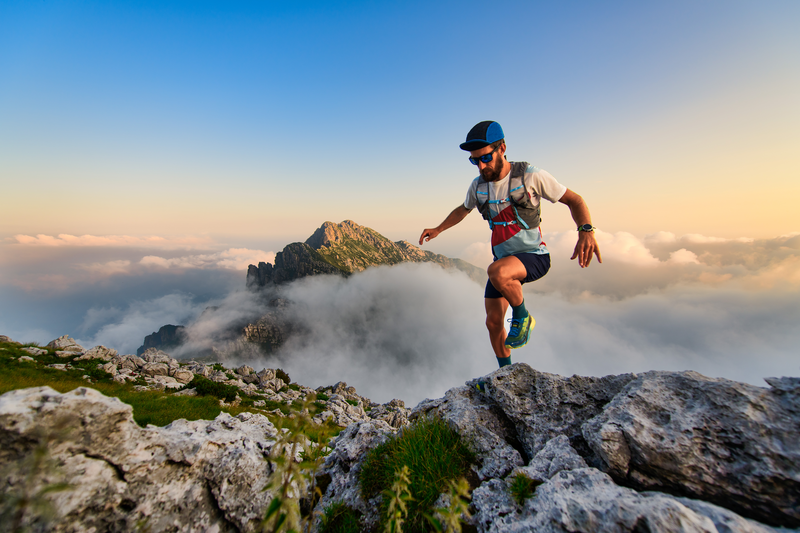
94% of researchers rate our articles as excellent or good
Learn more about the work of our research integrity team to safeguard the quality of each article we publish.
Find out more
MINI REVIEW article
Front. Built Environ. , 07 June 2023
Sec. Indoor Environment
Volume 9 - 2023 | https://doi.org/10.3389/fbuil.2023.1212920
This article is part of the Research Topic Global Excellence in Indoor Environment: 'Europe' View all 4 articles
The COVID-19 pandemic has shown that infection prevention actions need to be more efficient in public indoor environments. In addition to SARS-CoV-2, the cause of COVID-19, many pathogens, including other infectious viruses, antibiotic-resistant bacteria, and premise plumbing pathogens, are an invisible threat, especially in public indoor spaces. The indoor hygiene concept for comprehensive infection prevention in built environments highlights that the indoor environment should be considered as a whole when aiming to create buildings with increased infection prevention capacity. Within indoor environments, infections can indirectly spread through surfaces, air, and water systems. Many methods, such as antimicrobial technologies and engineering solutions, targeting these indoor elements are available, which aim to increase the hygiene level in indoor environments. The architectural design itself lays a foundation for more efficient infection prevention in public buildings. Touchless solutions and antimicrobial coatings can be applied to frequently touched surfaces to prevent indirect contact infection. Special ventilation solutions and air purification systems should be considered to prevent airborne infection transmissions. Proper design and use of water supply systems combined with water treatment devices, if necessary, are important in controlling premise plumbing pathogens. This article gives a concise review of the functional and available hygiene-increasing methods—concentrating on indoor surfaces, indoor air, and water systems—to help the professionals, such as designers, engineers, and maintenance personnel, involved in the different stages of a building’s lifecycle, to increase the infection prevention capacity of public buildings.
Despite the development of medicine, humankind still suffers from numerous infectious diseases. Emerging zoonotic viruses, drug-resistant bacteria and fungi, as well as well-known older pathogens, such as Legionella pneumophila and influenza viruses, are a concern (Kanarek et al., 2022; Mohapatra and Menon, 2022; Rehman, 2023). As attempts to treat infections have often turned out to be expensive and insufficient, more attention should be paid, in advance, to preventing infections.
The indoor environment plays an important role in mediating infections because people generally spend a lot of time indoors. Many infections can be transmitted through indoor environments, and the possible transmission routes are fomite transmission caused by touching contaminated surfaces, airborne transmission caused by breathing contaminated air, and waterborne transmission caused by being exposed to contaminated water (Dai et al., 2017).
Green building has gained much attention to achieve energy efficiency and low greenhouse gas emissions in construction (Doan et al., 2017; Udomiaye et al., 2022). However, few design and engineering-based measures have been employed to limit infection transmissions in public buildings, excluding healthcare facilities (Morawska et al., 2021). Thus, there is a need for designing, constructing, and renovating healthier buildings that can limit infection transmissions within built environments. Public buildings where many people pass through, such as public transport terminals and shopping centers, and buildings accommodating people with low immunity, such as nurseries and rest homes, should be the focus. Implementing solutions for infection prevention in indoor environments, such as antimicrobial materials, increases building costs, but it will also prevent economic losses in the form of medical treatment and sick leaves (Cutler and Summers, 2020; Falkinham, 2020; Abraham et al., 2021; Morawska et al., 2021).
We have previously introduced the indoor hygiene concept, summarized in Figure 1, which establishes a comprehensive infection prevention framework for built environments (Salonen et al., 2022). Creating healthy and hygienic buildings requires technical knowledge from the professionals, involved in different phases of the building’s lifecycle, on how to improve the infection-prevention capacity of indoor environments. To meet this challenge, the current review summarizes the available methods, which have capacity to decrease the spread of infections in indoor environments, concentrating on antimicrobial technologies and solutions targeted to indoor surfaces, indoor air, and water systems.
FIGURE 1. Simplified presentation of the indoor hygiene concept for creating hygienic indoor environments. All the elements of the indoor environment—surfaces, air, and water system—should be considered for infection prevention because of the different habitats of micro-organisms and different routes of infection transmission. Efficient infection prevention in built environments includes appropriate infection prevention methods targeted at all these elements. A hygienic indoor environment is created throughout a building’s lifecycle, starting from defining the hygiene objectives at an early stage, continuing to choose suitable solutions, and then implementing and maintaining them. A building’s lifecycle starts from the needs assessment and design stages and continues to construction, commissioning, and use (Salonen et al., 2022). The icons in the figure are downloaded from Icons8 (Icons8, 2023).
Construction engineering decisions influence the building’s infection-prevention capacity throughout its lifecycle. Health-related choices are made in the architectural, spatial, internal facilities, premise plumbing system, and HVAC (heating, ventilating, and air-conditioning) design.
The architectural design can support infection prevention by prioritizing compact, clear, and easy-to-clean structures and flexible design solutions to cope with changing demands. Adequate spacing is required to support social distancing when needed. Decreasing opportunities for close social interactions, for example, by designing private offices instead of densely populated open offices, lessens the probability of infection transmissions (Dietz et al., 2020; Udomiaye et al., 2020; Shepley et al., 2021). Building design can be utilized to control the flow of people and supply traffic. Separating dirty areas from clean ones should be carefully designed to prevent cross-contamination. Cleaning and maintenance rooms should be centrally located and easily accessible.
Spatial planning can support adequate ventilation, especially when utilizing natural ventilation, by avoiding closed-end corridors, lobbies, and waiting areas. In areas of abundant sunlight, adequate windows can allow daylight to reach the indoor space decreasing the spread of pathogens (Udomiaye et al., 2022).
High hygiene in furnishing and equipment can be pursued, for example, by choosing antimicrobial and antifouling materials and utilizing touchless technologies. When installing any product, the accumulation of dirt on the product’s surface should be minimal, and the product and its surroundings should be easily cleanable. Maintaining hand hygiene should be made easy, such as by appropriately locating hand sanitizer dispensers and hand washing points (Stiller et al., 2016; Clancy et al., 2021).
The next sections will discuss how to improve the infection prevention capacity of indoor surfaces, ventilation, and water systems. The available and functional infection-preventing technologies and solutions are summarized in Table 1.
TABLE 1. The available and functional antimicrobial technologies and solutions for indoor surfaces, HVAC, and water systems for establishing hygienic indoor environments with increased infection-prevention capacity. Determining the hygiene requirements for the indoor environment in question helps to select the appropriate solutions. The list of references is not fully comprehensive.
Microbial contamination on indoor surfaces often originates from people touching the surfaces with contaminated hands or causing airborne contamination settling on surfaces (Dai et al., 2017). Many harmful micro-organisms can stay viable on dry inanimate surfaces from hours to several months, thus increasing the likelihood of onward transmission via touching surfaces (Otter et al., 2013; Cook et al., 2016; Cassidy et al., 2020; Kampf et al., 2020; Riddell et al., 2020).
Frequent cleaning and disinfection are important when controlling the microbial load on surfaces. However, they are often not sufficient to fully eliminate harmful micro-organisms because of poor cleaning practices, overwhelming bioburden, and disinfectant tolerance (Dancer, 2014; Meyer et al., 2021). Cleaning is often not performed immediately after contamination, and, thus, there is time for infection transmission before cleaning. Replacing as many touch surfaces as possible with touchless options, such as touchless faucets, soap containers, and automatic doors and lights, helps to decrease human contact with surfaces (Dancer et al., 2021; Navaratnam et al., 2022).
Using antimicrobial materials that repel or kill microbes can also improve surface hygiene. Antimicrobial materials typically offer a continuous and nonspecific intervention targeting a wide spectrum of microbes, including bacteria, viruses, and fungi. Inactivation can occur even minutes after contamination depending on the used technology, the microbes present, and environmental conditions. Using antimicrobial materials on critical surfaces, such as door handles, handrails, and toilet flush buttons, would stop these surfaces from functioning as microbial reservoirs, thus reducing the risk of indirect contact infections. Antimicrobial material can be used as the surface itself, such as copper, or can be incorporated into a bulk material to be used, for example, as a paint, coating, or fabric.
Antimicrobial surfaces can be classified by their functional principle (Ahonen et al., 2017), but several mechanisms may also act in parallel (Adlhart et al., 2018). Different antimicrobial solutions for indoor surfaces are summarized in Table 1. Light-activated antimicrobial surfaces can excite electrons under a specific light, producing the reactive oxygen species (ROS) on the surface that degrade organic contaminants, including microbes. Titanium dioxide (TiO2) is probably the best-known light-activated antimicrobial material and is widely used in antimicrobial coatings (Shang et al., 2022). For better stability and action under visible light, TiO2 has been morphologically modified and doped with metal and non-metal elements (Nigussie et al., 2018; Schutte-Smith et al., 2023). For example, Ag-doped TiO2 caused the decomposition of Escherichia coli cells in 3 h under visible light (Endo et al., 2018).
Silver and copper are classified as release killing because the release of the ionic species is required for the antimicrobial effect (Ahonen et al., 2017). Copper and some copper alloys can destroy even over 99% of bacteria within 2 hours after contamination even after repeated contamination (Abraham et al., 2021). SARS-CoV-2 was inactivated in 4 h, coronavirus 229E in a couple of minutes, and norovirus in 5–30 min on copper surfaces (Warnes and Keevil, 2013; Warnes et al., 2015; van Doremalen et al., 2020). In real-life studies, copper surfaces have been shown to harbor 33%–90% fewer bacteria than conventional touch surfaces (Inkinen et al., 2017a; Colin et al., 2018). Hard surfaces and linens containing copper have been associated with fewer healthcare-associated infections (von Dessauer et al., 2016; Lazary et al., 2014; Marcus et al., 2017; Salgado et al., 2013; Sifri et al., 2016; Zerbib et al., 2020). Unlike copper, silver is more effective in moist surroundings or as silver compounds or nanoparticles, because silver is less susceptible to the surface oxidation required to produce the ionic species (Pietsch et al., 2020). When several fittings in a hospital setting were replaced with silver-incorporated replicates, the average microbial contamination was reduced by 96% (Taylor et al., 2009). However, studies on the effects of silver-containing surfaces on preventing infections are scarce.
When comparing antimicrobial coatings, the time required for the elimination of microbes is an important factor. The standards used for evaluating the efficacy of antimicrobial coatings often have a testing time of 24 h (ISO 22196:2011, 2011; ISO 21702:2019, 2019). However, a significant level of elimination should be more quickly reached for the coating to fulfill its purpose. Before installing antimicrobial coating, it is also important to consider that the possible release of antimicrobial agents from the coating may affect its shelf life and lead to environmental contamination (Rosenberg et al., 2019). The potential effects of antimicrobial surfaces on microbial communities and resistance need further study (Mäki, et al., 2023). Until then, the application of antimicrobial surfaces should be limited to frequently touched locations in public indoor environments (Dunne et al., 2018).
Regular cleaning maintains a hygienic indoor environment. Antimicrobial surfaces also need cleaning because dirtiness may hinder their function. The cleaning method should be suitable for the material in question to retain its desired function (Dunne et al., 2018). The cleanliness of surfaces can be verified, for example, by ATP or optical measurements (Inkinen et al., 2019; Kwan et al., 2019).
Harmful micro-organisms in indoor air typically originate when human carriers cough, sneeze, talk, or simply exhale. These actions spread microbes in droplets and aerosols to the surroundings. Droplets usually settle close to their origin, while aerosols can travel a longer distance and be inhaled, causing airborne transmission. For example, SARS-CoV-2 can remain infectious in aerosols for several hours, making airborne transmission a risk even when the source is not present anymore (van Doremalen et al., 2020). Although many viral diseases, such as chicken pox and measles, are well-known for airborne transmission, airborne bacteria, such as Mycobacterium tuberculosis, also cause infections (Fujiyoshi et al., 2017; Swaminathan et al., 2021).
HVAC systems have received attention because of the airborne spread of COVID-19 (Zhang et al., 2020a). Poor ventilation allows contagious aerosols to stay longer in indoor air and is thus associated with increased transmission of airborne infections (Guo et al., 2021). In general, higher outside air fractions and higher air exchange rates in buildings help to dilute indoor air contaminants, including pathogen-containing aerosols, thus decreasing the probability of infection transmission (Dietz et al., 2020). Demand-controlled, flexible ventilation can be adjusted to control energy use (Morawska et al., 2021). Different infection transmission-decreasing solutions for ventilation and air purification are summarized in Table 1.
In mechanical ventilation, air distribution should be designed to deliver external air to each part of the space to efficiently remove airborne pollutants. Exhaled aerosols can be transmitted both directly and via the room air distribution method. Mixing air ventilation—that is, mixing fresh air with polluted air—is not always the best choice. Displacement ventilation, which pushes pollutants upwards from the lower part of the room without mixing the polluted and fresh air, has shown better performance in contaminant removal efficiency. However, when the distance between two people is short, exposure to contaminants seems to be higher with displacement ventilation than with mixing ventilation (Olmedo et al., 2012; Cao et al., 2014a), probably because of the direct connection. Thus, choosing an optimal air distribution system is not straightforward and should be based on the dimensions, the heating strategy, and the planned use of the space. In a warm atmosphere, natural ventilation can sometimes provide a higher ventilation rate in an energy-efficient manner. In a hybrid approach, mechanical ventilation is available if necessary (Udomiaye et al., 2020).
Special ventilation solutions include personalized ventilation installed to workstations, and protective occupied zone ventilation, which separates the indoor area into a few subzones protected from one another (Cao et al., 2014a; Cao et al., 2014b; Cao et al., 2017). Pressure differentials between zones in the building should be controlled so that air flows from less contaminated to more contaminated areas (Guo et al., 2021). Physical barriers placed, for instance, to open offices, can mitigate the spread of aerosols, lowering the risk of infection transmission (Ren et al., 2021).
Air-conditioning systems in large buildings often require circulation of indoor air, especially when a larger cooling capacity is needed. Circulating indoor air creates a certain risk of airborne infection transmission. Air-conditioning systems have been associated with the transmission of SARS-CoV-2 and L. pneumophila (Hamilton et al., 2018; Lu et al., 2020; Elsaid and Ahmed, 2021). It is sometimes not possible to increase the ventilation rate enough to lower the risk of infection to an acceptable level (Blocken et al., 2021). These spaces can benefit from air filtration and disinfection strategies (Brągoszewska and Biedroń, 2021; Alvarenga et al., 2023). For example, SARS-CoV-2 was detected in the hospital ward air before the activation of HEPA air filtration and after its deactivation but not during the filter operation (Morris et al., 2022). In an intervention study implementation of an air purifier significantly decreased the number of microbes detected in the air and on surfaces. In addition, the number of hospital-acquired infections was lower when compared to the control space (Arikan et al., 2022). Special air purifiers can be portable or incorporated into a building’s HVAC system (Cheek et al., 2021). The air purifier should be selected carefully based on the required capacity and safe performance (Blocken et al., 2021).
For desired performance, the building’s HVAC system requires regular maintenance, such as replacing the filters and cleaning the air terminal units and ventilation ducts. The performance of the ventilation system can be monitored by certain parameters, such as temperature, carbon dioxide, humidity, and particle content.
In moist surroundings, many bacteria form biofilms with increased tolerance to biocides and other environmental factors. Building water systems are prone to develop microbiological problems because of high surface area-to-volume ratios, stagnation periods, diverse materials, and low disinfectant levels (McCoy and Rosenblatt, 2015). Biofilms in premise plumbing can form a reservoir for harmful micro-organisms that is difficult to destroy. Starting and stopping pumps as well as opening and closing valves create pressure shocks that may release biofilms into the drinking water. In addition, favorable conditions make biofilm microbes proliferate in water. Waterborne infections can be transmitted when exposed to contaminated water through the gastrointestinal tract, skin, or mucous membranes. In addition, the building’s water system, such as toilets and showers, generates aerosols that may cause infection transmissions via the respiratory tract (Dai et al., 2017). Water systems are an important source of L. pneumophila and Pseudomonas aeruginosa, both of which cause mild to severe infections (Moriz et al., 2010). Biofilms in handwashing sinks can also play a role in outbreaks (Breathnach et al., 2012; Roux et al., 2013; Franco et al., 2020).
The water treatment plants and distribution systems have limited potential to control opportunistic pathogens in a building’s plumbing systems. Thus, to reduce the risk of water-borne infection transmission, it is necessary to decrease microbial concentrations in premise plumbing. Implementing a water safety plan for public buildings is recommended (McCoy and Rosenblatt., 2015; Schmidt et al., 2019). Strategies to control premise plumbing pathogens are summarized in Table 1.
Water temperature is an important factor when preventing microbial growth in premise plumbing. Cold water should be kept below 20°C and warm water over 55°C, preferably 60°C at the outlets, to avoid temperatures favorable to micro-organisms. Energy saving often results in too low warm water temperatures, which encourages the growth of Legionella (Falkinham, 2020). In a 2-year study, the renovation of a hospital’s hot water pipelines and keeping the hot water temperatures around 60°C throughout the whole circuit led to the disappearance of Legionella from water samples (Quero et al., 2021). In this context, it is also important to adequately insulate water pipes. Premise hot water systems can be frequently decontaminated by raising the hot water temperature to around 70°C for a certain time and flushing the outlets with hot water (Gavalda et al., 2019). Despite decontamination, biofilms can protect the pathogens, and regrowth can happen within weeks or months (Cazals et al., 2022; Molina et al., 2022). Maintaining regular flow is also important to ensure that the cold water does not increase in temperature, which would enable colonization (Leslie et al., 2021).
Extended water retention time in pipelines and water stagnation in dead-ends or rarely used pipelines result in the loss of residual disinfectant and the proliferation of micro-organisms (Singh et al., 2020; Julien et al., 2022; Rahmatika et al., 2022). Water-efficient fixtures both increase water age and can cause aerosolization, increasing the risk of infection transmission (Leslie et al., 2021). Regular flushing, avoiding dead-ends, and correct sizing of a premise plumbing system help to decrease stagnation.
Copper-silver ionization has been successfully used to control Legionella and other opportunistic pathogens in public buildings, however, it must be properly designed, operated, and maintained to be effective. In a hospital case study, copper-silver ionization was installed in two hospital buildings where Legionella samples were regularly positive. After installation, the Legionella concentrations started to decline and were no more detected after 3 months (LeChevallier, 2023). In the case of disease outbreak or the detection of opportunistic pathogens in building water samples, on-site chemical disinfection can be useful, especially in facilities accommodating at-risk populations. However, biofilms can be 100 to 1,000 times less susceptible than planktonic bacteria to different disinfectants. Even prolonged treatment with chlorine-based disinfectants usually fails to remove all adherent biofilm (Zubris et al., 2017). Thus, reliable control of biofilms requires stringent and repeated cleaning strategies, aimed at physically disrupting them. Magnetic water treatment devices installed to premise plumbing have been shown to remove scales, hence limiting biofilm formation (Latva et al., 2016). In addition, generated nanobubbles may decrease biofilm formation (Xiao et al., 2020). Total eradication of opportunistic pathogens is still difficult to achieve. Instead, limiting their growth and human exposure should be pursued (Dancer, 2014; Julien et al., 2022).
Maintaining a building’s drinking water system requires verifying that the water temperatures remain within the required thresholds. The flow must be steady, without harmful pressure buildups. If automatic flushing is used, its function should be regularly checked. In spaces where the quality of water is critical, various parameters, such as water temperatures, disinfectant residuals, and bacterial counts, need to be monitored (Falkinham, 2020; Nakade et al., 2023).
COVID-19 has shown that more attention should be paid to the role of indoor environments in infection prevention, especially in public buildings. Being a current topic, infection prevention in indoor environments has been approached in some recent reports discussing healthy architecture, antimicrobial surfaces, and air purification strategies (Dietz et al., 2020; Udomiaye et al., 2020; Shepley et al., 2021; Alhusban and Alhusban, 2022; Amran et al., 2022; Navaratnam et al., 2022; Tokazhanov et al., 2022; Udomiaye et al., 2022; Yong and Calautit, 2023). The articles provide useful recommendations on how to prevent the spread of infections, in particular COVID-19, through air and contact surfaces.
To broaden the perspective to an even more comprehensive approach, the indoor environment should be considered as whole to establish buildings with increased infection prevention capacity. Indoor environments can mediate infections via air, surfaces, and the building’s water system. For example, antibiotic-resistant bacteria spread through contaminated indoor surfaces, and premise plumbing pathogens cause a threat via building water systems, especially to people with low immunity. The methods available for increasing indoor hygiene in these areas include building design, antimicrobial technologies and solutions, and cleaning and maintenance. These methods should be implemented already during the design and construction phases and throughout the building’s lifecycle. For this purpose, building design and engineering professionals involved in the early stage of the construction or renovation process need to be aware of the opportunities to limit infection transmissions via the indoor environment. Nominating a hygiene-dedicated expert for each construction or renovation project to help set the hygiene targets and monitor their fulfillment throughout the project might be useful (Salonen et al., 2022). Moreover, guidelines for constructing hygienic indoor environments, set by authorities or certificates, would be necessary when integrating the described methods throughout the building’s lifecycle.
The goal of infection prevention may sometimes conflict with other objectives, such as sustainability. Energy and water conservation strategies can enable pathogens to proliferate in a building’s water system. Thus, it is important to design and operate a building according to its purpose to keep the infection risk at an acceptable level. For example, hospitals have different requirements for indoor hygiene than museums or swimming halls. Flexible and demand-controlled design solutions help to adapt to changing situations.
Plenty of antimicrobial technologies and solutions for indoor surfaces, ventilation, and water systems are available and more are under research. It is not always easy to evaluate which of these are effective. More real-life studies are required to clarify the impacts of antimicrobial technologies and engineering solutions on the viability and spread of pathogens. However, no standard protocols are available, for example, for testing the antimicrobial efficacy of antimicrobial coatings in real-life settings. More research is also required to determine the effects of antimicrobial technologies and solutions, or more generally, hygienic indoor environments, on morbidity to infectious diseases, and demonstrate their cost-effectiveness.
NS prepared the manuscript. MA contributed to supervising the writing process, editing the text, and administering the project. KS contributed to supervising and editing the indoor air section. RM contributed to editing the text and acquiring funding. V-JA contributed to editing the text concerning clinical aspects. MK contributed to editing the indoor surfaces section. KS contributed to creating Figure 1. AP-H contributed to editing the building water systems section. ML contributed to supervising the writing process, editing the text, and acquiring funding. All authors contributed to the article and approved the submitted version.
This study was funded by the Ministry of Education and Culture in Finland and Satakunta University of Applied Sciences. It is a part of the project HEAL: healthier life with the comprehensive indoor hygiene concept. The grant number for the HEAL project is OKM/119/523/2021.
We thank the funders and all participants of the following project: HEAL: healthier life with the comprehensive indoor hygiene concept, 1/2022 onwards, the Ministry of Education and Culture, Finland.
The authors declare that the research was conducted in the absence of any commercial or financial relationships that could be construed as a potential conflict of interest.
All claims expressed in this article are solely those of the authors and do not necessarily represent those of their affiliated organizations, or those of the publisher, the editors and the reviewers. Any product that may be evaluated in this article, or claim that may be made by its manufacturer, is not guaranteed or endorsed by the publisher.
Abraham, J., Dowling, K., and Florentine, S. (2021). Can copper products and surfaces reduce the spread of infectious microorganisms and hospital-acquired infections. Materials 14, 3444. doi:10.3390/ma14133444
Adlhart, C., Verran, J., Azevedo, N. F., Olmez, H., Keinänen-Toivola, M. M., Gouveia, I., et al. (2018). Surface modifications for antimicrobial effects in the healthcare setting: A critical overview. J. Hosp. Infect. 99, 239–249. doi:10.1016/j.jhin.2018.01.018
Ahmadi, Y., Bhardwaj, N., Kim, K.-H., and Kumar, S. (2021). Recent advances in photocatalytic removal of airborne pathogens in air. Sci. Total Environ. 794, 148477. doi:10.1016/j.scitotenv.2021.148477
Ahonen, M., Kahru, A., Ivask, A., Kasemets, K., Kõljalg, S., Mantecca, P., et al. (2017). Proactive approach for safe use of antimicrobial coatings in healthcare settings: Opinion of the COST action network AMiCI. Int. J. Environ. Res. Public Health. 14, 366. doi:10.3390/ijerph14040366
Alhusban, S. A., and Alhusban, M. A. (2022). How the COVID 19 pandemic would change the future of architectural design. J. Eng. Des. 20, 339–357. doi:10.1108/JEDT-03-2021-0148
Al-Juboori, R., and Bowtell, L. (2019). “Ultrasound technology integration into drinking water treatment train,” in Sonochemical reactions (London: IntechOpen). doi:10.5772/intechopen.88124
Alvarenga, M. O. P., Dias, J. M. M., Lima, B. J. L. A., Gomes, A. S. L., and Monteiro, G. Q. M. (2023). The implementation of portable air-cleaning technologies in healthcare settings – A scoping review. J. Hosp. Infect. 132, 93–103. doi:10.1016/j.jhin.2022.12.004
Amran, M., Makul, N., Fediuk, R., Borovkov, A., Ali, M., and Zeyad, A. M. (2022). A review on building design as a biomedical system for preventing COVID-19 Pandemic. Buildings 12, 582. doi:10.3390/buildings12050582
Arikan, I., Genc, Ö., Uyar, C., Tokur, M. E., Balci, C., and Renders, D. P. (2022). Effectiveness of air purifiers in intensive care units: An intervention study. J. Hosp. Infect. 120, 14–22. doi:10.1016/j.jhin.2021.10.011
Azimi, P., and Stephens, B. (2013). HVAC filtration for controlling infectious airborne disease transmission in indoor environments: Predicting risk reductions and operational costs. Build. Environ. 70, 150–160. doi:10.1016/j.buildenv.2013.08.025
Bahri, M., and Haghighat, F. (2013). Plasma-based indoor air cleaning technologies: The state of the art-review. Clean. Soil Air Water 42, 1667–1680. doi:10.1002/clen.201300296
Bhagat, R. K., and Linden, P. F. (2020). Displacement ventilation: A viable ventilation strategy for makeshift hospitals and public buildings to contain COVID-19 and other airborne diseases. R. Soc. Open Sci. 7, 200680. doi:10.1098/rsos.200680
Binas, V., Venieri, D., Kotzias, D., and Kiriakidis, G. (2017). Modified TiO2 based photocatalysts for improved air and health quality. J. Materiomics. 3, 3–16. doi:10.1016/j.jmat.2016.11.002
Bishweshwar, P., Park, M., and Park, S.-J. (2019). Recent advances in TiO2 films prepared by sol-gel methods for photocatalytic degradation of organic pollutants and antibacterial activities. Coatings 9, 613. doi:10.3390/coatings9100613
Blocken, B., van Druenen, T., Ricci, A., Kang, L., van Hooff, T., Qin, P., et al. (2021). Ventilation and air cleaning to limit aerosol particle concentrations in a gym during the COVID-19 pandemic. Build. Environ. 193, 107659. doi:10.1016/j.buildenv.2021.107659
Blomberg, E., Herting, G., Rajarao, G. K., Mehtiö, T., Uusinoka, M., Ahonen, M., et al. (2022). Weathering and antimicrobial properties of laminate and powder coatings containing silver phosphate glass used as high-touch surfaces. Sustainability 14, 7102. doi:10.3390/su14127102
Brągoszewska, E., and Biedroń, I. (2021). Efficiency of air purifiers at removing air pollutants in educational facilities: A preliminary study. Front. Environ. Sci. 9, 709718. doi:10.3389/fenvs.2021.709718
Breathnach, A., Cubbon, M., Karunaharan, R., Pope, C., and Planche, T. (2012). Multidrug-resistant Pseudomonas aeruginosa outbreaks in two hospitals: Association with contaminated hospital waste-water systems. J. Hosp. Infect. 82, 19–24. doi:10.1016/j.jhin.2012.06.007
Buse, H. Y., Hall, J. S., Hunter, G. L., and Goodrich, J. A. (2022). Differences in UV-C-LED inactivation of Legionella pneumophila serogroups in drinking water. Microorganisms 10, 352. doi:10.3390/microorganisms10020352
Cao, G., Awbi, H., Yao, R., Fan, Y., Sirén, K., Kosonen, R., et al. (2014a). A review of the performance of different ventilation and airflow distribution systems in buildings. Energy Build. 73, 171–186. doi:10.1016/j.buildenv.2013.12.009
Cao, G., Sirén, K., and Kilpeläinen, S. (2014b). Modelling and experimental study of performance of the protected occupied zone ventilation. Energy Build. 68, 515–531. doi:10.1016/j.enbuild.2013.10.008
Cao, G., Liu, S., Boor, B., and Novselac, A. (2017). Dynamic interaction of a downward plane jet and a cough jet with respect to particle transmission: An analytical and experimental study. J. Occup. Environ. Hyg. 14, 618–631. doi:10.1080/15459624.2017.1316383
Cassidy, S. S., Sanders, D. J., Wade, J., Parkin, I. P., Carmalt, C. J., Smith, A. M., et al. (2020). Antimicrobial surfaces: A need for stewardship? PLOS Pathog. 16, e1008880. doi:10.1371/journal.ppat.1008880
Cazals, M., Bédard, E., Doberva, M., Faucher, S., and Prévost, M. (2022). Compromised effectiveness of thermal inactivation of Legionella pneumophila in water heater sediments and water, and influence of the presence of Vermamoeba vermiformis. Microorganisms 10, 443. doi:10.3390/microorganisms10020443
Cervia, J. S., Farber, B., Armellino, D., Klocke, J., Bayer, R.-L., McAlister, M., et al. (2010). Point-of-use water filtration reduces healthcare associated infections in bone marrow transplant recipients. Transpl. Infect. Dis. 12, 238–241. doi:10.1111/j.1399-3062.2009.00459.x
Cheek, E., Guercia, V., Shrubsole, C., and Dimitroulopoulou, S. (2021). Portable air purification: Review of impact on indoor air quality and health. Sci. Total Environ. 766, 1–41. doi:10.1016/j.scitotenv.2020.142585
Clancy, C., Delungahawatta, T., and Dunne, C. P. (2021). Hand-hygiene-related clinical trials reported between 2014 and 2020: A comprehensive systematic review. J. Hosp. Infect. 111, 6–26. doi:10.1016/j.jhin.2021.03.007
Colin, M., Klingelschmitt, F., Charpentier, E., Josse, J., Kanagaratnam, L., De Champs, C., et al. (2018). Copper alloy touch surfaces in healthcare facilities: An effective solution to prevent bacterial spreading. Materials 11, 2479. doi:10.3390/ma11122479
Cook, N., Knight, A., and Richards, G. P. (2016). Persistence and elimination of human norovirus in food and on food contact surfaces: A critical review. J. Food Prot. 79, 1273–1294. doi:10.4315/0362-028X.JFP-15-570
Cutler, D. M., and Summers, L. H. (2020). The COVID-19 pandemic and the $16 trillion virus. JAMA 324, 1495–1496. doi:10.1001/jama.2020.19759
Dai, D., Prussin, A. J., Marr, L. C., Vikesland, P. J., Edwards, M. A., and Pruden, A. (2017). Factors shaping the human exposome in the built environment: Opportunities for engineering control. Environ. Sci. Technol. 51, 7759–7774. doi:10.1021/acs.est.7b01097
Dancer, S. J., Li, Y., Hart, A., Tang, J. W., and Jones, D. L. (2021). What is the risk of acquiring SARS-CoV-2 from the use of public toilets? Sci. Total Environ. 792, 148341. doi:10.1016/j.scitotenv.2021.148341
Dancer, S. J. (2014). Controlling hospital-acquired infection: Focus on the role of the environment and new technologies for decontamination. Clin. Microbiol. Rev. 27, 665–690. doi:10.1128/CMR.00020-14
Demeersseman, N., Saegman, V., Cossey, V., Devriese, H., and Schuermans, A. (2023). Shedding a light on ultraviolet-C technologies in the hospital environment. J. Hosp. Infect. 132, 85–92. doi:10.1016/j.jhin.2022.12.009
Dietz, L., Horve, P. F., Coil, D. A., Fretz, M., Eisen, J. A., and Van Den Wymelenberg, K. (2020). 2019 novel coronavirus (COVID-19) pandemic: Built environment considerations to reduce transmission. Appl. Environ. Sci. 5, e00245–20. doi:10.1128/mSystems.00245-20
Doan, D. T., Ghaffarianhoseini, A., Naismith, N., Zhang, T., Ghaffarianhoseini, A., and Tookey, J. (2017). A critical comparison of green building rating systems. Build. Environ. 123, 243–260. doi:10.1016/j.buildenv.2017.07.007
Dunne, S. S., Ahonen, M., Modic, M., Crijns, F. R. L., Keinänen-Toivola, M. M., Meinke, R., et al. (2018). Specialized cleaning associated with antimicrobial coatings for reduction of hospital acquired infection. Opinion of the COST Action Network AMiCI (CA15114). J. Hosp. Infect. 99, 250–255. doi:10.1016/j.jhin.2018.03.006
Elsaid, A. M., and Ahmed, M. S. (2021). Indoor air quality strategies for air-conditioning and ventilation systems with the spread of the global coronavirus (COVID-19) epidemic: Improvements and recommendations. Environ. Res. 199, 111314–111330. doi:10.1016/j.envres.2021.111314
Encinas, N., Yang, C.-Y., Geyer, F., Kaltbeitzel, A., Baumli, P., Reinholz, J., et al. (2020). Submicrometer-sized roughness suppresses bacteria adhesion. Appl. Mat. Interfaces. 12, 21192–21200. doi:10.1021/acsami.9b22621
Endo, M., Wei, Z., Wang, K., Karabiyik, B., Yoshiiri, K., Rokicka, P., et al. (2018). Noble metal-modified titania with visible-light activity for the decomposition of microorganisms. Beilstein J. Nanotechnol. 9, 829–841. doi:10.3762/bjnano.9.77
Falkinham, J. O. (2020). Living with Legionella and other waterborne pathogens. Microorganisms 8, 2026. doi:10.3390/microorganisms8122026
Feng, Z., Cao, S.-J., Wang, J., Kumar, P., and Haghighat, F. (2021). Indoor airborne disinfection with electrostatic disinfector (ESD): Numerical simulations of ESD performance and reduction of computing time. Build. Environ. 200, 107956. doi:10.1016/j.buildenv.2021.107956
Franco, L. C., Tanner, W., Ganim, C., Davy, T., Edwards, J., and Donlan, R. (2020). A microbiological survey of handwashing sinks in the hospital built environment reveals differences in patient room and healthcare personnel sinks. Sci. Rep. 10, 8234. doi:10.1038/s41598-020-65052-7
Fujiyoshi, S., Tanaka, D., and Maruyama, F. (2017). Transmission of airborne bacteria across built environments and its measurement standards: A review. Front. Microbiol. 8, 2336. doi:10.3389/fmicb.2017.02336
Füszl, A., Zatorska, B., Van den Nest, M., Ebner, J., Presterl, E., and Diab-Elschahawi, M. (2021). The use of a UV-C disinfection robot in the routine cleaning process: A field study in an academic hospital. Antimicrob. Resist. Infect. Control 10, 84. doi:10.1186/s13756-021-00945-4
Gavalda, L., Garcia-Nunez, M., Quero, S., Gutierrez-Milla, C., and Sabria, M. (2019). Role of hot water temperature and water system use on Legionella control in a tertiary hospital: An 8-year longitudinal study. Water Res. 149, 460–466. doi:10.1016/j.watres.2018.11.032
Graeffe, F., Luo, Y., Guo, Y., and Ehn, M. (2023). Unwanted indoor air quality effects from using ultraviolet C lamps for disinfection. Environ. Sci. Technol. Lett. 10, 172–178. doi:10.1021/acs.estlett.2c00807
Guo, M., Xu, P., Xiao, T., He, R., Dai, M., and Miller, S. L. (2021). Review and comparison of HVAC operation guidelines in different countries during the COVID-19 pandemic. Build. Environ. 197, 107368–107369. doi:10.1016/j.buildenv.2020.107368
Hamilton, K. A., Prussin, A. J., Ahmed, W., and Haas, C. N. (2018). Outbreaks of legionnaires’ disease and pontiac fever 2006-2017. Curr. Environ. Health Rep. 5, 263–271. doi:10.1007/s40572-018-0201-4
Hernández-Díaz, D., Martos-Ferreira, D., Hernández-Abad, V., Villar-Ribera, R., Tarrés, Q., and Rojas-Sola, J. I. (2021). Indoor PM2.5 removal efficiency of two different non-thermal plasma systems. J. Environ. Manage. 278, 111515–111518. doi:10.1016/j.jenvman.2020.111515
Hwang, G. B., Huang, H., Wu, G., Shin, J., Kafizas, A., Karu, K., et al. (2020). Photobactericidal activity activated by thiolated gold nanoclusters at low flux levels of white light. Nat. Commun. 11, 1207. doi:10.1038/s41467-020-15004-6
Icons8 (2023). Icons8. www.icons8.com (Accessed April 19, 2023).
Imani, S. M., Ladouceur, L., Marshall, T., Maclachlan, R., Soleymani, L., and Difar, T. F. (2020). Antimicrobial nanomaterials and coatings: Current mechanisms and future perspectives to control the spread of viruses including SARS- CoV-2. ACS Nano 14, 12341–12369. doi:10.1021/acsnano.0c05937
Inagaki, H., Saito, A., Sugiyama, H., Okabayashi, T., and Fujimoto, S. (2020). Rapid inactivation of SARS-CoV-2 with deep-UV LED irradiation. Emerg. Microbes Infect. 9, 1744–1747. doi:10.1080/22221751.2020.1796529
Inkinen, J., Mäkinen, R., Keinänen-Toivola, M. M., Nordström, K., and Ahonen, M. (2017a). Copper as an antibacterial material in different facilities. Lett. Appl. Microbiol. 64, 19–26. doi:10.1111/lam.12680
Inkinen, J., Jayaprakash, B., Ahonen, M., Pitkänen, T., Mäkinen, R., Pursiainen, A., et al. (2017b). Bacterial community changes in copper and PEX drinking water pipeline biofilms under extra disinfection and magnetic water treatment. J. Appl. Microbiol. 124, 611–624. doi:10.1111/jam.13662
Inkinen, J., Ahonen, M., Iakovleva, E., Karppinen, P., Mielonen, E., Mäkinen, R., et al. (2019). Contamination detection by optical measurements in a real-life environment: A hospital case study. J. Biophot. 13, e201960069. doi:10.1002/jbio.201960069
ISO 21702:2019 (2019). Measurement of antiviral activity on plastics and other nonporous surfaces. Available at: https://www.iso.org/standard/71365.html (Accessed October 4, 2022).
ISO 22196:2011 (2011). Measurement of antibacterial activity on plastics and other nonporous surfaces. Available at: https://www.iso.org/standard/54431.html (Accessed October 4, 2022).
Izadyar, N., and Miller, W. (2022). Ventilation strategies and design impacts on indoor airborne transmission: A review. Build. Environ. 218, 109158. doi:10.1016/j.buildenv.2022.109158
Julien, R., Dreelin, E., Whelton, A. J., Lee, J., Aw, T. G., Dean, K., et al. (2020). Knowledge gaps and risks associated with premise plumbing drinking water quality. AWWA Water Sci. 2, 1–18. doi:10.1002/aws2.1177
Julien, R., Saravi, B., Nejadhashemi, A., Whelton, A. J., Aw, T. G., and Mitchell, J. (2022). Identifying water quality variables most strongly influencing Legionella concentrations in building plumbing. AWWA Water Sci. e1267. doi:10.1002/aws2.1267
Kampf, G., Todt, D., Pfander, S., and Steinmann, E. (2020). Persistence of coronaviruses on inanimate surfaces and their inactivation with biocidal agents. J. Hosp. Infect. 104, 246–251. doi:10.1016/j.jhin.2020.01.022
Kanarek, P., Bogiel, T., and Breza-Boruta, B. (2022). Legionellosis risk – An overview of Legionella spp. habitats in Europe. Environ. Sci. Pollut. Res. Int. 29, 76532–76542. doi:10.1007/s11356-022-22950-9
Kaur, R., and Liu, S. (2016). Antibacterial surface design – contact kill. Prog. Surf. Sci. 91, 136–153. doi:10.1016/j.progsurf.2016.09.001
Kowalski, W. J. (2009). “UVGI for air and surface disinfection,” in Ultraviolet germicidal irradiation handbook (New York. NY: Springer-Verlag), 2, 17–50. doi:10.1007/978-3-642-01999-9_2
Kwan, S. E., Peccia, J., Simonds, J., Haverinen-Shaughnessy, U., and Shaughnessy, R. J. (2019). Comparing bacterial, fungal, and human cell concentrations with rapid adenosine triphosphate measurements for indicating microbial surface contamination. Am. J. Infect. Control. 47, 671–676. doi:10.1016/j.ajic.2018.11.011
Lara, H. H., Ixtepan-Turrent, L., Yacaman, M. J., and Lopez-Ribot, J. (2020). Inhibition of Candida auris biofilm formation on medical and environmental surfaces by silver nanoparticles. ACS Appl. Mat. Interfaces. 12, 21183–21191. doi:10.1021/acsami.9b20708
Latva, M., Inkinen, J., Rämö, J., Kaunisto, T., Mäkinen, R., Ahonen, M., et al. (2016). Studies on the magnetic water treatment in new pilot scale drinking water system and in old existing real-life water system. J. Water Process Eng. 9, 215–224. doi:10.1016/j.jwpe.2016.01.009
Lazary, A., Weinberg, I., Vatine, J.-J., Jefidoff, A., Bardenstein, R., Borkow, G., et al. (2014). Reduction of healthcare-associated infections in a long term care brain injury ward by replacing regular linens with biocidal copper oxide impregnated linens. Int. J. Infect. Dis. 24, 23–29. doi:10.1016/j.ijid.2014.01.022
LeChevallier, M. (2023). Examining the efficacy of copper-silver ionization for management of Legionella: Recommendations for optimal use. Water Sci. 5, e1325. doi:10.1002/aws2.1327
Leslie, E., Hinds, J., and Hai, F. I. (2021). Causes, factors and control measures of opportunistic premise plumbing pathogens – A critical review. Appl. Sci. 11, 4474. doi:10.3390/app11104474
Lin, Y., Stout, J., and Yu, V. (2011). Controlling Legionella in hospital drinking water: An evidence-based review of disinfection methods. Infect. Control Hosp. Epidemiol. 32, 166–173. doi:10.1086/657934
Logan-Jackson, A. R., Batista, M. D., Healy, W., Ullah, T., Whelton, A. J., Bartrand, T. A., et al. (2023). A critical review on the factors that influence opportunistic premise plumbing pathogens: From building entry to fixtures in residences. Environ. Sci. Technol. 57, 6360–6372. doi:10.1021/acs.est.2c04277
Lu, J., Gu, J., Li, K., Xu, C., Su, W., Lai, Z., et al. (2020). COVID-19 outbreak associated with air conditioning in restaurant, guangzhou, China. Emerg. Infect. Dis. 26, 1628–1631. doi:10.3201/eid2607.200764
Mäki, A., Salonen, N., Kivisaari, M., Ahonen, M., and Latva, M. (2023). Microbiota shaping and bioburden monitoring of indoor antimicrobial surfaces. Front. Built Environ. 9. doi:10.3389/fbuil.2023.1063804
Marcus, E.-L., Yosef, H., Borkow, G., Caine, Y., Sasson, A., and Moses, A. E. (2017). Reduction of health care–associated infection indicators by copper oxide–impregnated textiles: Crossover, double-blind controlled study in chronic ventilator-dependent patients. Am. J. Infect. Control. 45, 401–403. doi:10.1016/j.ajic.2016.11.022
Mathew, S., Gangguly, P., Rhatigan, S., Kumaravel, V., Byrne, C., Hinger, S. J., et al. (2018). Cu-Doped TiO2: Visible light assisted photocatalytic antimicrobial activity. Appl. Sci. 8 (2067), 1–20. doi:10.3390/app8112067
McCoy, W. F., and Rosenblatt, A. A. (2015). HACCP-Based programs for preventing disease and injury from premise plumbing: A building consensus. Pathogens 4, 513–528. doi:10.3390/pathogens4030513
Meng, D., Liu, X., Xie, Y., Du, Y., Yang, Y., and Xiao, C. (2019). Antibacterial activity of visible light-activated TiO2 thin films with low level of Fe doping. Adv. Mat. Sci. Eng. 2019, 1–8. doi:10.1155/2019/5819805
Meyer, J., Nippak, P., and Cumming, A. (2021). An evaluation of cleaning practices at a teaching hospital. Am. J. Infect. Control. 49, 40–43. doi:10.1016/j.ajic.2020.06.187
Mitra, D., Kang, E.-T., and Neoh, K. G. (2020). Antimicrobial copper-based materials and coatings: Potential multifaceted biomedical applications. ACS Appl. Mat. Interfaces. 12, 21159–21182. doi:10.1021/acsami.9b17815
Modaresifar, K., Azizian, S., Ganjian, M., Fratila-Apachitei, L. E., and Zadpoor, A. A. (2019). Bactericidal effects of nanopatterns: A systematic review. Acta Biomater. 83, 29–36. doi:10.1016/j.actbio.2018.09.059
Mohapatra, S., and Menon, N. G. (2022). Factors responsible for the emergence of novel viruses: An emphasis on SARS-CoV-2. Curr. Opin. Environ. Sci. Health. 27, 100358. doi:10.1016/j.coesh.2022.100358
Molina, J. J., Bennassar, M., Palacio, E., and Crespi, S. (2022). Low efficacy of periodical thermal shock for long-term control of Legionella spp. in hot water systems of hotels. Pathogens 11, 152. doi:10.3390/pathogens11020152
Molloy, S. L., Ives, R., Hoyt, A., Taylor, R., and Rose, J. B. (2008). The use of copper and silver in carbon point-of-use filters for the suppression of Legionella throughput in domestic water systems. J. Appl. Microbiol. 104, 998–1007. doi:10.1111/j.1365-2672.2007.03655.x
Morawska, L., Tang, J. W., Bahnfleth, W., Bluyssen, P. M., Boerstra, A., Buonnonno, G., et al. (2020). How can airborne transmission of COVID-19 indoors be minimised? Environ. Int. 142, 105832–105837. doi:10.1016/j.envint.2020.105832
Morawska, L., Allen, J., Bahnfleth, W., Bluyssen, P. M., Boerstra, A., Buonanno, G., et al. (2021). A paradigm shift to combat indoor respiratory infection. Science 372, 689–691. doi:10.1126/science.abg2025
Moriz, M. M., Flemming, H.-C., and Wingender, J. (2010). Integration of Pseudomonas aeruginosa and Legionella pneumophila in drinking water biofilms grown on domestic plumbing materials. Int. J. Hyg. Environ. Health. 213, 190–197. doi:10.1016/j.ijheh.2010.05.003
Morris, A. C., Sharrocks, K., Bousfield, R., Kermack, L., Maes, M., Higginson, E., et al. (2022). The removal of airborne severe acute respiratory syndrome coronavirus 2 (SARS-CoV-2) and other microbial bioaerosols by air filtration on coronavirus disease 2019 (COVID-19) Surge Units. Clin. Infect. Dis. 75, e97–e101. doi:10.1093/cid/ciab933
Nakade, J., Nakamura, Y., Katayama, Y., Obata, H., Takahashi, Y., Zaimoku, Y., et al. (2023). Systematic active environmental surveillance successfully identified and controlled the Legionella contamination in the hospital. J. Infect. Chemother. 29, 43–47. doi:10.1016/j.jiac.2022.09.010
Navaratnam, S., Nguyen, K., Selvaranjan, K., Zhang, G., Mendis, P., and Aye, L. (2022). Designing post COVID-19 buildings: Approaches for achieving healthy buildings. Buildings 12, 74. doi:10.3390/buildings12010074
Nigussie, G. Y., Tesfamariam, G. M., Tegegne, B. M., Weldemichel, Y. A., Gebreab, T. W., Gebrehiwot, D. G., et al. (2018). Antibacterial activity of Ag-Doped TiO2 and Ag-doped ZnO nanoparticles. Int. J. Photoenergy. 2018, 1–7. doi:10.1155/2018/5927485
Offermann, F. J., Eagan, A., Offermann, A. C., Subhash, S. S., Miller, S. L., and Radonovich, L. J. (2016). Potential airborne pathogen transmission in a hospital with and without surge control ventilation system modifications. Build. Environ. 106, 175–180. doi:10.1016/j.buildenv.2016.06.029
Olmedo, I., Nielsen, P. V., de Adana, M. R., Jensen, R. L., and Grzelecki, P. (2012). Distribution of exhaled contaminants and personal exposure in a room using three different air distribution strategies. Indoor Air 22, 64–76. doi:10.1111/j.1600-0668.2011.00736.x
Olmos, D., and González-Benito, J. (2021). Polymeric materials with antibacterial activity: A review. Polymers 13, 613. doi:10.3390/polym13040613
Otter, J. A., Yezli, S., Salkeld, J. A. G., and French, G. L. (2013). Evidence that contaminated surfaces contribute to the transmission of hospital pathogens and an overview of strategies to address contaminated surfaces in hospital settings. Am. J. Infect. Control. 41, 6–11. doi:10.1016/j.ajic.2012.12.004
Pantelic, J., and Tham, K. W. (2013). Adequacy of air change rate as the sole indicator of an air distribution system's effectiveness to mitigate airborne infectious disease transmission caused by a cough release in the room with overhead mixing ventilation: A case study. HVAC&R Res. 19, 947–961. doi:10.1080/10789669.2013.842447
Pečnik, B., Hočevar, M., Širok, B., and Bizjan, B. (2016). Scale deposit removal by means of ultrasonic cavitation. Wear 356-357, 45–52. doi:10.1016/j.wear.2016.03.012
Pietsch, F., O’Neill, A. J., Ivask, A., Jenssen, H., Inkinen, J., Kahru, A., et al. (2020). Selection of resistance by antimicrobial coatings in the healthcare setting. J. Hosp. Infect. 106, 115–125. doi:10.1016/j.jhin.2020.06.006
Quach, N., Li, A., and Earthman, J. (2020). Interaction of calcium carbonate with nanobubbles produced in an alternating magnetic Field. ACS Appl. Mat. Interfaces. 12, 43714–43719. doi:10.1021/acsami.0c12060
Quero, S., Párraga-Niño, N., Garcia-Núñez, M., Pedro-Botet, M. L., Gavaldà, L., Mateu, L., et al. (2021). The impact of pipeline changes and temperature increase in a hospital historically colonised with Legionella. Sci. Rep. 11, 1916. doi:10.1038/s41598-021-81625-6
Rahmatika, I., Kurisu, F., Furumai, H., and Kasuga, I. (2022). Dynamics of the microbial community and opportunistic pathogens after water stagnation in the premise plumbing of a building. Microbes Environ. 37, ME21065. doi:10.1264/jsme2.me21065
Rehman, S. (2023). A parallel and silent emerging pandemic: Antimicrobial resistance (AMR) amid COVID-19 pandemic. J. Infect. Public Health. 16, 611–617. doi:10.1016/j.jiph.2023.02.021
Ren, C., Xi, C., Wang, J., Feng, Z., Nasiri, F., Cao, S.-J., et al. (2021). Mitigating COVID-19 infection disease transmission in indoor environment using physical barriers. Sustain. Cities Soc. 74, 1–19. doi:10.21203/rs.3.rs-373337/v1
Riddell, S., Goldie, S., Hill, A., Eagles, D., and Drew, T. W. (2020). The effect of temperature on persistence of SARS-CoV-2 on common surfaces. Virol. J. 17, 145. doi:10.1186/s12985-020-01418-7
Rosenberg, M., Ilić, K., Juganson, K., Ivask, A., Ahonen, M., Vrček, I. V., et al. (2019). Potential ecotoxicological effects of antimicrobial surface coatings: A literature survey backed up by analysis of market reports. PeerJ 7, e6315. doi:10.7717/peerj.6315
Roux, D., Aubier, B., Cochard, H., Quentin, R., and van der Mee-Marquet, N. (2013). Contaminated sinks in intensive care units: An underestimated source of extended-spectrum beta-lactamase-producing enterobacteriaceae in the patient environment. J. Hosp. Infect. 85, 106–111. doi:10.1016/j.jhin.2013.07.006
Salgado, C. D., Sepkowitz, K. A., John, J. F., Cantey, J. R., Attaway, H. H., Freeman, K. D., et al. (2013). Copper surfaces reduce the rate of healthcare-acquired infections in the intensive care unit. Infect. Control Hosp. Epidemol. 34, 479–486. doi:10.1086/670207
Salonen, N., Mäkinen, R., Ahonen, M., Mäkitalo, T., Pelto-Huikko, A., and Latva, M. (2022). A comprehensive indoor hygiene concept for infection prevention and control within built environments. Front. Built. Environ. 8, 1075009. doi:10.3389/fbuil.2022.1075009
Schmidt, I., Rickert, B., Schmoll, O., and Rapp, T. (2019). Implementation and evaluation of the water safety plan approach for buildings. J. Water Health 17, 870–883. doi:10.2166/wh.2019.046
Schutte-Smith, E., Erasmus, E., Mogale, R., Marogoa, N., Jayiya, N., and Visser, H. G. (2023). Using visible light to activate antiviral and antimicrobial properties of TiO 2 nanoparticles in paints and coatings: Focus on new developments for frequent-touch surfaces in hospitals. J. Coat. Technol. 20, 789–817. doi:10.1007/s11998-022-00733-8
Shang, C., Bu, J., and Song, C. (2022). Preparation, antimicrobial properties under different light sources, mechanisms and applications of TiO2: A review. Materials 15, 5820. doi:10.3390/ma15175820
Shepley, M. M., Kolakowski, H., Ziebarth, N., and Valenzuela-Mendoza, E. (2021). How COVID-19 will change health, hospitality and senior facility design. Front. Built Environ. 7, 1–10. doi:10.3389/fbuil.2021.740903
Sifri, C. D., Burke, G. H., and Enfield, K. B. (2016). Reduced health care-associated infections in an acute care community hospital using a combination of self-disinfecting copper-impregnated composite hard surfaces and linens. Am. J. Infect. Control. 44, 1565–1571. doi:10.1016/j.ajic.2016.07.007
Singh, R., Hamilton, K. A., Rasheduzzaman, M., Yang, Z., Kar, S., Fasnacht, A., et al. (2020). Managing water quality in premise plumbing: Subject matter experts’ perspectives and a systematic review of guidance documents. Water 12, 347. doi:10.3390/w12020347
Stiller, A., Salm, F., Bischoff, P., and Gastmeier, P. (2016). Relationship between hospital ward design and healthcare-associated infection rates: A systematic review and meta-analysis. Antimicrob. Resist. Infect. Control. 5, 51. doi:10.1186/s13756-016-0152-1
Swaminathan, N., Perloff, S. R., and Zuckerman, J. M. (2021). Prevention of Mycobacterium tuberculosis transmission in health care settings. Infect. Dis. Clin. N. Am. 35, 1013–1025. doi:10.1016/j.idc.2021.07.003
Szczotko, M., Orych, I., Maka, L., and Solecka, J. (2022). A review of selected types of indoor air purifiers in terms of microbial air contamination reduction. Atmosphere 13, 800. doi:10.3390/atmos13050800
Taylor, L., Phillips, P., and Hastings, R. (2009). Reduction of bacterial contamination in a healthcare environment by silver antimicrobial technology. J. Infect. Prev. 10, 6–12. doi:10.1177/1757177408099083
Tokazhanov, G., Tleuken, A., Guney, M., Turkyilmaz, A., and Karaca, F. (2022). How is COVID-19 experience transforming sustainability requirements of residential buildings? A review. Sustainability 12, 8732. doi:10.3390/su12208732
Udomiaye, E., Osondu, E. D., and Kalu, K. C. (2020). Architectural design strategies for infection prevention and control (IPC) in health-care facilities: Towards curbing the spread of covid-19. J. Environ. Health Sci. Eng. 18, 1699–1707. doi:10.1007/s40201-020-00580-y
Udomiaye, E., Ukpong, E., Kalu, K. C., Odum, C., and Okon, I. U. (2022). A review of sustainable design strategies for infection prevention and control (IPC) in public buildings. IOP Conf. Ser. Earth Environ. Sci. 1054, 012015. doi:10.1088/1755-1315/1054/1/012015
van Doremalen, N., Lloyd-Smith, J. O., Munster, V. J., Holbrook, M. G., Gamble, A., Williamson, B. N., et al. (2020). Aerosol and surface stability of HCoV-19 (SARS-CoV-2) compared to SARS-CoV-1. N. Engl. J. Med. 382, 16. doi:10.1101/2020.03.09.20033217
Verbič, A., Gorjanc, M., and Simončič, B. (2019). Zinc oxide for functional textile coatings: Recent advances. Coatings 9, 550. doi:10.3390/coatings9090550
von Dessauer, B., Navarrete, M. S., Benadof, D., Benavente, C., and Schmidt, M. G. (2016). Potential effectiveness of copper surfaces in reducing health care-associated infection rates in a pediatric intensive and intermediate care unit: A nonrandomized controlled trial. Am. J. Infect. Control. 44, e133–e139. doi:10.1016/j.ajic.2016.03.053
Walker, T., Canales, M., Noimark, S., Page, K., Parkin, I., Faull, J., et al. (2017). A light-activated antimicrobial surface is active against bacterial, viral and fungal organisms. Sci. Rep. 7, 15298. doi:10.1038/s41598-017-15565-5
Wang, Y., Wang, Y., Wang, Y., Murray, C., Hamblin, M. R., Hooper, D. C., et al. (2017). Antimicrobial blue light inactivation of pathogenic microbes: State of the art. Drug resist. updat. 33-35, 1–22. doi:10.1016/j.drup.2017.10.002
Warnes, S. L., and Keevil, C. W. (2013). Inactivation of norovirus on dry copper alloy surfaces. PLOS one 8, e75017. doi:10.1371/journal.pone.0075017
Warnes, S. L., Little, Z. R., and Keevil, C. W. (2015). Human coronavirus 229E remains infectious on common touch surface materials. mBio 6 (6), e01697–15. doi:10.1128/mBio.01697-15
Xiao, Y., Jiang, S. C., Wang, X., Muhammad, T., Song, P., Zhou, B., et al. (2020). Mitigation of biofouling in agricultural water distribution systems with nanobubbles. Environ. Int. 141, 105787. doi:10.1016/j.envint.2020.105787
Yong, X. L., and Calautit, J. K. (2023). A comprehensive review on the integration of antimicrobial technologies onto various surfaces of the built environment. Sustainability 15, 3394. doi:10.3390/su15043394
Zerbib, S., Vallet, L., Muggeo, A., de Champs, C., Lefebvre, A., Jolly, D., et al. (2020). Copper for the prevention of outbreaks of health care-associated infections in a long-term care facility for older adults. J. Am. Med. Dir. Assoc. 21, 68–71.e1. doi:10.1016/j.jamda.2019.02.003
Zhang, R., Li, Y., Zhang, A. L., Wang, Y., and Molina, M. (2020a). Identifying airborne transmission as the dominant route for the spread of COVID-19. PNAS 117, 14857–14863. doi:10.1073/pnas.2009637117
Zhang, J., Huntley, D., Fox, A., Gerhardt, B., Vatine, A., and Cherne, J. (2020b). Study of viral filtration performance of residential HVAC filters. Ashrae J. 62.8, 26–32. http://smsteam.co/wp-content/uploads/2020/12/Study-of-Viral-Filtration-Performance-ASHRAE.pdf
Zhong, L., and Haghighat, F. (2015). Photocatalytic air cleaners and materials technologies—abilities and limitations. Build. Environ. 91, 191–203. doi:10.1016/j.buildenv.2015.01.033
Zou, H., and Tang, H. (2019). Comparison of different bacteria inactivation by a novel continuous-flow ultrasound/chlorination water treatment system in a pilot scale. Water 11, 258. doi:10.3390/w11020258
Zou, Y., Zhang, Y., Yu, Q., and Chen, H. (2021). Dual-function antibacterial surfaces to resist and kill bacteria: Painting a picture with two brushes simultaneously. J. Mat. Sci. Technol. 70, 24–38. doi:10.1016/j.jmst.2020.07.028
Keywords: indoor environment, infection prevention, healthy buildings, antimicrobial surfaces, indoor air, premise plumbing
Citation: Salonen N, Ahonen M, Sirén K, Mäkinen R, Anttila V-J, Kivisaari M, Salonen K, Pelto-Huikko A and Latva M (2023) Methods for infection prevention in the built environment—a mini-review. Front. Built Environ. 9:1212920. doi: 10.3389/fbuil.2023.1212920
Received: 27 April 2023; Accepted: 30 May 2023;
Published: 07 June 2023.
Edited by:
Hasim Altan, Prince Mohammad Bin Fahd University, Saudi ArabiaReviewed by:
Giacomo Viccione, University of Salerno, ItalyCopyright © 2023 Salonen, Ahonen, Sirén, Mäkinen, Anttila, Kivisaari, Salonen, Pelto-Huikko and Latva. This is an open-access article distributed under the terms of the Creative Commons Attribution License (CC BY). The use, distribution or reproduction in other forums is permitted, provided the original author(s) and the copyright owner(s) are credited and that the original publication in this journal is cited, in accordance with accepted academic practice. No use, distribution or reproduction is permitted which does not comply with these terms.
*Correspondence: Noora Salonen, bm9vcmEuc2Fsb25lbkBzYW1rLmZp
Disclaimer: All claims expressed in this article are solely those of the authors and do not necessarily represent those of their affiliated organizations, or those of the publisher, the editors and the reviewers. Any product that may be evaluated in this article or claim that may be made by its manufacturer is not guaranteed or endorsed by the publisher.
Research integrity at Frontiers
Learn more about the work of our research integrity team to safeguard the quality of each article we publish.