release it during cooler periods, regulating indoor temperatures and reducing the need for active cooling (Kuczyński and Staszczuk, 2020; Lu et al., 2021). Implementing proper insulation in walls, roofs, and floors helps reduce heat transfer and minimize heat gain during heat waves. It provides a thermal barrier, preventing excessive heat penetration into the building (Deng et al., 2019; Shan et al., 2019). Opting for cool roofs that reflect sunlight rather than absorbing, can significantly reduce heat absorption and minimize the urban heat island effect. Cool roofs have higher solar reflectance and thermal emittance properties, as well as a capability to significantly raise roof’s albedo (by 60%–65%) (Lu et al., 2023), saving up to 8% on energy demand in cool, temperate climates (Bamdad, 2023). Incorporating green spaces, trees, and vegetation around buildings can help create shading, reduce ambient air temperatures, and improve outdoor microclimates. This natural landscaping also enhances air quality and provides a visually pleasing environment. Utilizing high-performance glazing with low solar heat gain coefficients (SHGC) and proper shading strategies can minimize heat transfer through windows while allowing for daylighting and views (Pereira et al., 2022; Araújo et al., 2023). Utilizing passive solar design principles, such as strategically locating windows and utilizing thermal mass, can optimize solar heat gain during cooler periods while minimizing overheating during heat waves (Borodinecs et al., 2012; Albayyaa et al., 2019). Incorporating reflective surfaces, such as light-colored exterior finishes or pavement, can help reduce heat absorption and lower surface temperatures, mitigating the urban heat island effect (El-Hattab et al., 2018; Aboelata and Sodoudi, 2019).
By incorporating these passive design solutions, buildings can effectively mitigate the effects of heat waves, reduce reliance on mechanical cooling, and provide comfortable and sustainable indoor environments. While, due to increasing summer temperature, mechanical cooling systems are the necessity to ensure healthy indoor climate, consideration of passive solutions may play a key role in reducing the energy use of the mechanical cooling equipment. The passive design solutions, in conjunction with cooling pad technology, can effectively mitigate the effects of heat waves and their impact on building occupants, ensuring improved indoor comfort, reduced energy consumption, and enhanced resilience to rising temperatures. The combined approach of passive design and cooling pad technology demonstrates the potential for sustainable and energy-efficient solutions to address the challenges of indoor overheating in the face of increasing heat wave occurrences (Yew et al., 2018; Vaseghi et al., 2020; Zavrl et al., 2022; Mayrhofer et al., 2023a).
The analysis of hourly-measured temperature data in Latvia highlights the increasing summer temperatures and the consequent higher demand for cooling, as previously discussed. In addressing these challenges, the findings from study (Kousar et al. (2021) offer valuable insights on optimizing the operation of evaporative cooling systems, such as cooling pads, in mitigating indoor overheating during heatwaves. The study highlights the importance of factors like air face velocity, pad thickness, and pad type (material and configuration), that can effectively increase the performance of the cooling pad. The influence of pad thickness on the cooling capacity and energy efficiency (COP) is complex, as larger thicknesses can enhance temperature drop and cooling capacity but also increase pressure drop and fan requirements (Malli et al., 2011; Tejero-González and Franco-Salas, 2021a).
The study conducted by Abdullah et al. (2023) provide valuable insights into the energy-saving potential and viability of different enhanced evaporative cooling systems compared to conventional cooling methods, facilitating the discussion on cooling pad efficacy in Nordic regions. These findings align with the previously discussed climate data in Latvia, indicating the increasing demand for cooling solutions. Enhanced evaporative cooling systems offer a promising alternative, addressing challenges associated with high humidity and offering energy efficiency improvements (Tejero-González and Franco-Salas, 2021a; Abdullah et al., 2023). The impact of various enhanced evaporative cooling technologies on total power consumption is highlighted, emphasizing the need for selecting appropriate technology for specific applications and different climate zones. These systems demonstrate the potential to enhance energy efficiency, aligning with sustainable development goals related to energy efficiency, climate action, and sustainable consumption and production. The potential of evaporative cooling systems as alternatives to conventional cooling methods is very high. The combination of effective evaporative cooling systems with separate dehumidification systems has demonstrated improved performance and the potential to achieve thermal comfort.
The increasing occurrence of high temperatures and heat wave events across the world require proactive measures to mitigate indoor overheating risks. By implementing cooling pad technology alongside architectural passive design solutions, optimal indoor comfort, reduced heat-related risks, and enhanced energy efficiency can be achieved. These findings highlight the importance of adopting innovative cooling strategies to ensure sustainable and resilient building environments in the face of changing climatic conditions. The integration of architectural passive design solutions, such as shading devices, natural ventilation, insulation, cool roofs, green spaces, and reflective surfaces, complements the efficacy of cooling pad technology in mitigating the effects of heat waves (Schnieders et al., 2015; Kohler et al., 2017; Harkouss et al., 2018). Passive design strategies, when integrated with cooling pad technology, create a symbiotic relationship that optimizes indoor comfort and energy efficiency. Natural ventilation enhances airflow and evaporative cooling, while shading devices and cool roofs mitigate solar heat gain, collectively reinforcing cooling pads’ temperature-reducing effects. As such, one review study suggested that building cooling demand could be reduced by up to 82% in combined passive/active cooling scenarios. Furthermore, ambitious combined (active + passive) building cooling strategies could reduce cooling demand by 68%–73%, resulting in a stagnation of the energy demand for space cooling between 2030 and 2050 (Mayrhofer et al., 2023b). This holistic approach ensures a balanced and sustainable solution to address indoor overheating and improve occupant wellbeing, which is in line with other literature investigating a combination of active and passive cooling strategies. (Schnieders et al., 2015; Chen Y. et al., 2022; Yang et al., 2022; González-Lezcano, 2023).
Further advancements, such as hybrid system development and simplification of design, are needed to enhance the viability and practicality of these alternative cooling systems. These efforts will contribute to providing environmentally friendly, economically viable, and energy-efficient alternatives to traditional vapor compression systems in response to the increasing demand for cooling in buildings. Also, the evaluation of air psychrometric conditions, including both dry bulb temperature and air humidity, is crucial for accurate system performance assessment. Neglecting air humidity can lead to incomplete analysis. Optimization of water flow rates should consider factors such as air velocity, psychrometric conditions, pad material, and salt deposition risks (Fouda and Melikyan, 2011; Sellami et al., 2019a; Sellami et al., 2019b; Laknizi et al., 2021a).
5 Conclusion
The current study comprehensively evaluated the applicability of cooling pad technology within Nordic climatic conditions through analysis and comparison of climate conditions in three Latvian cities. The analysis of climate data for three Latvian cities, Riga, Liepaja, and Daugavpils, demonstrated an increasing pattern in average summer temperatures and humidity levels over the past decade. These findings highlight the growing challenges posed by rising temperatures and the need to address the rising demand for mechanical cooling in buildings. In this context, cooling pad technology is viewed as a promising solution to effectively mitigate indoor overheating and maintain comfortable indoor environments during periods of high outdoor temperatures and humidity.
The hourly-measured temperature data analysis demonstrates a significant occurrence of temperature recordings exceeding 25°C, indicating the frequent occurrence of heat wave events. This underscores the increased risk of compromised indoor environmental quality for building occupants in the absence of proper cooling systems. The psychrometric chart analysis for Riga revealed the prevalence of temperature over 25°C and relative humidity over 65%, facilitating the importance of implementing adequate cooling systems or passive design solutions to mitigate the effects of heat waves. The findings of this study indicate that integration of cooling pad before the condenser unit will result in an increase of chiller COP (5.47%) during average summer temperature conditions, and a substantial increase in COP (17.78%) during peak summer temperature conditions (Table 2).
This study underscores the significance of innovative and efficient cooling strategies in meeting the challenges of increasing global cooling demand. In addition to the findings, it is imperative to consider policy frameworks that incentivize the adoption of these solutions and address potential barriers such as upfront costs, regulatory alignment, and technology scalability to facilitate their widespread implementation, thereby fostering resilient and sustainable building environments. Regulatory building codes are an effective policy measure for reducing energy consumption in buildings. Many countries have been adopting more stringent building energy codes over time, which have resulted in more efficient building stock in those countries.
However, further research and development are required to optimize these systems for different climates. Incorporating long-term field studies in representative locations across Nordic countries could provide valuable insights into the technology’s real-world performance and adaptability. Additionally, assessing the economic feasibility and scalability of implementing cooling pad systems on a larger scale, while considering regional regulations and incentives, would contribute to a holistic understanding of their suitability and potential benefits in Nordic climates.
As the global climate change results in more intense and frequent heat waves across the world, the imperative to employ efficient cooling solutions in buildings becomes very topical. With a collective commitment to innovative and efficient cooling solutions, stakeholders can shape a future where resilient, energy-efficient buildings redefine humans’ relationship with the environment and improve occupant wellbeing.
Data availability statement
The original contributions presented in the study are included in the article/supplementary material, further inquiries can be directed to the corresponding authors.
Author contributions
AP: Writing–review and editing, Data curation, Formal Analysis, Methodology, Writing–original draft. AB: Conceptualization, Data curation, Funding acquisition, Project administration, Resources, Supervision, Validation, Writing–review and editing. KL: Investigation, Methodology, Resources, Writing–review and editing. AP: Resources, Software, Visualization, Writing–review and editing.
Funding
The author(s) declare financial support was received for the research, authorship, and/or publication of this article. This research was supported by ERDF project “Development of a new prototype of adiabatic cooling panels to ensure the sustainability and energy efficiency of cooling equipment,” Nr. 1.1.1.1/19/A/002.
Conflict of interest
The authors declare that the research was conducted in the absence of any commercial or financial relationships that could be construed as a potential conflict of interest.
Publisher’s note
All claims expressed in this article are solely those of the authors and do not necessarily represent those of their affiliated organizations, or those of the publisher, the editors and the reviewers. Any product that may be evaluated in this article, or claim that may be made by its manufacturer, is not guaranteed or endorsed by the publisher.
References
Abdullah, S., Zubir, M. N. B. M., Muhamad, M. R. Bin, Newaz, K. M. S., Öztop, H. F., Alam, M. S., et al. (2023). Technological development of evaporative cooling systems and its integration with air dehumidification processes: A review. Energy Build. 283, 112805. doi:10.1016/j.enbuild.2023.112805
Aboelata, A., and Sodoudi, S. (2019). Evaluating urban vegetation scenarios to mitigate urban heat island and reduce buildings’ energy in dense built-up areas in Cairo. Build. Environ. 166, 106407. doi:10.1016/j.buildenv.2019.106407
Aburas, M., Soebarto, V., Williamson, T., Liang, R., Ebendorff-Heidepriem, H., and Wu, Y. (2019). Thermochromic smart window technologies for building application: A review. Appl. Energy 255, 113522. doi:10.1016/j.apenergy.2019.113522
Albayyaa, H., Hagare, D., and Saha, S. (2019). Energy conservation in residential buildings by incorporating Passive Solar and Energy Efficiency Design Strategies and higher thermal mass. Energy Build. 182, 205–213. doi:10.1016/j.enbuild.2018.09.036
Ana Picallo-Perez, J. M. S., Odriozola-Maritorena, M., Hidalgo, J. M., Hidalgo-Betanzos, J., and Gomez-Arriaran, I. (2021). Ventilation of buildings with heat recovery systems: thorough energy and exergy analysis for indoor thermal wellness. J. Build. Eng. 39, 102255. doi:10.1016/j.jobe.2021.102255
Araújo, G. R., Teixeira, H., Gomes, M. G., and Rodrigues, A. M. (2023). Multi-objective optimization of thermochromic glazing properties to enhance building energy performance. Sol. Energy 249, 446–456. doi:10.1016/j.solener.2022.11.043
Arundel, A. V., Sterling, E. M., Biggin, J. H., and Sterling, T. D. (1986). Indirect health effects of relative humidity in indoor environments. Environ. Health Perspect. 65, 351. doi:10.2307/3430203
Attia, S., Benzidane, C., Rahif, R., Amaripadath, D., Hamdy, M., Holzer, P., et al. (2023). Overheating calculation methods, criteria, and indicators in European regulation for residential buildings. Energy Build. 292, 113170. doi:10.1016/J.ENBUILD.2023.113170
Attia, S., Kurnitski, J., Kosiński, P., Borodiņecs, A., Deme Belafi, Z., István, K., et al. (2022). Overview and future challenges of nearly zero-energy building (nZEB) design in Eastern Europe. Energy Build. 267, 112165. doi:10.1016/j.enbuild.2022.112165
Baiburin, A. K., Rybakov, M. M., and Vatin, N. I. (2019). Heat loss through the window frames of buildings. Mag. Civ. Eng. 85 (1), 3–14. doi:10.18720/MCE.85.1
Baldwin, J. W., Dessy, J. B., Vecchi, G. A., and Oppenheimer, M. (2019). Temporally compound heat wave events and global warming: an emerging hazard. Earth’s Future 7, 411–427. doi:10.1029/2018EF000989
Bamdad, K. (2023). Cool roofs: A climate change mitigation and adaptation strategy for residential buildings. Build. Environ. 236, 110271. doi:10.1016/j.buildenv.2023.110271
Bardage, S. L. (2017). Performance of buildings. Perform. Bio-Based Build. Mater., 335–383. doi:10.1016/B978-0-08-100982-6.00006-9
Borodinecs, A., Dzelzitis, E., and Kreslins, A. (2007). General requirements for the energy performance of buildings in Latvia. Water Technol.
Borodinecs, A., Zemitis, J., and Prozuments, a. (2012). Passive use of solar energy in double skin facades for reduction of cooling loads. Riga, Latvia: Riga Technical University.
Camacho, M., Caro, A., and Peña, D. (2023). What drives industrial energy prices? Econ. Model. 120, 106158. doi:10.1016/J.ECONMOD.2022.106158
Cao, X., Dai, X., and Liu, J. (2016). Building energy-consumption status worldwide and the state-of-the-art technologies for zero-energy buildings during the past decade. Energy Build. 128, 198–213. doi:10.1016/j.enbuild.2016.06.089
Chen, Q. M. K. J., Burhan, M., Shahzad, M. W., Ybyraiymkul, D., Oh, S., Cui, X., et al. (2022a). Long-term performance of a hybrid indirect evaporative cooling-mechanical vapor compression cycle: A case study in Saudi arabia. Front. Built Environ. 8. doi:10.3389/fbuil.2022.1032961
Chen, Y., Xu, J., Wang, J., and Lund, P. D. (2022b). Optimization of a weather-based energy system for high cooling and low heating conditions using different types of water-cooled chiller. Energy 252, 124094. doi:10.1016/j.energy.2022.124094
CIBSE (2017). “Design methodology for the assessment of overheating risk in homes,” in Technical memoranda (London, UK: CIBSE).
De la Paz Pérez, G. A., Couret, D. G., Rodríguez-Algeciras, J. A., and De la Paz Vento, G. (2023). Influence of the urban context on solar protection of the vertical envelope and the cooling energy demand of buildings in Cuba. J. Build. Eng. 76, 107224. doi:10.1016/j.jobe.2023.107224
Deng, J., Yao, R., Yu, W., Zhang, Q., and Li, B. (2019). Effectiveness of the thermal mass of external walls on residential buildings for part-time part-space heating and cooling using the state-space method. Energy Build. 190, 155–171. doi:10.1016/j.enbuild.2019.02.029
Deshko, V., Bilous, I., Sukhodub, I., and Yatsenko, O. (2021). Evaluation of energy use for heating in residential building under the influence of air exchange modes. J. Build. Eng. 42, 103020. doi:10.1016/j.jobe.2021.103020
EEA (2023). Indicator assessment. Global and European temperatures. Copenhagen, Denmark: European Environment Agency EEA.
El-Hattab, M., Amany, S. M., and Lamia, G. E. (2018). Monitoring and assessment of urban heat islands over the Southern region of Cairo Governorate, Egypt. Egypt. J. Remote Sens. Space Sci. 21 (3), 311–323. doi:10.1016/j.ejrs.2017.08.008
Engvall, K., Wickman, P., and Norbäck, D. (2005). Sick building syndrome and perceived indoor environment in relation to energy saving by reduced ventilation flow during heating season: A 1 year intervention study in dwellings. Indoor Air 15, 120–126. doi:10.1111/j.1600-0668.2004.00325.x
European Commission (2019). Going climate-neutral by 2050: A strategic long-term vision for a prosperous, modern, competitive and climate-neutral eu economy. Brussels, Belgium: European Commission.
Fernández-Agüera, J., Domínguez-Amarillo, S., Alonso, C., and Martín-Consuegra, F. (2019). Thermal comfort and indoor air quality in low-income housing in Spain: the influence of airtightness and occupant behaviour. Energy Build. 199, 102–114. doi:10.1016/j.enbuild.2019.06.052
Fouda, A., and Melikyan, Z. (2011). A simplified model for analysis of heat and mass transfer in a direct evaporative cooler. Appl. Therm. Eng. 31, 932–936. doi:10.1016/j.applthermaleng.2010.11.016
Franco, A., Valera, D. L., and Peña, A. (2014). Energy efficiency in greenhouse evaporative cooling techniques: cooling boxes versus cellulose pads. Energies 7, 1427–1447. doi:10.3390/en7031427
Gi, K., Sano, F., Hayashi, A., Tomoda, T., and Akimoto, K. (2018). A global analysis of residential heating and cooling service demand and cost-effective energy consumption under different climate change scenarios up to 2050. Mitig. Adapt. Strategies Glob. Change 23 (1), 51–79. doi:10.1007/s11027-016-9728-6
Godish, T., and Spengler, J. D. (1996). Relationships between ventilation and indoor air quality: A review. Indoor Air 6, 135–145. doi:10.1111/j.1600-0668.1996.00010.x
González-Lezcano, R. A. (2023). Editorial: design of efficient and healthy buildings. Front. Built Environ. 9. doi:10.3389/fbuil.2023.1210956
Gulotta, T. M., Cellura, M., Guarino, F., and Longo, S. (2021). A bottom-up harmonized energy-environmental models for europe (boheeme): A case study on the thermal insulation of the EU-28 building stock. Energy Build. 231, 110584. doi:10.1016/J.ENBUILD.2020.110584
Harkouss, F., Fardoun, F., and Biwole, P. H. (2018). Passive design optimization of low energy buildings in different climates. Energy 165, 591–613. doi:10.1016/j.energy.2018.09.019
Hernberg, S., Sripaiboonkij, P., Quansah, R., Jaakkola, J. J. K., and Jaakkola, M. S. (2014). Indoor molds and lung function in healthy adults. Respir. Med. 108, 677–684. doi:10.1016/j.rmed.2014.03.004
Iacobucci, G. (2023). Europe’s record heatwave in 2022 caused many health harms, report finds. BMJ Clin. Res. Ed.) 381, 905. doi:10.1136/bmj.p905
Jain, J. K., and Hindoliya, D. A. (2011). Experimental performance of new evaporative cooling pad materials. Sustain. Cities Soc. 1, 252–256. doi:10.1016/j.scs.2011.07.005
Ji, Y., Fu, J., Lu, Y., and Liu, B. (2023). Three-dimensional-based global drought projection under global warming tendency. Atmos. Res. 291, 106812. doi:10.1016/J.ATMOSRES.2023.106812
Jiang, Y., and Chen, Q. (2001). Study of natural ventilation in buildings by large eddy simulation. J. Wind Eng. Industrial Aerodynamics 89, 1155–1178. doi:10.1016/S0167-6105(01)00106-4
Jiansheng, W., Xin, W., and Xueling, L. (2022). Numerical investigation on flow behavior and heat transfer feature of flexible wings located at the bottom of a two-dimensional channel. Appl. Therm. Eng. 206, 118112. doi:10.1016/j.applthermaleng.2022.118112
Jung, C., Mahmoud, N. S. A., and Alqassimi, N. (2022). Identifying the relationship between VOCs emission and temperature/humidity changes in new apartments in the hot desert climate. Front. Built Environ. 8. doi:10.3389/fbuil.2022.1018395
Kousar, R., Ali, M., Amjad, M. K., Sheikh, N. A., and Ahmad, W. (2021). Performance characterization and optimization of counter-flow dew point indirect evaporative cooler through response surface methodology. Proc. Institution Mech. Eng. Part A J. Power Energy 235 (7), 1789–1805. doi:10.1177/09576509211007976
Kumar, D., Alam, M., Zou, P. X. W., Sanjayan, J. G., and Memon, R. A. (2020). Comparative analysis of building insulation material properties and performance. Renew. Sustain. Energy Rev. 131, 110038. doi:10.1016/j.rser.2020.110038
Kapilan, N., Isloor, A. M., and Karinka, S. (2023). A comprehensive review on evaporative cooling systems. Results Eng. 18, 101059. doi:10.1016/J.RINENG.2023.101059
Kapoor, N. R., Kumar, A., Meena, C. S., Kumar, A., Alam, T., Balam, N. B., et al. (2021). A systematic review on indoor environmental quality in naturally ventilated school classrooms: A way forward. Adv. Civ. Eng. 2021, 1–19. doi:10.1155/2021/8851685
Kohler, K., Shulka, Y., and Rwal, R. (2017). Calculating the effect of external shading on the solar heat gain coefficient of windows. https://escholarship.org/content/qt2769w7wr/qt2769w7wr_noSplash_bbc9631d2a0e9353e3dc632b7ae9485b.pdf?t=owiy84.
Krumins, A., Lebedeva, K., Tamane, A., and Millers, R. (2022). Possibilities of balancing buildings energy demand for increasing energy efficiency in Latvia. Environ. Clim. Technol. 26 (1), 98–114. doi:10.2478/rtuect-2022-0009
Kuczyński, T., and Staszczuk, A. (2020). Experimental study of the influence of thermal mass on thermal comfort and cooling energy demand in residential buildings. Energy 195, 116984. doi:10.1016/j.energy.2020.116984
Kumar, D. M., Catrini, P., Piacentino, A., and Cirrincione, M. (2023). Advanced modeling and energy-saving-oriented assessment of control strategies for air-cooled chillers in space cooling applications. Energy Convers. Manag. 291, 117258. doi:10.1016/j.enconman.2023.117258
Laknizi, A., Ben Abdellah, A., Faqir, M., Essadiqi, E., and Dhimdi, S. (2021a). Performance characterization of a direct evaporative cooling pad based on pottery material. Int. J. Sustain. Eng. 14, 46–56. doi:10.1080/19397038.2019.1677800
Laknizi, A., Ben Abdellah, A., Mahdaoui, M., and Anoune, K. (2021b). Application of Taguchi and ANOVA methods in the optimisation of a direct evaporative cooling pad. Int. J. Sustain. Eng. 14 (5), 1218–1228. doi:10.1080/19397038.2020.1866707
Laknizi, A., Mahdaoui, M., Ben Abdellah, A., Anoune, K., Bakhouya, M., and Ezbakhe, H. (2019). Performance analysis and optimal parameters of a direct evaporative pad cooling system under the climate conditions of Morocco. Case Stud. Therm. Eng. 13, 100362. doi:10.1016/J.CSITE.2018.11.013
Laouadi, A., Bartko, M., and Lacasse, M. A. (2020a). A new methodology of evaluation of overheating in buildings. Energy Build. 226, 110360. doi:10.1016/j.enbuild.2020.110360
Laouadi, A., Bartko, M., and Lacasse, M. A. (2020b). A new methodology of evaluation of overheating in buildings. Energy Build. 226, 110360. doi:10.1016/J.ENBUILD.2020.110360
Liu, Y., Gao, Y., Zhuang, C., Shi, D., Xu, Y., Guan, J., et al. (2023). Optimization of top-floor rooms coupling cool roofs, natural ventilation and solar shading for residential buildings in hot-summer and warm-winter zones. J. Build. Eng. 66, 105933. doi:10.1016/j.jobe.2023.105933
Lu, F., Yu, Z., Zou, Y., and Yang, X. (2021). Cooling system energy flexibility of a nearly zero-energy office building using building thermal mass: potential evaluation and parametric analysis. Energy Build. 236, 110763. doi:10.1016/j.enbuild.2021.110763
Lu, M., Zeng, L., Li, Q., Hang, J., Hua, J., Wang, X., et al. (2023). Quantifying cooling benefits of cool roofs and walls applied in building clusters by scaled outdoor experiments. Sustain. Cities Soc. 97, 104741. doi:10.1016/j.scs.2023.104741
Malli, A., Seyf, H. R., Layeghi, M., Sharifian, S., and Behravesh, H. (2011). Investigating the performance of cellulosic evaporative cooling pads. Energy Convers. Manag. 52, 2598–2603. doi:10.1016/j.enconman.2010.12.015
Mayrhofer, L., Müller, A., Bügelmayer-Blaschek, M., Malla, A., and Kranzl, L. (2023a). Modelling the effect of passive cooling measures on future energy needs for the Austrian building stock. Energy Build. 296, 113333. doi:10.1016/j.enbuild.2023.113333
Mayrhofer, L., Müller, A., Bügelmayer-Blaschek, M., Malla, A., and Kranzl, L. (2023b). Modelling the effect of passive cooling measures on future energy needs for the Austrian building stock. Energy Build. 296, 113333. doi:10.1016/j.enbuild.2023.113333
McLeod, R. S., Mathew, M., Salman, D., and Thomas, C. L. P. (2022). An investigation of indoor air quality in a recently refurbished educational building. Front. Built Environ. 7. doi:10.3389/fbuil.2021.769761
Murtagh, N., Gatersleben, B., and Fife-Schaw, C. (2019). Occupants’ motivation to protect residential building stock from climate-related overheating: A study in southern england. J. Clean. Prod. 226, 186–194. doi:10.1016/J.JCLEPRO.2019.04.080
Ndukwu, M. C., Ibeh, M. I., Akpan, G. E., Ugwu, E., Akuwueke, L., Oriaku, L., et al. (2023). Analysis of the influence of outdoor surface heat flux on the inlet water and the exhaust air temperature of the wetting pad of a direct evaporative cooling system. Appl. Therm. Eng. 226, 120292. doi:10.1016/J.APPLTHERMALENG.2023.120292
Nikoofard, S., Ugursal, V. I., and Beausoleil-Morrison, I. (2011). Effect of external shading on household energy requirement for heating and cooling in Canada. Energy Build. 43, 1627–1635. doi:10.1016/j.enbuild.2011.03.003
Obando, F. A., Montoya, A. P., Osorio, J. A., Damasceno, F. A., and Norton, T. (2020). Evaporative pad cooling model validation in a closed dairy cattle building. Biosyst. Eng. 198, 147–162. doi:10.1016/j.biosystemseng.2020.08.005
Oliveira, A., Lopes, A., and Correia, E. (2022). Annual summaries dataset of heatwaves in europe, as defined by the excess heat factor. Data Brief 44, 108511. doi:10.1016/j.dib.2022.108511
Pacak, A., Jurga, A., Kaźmierczak, B., and Pandelidis, D. (2023). Experimental verification of the effect of air pre-cooling in dew point evaporative cooler on the performance of a solid desiccant dehumidifier. Int. Commun. Heat Mass Transf. 142, 106651. doi:10.1016/j.icheatmasstransfer.2023.106651
Pan, Y., Du, C., Fu, Z., and Fu, M. (2021). Re-thinking of engineering operation solutions to HVAC systems under the emerging COVID-19 pandemic. J. Build. Eng. 43, 102889. doi:10.1016/j.jobe.2021.102889
Pereira, J., Teixeira, H., Gomes, M. da G., and Moret Rodrigues, A. (2022). Performance of solar control films on building glazing: A literature review. Appl. Sci. 12 (12), 5923. doi:10.3390/app12125923
Prozuments, A., Staveckis, A., Zemitis, J., and Bajare, D. (2021). Evaluation of heating and cooling loads for a well-insulated single-family house under variable climate pattern. Environ. Clim. Technol. 25 (1), 750–763. doi:10.2478/rtuect-2021-0056
Psomas, T., Teli, D., Langer, S., Wahlgren, P., and Wargocki, P. (2021). Indoor humidity of dwellings and association with building characteristics, behaviors and health in a northern climate. Build. Environ. 198, 107885. doi:10.1016/j.buildenv.2021.107885
Qian, B., Yu, T., Zhang, C., Heiselberg, P. K., Lei, B., and Yang, L. (2023). Suitability of heat wave event definitions for assessing indoor overheating in current and future climate: A case study in China. Build. Environ. 241, 110487. doi:10.1016/J.BUILDENV.2023.110487
Rahman, M. S., MacPherson, S., and Lefsrud, M. (2023). Experimental investigation of a novel evaporative cooling pad made of cement-free porous concrete. Build. Environ. 228, 109867. doi:10.1016/j.buildenv.2022.109867
Ramallo-González, A. P., Eames, M. E., Natarajan, S., Fosas-de-Pando, D., and Coley, D. A. (2020). An analytical heat wave definition based on the impact on buildings and occupants. Energy Build. 216, 109923. doi:10.1016/J.ENBUILD.2020.109923
Rootzen, J. (2012). Reducing carbon dioxide emissions from the EU power and industry sectors. Gothenburg, Sweden: Chalmers University of Technology.
Salata, F., Falasca, S., Ciancio, V., Curci, G., Grignaffini, S., and de Wilde, P. (2022). Estimating building cooling energy demand through the cooling degree hours in a changing climate: A modeling study. Sustain. Cities Soc. 76, 103518. doi:10.1016/j.scs.2021.103518
Salehi, A., Torres, I., and Ramos, A. (2017). Experimental analysis of building airtightness in traditional residential Portuguese buildings. Energy Build. 151, 198–205. doi:10.1016/j.enbuild.2017.06.037
Santos-Herrero, J. M., Lopez-Guede, J. M., and Flores-Abascal, I. (2021). Modeling, simulation and control tools for nZEB: A state-of-the-art review. Renew. Sustain. Energy Rev. 142, 110851. doi:10.1016/j.rser.2021.110851
Schnieders, J., Feist, W., and Rongen, L. (2015). Passive Houses for different climate zones. Energy Build. 105, 71–87. doi:10.1016/j.enbuild.2015.07.032
Sellami, K., Feddaoui, M., Labsi, N., Najim, M., and Benkahla, Y. K. (2019a). Numerical simulations of heat and mass transfer process of a direct evaporative cooler from a porous layer. J. Heat Transf. 141. doi:10.1115/1.4043302
Sellami, K., Feddaoui, M., Labsi, N., Najim, M., Oubella, M., and Benkahla, Y. K. (2019b). Direct evaporative cooling performance of ambient air using a ceramic wet porous layer. Chem. Eng. Res. Des. 142, 225–236. doi:10.1016/j.cherd.2018.12.009
Seppänen, O. A., Fisk, W. J., and Mendell, M. J. (1999). Association of ventilation rates and CO2 concentrations with health and other responses in commercial and institutional buildings. Indoor Air 9, 226–252. doi:10.1111/j.1600-0668.1999.00003.x
Shan, K., Wang, J., Hu, M., and Gao, D. (2019). A model-based control strategy to recover cooling energy from thermal mass in commercial buildings. Energy 172, 958–967. doi:10.1016/j.energy.2019.02.045
Sohani, A., Zabihigivi, M., Moradi, M. H., Sayyaadi, H., and Hasani Balyani, H. (2017a). A comprehensive performance investigation of cellulose evaporative cooling pad systems using predictive approaches. Appl. Therm. Eng. 110, 1589–1608. doi:10.1016/j.applthermaleng.2016.08.216
Sohani, A., Zabihigivi, M., Moradi, M. H., Sayyaadi, H., and Hasani Balyani, H. (2017b). A comprehensive performance investigation of cellulose evaporative cooling pad systems using predictive approaches. Appl. Therm. Eng. 110, 1589–1608. doi:10.1016/j.applthermaleng.2016.08.216
Sreeram, V., Gebrehiwot, B., Sathyanarayan, S., Sawant, D., Agonafer, D., Kannan, N., et al. “Factors that affect the performance characteristics of wet cooling pads for data center applications,” in Proceedings of the Annual IEEE Semiconductor Thermal Measurement and Management Symposium, San Jose, CA, USA, March 2015. doi:10.1109/SEMI-THERM.2015.7100160
Sun, Y., Ji, H., Cai, X., and Li, J. (2023). Joint extreme risk of energy prices-evidence from European energy markets. Finance Res. Lett. 56, 104036. doi:10.1016/J.FRL.2023.104036
Tafakkori, R., and Fattahi, A. (2021). Introducing novel configurations for double-glazed windows with lower energy loss. Sustain. Energy Technol. Assessments 43, 100919. doi:10.1016/j.seta.2020.100919
Taylor, J., McLeod, R., Petrou, G., Hopfe, C., Mavrogianni, A., Castaño-Rosa, R., et al. (2023). Ten questions concerning residential overheating in Central and Northern Europe. Build. Environ. 234, 110154. doi:10.1016/J.BUILDENV.2023.110154
Tejero-González, A., and Franco-Salas, A. (2021a). Optimal operation of evaporative cooling pads: A review. Renew. Sustain. Energy Rev. 151, 111632. doi:10.1016/J.RSER.2021.111632
Tejero-González, A., and Franco-Salas, A. (2021b). Optimal operation of evaporative cooling pads: A review. Renew. Sustain. Energy Rev. 151, 111632. doi:10.1016/j.rser.2021.111632
Vaseghi, M., Fazel, M., and Ekhlassi, A. (2020). Numerical investigation of solar radiation effect on passive and active heating and cooling system of a concept museum building. Therm. Sci. Eng. Prog. 19, 100582. doi:10.1016/j.tsep.2020.100582
Westerhold, T., Marwan, N., Drury, A. J., Liebrand, D., Agnini, C., Anagnostou, E., et al. (2020). An astronomically dated record of Earth’s climate and its predictability over the last 66 million years. Science 369 (6509), 1383–1387. doi:10.1126/science.aba6853
Williams, A. A., Baniassadi, A., Izaga Gonzalez, P., Buonocore, J. J., Cedeno-Laurent, J. G., and Samuelson, H. W. (2020). Health and climate benefits of heat adaptation strategies in single-family residential buildings. Front. Sustain. Cities 2. doi:10.3389/frsc.2020.561828
Yagi, M., and Managi, S. (2023). The spillover effects of rising energy prices following 2022 Russian invasion of Ukraine. Econ. Analysis Policy 77, 680–695. doi:10.1016/J.EAP.2022.12.025
Yang, R., Yang, J., Wang, L., Xiao, X., and Xia, J. (2022). Contribution of local climate zones to the thermal environment and energy demand. Front. Public Health 10, 992050. doi:10.3389/fpubh.2022.992050
Yew, M. C., Yew, M. K., Saw, L. H., Ng, T. C., Chen, K. P., Rajkumar, D., et al. (2018). Experimental analysis on the active and passive cool roof systems for industrial buildings in Malaysia. J. Build. Eng. 19, 134–141. doi:10.1016/j.jobe.2018.05.001
Zavrl, E., El Mankibi, M., Dovjak, M., and Stritih, U. (2022). Experimental investigation of air-based active-passive system for cooling application in buildings. Sustain. Cities Soc. 85, 104031. doi:10.1016/j.scs.2022.104031
Zhao, J., Wang, P., Li, J., Gu, T., and Lu, J. (2022). Energy-saving and emission reduction system of data center heat pipe based on latent heat of water evaporation. Therm. Sci. 26 (5), 4475–4487. doi:10.2298/TSCI2205475Z
Zhao, L., and Liu, J. (2020). Operating behavior and corresponding performance of mechanical ventilation systems in Chinese residential buildings. Build. Environ. 170, 106600. doi:10.1016/j.buildenv.2019.106600
Keywords: building cooling, temperature rise, cooling pads, overheating, indoor climate
Citation: Prozuments A, Borodinecs A, Lebedeva K and Palcikovskis A (2023) Examining the efficacy of cooling pad technology to address increasing building cooling demand in Latvia. Front. Built Environ. 9:1258625. doi: 10.3389/fbuil.2023.1258625
Received: 14 July 2023; Accepted: 23 August 2023;
Published: 01 September 2023.
Edited by:
Napoleon A. Enteria, Mindanao State University, PhilippinesReviewed by:
Roberto Alonso González-Lezcano, CEU San Pablo University, SpainSunil K. Sansaniwal, Malaviya National Institute of Technology, India
Copyright © 2023 Prozuments, Borodinecs, Lebedeva and Palcikovskis. This is an open-access article distributed under the terms of the Creative Commons Attribution License (CC BY). The use, distribution or reproduction in other forums is permitted, provided the original author(s) and the copyright owner(s) are credited and that the original publication in this journal is cited, in accordance with accepted academic practice. No use, distribution or reproduction is permitted which does not comply with these terms.
*Correspondence: Aleksejs Prozuments, YWxla3NlanMucHJvenVtZW50c0BydHUubHY=; Anatolijs Borodinecs, YW5hdG9saWpzLmJvcm9kaW5lY3NAcnR1Lmx2