- 1Department of Built Environment, Oslo Metropolitan University (OsloMet), Oslo, Norway
- 2Department of Civil and Architectural Engineering, Qatar University, Doha, Qatar
- 3Department of Materials, Mechanics, Management and Design, Delft University of Technology, Delft, Netherlands
Earthen structures have an important representation among the UNESCO World Heritage List sites as well as among the built environment in general. Unfortunately, earthen heritage structures are also numerous within the UNESCO List of World Heritage in Danger whereas other existing common earthen structures are extremely vulnerable to seismic and climate change events. Within the field of heritage conservation, structural analysis contributes to the safety evaluation of the structure, the diagnosis of the causes of damage and decay, and to the validation of interventions. Thus, the need to develop effective and accurate computational methods suitable for the study of both monumental and vernacular earthen structures becomes evident. This paper compiles, summarizes, and highlights the latest developments and implementations of computational methods for the study of such structure typologies. The literature has been explored following the PRISMA-S checklist methodology and a narrative synthesis was used for the presentation of results. Finally, future trends, opportunities, and challenges are discussed.
1 Introduction
The UNESCO World Heritage List (WHL) comprises 1157 sites in 167 countries (as of April 2023). Additionally, there are 55 properties inscribed in the UNESCO List of World Heritage in Danger (LWHD), which contains the sites that require major interventions to ensure conservation (UNESCO, 2023). In 2001, UNESCO established the World Heritage Earthen Architecture Programme (WHEAP) and published an inventory that identified the presence of Earth materials in 150 buildings on the WHL (as of 2012) (see Figure 1). Earthen architecture sites account for up to 25% of the enlisted sites on the LWHD, which indicates their vulnerability (UNESCO, 2007). The main factors affecting earthen architecture include floods and earthquakes, industrialization, urbanization, modern building technologies, the disappearance of traditional conservation practices, and specific causes of decay and structural failure (Jiménez Rios and O’Dwyer, 2018).
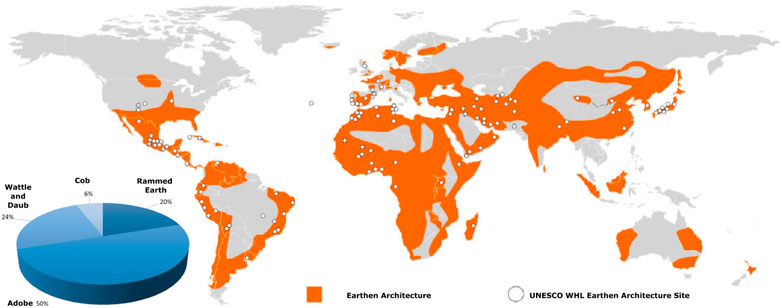
FIGURE 1. Global distribution of earthen architecture and location of UNESCO WHL sites on earthen architecture [adapted from Mužíková et al. (2018)] and percentage of use of the different earthen construction techniques on the listed sites included in the WHEAP inventory (Gandreau and Delboy, 2012).
Adobe walls consist of an arrangement of pieces or blocks assembled and glued together using clay mortars Teixeira et al. (2018). Different monolithic techniques implement the soil in different consistency states: humid for rammed Earth construction and plastic for cob. Rammed Earth requires the use of shuttering, whereas for cob, the earthen material is directly placed and this fact allows for great shape flexibility. Cob wall’s cohesion is provided mainly by the clay cementing properties and the added organic fibers such as straw or heather (Miccoli et al., 2014).
Regrettably, the state of conservation of the earthen-built environment is inadequate mainly due to: 1) the lack of knowledge and specialized personnel capable of implementing better-adapted strategies and restoration techniques, 2) natural degradation phenomena affecting 47% of the assets 3) negative climate change effects that affect 37% of the earthen-built stock Gandreau and Delboy (2012). Thus, the education and training of conservation stakeholders and technical staff needs to be improved, in particular, on the topic of computational methods implemented, as structural analysis contributes to the safety assessment and evaluation of the structures, the diagnosis of the causes of damage and decay, and the validation of conservation interventions (Roca et al., 2021).
Due to the great number of uncertainties involved in the study of existing earthen structures, the different numerical strategies adopted for their study usually rely on experimental campaigns (Jiménez Rios and O’Dwyer, 2020a; Jiménez Rios and O’Dwyer, 2020b; Jiménez Rios and O’Dwyer, 2020c) to determine the value of the needed input parameters and to validate the numerical results obtained. Within this context, an overview of the computational methods currently used for structural analysis of historic earthen buildings is presented. As only adobe, rammed Earth, and cob are commonly used to build load-bearing structural elements, the scope of this mini-review is limited to them.
2 Review methodology
The PRISMA-S checklist (Rethlefsen et al., 2021) was adopted in this mini-review to adequately document and report the process, ensure transparency, and facilitate reproducibility. Publications were searched in Scopus (Elsevier, 2023) and focused only on the main sources found. Relevant keywords were used and grouped into three different search queries within “TITLE-ABS-KEY”, for the years 2019-2023:
1. (“earthen heritage” OR “earthen historical building*” OR “earthen historical structure*” OR “earthen architect*” OR “earthen monument*”).
2. (adobe OR “rammed Earth” OR cob) AND (“computational method*” OR “numerical analy*”).
3. (adobe OR “rammed Earth” OR cob) AND (fem OR dem OR la OR “finite element” OR “discrete element” OR “limit analysis”).
The search was conducted on April 7, 2023. The records found were manually deduplicated, screened, and fully assessed to determine their suitability to be included in the mini-review. A narrative synthesis was adopted to summarize the information found in the records. The data has been first divided into the three main earthen construction typologies considered, namely, adobe, rammed Earth, and cob. Within each subsection, the information is presented and discussed based on the computational method applied following the four categories proposed by D’Altri et al. (2020): 1) Continuum Homogeneous Models [CHM, i.e., (Petracca et al., 2017)], 2) Block-Based Models [BBM, i.e., (D’Altri et al., 2018)], 3) Geometry-Based Models [GBM, i.e., (Jiménez Rios et al., 2022a; Jiménez Rios et al., 2022b; Nela et al., 2022, Nela et al., 2023)], and 4) Equivalent Frame Models [EFM, i.e., (Rinaldin et al., 2016)].
3 Review results
293 works were found. After deduplication, 278 unique records remained. The titles and abstracts were individually screened, from which 61 were shortlisted. These papers were read in full to assess their suitability to be included in this mini-review. 19 were removed because they did not deal directly with historical earthen structures, one more was removed since the numerical simulations presented on it did not relate to earthen construction techniques, and finally, one of them was removed as it did not present any numerical simulations. In total, 40 records were included in this review. The classification of the computational methods applied to the different earthen typologies discussed is presented in Table 1.
3.1 Adobe
Chácara et al. (2019) studied adobe using an EFM approach. They developed a novel 3D macro-element model capable of reproducing both the in-plane and out-of-plane response of adobe walls while using fewer degrees of freedom in comparison with conventional Finite Element Modeling (FEM). With this simplified computational method, they adequately assessed the seismic behavior of an existing adobe church and validated their results against values obtained via detailed FEM models.
Aguilar et al. (2019) found good agreement between the results using a Limit Analysis (LA) kinematic approach and a detailed FEM model of a 16th-century adobe church in Peru. Furthermore, with the LA evaluation performed, they concluded the safety of the church under frequent and occasional earthquakes and its vulnerability in front of more severe events. The same computational methods were applied by Briceño et al. (2021) to the study of the San Pedro Apostol adobe church, also in Peru, and by Misseri et al. (2020) to study the feasible overturning mechanisms that could be activated on existing adobe walls, both unreinforced and reinforced with timber elements.
Moreover, a simplified LA approach based upon conventional Heyman assumptions was used by Greco and Lourenço (2021) as a first approach analysis of Casa Arones in Peru, to inform the further development of more detailed and computationally expensive FEM simulations. Alternatively, Briceño et al. (2019) adopted a simplified rapid screening method to determine the vulnerability of the Virgen de la Asuncion adobe heritage church, where the geometry of the building plays an important role in vulnerability assessment results (5 out of the 14 considered parameters were directly related to the geometry of the structure).
Damerji et al. (2019); Invernizzi (2019); Mendes et al. (2020) studied adobe using a BBM approach either through a detailed micro-modeling FEM or a Discrete Element Method (DEM) analysis. By using a FEM micro-model in ABAQUS software, Damerji et al. (2019) were capable of reproducing experimentally observed damage patterns suffered by an adobe wall under a cyclic loading scenario. On the other hand, their numerical model failed to replicate the energy dissipation trend observed by the laboratory specimen.
A sort of BBM numerical approach was implemented by Invernizzi (2019) not by modeling interfaces between adobe bricks and mortar, but by explicitly modeling the mortar and assigning different mechanical properties to it. These simulations were carried out in DIANA software to assess the effect of a proposed geogrid reinforcement technique, which proved to increase the strength and ductility of experimentally tested wallettes.
Contrary to FEM approaches, Mendes et al. (2020) adopted a DEM methodology in 3DEC software to assess the seismic performance of adobe historical buildings. Their results showed the most vulnerable elements of the case study analyzed, the Santiago Apostol church in Peru, and served to inform the recommendation of suitable conservation intervention.
Aguilar et al. (2019) tested a novel integral methodology in the adobe heritage church of San Juan Bautista de Huaro, Peru, and Briceño et al. (2021) implemented it in the San Pedro Apostol church. Their framework included the creation of a detailed FEM macro-modeling (informed by a combination of Terrestrial Laser Scanning (TLS) and photogrammetry) and calibration (aided by Operational Modal Analysis (OMA) tests) of the earthen structure in DIANA. The seismic assessment of the structure was carried out through pushover analysis of the calibrated FEM model, as done by Noel et al. (2019) in the San Sebastian church and by Briceño et al. (2019) in the Sacsamarca church, and capacity curves comparison.
Also using DIANA, Vuoto et al. (2022) evaluated the safety levels of Torre de la Vela, in the Alhambra, Spain. Through a non-linear macro-modeling pushover analysis, they confirmed the adequate resistance of the structure upon foreseeing seismic events. Barontini and Lourenço (2021) assessed the condition of Hotel El Comercio, an adobe historical structure in Peru, through pushover analysis. Adobe walls were modeled with shell elements and calibrated based on in-situ survey and test results. They concluded that their results could adequately describe the global behavior of the adobe structure if it could be reinforced to prevent the formation of local mechanisms of collapse. Greco and Lourenço (2021) also performed a detailed seismic assessment of Casa Arones. Their model adopted the Total Strain Rotating Crack Model (TSRCM) material and followed a macro-modeling approach. Results showed how well-connected tie beams and timber diaphragms can significantly improve the capacity of the structure against out-of-plane failure mechanisms. Finally, Giordano et al. (2020) used a macro-modeling approach to simulate the structural response of adobe arches, and to validate the replicability and broader applicability of a proposed capability index for damage detection in this kind of structure.
An interesting work on the dynamic characterization and seismic response of traditional Chinese adobe structures was done by Wu et al. (2021). To simulate the adobe walls, they implemented a simplified diagonal spring element in ANSYS software whose mechanical parameters were determined through the calibration of a solid adobe wall model. Meanwhile, Simou et al. (2020) aimed at reproducing the structural response of an adobe specimen under the three-point bending test using ANSYS so that their calibrated numerical model could be used in the study of whole adobe structures in the Chellah site, Morocco.
Henao et al. (2021) and Ospina Henao et al. (2021) used SAP2000 software to run a series of simplified linear elastic macro-modeling FEM simulations of adobe walls. Also in SAP 2000, Xekalakis and Christou (2022) implemented a linear elastic macro-modeling approach to study the dynamic response of plain and reinforced adobe walls with wooden ring beams, concluding that the reinforcement elements altered not only the displacement magnitude but also the stress distribution on the adobe structure. Similarly, linear analyses of adobe structures were performed by Tancredi et al. (2022), this time in ABAQUS. The dynamic analysis of a typical adobe building in Cusco, Peru, allowed the authors to identify the feasible kinematic collapse mechanisms of the studied building typology. Nevertheless, the authors recognized the need of adopting non-linear constitutive models to better describe the structural behavior of adobe walls. Also in ABAQUS, Al Aqtash and Bandini. (2020) performed a parametric non-linear analysis to assess the influence of wall thickness and water content in the out-of-plane structural response of existing adobe walls. Their approach consisted of decreasing the mechanical properties of the adobe in the moist areas using a macro-modeling strategy.
LS-DYNA software has also been employed to replicate the structural response of adobe structures. Parisi et al. (2019) implemented a macro-modeling approach to model and validate adobe walls under simple and diagonal compression tests achieving an accurate representation of load-displacement curves, crack patterns, local stresses, and deformation in comparison to experimentally observed results. Alternatively (Fages et al., 2022), used the MIDAS FEA software to calibrate a total-strain crack constitutive model capable of reproducing the structural behavior of adobe walls under a 2D plane stress simplification assumption. A further extension to 3D simulations would be required before this model could be used on the seismic vulnerability assessment of full heritage adobe structures, though.
3.2 Rammed Earth
Wangmo et al. (2019) performed both macro and micro FEM analysis in DIANA of rammed Earth walls subjected to pull-down forces, as an analogy to the lateral loads that walls may experience during a seismic event. Wangmo et al. (2021) simulated the effect that a metallic mesh reinforcement had in the retrofitting of existing rammed Earth walls. Good agreement was found in terms of peak load and crack location between both macro modeling and experimental results, but only the micro model allowed reproduction of the sliding failure mechanism observed on the laboratory specimens.
Bui et al. (2019b) implemented a 3D FEM model in ABAQUS to study the structural response of rammed Earth L-shaped walls, Bui and Bui (2020) simulated the dynamic in-plane behavior of rammed Earth walls meanwhile Bui T. L. et al. (2020b) studied their dynamic out-of-plane behavior. Their numerical approach considered the interfaces between rammed Earth layers and their results clearly showed the influence that these elements have on the numerical results.
Alternatively, Bui et al. (2019a) performed a DEM numerical modeling approach in the 3DEC software to assess the efficiency of a reinforcement approach of existing rammed Earth walls employing prestressed metallic roads placed vertically at the ends of the wall. Their simulations suggested a 25% increment for one-story walls and a 10% increment for two-story walls in terms of maximum horizontal load.
Fagone et al. (2019) performed simplified simulations of a rammed Earth arch using three different commercial software, namely, ANSYS, ABAQUS, and DIANA. However, they found discrepancies in the maximum load obtained with all tested software and also important differences between the load-displacement curves of the numerical simulations and the experimental results.
In DIANA, Allahvirdizadeh et al. (2019) found out the typical load capacity and damage mechanisms of a plain and a Textile Reinforced Mortar (TRM) reinforced u-shape wall through the macro modeling simulation whereas Allahvirdizadeh et al. (2021) analyzed TRM retrofitted rammed Earth walls under pushover and dynamic load scenarios. They both concluded that the proposed reinforcement technique studied would effectively increase the wall capacity and delay damage occurrence. Moreover, the proposed technique was judged as compatible with heritage structures and could be of great value to better preserve rammed earth-built heritage. Shrestha et al. (2020) used DIANA to simulate the strengthening technique applied in a series of rammed Earth blocks through a reinforced concrete dowel. Their computational models matched correctly the peak load of their experimental counterparts. Nevertheless, important discrepancies were observed between the force-displacement curves of the reported laboratory and numerical results.
Other structural response scenarios of rammed Earth walls have been simulated in ABAQUS, e.g., Bui Q. B. et al. (2020a) studied the load capacity of nails in existing rammed Earth walls and Chettri et al. (2021) assessed the seismic vulnerability of Pa Chim buildings (Buthanese rammed Earth vernacular constructions). The results from the former study led to suggesting the immediate strengthening of the built earthen Buthanese heritage stock due to its extreme vulnerability under future moderate and strong earthquakes.
Villacreses et al. (2021) assessed the influence of water content in the dynamic performance of rammed Earth structures using the open-source software OpenSees. They found an important effect in the structural stiffness of the rammed Earth wall due to the presence of water, thus confirming the well-known fact that the strength of earthen materials is inversely proportional to their water content. Moreover, Gil-Martín et al. (2022) applied ground motion displacement based on probabilistic seismic hazard analysis to their macro-modeling FEM models in ANSYS of the San Jeronimo Monastery, the Molino del Marques de Rivas, and the Puerta Elvira in Granada, Spain, to determine their fragility curves and the effectiveness of the restoration works performed in these buildings.
Alternatively, aside from the body of literature focused on CHM or BBM methodologies applied to rammed Earth walls, Ruiz et al. (2022) used a transformed section formulation, along with CHM FEM modeling in ABAQUS to evaluate the out-of-plane influence of steel plates added as reinforcement of existing rammed Earth walls. Their results showed increments between 300% and up to 500% in the ultimate load supported by the reinforced rammed Earth walls based on the amount of reinforcement installed. Nevertheless, the suitability of this reinforcing approach to heritage structures would be deemed at least controversial and most probably ill-advised.
Regardless of the recent advances in the detailed non-linear numerical modeling of rammed Earth structures, their computational cost prevents their broader use, as demonstrated by García-Macías et al. (2022). The authors had to replace their high-fidelity 3D FEM model of the Muhammad Tower in the Alhambra with a surrogate Kriging model to be able to integrate it into their online damage identification framework.
3.3 Cob
Numerical modeling of cob has been undertaken by Miccoli et al. (2019) using a FEM macro-modeling approach in DIANA. They assumed plane stress, homogeneity, and continuity of material. A TSRCM was used with a multi-linear definition of the stress/strain relationship for the compressive behavior with an initial linear segment of 0.3fc and a post-peak segment with a negative slope of 0.02 times the Young’s modulus and an exponential relationship for tensile behavior. Their calibrated model could replicate the development of the shear stress/shear strain relationship. On the other hand, the numerical model could not replicate the diagonal failure pattern observed in the experiments due to the non-symmetric conditions in the samples and the testing setup.
(Jiménez Rios and O’Dwyer, 2020d) presented a study on the simulation of cob’s nonlinear monotonic behavior using ANSYS and ABAQUS. The results were validated against the experimental outcomes reported by Miccoli et al. (2019) and the pros and cons of different available constitutive models were identified and discussed. The results showed that Concrete and Concrete Damaged Plasticity were the best constitutive material models to reproduce cob’s nonlinear monotonic behavior in ANSYS and ABAQUS, respectively.
4 Discussion and future perspectives
All works found have the main objective either to determine the causes of damage or to assess the safety level of the historical earthen structure under study. Adobe is by far the most studied earthen typology in the literature, thus, examples of all four different computational methods (CHM, BBM, GBM, and EFM) were found. The computational methods applied to the modeling of rammed Earth historical structures can be broadly classified into two main groups, namely, 1) those considering rammed Earth walls as monolithic elements and 2) those taking into account the effect of compression layers’ interfaces. As cob load-bearing structural walls are commonly formed by monolithic elements and their structural behavior is highly nonlinear, the preferred approaches adopted in the literature are the macro-modeling FEM, also classified as CHM.
Different pros and cons in terms of complexity, computational cost, fidelity of results, etc. are associated with the various numerical strategies available. A common aspect to all of them though is the relatively high level of uncertainties, both epistemic and aleatory, involved in the modeling and simulation of historical earthen structures. Thus, the combination of two or more computational methods is advised for cross-validation of results to overcome the uncertainty challenges.
Although many of the records found were not included because they do not deal directly with heritage structures, the fact that there is research being done on earthen structures presents a valuable opportunity for historical ones also, as relevant results could be extrapolated.
The key to adequate built heritage preservation lies in preventive action towards possible hazards to decrease their vulnerability. Therefore, the implementation of a Digital Twin paradigm (Jiménez Rios et al., 2023b), which is a generalized trend in the AEC industry, could greatly contribute to the improvement of cultural heritage conservation. As digital twins aid asset managers to take informed decisions about the optimal maintenance, retrofitting, and repairing of the physical asset, this results in extended life, operation costs reduction, as well as increased resilience and sustainability of the built environment.
Data availability statement
The datasets generated for this study can be found in the Zenodo repository (Jiménez Rios et al., 2023a).
Author contributions
AJR contributed to the conception and design of the study. AJR and SR-C performed the bibliographic search, organized the database, and wrote the first draft of the manuscript. VP and MN supervised the work. All authors contributed to the article and approved the submitted version.
Funding
This project has received funding from the European Union’s Horizon 2020 research and innovation programme under the Marie Sklodowska-Curie grant agreement No. 101066739. The APC was funded by Oslo Metropolitan University (OsloMet).
Acknowledgments
The content of the manuscript has previously appeared online in the form of a preprint (Jiménez Rios et al., 2023c).
Conflict of interest
The authors declare that the research was conducted in the absence of any commercial or financial relationships that could be construed as a potential conflict of interest.
Publisher’s note
All claims expressed in this article are solely those of the authors and do not necessarily represent those of their affiliated organizations, or those of the publisher, the editors and the reviewers. Any product that may be evaluated in this article, or claim that may be made by its manufacturer, is not guaranteed or endorsed by the publisher.
References
Aguilar, R., Noel, M. F., and Ramos, L. F. (2019). Integration of reverse engineering and non-linear numerical analysis for the seismic assessment of historical adobe buildings. Automation Constr. 98, 1–15. doi:10.1016/j.autcon.2018.11.010
Al Aqtash, U., and Bandini, P. (2020). Influence of wall thickness and water content on the out-of-plane stability of adobe walls. Infrastructures 5, 78. doi:10.3390/INFRASTRUCTURES5090078
Allahvirdizadeh, R., Oliveira, D. V., and Silva, R. A. (2021). Numerical investigation of the in-plane seismic performance of unstrengthened and trm-strengthened rammed Earth walls. Int. J. Archit. Herit. 15, 548–566. doi:10.1080/15583058.2019.1629507
Allahvirdizadeh, R., Oliveira, D. V., and Silva, R. A. (2019). Numerical modeling of the seismic out-of-plane response of a plain and trm-strengthened rammed Earth subassembly. Eng. Struct. 193, 43–56. doi:10.1016/j.engstruct.2019.05.022
Barontini, A., and Lourenço, P. B. (2021). Seismic safety assessment of mixed timber-masonry historical building: An example in lima, Peru. J. Earthq. Eng. 25, 872–891. doi:10.1080/13632469.2018.1540368
Briceño, C., Moreira, S., Noel, M. F., Gonzales, M., Vila-Chã, E., and Aguilar, R. (2019). Seismic vulnerability assessment of a 17th century adobe church in the peruvian andes. Int. J. Archit. Herit. 13, 140–152. doi:10.1080/15583058.2018.1497224
Briceño, C., Noel, M. F., Chácara, C., and Aguilar, R. (2021). Integration of non-destructive testing, numerical simulations, and simplified analytical tools for assessing the structural performance of historical adobe buildings. Constr. Build. Mater. 290, 123224. doi:10.1016/j.conbuildmat.2021.123224
Bui, Q. B., Bui, T. T., El-Nabouch, R., and Thai, D. K. (2019a). Vertical rods as a seismic reinforcement technique for rammed Earth walls: An assessment. Adv. Civ. Eng. 2019, 1–12. doi:10.1155/2019/1285937
Bui, Q. B., Bui, T. T., Jaffré, M., and Teytu, L. (2020a). Steel nail embedded in rammed Earth wall to support vertical loads: An investigation. Constr. Build. Mater. 234, 117836. doi:10.1016/j.conbuildmat.2019.117836
Bui, Q. B., and Bui, T. T. (2020). Seismic behaviour of rammed Earth walls: A time history analysis. Lect. Notes Civ. Eng. 54. 143–148. doi:10.1007/978-981-15-0802-8_19
Bui, Q. B., Bui, T. T., Tran, M. P., Bui, T. L., and Le, H. A. (2019b). Assessing the seismic behavior of rammed Earth walls with an l-form cross-section. Sustain. Switz. 11, 1296. doi:10.3390/su11051296
Bui, T. L., Bui, T. T., Bui, Q. B., Nguyen, X. H., and Limam, A. (2020b). Out-of-plane behavior of rammed Earth walls under seismic loading: Finite element simulation. Structures 24, 191–208. doi:10.1016/j.istruc.2020.01.009
Chácara, C., Pantò, B., and Aguilar, R. (2019). “Evaluation of the seismic response of a historical earthen structure based on a discrete macro-element modelling approach,” in 7th international conference on computational methods in structural dynamics and earthquake engineering, COMPDYN 2019. Editors M. Papadrakakis, and M. Fragiadakis (Greece: National Technical University of Athens).
Chettri, N., Gautam, D., and Rupakhety, R. (2021). From Tship Chim to Pa Chim: Seismic vulnerability and strengthening of Bhutanese vernacular buildings. Mason. Constr. Act. Seismic Regions 2021, 253–288. doi:10.1016/B978-0-12-821087-1.00009-0
D’Altri, A. M., de Miranda, S., Castellazzi, G., and Sarhosis, V. (2018). A 3d detailed micro-model for the in-plane and out-of-plane numerical analysis of masonry panels. Comput. Struct. 206, 18–30. doi:10.1016/j.compstruc.2018.06.007
D’Altri, A. M., Sarhosis, V., Milani, G., Rots, J., Cattari, S., Lagomarsino, S., et al. (2020). Modeling strategies for the computational analysis of unreinforced masonry structures: Review and classification. Archives Comput. Methods Eng. 27, 1153–1185. doi:10.1007/s11831-019-09351-x
Damerji, H., Yadav, S., Sieffert, Y., Vieux-Champagne, F., and Malecot, Y. (2019). “Damage investigation of adobe walls using numerical simulations,” in 7th international conference on computational methods in structural dynamics and earthquake engineering, COMPDYN 2019. Editors M. Papadrakakis, and M. Fragiadakis (Greece: National Technical University of Athens).
Fages, J. M., Tarque, N., Rodríguez-Mariscal, J. D., and Solís, M. (2022). Calibration of a total strain crack model for adobe masonry based on compression and diagonal compression tests. Constr. Build. Mater. 352, 128965. doi:10.1016/j.conbuildmat.2022.128965
Fagone, M., Loccarini, F., Ranocchiai, G., and Rotunno, T. (2019). “Numerical constitutive models of low tensile strength materials for the description of mechanical behavior of rammed Earth masonry,” in 6th international conference on mechanics of masonry structures strengthened with composite materials, MuRiCo6 2019. Editors A. Di Tommaso, C. Gentilini, and G. Castellazzi (Greece: Trans Tech Publications Ltd).
García-Macías, E., Hernández-González, I. A., Puertas, E., Gallego, R., Castro-Triguero, R., and Ubertini, F. (2022). Meta-model assisted continuous vibration-based damage identification of a historical rammed Earth tower in the alhambra complex. Int. J. Archit. Herit., 1–27. doi:10.1080/15583058.2022.2155883
Gil-Martín, L. M., Hdz.-Gil, L., Kohrangi, M., Menéndez, E., and Hernández-Montes, E. (2022). Fragility curves for historical structures with degradation factors obtained from 3d photogrammetry. Heritage 5, 3260–3279. doi:10.3390/heritage5040167
Giordano, E., Mendes, N., Masciotta, M. G., Clementi, F., Sadeghi, N. H., Silva, R. A., et al. (2020). Expeditious damage index for arched structures based on dynamic identification testing. Constr. Build. Mater. 265, 120236. doi:10.1016/j.conbuildmat.2020.120236
Greco, F., and Lourenço, P. B. (2021). Seismic assessment of large historic vernacular adobe buildings in the andean region of Peru. learning from casa arones in cusco. J. Build. Eng. 40, 102341. doi:10.1016/j.jobe.2021.102341
Henao, P. A. O., Ballesteros, R. D. O., and Vega, J. S. P. (2021). “Analysis of the mechanical behavior of adobe walls without reinforcement through computational modelling,” in 8th international conference days of applied mathematics, ICDAM 2021. Editors O. Valbuena, E. Gelvez-Almeida, and E. D. V. Nino (United Kingdom: IOP Publishing Ltd).
Invernizzi, S. (2019). “Numerical simulation of geogrid reinforced adobe walls,” in 6th international conference on mechanics of masonry structures strengthened with composite materials, MuRiCo6 2019. Editors A. Di Tommaso, C. Gentilini, and G. Castellazzi (Switzerland: Trans Tech Publications Ltd).
Jiménez Rios, A., Nela, B., Pingaro, M., Reccia, E., and Trovalusci, P. (2022a). Rotation and sliding collapse mechanisms for in plane masonry pointed arches: Statistical parametric assessment. Eng. Struct. 262, 114338. doi:10.1016/j.engstruct.2022.114338
Jiménez Rios, A., and O’Dwyer, D. (2020a). Adaptations of the flat jack test for its application in cob walls. MethodsX 7, 101003. doi:10.1016/j.mex.2020.101003
Jiménez Rios, A., and O’Dwyer, D. (2020b). Data collected from the experimental validation for the application of flat jack tests in cob walls. Data Brief 31, 105764. doi:10.1016/j.dib.2020.105764
Jiménez Rios, A., and O’Dwyer, D. (2020c). Experimental validation for the application of the flat jack test in cob walls. Constr. Build. Mater. 254, 119148. doi:10.1016/j.conbuildmat.2020.119148
Jiménez Rios, A., and O’Dwyer, D. (2020d). Numerical modeling of cob’s nonlinear monotonic structural behavior. Int. J. Comput. Methods 17, 1940013. doi:10.1142/S0219876219400139
Jiménez Rios, A., and O’Dwyer, D. W. (2018). Earthen buildings in Ireland. Boca Raton: CRC Press/Balkema.
Jiménez Rios, A., Pingaro, M., Reccia, E., and Trovalusci, P. (2022b). Statistical assessment of in-plane masonry panels using limit analysis with sliding mechanism. J. Eng. Mech. 148. doi:10.1061/(ASCE)EM.1943-7889.0002061
Jiménez Rios, A., Plevris, V., and Nogal, M. (2023a). Bibliographic data from the computational methods applied to earthen historical structures review. doi:10.5281/zenodo.7907456
Jiménez Rios, A., Plevris, V., and Nogal, M. (2023b). Bridge management through digital twin-based anomaly detection systems: A systematic review. Front. Built Environ. 9. doi:10.3389/fbuil.2023.1176621
Jiménez Rios, A., Plevris, V., and Nogal, M. (2023c). Computational methods applied to earthen historical structures preprint. doi:10.5281/zenodo.7907824
Mendes, N., Zanotti, S., and Lemos, J. V. (2020). Seismic performance of historical buildings based on discrete element method: An adobe church. J. Earthq. Eng. 24, 1270–1289. doi:10.1080/13632469.2018.1463879
Miccoli, L., Müller, U., and Fontana, P. (2014). Mechanical behaviour of earthen materials: A comparison between Earth block masonry, rammed Earth and cob. Constr. Build. Mater. 61, 327–339. doi:10.1016/j.conbuildmat.2014.03.009
Miccoli, L., Silva, R. A., Oliveira, D. V., and Müller, U. (2019). Static behavior of cob: Experimental testing and finite-element modeling. J. Mater. Civ. Eng. 31. doi:10.1061/(ASCE)MT.1943-5533.0002638
Misseri, G., Palazzi, C., and Rovero, L. (2020). Seismic vulnerability of timber-reinforced earthen structures through standard and non–standard limit analysis. Eng. Struct. 215, 110663. doi:10.1016/j.engstruct.2020.110663
Mužíková, B., Plaček Otcovská, T., and Padevět, P. (2018). Modulus of elascity of unfired rammed Earth. Acta Polytech. CTU Proc. 15, 63–68. doi:10.14311/APP.2018.15.0063
Nela, B., Jiménez Rios, A., Pingaro, M., Reccia, E., and Trovalusci, P. (2022). Limit analysis of locally reinforced masonry arches. Eng. Struct. 271, 114921. doi:10.1016/j.engstruct.2022.114921
Nela, B., Jiménez Rios, A., Pingaro, M., Reccia, E., and Trovalusci, P. (2023). Masonry arches simulations using cohesion parameter as code enrichment for limit analysis approach. Int. J. Mason. Res. Innovation 1, 1. doi:10.1504/IJMRI.2023.10053576
Noel, M. F., Moreira, S., Briceño, C., López-Hurtado, E., and Aguilar, R. (2019). “Seismic assessment of the church of san sebastian in cusco, Peru by means of pushover nonlinear analysis,” in RILEM bookseries (Netherlands: Springer).
Ospina Henao, P. A., Oñate Ballesteros, R. D., and Peñaranda Vega, J. S. (2021). “Analysis of the stresses obtained in adobe walls without reinforcement against lateral loads obtained through modeling in sap 2000,” in 11th international congress of physics engineering, CIIF 2021. Editors J. Granados Samaniego, F. Tavera Romero, R. T. Hernandez Lopez, and G. Lopez Gonsalez (London: Institute of Physics).
Parisi, F., Balestrieri, C., and Varum, H. (2019). Nonlinear finite element model for traditional adobe masonry. Constr. Build. Mater. 223, 450–462. doi:10.1016/j.conbuildmat.2019.07.001
Petracca, M., Pelà, L., Rossi, R., Zaghi, S., Camata, G., and Spacone, E. (2017). Micro-scale continuous and discrete numerical models for nonlinear analysis of masonry shear walls. Constr. Build. Mater. 149, 296–314. doi:10.1016/j.conbuildmat.2017.05.130
Rethlefsen, M. L., Kirtley, S., Waffenschmidt, S., Ayala, A. P., Moher, D., Page, M. J., et al. (2021). Prisma-s: An extension to the prisma statement for reporting literature searches in systematic reviews. Syst. Rev. 10, 39. doi:10.1186/s13643-020-01542-z
Rinaldin, G., Amadio, C., and Macorini, L. (2016). A macro-model with nonlinear springs for seismic analysis of urm buildings. Earthq. Eng. Struct. Dyn. 45, 2261–2281. doi:10.1002/eqe.2759
Roca, P., Pelà, L., and Molins, C. (2021). “The iscarsah guidelines on the analysis, conservation and structural restoration of architectural heritage,” in 12th International Conference on Structural Analysis of Historical Constructions (SAHC), Canada, 29 Semtember 2021.
Ruiz, D. M., Reyes, J. C., Bran, C., Restrepo, M., Alvarado, Y. A., Barrera, N., et al. (2022). Flexural behavior of rammed Earth components reinforced with steel plates based on experimental, numerical, and analytical modeling. Constr. Build. Mater. 320, 126231. doi:10.1016/j.conbuildmat.2021.126231
Shrestha, K. C., Aoki, T., Miyamoto, M., Wangmo, P., and Pema, (2020). In-plane shear resistance between the rammed Earth blocks with simple interventions: Experimentation and finite element study. Buildings 10, 57. doi:10.3390/buildings10030057
Simou, S., Baba, K., Akkouri, N., Lamrani, M., Tajayout, M., and Nounah, A. (2020). “Mechanical characterization of the adobe material of the archaeological site of chellah,” in 3rd GeoMEast international congress and exhibition on sustainable Civil infrastructures, GeoMEast 2019. Editors H. Ameen, M. Jamiolkowski, M. Manassero, and H. Shehata (Germany: Springer Science and Business Media B.V.).
Tancredi, A., Cocco, G., Spacone, E., and Brando, G. (2022). “Seismic assessment of a colonial adobe building in cusco, Peru,” in 8th international conference on structural engineering, mechanics and computation, SEMC 2022. Editor A. Zingoni (Balkema: CRC Press), 1980–1985. doi:10.1201/9781003348443-325
Teixeira, T., Konig, C., and Schwantner, U. (2018). BASEhabitat summer School 2018 handbook (linz, Austria: BASEhabitat). Linz, Austria: BASEhabitat.
UNESCO (2023). World heritage list statistics. https://whc.unesco.org/en/list/stat/ (Accessed on April 06, 2023).
Villacreses, J. P., Caicedo, B., Poveda, F., Yepez, F., Ibagón, L., and Buriticá, J. (2021). “A study of the influence of water content profile on the dynamic behavior of rammed Earth structures,” in 6th World congress on Civil, structural, and environmental engineering, CSEE 2021. Editors T. Abdoun, M. Arab, J. Clausen, J. Facciorusso, A. Fahmy, R. Goodaryet al. (Orléans: Avestia Publishing).
Vuoto, A., Ortega, J., Lourenço, P. B., Javier Suárez, F., and Claudia Núñez, A. (2022). Safety assessment of the torre de la vela in la alhambra, granada, Spain: The role of on site works. Eng. Struct. 264, 114443. doi:10.1016/j.engstruct.2022.114443
Wangmo, P., Shrestha, K. C., and Aoki, T. (2021). Exploratory study of rammed Earth walls under static element test. Constr. Build. Mater. 266, 121035. doi:10.1016/j.conbuildmat.2020.121035
Wangmo, P., Shrestha, K. C., Miyamoto, M., and Aoki, T. (2019). Assessment of out-of-plane behavior of rammed Earth walls by pull-down tests. Int. J. Archit. Herit. 13, 273–287. doi:10.1080/15583058.2018.1433903
Wu, C., Xue, J., Zhou, S., and Zhang, F. (2021). Seismic performance evaluation for a traditional Chinese timber-frame structure. Int. J. Archit. Herit. 15, 1842–1856. doi:10.1080/15583058.2020.1731626
Xekalakis, G., and Christou, P. (2022). “The contribution of wooden ring beams to the response of adobe structures,” in 4th European and mediterranean structural engineering and construction conference, EURO-MED-SEC-4 2022. Editors K. Holschemacher, U. Quapp, A. Singh, and S. Yazdani (Fargo: ISEC Press).
Keywords: adobe, rammed earth, cob, finite element method, discrete element method, limit analysis
Citation: Jiménez Rios A, Ruiz-Capel S, Plevris V and Nogal M (2023) Computational methods applied to earthen historical structures. Front. Built Environ. 9:1219108. doi: 10.3389/fbuil.2023.1219108
Received: 08 May 2023; Accepted: 07 June 2023;
Published: 15 June 2023.
Edited by:
Giovanni Castellazzi, University of Bologna, ItalyReviewed by:
Zeljana Nikolic, University of Split, CroatiaAntonio Maria D'Altri, University of Bologna, Italy
Copyright © 2023 Jiménez Rios, Ruiz-Capel, Plevris and Nogal. This is an open-access article distributed under the terms of the Creative Commons Attribution License (CC BY). The use, distribution or reproduction in other forums is permitted, provided the original author(s) and the copyright owner(s) are credited and that the original publication in this journal is cited, in accordance with accepted academic practice. No use, distribution or reproduction is permitted which does not comply with these terms.
*Correspondence: Alejandro Jiménez Rios, YWxlamFuZEBvc2xvbWV0Lm5v