- 1Department of Built Environment, Faculty of Engineering and Technology, Walter Sisulu University, Butterworth, South Africa
- 2cidb Centre of Excellence, Faculty of Engineering and the Built Environment, University of Johannesburg, Johannesburg, South Africa
The construction industry has been globally fingered as the major sector responsible for the continued deplorable state of the environment. The rising exploitation of the natural environment by the sector decapacitates the function of the flora and fauna to sustain life on earth. Therefore, the adoption and implementation of sustainability concepts in the construction industry are imperative to reduce the sector’s negative impacts on the environment. The growing field of biomimicry as a sustainability concept has increased global interest and call to maximize the numerous benefits offered by nature. This article is aimed at exploring biomimicry potentials in solving human challenges in a sustainable manner through responsible imitation, emulation, and drawing inspiration from nature. The first part of this paper explores the construction industry with rapt attention to its positive and negative impact on the human and natural environment. The second part provides a comprehensive overview of the biomimicry concept looking at its definitions, tenets, and sustainability standpoint. Finally, biomimicry inspiration, imitation, and emulation are discussed citing examples of their applications within and outside the built environment.
1 Introduction
The construction industry (CI) has been identified globally to enhance the quality of life and aid economic growth. The sector remains a significant employer and provider of employment opportunities thereby improving the socio-economic status of the people (Murray and Dainty, 2009). The CI provides infrastructures, buildings, and other basic and social amenities that have benefited mankind (Azis et al., 2012). As reported by Miller. (2002), the St. Louis construction industry’s employment increased during the period when Trans World Dome and Kiel Center were built. Also, the sector plays a significant role in the overall economic development of the United Arab Emirates (UAE) by contributing 14% to the gross domestic product (Faridi and El-Sayegh, 2006). As affirmed by Pero et al. (2017), the CI is the biggest industrial sector in the United States and Europe. Despite its multidimensional and complex nature (Ofori, 2000), the CI is key to globalization, industrialization, and achieving sustainable development. Owing to its numerous beneficial impacts on the human environment, the sector is continually financed, improved, and invested in by public and private entities with little or no regard for their environmental impacts. As indicated by Raftery et al. (1998), there is an increase in vertical incorporation of construction projects, private sector participation in construction projects, and foreign participation in domestic construction projects. To further establish the importance of the construction industry in the present fourth industrial revolution (4IR) era has also seen a massive influx and deployment of technologies and approaches with a high environmental footprint. This has led to the manifestation of dual impacts (positive and negative) on the environment by the CI.
It is common knowledge that the CI is directly linked to employment creation, socio-economic growth, and economic development globally. However, most of the processes and activities engaged by the sector are negatively impacting the natural environment. There are several adverse impacts of the CI as established in the literature. As summarized by Fuertes et al. (2013), these environmental impacts can be categorized as water emissions, waste generation, resource consumption, soil alteration, transport issues, local issues, effects on biodiversity, incidents, accidents, and emergencies, and lastly atmospheric emissions of greenhouse gases (GHGs), volatile organic compounds (VOCs), and Chlorofluorocarbons (CFCs). A major negative impact of the CI is construction-related accidents. According to Yan et al. (2020), construction accidents result in injuries, deaths, and grave financial consequences. High energy consumption and pollution are also other negative impacts of the CI. Interest in environmental health globally has been linked to noise pollution emanating from construction sites (Hamoda, 2008). Energy consumed in extracting, processing, and transporting construction raw materials results in increased pollution (Morel et al., 2001). The embodied and operational energy usage in the CI has been identified as one of the factors responsible for the continued rise in the atmospheric carbon footprint (Lawrence, 2015). Due to the persistent use of conventional materials, there has also been an increase in the construction and demolition waste (CDW) generated by the CI. From a Life Cycle Analysis (LCA) standpoint, the environmental impact of CDW is quite severe on both the human and natural environment (Coelho and de Brito, 2012). According to Benachio et al. (2020), the global CI is accountable for 25% of solid waste and 30% of the extracted natural resources. However, the study of González and Navarro. (2006) indicated that carbon dioxide emissions due to construction processes and operations are very noticeable and more profound. With the increasing release of GHGs into the atmosphere thereby precipitating ozone layer depletion, unnatural climate change, and global warming, it is imperative to urgently tackle these menaces. Hence, global attention is now on sustainability and digitalization as a means of addressing the adverse impacts of the CI.
Compared to the telecommunication, retail, and manufacturing sectors, the CI is one of the least digitalized industries with a low-level adoption of emerging technologies (Oguntona et al., 2022; Regona et al., 2022). There are several factors such as low awareness and age-long culture of resistance to change hindering the adoption of these technologies. However, the present 4IR era has seen the CI gradually integrating and utilizing emerging technologies to curb and minimize the environmental footprint of the sector. A few of these advanced technologies include robotics, artificial intelligence (AI), internet-of-things (IoT), blockchain, building information modeling (BIM), big data, autonomous vehicles, smart wearable technologies, and virtual and augmented reality among others (Perera et al., 2020; Abioye et al., 2021; McNamara and Sepasgozar, 2021; Opoku et al., 2021; Regona et al., 2022). Also, the global call for the adoption and implementation of sustainability in the CI is a major panacea to the negative environmental impacts exhibited by the sector. Likewise, there is mounting pressure on construction entities to ensure sustainability is an important consideration in the firm’s decision-making processes (Afzal et al., 2017). In addition, several challenges are hindering the transition to sustainability in the CI, and they can be categorized from the perspective of either operational, managerial, or strategic (Lima et al., 2021). Therefore, a truly sustainable CI is the solution to minimizing the negative impacts of the sector on the environment.
The sustainability concept has been widely preached and introduced into the CI. This is in response to the adverse impacts of the sector on both human and natural environments. Sustainable construction (SC) is a path through which the CI attains sustainable development (SD) by considering cultural, environmental, and socio-economic issues (Majdalani et al., 2006). Several terms are found in the literature to be used interchangeably with SC. These terms are green building (GB), sustainable building (SB), sustainable architecture, green construction, green, environmental or eco-friendly, and integrated design among others (Azis et al., 2012; Kubba, 2012; Zabihi et al., 2012; AlSanad, 2015; Kibert, 2016). SC entails a cradle-to-grave process in construction involving many role players while embracing technological and non-technical responses to social and economic sustainability, emphasizing environmental protection, and enhancement of individuals’ and communities’ quality of life (Du Plessis, 2007). The environmental aspect of sustainability has been majorly concentrated upon in the CI because its adverse impacts have been severe ecologically. As affirmed by Zabihi et al. (2012), the rationale behind sustainability in the CI is to focus on ecological conditions to achieve a built environment with maximum eco-friendly attributes that can counter the adverse impacts of the sector. It is therefore important to embrace SC in a manner that the triad of environmental, social, and economic issues are holistically addressed in the CI. As corroborated by Yip Robin and Poon. (2009), a sustainable culture among the stakeholders is imperative to driving the sustainability agenda in the CI. Hence, the clamor for the adoption and implementation of SC has led to the postulation of several practices or trends to aid the drive. A few of these are biomimicry, life cycle assessments , lean construction, whole building design, biophilia, ecological footprint, ecological rucksack, embodied energy, net-zero energy buildings, passive design, sustainable construction, resilience, environmental product declarations (EPDs), industrial ecology, the Natural Step, Factor 4 and Factor 10, life-cycle costing, construction ecology, eco-efficiency, the precautionary principle, and carrying capacity among others (Ortiz et al., 2009; Alves et al., 2012; Blizzard and Klotz, 2012; Kibert, 2016; Kibert and Fenner, 2017). These SC practices or trends in one way or another contribute their quota towards achieving sustainability. While most of them concentrate on an aspect of sustainability, some are aimed toward addressing the economic, environmental, and social aspects. However, biomimicry stood out among the list as one with the overarching goal of sustainability that is all-encompassing. Biomimicry as a concept solely focuses on and promises absolute sustainability (Ilieva et al., 2022).
The natural environment is a vast repository of innovative and sustainable ideas to solve the numerous challenges facing humanity. Natural organisms have been able to sustain themselves for over 3.8 billion years because of their capacity to address the countless issues they face. The abilities in nature to regenerate, sustain, overcome, and proffer sustainable solutions to their challenges which are like what the human environment is grappling with is what the biomimicry concept is premised upon. The knowledge, adoption, integration, and implementation of biomimicry thinking are therefore significant in today’s world owing to the alarming rate of environmental degradation and rapid climate change occurrence (Jamei and Vrcelj, 2021). Since it became popularized, the concept of biomimicry has been successfully applied globally across disciplines. According to Aboulnaga and Helmy. (2022), biomimicry is one of the biologically rooted sustainable mechanisms that aids the creation of an eco-friendly built environment. With nature consciousness instilled in humanity through the practice of biomimicry, a clear path towards sustainability is assured especially in the built environment discipline. Hence, this paper is aimed at exploring the avenues of nature inspiration, imitation, and emulation through which biomimicry thinking can accelerate the transition of the CI to a truly sustainable state. The next section gives a glimpse of the natural world, followed by an overview of the biomimicry concept, and lastly biomimicry thinking vis a vis nature as a source of inspiration, imitation, and emulation for sustainability. The paper will also explore the examples of innovative solutions, ideas, and technologies that are birthed as a result of biomimicry thinking through nature’s inspiration, imitation, and emulation.
2 Methodology
What are the avenues and strategies through which biomimicry can achieve its overarching goal of sustainability in the CI? To address this research question, this paper presents a review of relevant literature on the genius of nature and how it informs the biomimicry potential to aid the achievement of sustainability in the CI. According to Snyder. (2019), a literature review offers an exceptional mode of synthesizing findings from research to present evidence and unravel areas for further research which is critical to establishing theoretical and conceptual models. A critical analysis of the literature about nature and biomimicry vis-a-vis sustainability was conducted. Scholarly publications such as books, book chapters, journal articles, conference proceedings, and relevant resources from websites constitute the literature for this paper. Based on the examples of existing guidelines for conducting a literature review as presented by Snyder. (2019), Figure 1 presents a pictorial representation of the integrative literature review approach employed in this paper. The integrative literature review approach was used to critique and synthesize the subject of nature and biomimicry. The analysis and evaluation were qualitative and the examples of contribution were duly classified. Also, the AskNature database which is home to the ever-growing record of biological abstractions was utilized alongside published scholarly articles to present relevant nature-inspired innovative breakthroughs. These innovative technology solutions provide credence to the successful application and implementation of biomimicry thinking for sustainability in the CI.
3 The natural ecosystem: A glimpse
The natural environment has been a victim of the human environment’s parasitic relationship over the years. Human beings significantly depended on nature for everything ranging from food, shelter, security, and medicine among others. To pave the way for civilization and industrialization (with the construction of industrial, residential, religious, commercial, hospitality, and leisure facilities), the natural ecosystems are continually destroyed by humans leading to deforestation, the extinction of rare animals and plants, desertification, increased emission of GHGs, climate change, soil erosion, drought, flooding, and a host of other challenges. According to Zang et al. (2011), the goals of achieving socioeconomic development and improved quality of life are solely responsible for the degradation of the natural ecological systems. Therefore, it is imperative to consider the natural environment in every industrialization and urbanization drive to maximize the numerous deposits of benefits in the natural ecosystems. Since the human environment depends on nature for survival (food, water, air, etc), it is therefore imperative to ensure the conservation and preservation of the natural ecosystem.
The natural ecosystem is known to be a perfect or balanced system. This implies that the relationship that exists between the different constituting organisms ensures the stability of the ecosystem or the natural environment. Significantly, the flora and fauna of a natural ecosystem are heavily and cordially dependent on each other for survival and regeneration. For example, plants are known to deter the event of soil erosion, provide shelter, indirectly or directly provide nutrients to living organisms, and improve air quality by consuming carbon dioxide and releasing oxygen which is pivotal to the survival of humans and animals. There are also indoor plants that are utilized as a cost-effective indoor air purification mechanism (Damiati et al., 2022). Forests are also known for carbon sequestration, capture, and storage. The natural ecosystem is of immense value and benefit to the human environment through the various services they offer. According to Youmatter. (2020), the natural ecosystem offer four main services namely: regulating services (waste decomposition, crop pollination, pests and diseases control, regulation and purification of air and water, and climate regulation); provisioning services (renewable energy, food, water, and biochemicals, pharmaceuticals and industrial products). Others are cultural services (ecotourism, scientific discoveries, inspiration for spiritual, intellectual, and creativity purposes) and supporting and habitat services (primary reproduction, and seed and nutrient dispersal). Therefore, by attaining ecological sustainability and conservation of native biodiversity (Kuuluvainen, 2009), an array of opportunities to draw innovative solutions to human challenges will be possible.
Based on the numerous sustainable and amazing features exhibited by the natural ecosystem, the potential for innovative ideas to solve human challenges can be inferred and extracted through avenues of either or all of nature’s inspiration, imitation, or emulation. As corroborated by Hart. (1980), the natural ecosystem can be utilized as a rich source of informative ideas through the selective application of its principles. Nature principles (also known as Life’s principles) are the distinct attributes of natural organisms that enable them to thrive, live and survive sustainably and cordially in their over 3.8 billion years of existence. The study of Jacome Pólit. (2014) describes these principles as the abstracted forms, processes, functions, and strategies domiciled in natural organisms that ensure their regeneration. However, the book by Janine M. Benyus titled Biomimicry: Innovation Inspired by Nature and published in the year 1997 presented the nine (9) principles of nature. These principles resonate that nature runs on sunlight; nature recycles everything; nature uses only the energy it needs; nature fits form to function; nature demands local expertise; nature banks on diversity; nature taps the power of limits; nature rewards cooperation; and nature curbs excesses from within (Neill, 2018). These principles are what inform the formulation of biomimicry principles.
4 A cursory overview of biomimicry
Despite the novelty surrounding the concept of biomimicry, the idea of learning and applying the knowledge gained from natural ecosystems dates to ancient times. Strategies in natural organisms were examined and adapted by early humans to meet their housing, security, health, food production, and agricultural needs among others (Murr, 2015). Numerous inventions and technologies of the past were influenced by the study of natural models thereby establishing that nature’s inspiration, imitation, and emulation started before the emergence of westernization (Dicks et al., 2019). An example is the use of animal representation and stylized leaves for the decorative and symbolic ornamentation of ancient edifices such as the Neolithic Gobekli Tepe, Greek temples, and the Egyptian Great Sphinx of Giza (Browning et al., 2014). The Greco-Roman dispensation also saw plants and trees as sources of inspiration to architects in the design of columns with a structural resemblance to tree trunks (Freitas and Leitão, 2019).
Biomimicry is used interchangeably with other terms that describe the concept of studying and learning from nature. Some of the terms are biomimetics, biophilic architecture, bio-inspired design, bio-inspiration, bionics, biomimesis, biognosis, bioanalogous design, and biophilia (Garcia, 2017; Graeff et al., 2019; Lee and Baek, 2019). Biomimicry is described by Jamei and Vrcelj. (2021) as a multidisciplinary field of science that encourages a robust collaboration between different stakeholders including engineers and biologists in creating sustainable solutions to human challenges. The study of ElDin et al. (2016) defines biomimicry as the study of strategies, procedures, systems, and elements in the natural ecosystem to solve human challenges. According to Benyus. (1997), biomimicry entails the exploration and copying of nature’s geniuses such as photosynthesis, self-cleaning, and self-assembling to solve the challenges facing humanity. Similarly, Araque et al. (2021) describe it as an imitation science that combines engineering and biology to achieve the goal of sustainability for human development. Several barriers have been identified in the literature to impede the adoption and practice of biomimicry in the CI and other sectors. According to the study of Oguntona and Aigbavboa. (2019a), the four underlying factors hindering biomimicry adoption are knowledge-related, regulations-related, risk and cost-related, and information and technology-related. Also, lack of training and education, lack of awareness, and lack of professional knowledge is further identified as barriers to biomimicry adoption in the CI (Oguntona and Aigbavboa, 2019b). The analysis of the barriers identified in these two studies revealed a similar trend of a lack of a clear template and guidelines to adopt in the incorporation and implementation of biomimicry thinking for sustainability in the CI. For the overarching goal of sustainability to be achieved, Benyus. (1997) further indicated that the human relationship with nature must be from the three dimensions of “model, measure, and mentor”. By relating to the natural world as a model, the amazing attributes of natural organisms are studied and followed as a template to inspire the birth of novel sustainable solutions to human challenges. Nature as a measure on the other hand entails the assessment of innovations, ideas, technologies, solutions, and designs against Nature or Life’s principles to establish their sustainability compliance level. Lastly, by perceiving nature as a mentor, the natural ecosystem and organisms are constantly consulted as a rich database and source of innovative ideas for inspiration, emulation, and imitation. When the views and relationship of human beings and the natural environment are centered on these three dimensions (model, measure, and mentor), the level of environmental degradation and exploitation is bound to significantly diminish.
5 Biomimicry thinking for sustainability
Numerous kinds of research studies have indicated that nature is an embodiment of amazing and outstanding algorithms and solutions that requires diligent extraction and application to solve human challenges (Zang et al., 2010). According to Yan et al. (2021), biomimicry provides a viable blueprint for proffering innovative and eco-friendly solutions by learning from nature’s geniuses’ forms, principles, and models. Therefore, a successful abstraction and application of the biomimicry thinking concept will require a relationship with nature from the model, measure, and mentor perspectives. Hence, to maximize the vast potential of biomimicry to inform a seamless transition to sustainability in the CI, it is important to explore the triple avenues of nature inspiration, imitation, and emulation. Figure 2 shows a graphical representation of biomimicry’s thinking path to sustainability or sustainable development. It can be seen from the figure that the potential of biomimicry to achieve sustainable development can only be achieved when the trio of nature inspiration, emulation, and imitation is engaged fully as detailed in Section 5.1. The study of MacKinnon et al. (2020) also affirmed that biomimicry has the potential for sustainability, innovation, and transformation. The study performed a text-mining technique on 19 Biomimicry Global Network (BGN) web pages and created a word tree of quotes. Table 1 presents these attributes of biomimicry under the heading of its potential for sustainability, innovation, and transformation. The next section provides an overview and exploration of biomimicry thinking from these three contexts.
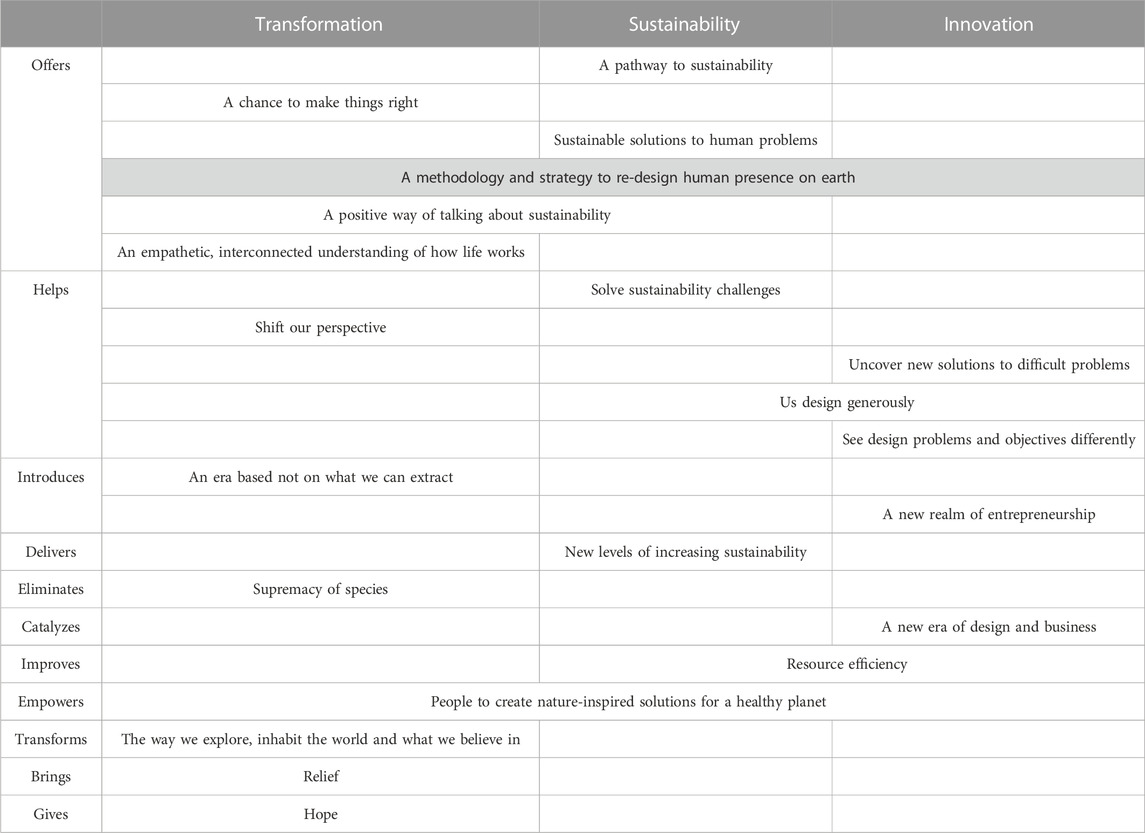
TABLE 1. Biomimicry potentials for sustainability, innovation, and transformation (MacKinnon et al. 2020).
5.1 Biomimicry thinking: Nature inspiration, imitation, and emulation
Nature has the potential to provide methodological innovations when consulted for inspiration (Zheng and Hong, 2021). As affirmed by Damiati et al. (2022), drasupplywing inspiration from nature has aided the manufacturing of versatile and effective modern technologies with sustainable attributes. Also, a wide range of microstructures with efficient mechanical attributes has been developed and perfected by nature in its years of evolution (Yan et al., 2021). According to Wurz et al. (2022), biosensing strategies exhibited in some natural organisms are shaping the research on the management of cytoskeletal dysregulation. Similarly, the resilient, self-healing, adaptive, and multifunctional characteristics of root systems offer insights into the design of coastal and civil infrastructure (Stachew et al., 2021). The study of Chenaghlou et al. (2020) further noted the inherent adaptivity attribute of natural structures under changing loading conditions (such as the self-centering system of the human spine) to control instability in long-span truss structures. A studious and keen observation of the natural ecosystems, therefore, has the potential to inspire the birth of novel technologies and solutions to the numerous challenges facing humanity today.
To date, several applications are birthed and inspired by one or more strategies in nature. According to El-Zeiny (2012), biomimicry thinking in the form of nature inspiration, imitation, and emulation can occur on three application levels of either or all of the forms, processes, and ecosystems of organisms. Based on the forms which entail the features of the organism itself, the biomimicry inspiration, emulation, and imitation emanate from their formal attributes such as shape, rhythm, color, transparency, and volumetric behavior. Others include self-assembly; construction materials and process; structure, stability, and gravity resistance; mutation, growth, and lifecycle; motion and aerodynamics; portability and mobility; organization and hierarchy of parts and systems; morphology, anatomy, modularity, and patterns; function and behavior; healing, recovery, survival and maintenance; homeostasis that balances internal systems while external forces change; and systems which include locomotive, sensory, excretory, nervous, muscular, skeletal, respiratory, circulatory, digestive, and organ systems. Based on the processes/strategies which entail the relationship of organisms with its biome of similar or other organisms that it may interact with, the biomimicry inspiration, emulation, and imitation emanate from their self-protection; communication; risk management; sensing, responding, and interaction; collaboration and teamwork; group management and coordination; hierarchy of community members; interaction with other creatures; transgenerational knowledge transfer and training; and survival techniques. Lastly, based on the ecosystem which entails the relationship and fitting of an organism in its biome or environment, the biomimicry inspiration, emulation, and imitation emanate from their shelter building; adjustment to change; contextual fit; response to climate by cooling, heating and ventilation solutions; waste management; input/output process cycling; limited resource management such as adaptation to the lack of water, light or food; response to the context of camouflaging, self-cleaning and self-protection; and adaptation to ecosystems (e.g adjustments to various sound or light levels, self-illumination and shading).
Nature is rich with countless sustainable and innovative ideas and solutions to the array of challenges the human environment is grappling with today. The natural world can serve as a mentor, measure, and model in addressing and proffering sustainable solutions to whatever challenge. According to Nerem and Schutte. (2014), the only way to guarantee the success of biomimicry applications is through a multidisciplinary integration which is a clear channel to having a deeper comprehension of the natural ecosystem for developing novel and innovative solutions. Whether there is a problem that requires an immediate solution or there is a particular biological strategy, process, or form that draws attention, biomimicry has the potential to birth the required sustainable solution. This is because the methodology of imitating, emulating, and drawing inspiration from the natural ecosystems is all geared towards the same overarching goal of sustainability. Therefore, to successfully engage any of these three biomimicry thinking paths, a challenge that requires a sustainable solution or an innovation that is intended to be created must be well defined. How does nature regulate, adapt, and mitigate climate change? How does nature conserve energy and water? How does nature process information? How does nature manage disturbances and stay resilient? How does nature coordinate, communicate and protect from harm? and How does nature distribute resources? These are a few of the questions to be posed in the application of biomimicry thinking in the form of nature inspiration, imitation, and emulation.
Biomimicry inspiration entails the rapt attention and observation of the feature/shape, strategies/processes, and organism ecosystem to inform an invention or innovation. Inventions borne out of such a path are usually shy of sustainability considerations at the initial stage. However, further interventions, updates, and upgrades can be incorporated to ensure the inventions or innovations pass the sustainability integrity test. Examples are the proposed design of flying machines by Leonardo da Vinci which are inspired by bats and birds. While da Vinci only drew sketches of the flying machines and never built one, his drawings paved the way for free flights, unmanned and manned electrically, mechanically, and robotically powered aircraft that now exist. Also, present-day airplanes have advanced far beyond the practice of flights in birds and the sketches of da Vinci upon which the idea emanated from.
Biomimicry imitation entails the outright simulation of the feature/shape (color, shape, structure, behavior, etc), process (survival, communication, interaction, or sensing techniques amongst others), and ecosystem (waste management, response to climate, adaptation or mitigation techniques amongst others) of an organism. While the immediate solution or innovation as a result of biomimicry imitation might not be entirely sustainable, it is important to note that such would have been able to solve a challenge and offer a platform for its further retrofit or update to conform to sustainability yardsticks. A known and perfect example of an industry innovation that employs biomimicry imitation is the i2 modular carpet tiles from Interface that imitates the forest floor which “has a wide variety of colors and textures, and feature shapes that repeat but in infinitely varied arrangements” (AskNature, 2021). The non-directional installation method of these recyclable and individually replaceable carpet tiles which are characterized by their variable color and patterns are installed in a non-directional way to create a cohesive design similar to a forest floor. The amazing fact about the i2 modular carpet tiles is their product transparency, carbon neutrality, resilience, cost-effectiveness, reusability, and recyclability. Another example is the invention of the Velcro versatile fastener which is applied in manufacturing, textiles, clothing, and medical services amongst others. The Velcro faster imitates the attachment of the tiny hooks that covered the burr seeds to mammal fur (AskNature, 2021).
Finally, biomimicry emulation entails careful and diligent abstraction of the amazing functionalities and strategies in a natural ecosystem. This biomimicry thinking path is the most rigorous, intense, and intriguing as it encompasses all three levels of biomimicry application. The successful incorporation and implementation of biomimicry emulation require a comprehensive collaboration between biologists, scientists, engineers, designers, and other disciplines as may be required or demanded by the task/project at hand. It is also at this level that biomimicry has the highest possibility of achieving its goal of sustainability. This is because innovative ideas, technologies, solutions, and designs borne out of this biomimicry thinking path are measured against Nature or Life’s principles to establish their level of sustainability compliance. The i2 modular carpet by Interface also fits an example of this biomimicry thinking path due to its verifiable sustainability characteristics.
There are several examples of designs, solutions, technologies, products, and projects that engaged biomimicry inspiration, emulation, and imitation. For example, the study of Ganpule et al. (2020) further reinforces other studies that investigate the biomedical response of a woodpecker as an impact mitigation strategy for minimizing the burden of chronic traumatic encephalopathy (CTE) and traumatic brain injury (TBI). While the woodpecker inspires scientific curiosity in such phenomenon among returning soldiers and American Football players, construction site workers and their Personal Protective Equipment (PPE) can also be drawn into the category. Another outstanding example of nature-inspired innovative products is the Lotusan self-cleaning paint (inspired by the self-cleaning attribute of the lotus plant’s leaves) that allows for the automatic removal of dirt on walls with the mere rinse of a rain shower (Sto SEA, 2021). The leaves of lotus plants stay dry and clean after rainfall as water drops collect specks of dirt, form water beads on the surface, and roll off like mercury due to the attribute of the leaves. Also, the i2 modular carpet by Interface is another nature-inspired product that emulates and imitates a forest floor. These carpets are individually replaceable, made from recycled and bio-based materials, stores carbon, and prevent their release into the atmosphere (Interface, 2022).
As presented by du Plessis et al. (2021), a few other examples of designs and projects birthed from biomimicry inspiration, emulation, and imitation are the Gherkin Tower in London, the Eastgate Centre in Zimbabwe, and the Esplanade in Singapore. The Gherkin Tower imitates the lattice-like exoskeleton of the basket sponge of the Venus flower to reduce wind deflections and disperse stress emanating from the strong water currents occurring in the underwater environment. The Eastgate Centre self-regulates the temperature of the building without any cooling or heating system by imitating and emulating the passive thermoregulation system similar to the gigantic mounds of African termites. Inspired by the spikes of the Durian fruit, the Esplanade imitates and emulates these spikes to provide shading and prevent overheating in hot climatic conditions. Similarly, the Japanese Shinkansen bullet train which is one of the world’s high-speed rail lines was modeled to imitate the Kingfisher bird to overcome the problem of tunnel sonic booms associated with it. As inferred from AskNature. (2021) which is a database for biological/nature strategies, innovations, and collections, Table 2 presents a careful selection of other innovative solutions birthed through biomimicry inspiration, emulation, and imitation. From Table 2, the potential benefits of these innovations and the United Nations Sustainable Development Goals they address are also presented. The innovations presented in this table emanated from the multidisciplinary collaboration between professionals, sustainability proponents, biomimicry practitioners, designers, biologists, and scientists in the industry and academic sphere. While most of these examples are not fully sustainable considering their life cycle analysis, encouraging the application of biomimicry, guided by Life’s principles via nature’s inspiration, emulation, and imitation is notwithstanding a reliable path to achieving sustainable development.
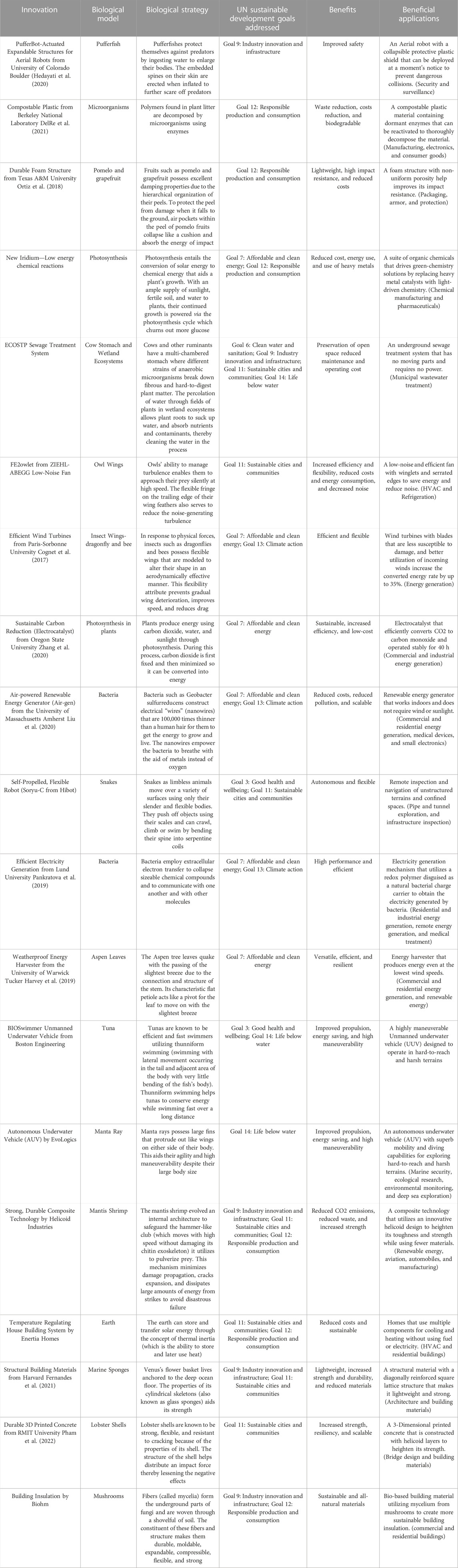
TABLE 2. Innovative solutions from biomimicry inspiration, emulation, and imitation (AskNature, 2021).
6 Conclusion and recommendations
This article sets out to explore biomimicry potentials in sustainably addressing human challenges through responsible imitation, emulation, and drawing inspiration from nature. Despite the various global sustainability agendas, the negative impact of the CI on the environment remains on the increase. Without a decisive and urgent quest toward the adoption and implementation of eco-friendly practices especially in the CI, the consequences in terms of climate change, ozone layer depletion, flood, drought, and other disasters will persist. Biomimicry thinking therefore offers a credible route to integrating sustainability values into the construction practices, design processes, activities, and systems. A major hindrance to the application and implementation of biomimicry for sustainability is the lack of a clear and simplified approach to its incorporation. The trio of emulation, inspiration, and imitation has been established as the ways through which the novel field of biomimicry can be maximally explored to aid the CI’s drive toward sustainability. When the amazing and eco-friendly strategies in nature are imitated, emulated, or drawn inspiration from to inform solutions to issues facing the human environment, the goal of achieving sustainability becomes a step closer. A few examples of technological and innovative solutions birthed through the application of these strategies were presented to authenticate the viability and potential of these biomimicry thinking routes to solving human challenges sustainably. The article was also able to establish the perception of nature as a mentor, measure, and model in a bid to maximally mine the sustainability treasures in the natural world. The exploitative disposition of human beings to the natural ecosystem is also bound to change when nature is viewed as a mentor, measure, and model of innovative and sustainable solutions to human challenges. It is also noteworthy that multinational companies and firms are now incorporating biomimicry and ecological consciousness into their organizational process to protect and conserve the environment. An example of such a company is Interface driven by the mission “to restore the health of the planet”. With the proliferation of biomimicry and global realignment to the natural ecosystem, more construction products, materials, and technologies will be conceptualized, manufactured, and commercialized. These will replace the conventional ones that are known to have significantly contributed to the high level of environmental degradation occurring today. It is therefore important that sustainability collaborative networks are empowered to ensure that biomimicry and nature awareness and consciousness are proliferated among all stakeholders. A robust and effective multidisciplinary collaboration is highly recommended for an accelerated provision of biomimicry-rooted innovative and sustainable solutions to the severe environmental challenges facing the world today. The incorporation of biomimicry thinking into the educational curriculum (from primary to tertiary level) is recommended to ensure that the number of biomimicry enthusiasts and sustainability proponents is on the rise which in turn will see a symbiotic relationship between the human and natural environment.
Author contributions
Conceptualization and organization, OO; Writing, OO; Review and editing, OO and CA; Supervision, CA.
Acknowledgments
We would like to acknowledge the works, support, and efforts of Claire Janisch who passed away on 7 February 2022. She was instrumental in helping to establish BiomimicrySA through advocacy, education, and practice of biomimicry thinking in the Southern Africa region. The author also acknowledges the support from the Department of Built Environment, Faculty of Engineering and Technology, and the Directorate of Research Development and Innovation, Walter Sisulu University, South Africa.
Conflict of interest
The authors declare that the research was conducted in the absence of any commercial or financial relationships that could be construed as a potential conflict of interest.
Publisher’s note
All claims expressed in this article are solely those of the authors and do not necessarily represent those of their affiliated organizations, or those of the publisher, the editors and the reviewers. Any product that may be evaluated in this article, or claim that may be made by its manufacturer, is not guaranteed or endorsed by the publisher.
References
Abioye, S. O., Oyedele, L. O., Akanbi, L., Ajayi, A., Delgado, J. M. D., Bilal, M., et al. (2021). Artificial intelligence in the construction industry: A review of present status, opportunities and future challenges. J. Build. Eng. 44, 103299. doi:10.1016/j.jobe.2021.103299
Aboulnaga, M., and Helmy, S. E. (2022). “Biomimicry in criticism: Argument, defense, and the direction toward sustainability,” in Biomimetic architecture and its role in developing sustainable, regenerative, and livable cities (Cham: Springer), 411–454.
Afzal, F., Lim, B., and Prasad, D. (2017). An investigation of corporate approaches to sustainability in the construction industry. Procedia Eng. 180, 202–210. doi:10.1016/j.proeng.2017.04.179
AlSanad, S. (2015). Awareness, drivers, actions, and barriers of sustainable construction in Kuwait. Procedia Eng. 118, 969–983. doi:10.1016/j.proeng.2015.08.538
Alves, T. D. C., Milberg, C., and Walsh, K. D. (2012). Exploring lean construction practice, research, and education. Eng. Constr. Archit. Manag. 19 (5), 512–525. doi:10.1108/09699981211259595
Araque, K., Palacios, P., Mora, D., and Chen Austin, M. (2021). Biomimicry-based strategies for urban heat island mitigation: A numerical case study under tropical climate. Biomimetics 6 (3), 48. doi:10.3390/biomimetics6030048
AskNature (2021). Innovations. Avaliable At: https://asknature.org/innovations/(Accessed December 2, 2022).
Azis, A. A. A., Memon, A. H., Rahman, I. A., Nagapan, S., and Latif, Q. B. A. I. (2012). “Challenges faced by construction industry in accomplishing sustainability goals,” in 2012 IEEE Symposium on Business, Engineering and Industrial Applications, Bandung, Indonesia, 23-26 September 2012 (IEEE), 630–634.
Benachio, G. L. F., Freitas, M. D. C. D., and Tavares, S. F. (2020). Circular economy in the construction industry: A systematic literature review. J. Clean. Prod. 260, 121046. doi:10.1016/j.jclepro.2020.121046
Blizzard, J. L., and Klotz, L. E. (2012). A framework for sustainable whole systems design. Des. Stud. 33 (5), 456–479. doi:10.1016/j.destud.2012.03.001
Browning, W. D., Ryan, C. O., and Clancy, J. O. (2014). 14 patterns of biophilic design: Improving health and well-being in the built environment. New York NY, USA: Terrapin Bright Green LLC.
Chenaghlou, M. R., Kheirollahi, M., Abedi, K., Akbari, A., and Fathpour, A. (2020). Inherent adaptive structures using nature-inspired compound elements. Front. Built Environ. 6, 561902. doi:10.3389/fbuil.2020.561902
Coelho, A., and De Brito, J. (2012). Influence of construction and demolition waste management on the environmental impact of buildings. Waste Manag. 32 (3), 532–541. doi:10.1016/j.wasman.2011.11.011
Cognet, V., Courrech du Pont, S., Dobrev, I., Massouh, F., and Thiria, B. (2017). Bioinspired turbine blades offer new perspectives for wind energy. Proc. R. Soc. A Math. Phys. Eng. Sci. 473, 20160726. doi:10.1098/rspa.2016.0726
Damiati, S., Mhanna, R., Awan, S. A., Kodzius, R., and Schuster, B. (2022). The dual-use dilemma for biomimicry. Front. Mol. Biosci. 531, 915663. doi:10.3389/fmolb.2022.915663
DelRe, C., Jiang, Y., Kang, P., Kwon, J., Hall, A., Jayapurna, I., et al. (2021). Near-complete depolymerization of polyesters with nano-dispersed enzymes. Nature 592 (7855), 558–563. doi:10.1038/s41586-021-03408-3
Dicks, H., and Blok, V. (2019). Can imitating nature save the planet? Environ. Values 28 (5), 519–526. doi:10.3197/096327119X15579936381780
du Plessis, A., Babafemi, A. J., Paul, S. C., Panda, B., Tran, J. P., and Broeckhoven, C. (2021). Biomimicry for 3D concrete printing: A review and perspective. Addit. Manuf. 38, 101823. doi:10.1016/j.addma.2020.101823
Du Plessis, C. (2007). A strategic framework for sustainable construction in developing countries. Constr. Manag. Econ. 25 (1), 67–76. doi:10.1080/01446190600601313
ElDin, N. N., Abdou, A., and ElGawad, I. A. (2016). Biomimetic potentials for building envelope adaptation in Egypt. Procedia Environ. Sci. 34, 375–386. doi:10.1016/j.proenv.2016.04.033
El-Zeiny, R. M. A. (2012). Biomimicry as a problem solving methodology in interior architecture. Procedia—Social and Behavioral Sciences 50, 502–512. doi:10.1016/j.sbspro.2012.08.054
Faridi, A. S., and El-Sayegh, S. M. (2006). Significant factors causing delay in the UAE construction industry. Constr. Manag. Econ. 24 (11), 1167–1176. doi:10.1080/01446190600827033
Fernandes, M. C., Aizenberg, J., Weaver, J. C., and Bertoldi, K. (2021). Mechanically robust lattices inspired by deep-sea glass sponges. Nat. Mater. 20 (2), 237–241. doi:10.1038/s41563-020-0798-1
Freitas, J., and Leitão, A. (2019). “Back to reality: Dendritic structures using current construction techniques. Intelligent and Informed,” in 24th international conference of the association for computer-aided architectural design research in asia (Hong Kong: CAADRIA) 1, 173–182.
Fuertes, A., Casals, M., Gangolells, M., Forcada, N., Macarulla, M., and Roca, X. (2013). An environmental impact causal model for improving the environmental performance of construction processes. J. Clean. Prod. 52, 425–437. doi:10.1016/j.jclepro.2013.02.005
Ganpule, S., Sutar, S., and Shinde, K. (2020). Biomechanical analysis of woodpecker response during pecking using a two-dimensional computational model. Front. Bioeng. Biotechnol. 8, 810. doi:10.3389/fbioe.2020.00810
Garcia, P. R. (2017). The influence of the concepts of biophilia and biomimicry in contemporary architecture. J. Civ. Eng. Archit. 11 (5), 500–513. doi:10.17265/1934-7359/2017.05.010
González, M. J., and Navarro, J. G. (2006). Assessment of the decrease of CO2 emissions in the construction field through the selection of materials: Practical case study of three houses of low environmental impact. Build. Environ. 41 (7), 902–909. doi:10.1016/j.buildenv.2005.04.006
Graeff, E., Maranzana, N., and Aoussat, A. (2019). Engineers’ and biologists’ roles during biomimetic design processes, towards a methodological symbiosis. Proc. Des. Soc. Int. Conf. Eng. Des. 1 (1), 319–328. doi:10.1017/dsi.2019.35
Hamoda, M. F. (2008). Modeling of construction noise for environmental impact assessment. J. Constr. Dev. Ctries. 13 (1), 79–89.
Hart, R. D. (1980). A natural ecosystem analog approach to the design of a successional crop system for tropical forest environments. Biotropica 12, 73–82. doi:10.2307/2388159
Hedayati, H., Suzuki, R., Leithinger, D., and Szafir, D. (2020). “Pufferbot: Actuated expandable structures for aerial robots,” in 2020 IEEE/RSJ International Conference on Intelligent Robots and Systems (IROS), Las Vegas, NV, USA, 24 October 2020 - 24 January 2021 (IEEE), 1338–1343.
Ilieva, L., Ursano, I., Traista, L., Hoffmann, B., and Dahy, H. (2022). Biomimicry as a sustainable design methodology—introducing the ‘biomimicry for Sustainability’Framework. Biomimetics 7 (2), 37. doi:10.3390/biomimetics7020037
Interface Inc (2022). We see carbon as a resource. Avaliable At: https://www.interface.com/US/en-US/sustainability/sustainability-overview.html (Accessed September 22, 2022).
Jácome Pólit, C. D. (2014). Regreening Nature: Turning negative externalities into opportunities. master’s thesis. TU Delft: Delft University of Technology.
Jamei, E., and Vrcelj, Z. (2021). Biomimicry and the built environment, learning from nature’s solutions. Appl. Sci. 11 (16), 7514. doi:10.3390/app11167514
Kibert, C. J., and Fenner, A. E. (2017). Sustainable manufacturing: Design and construction strategies for manufactured construction. Florida, US: University of Florida.
Kibert, C. J. (2016). Sustainable construction: Green building design and delivery. USA: John Wiley and Sons.
Kubba, S. (2012). Handbook of green building design and construction: LEED BREEAM, and green globes. USA: Butterworth-Heinemann.
Kuuluvainen, T. (2009). Forest management and biodiversity conservation based on natural ecosystem dynamics in northern europe: The complexity challenge. AMBIO A J. Hum. Environ. 38 (6), 309–315. doi:10.1579/08-a-490.1
Lawrence, M. (2015). Reducing the environmental impact of construction by using renewable materials. J. Renew. Mater. 3 (3), 163–174. doi:10.7569/jrm.2015.634105
Lee, S., and Baek, J. S. (2019). Nature-inspired design for self-organized social systems: A tool for collaborative communities. Proc. Des. Soc. Int. Conf. Eng. Des. 1 (1), 189–198. doi:10.1017/dsi.2019.22
Lima, L., Trindade, E., Alencar, L., Alencar, M., and Silva, L. (2021). Sustainability in the construction industry: A systematic review of the literature. J. Clean. Prod. 289, 125730. doi:10.1016/j.jclepro.2020.125730
Liu, X., Gao, H., Ward, J. E., Liu, X., Yin, B., Fu, T., et al. (2020). Power generation from ambient humidity using protein nanowires. Nature 578 (7796), 550–554. doi:10.1038/s41586-020-2010-9
Majdalani, Z., Ajam, M., and Mezher, T. (2006). Sustainability in the construction industry: A Lebanese case study. Constr. Innov. 6, 33–46. doi:10.1108/14714170610710613
MacKinnon, R. B., Oomen, J., and Zari, M. P. (2020). Promises and presuppositions of biomimicry. Biomimetics 5 (3), 1–14. doi:10.3390/biomimetics5030033
McNamara, A. J., and Sepasgozar, S. M. (2021). Intelligent contract adoption in the construction industry: Concept development. Automation Constr. 122, 103452. doi:10.1016/j.autcon.2020.103452
Miller, P. A. (2002). The economic impact of sports stadium construction: The case of the construction industry in St. Louis, MO. J. Urban Aff. 24 (2), 159–173. doi:10.1111/1467-9906.00120
Morel, J. C., Mesbah, A., Oggero, M., and Walker, P. (2001). Building houses with local materials: Means to drastically reduce the environmental impact of construction. Build. Environ. 36 (10), 1119–1126. doi:10.1016/s0360-1323(00)00054-8
Murr, L. E. (2015). “Biomimetics and biologically inspired materials,” in Handbook of materials structures, properties, processing and performance (Switzerland: Springer International Publishing), 521–552. doi:10.1007/978-3-319-01815-7_30
Murray, M., and Dainty, A. (2009). “Corporate social responsibility: Challenging the construction industry,” in Corporate social responsibility in the construction industry (UK: Taylor and Francis).
Neill, P. (2018). Biomimicry principles: Providing a framework for the future. Avaliable At: https://www.huffpost.com/entry/biomimicry-principles-providing-a-framework-for-the_b_5a5534dce4b0e3dd5c3f8ce4 (Accessed September 13, 2022).
Nerem, R. M., and Schutte, S. C. (2014). “The challenge of imitating nature,” in Principles of tissue engineering. 4th Edn (United Kingdom: Academic Press, Elsevier), 9–24. doi:10.1016/B978-0-12-398358-9.00002-1
Ofori, G. (2000). Globalization and construction industry development: Research opportunities. Constr. Manag. Econ. 18 (3), 257–262. doi:10.1080/014461900370627
Oguntona, O. A., and Aigbavboa, C. O. (2019a). Barriers hindering biomimicry adoption and application in the construction industry. Afr. J. Sci. Technol. Innovation Dev. 11 (3), 289–297. doi:10.1080/20421338.2018.1527968
Oguntona, O. A., and Aigbavboa, C. O. (2019b). “Barriers militating against the adoption of biomimicry as a sustainable construction practice,” in MATEC web of conferences 266 (03010), 1–6. doi:10.1051/matecconf/201926603010
Oguntona, O., Aigbavboa, C., Thwala, W., and Mautlane, M. (2022). Assessing the awareness level of emerging technologies for resilience in the construction industry. Proc. Int. Struct. Eng. Constr. 9, 1. doi:10.14455/10.14455/isec.2022.9(1).con-13
Opoku, D. G. J., Perera, S., Osei-Kyei, R., and Rashidi, M. (2021). Digital twin application in the construction industry: A literature review. J. Build. Eng. 40, 102726. doi:10.1016/j.jobe.2021.102726
Ortiz, J., Zhang, G., and McAdams, D. A. (2018). A model for the design of a pomelo peel bioinspired foam. J. Mech. Des. 140 (11), 114501. doi:10.1115/1.4040911
Ortiz, O., Castells, F., and Sonnemann, G. (2009). Sustainability in the construction industry: A review of recent developments based on LCA. Constr. Build. Mater. 23 (1), 28–39. doi:10.1016/j.conbuildmat.2007.11.012
Pankratova, G., Pankratov, D., Milton, R. D., Minteer, S. D., and Gorton, L. (2019). Following nature: Bioinspired mediation strategy for gram-positive bacterial cells. Adv. Energy Mater. 9 (16), 1900215. doi:10.1002/aenm.201900215
Perera, S., Nanayakkara, S., Rodrigo, M. N. N., Senaratne, S., and Weinand, R. (2020). Blockchain technology: Is it hype or real in the construction industry? J. Industrial Inf. Integration 17, 100125. doi:10.1016/j.jii.2020.100125
Pero, M., Moretto, A., Bottani, E., and Bigliardi, B. (2017). Environmental collaboration for sustainability in the construction industry: An exploratory study in Italy. Sustainability 9 (1), 125. doi:10.3390/su9010125
Pham, L., Lu, G., and Tran, P. (2022). Influences of printing pattern on mechanical performance of three-dimensional-printed fiber-reinforced concrete. 3D Print. Addit. Manuf. 9 (1), 46–63. doi:10.1089/3dp.2020.0172
Raftery, J., Pasadilla, B., Chiang, Y. H., Hui, E. C., and Tang, B. S. (1998). Globalization and construction industry development: Implications of recent developments in the construction sector in asia. Constr. Manag. Econ. 16 (6), 729–737. doi:10.1080/014461998372024
Regona, M., Yigitcanlar, T., Xia, B., and Li, R. Y. M. (2022). Opportunities and adoption challenges of AI in the construction industry: A PRISMA review. J. Open Innovation Technol. Mark. Complex. 8 (1), 45. doi:10.3390/joitmc8010045
Snyder, H. (2019). Literature review as a research methodology: An overview and guidelines. J. Bus. Res. 104, 333–339. doi:10.1016/j.jbusres.2019.07.039
Stachew, E., Houette, T., and Gruber, P. (2021). Root systems research for bioinspired resilient design: A concept framework for foundation and coastal engineering. Front. Robotics AI 8, 548444. doi:10.3389/frobt.2021.548444
Sto SEA (2021). StoColor Lotusan®. Avaliable At: https://www.sto-sea.com/en/about-sto/sto-innovations/stocolor-lotusan-/sto-color-lotusan.html (Accessed September 19, 2022).
Tucker Harvey, S., Khovanov, I. A., and Denissenko, P. (2019). A galloping energy harvester with flow attachment. Appl. Phys. Lett. 114 (10), 104103. doi:10.1063/1.5083103
Wurz, A. I., Schulz, A. M., O’Bryant, C. T., Sharp, J. F., and Hughes, R. M. (2022). Cytoskeletal dysregulation and neurodegenerative disease: Formation, monitoring, and inhibition of cofilin-actin rods. Front. Cell. Neurosci. 16, 982074. doi:10.3389/fncel.2022.982074
Yan, H., Yang, N., Peng, Y., and Ren, Y. (2020). Data mining in the construction industry: Present status, opportunities, and future trends. Automation Constr. 119, 103331. doi:10.1016/j.autcon.2020.103331
Yan, X., Bethers, B., Chen, H., Xiao, S., Lin, S., Tran, B., et al. (2021). Recent advancements in biomimetic 3D printing materials with enhanced mechanical properties. Front. Mater. 8, 518886. doi:10.3389/fmats.2021.518886
Yip Robin, C. P., and Poon, C. S. (2009). Cultural shift towards sustainability in the construction industry of Hong Kong. J. Environ. Manag. 90 (11), 3616–3628. doi:10.1016/j.jenvman.2009.06.017
Youmatter (2020). Ecosystem: Definition, examples, importance – all about ecosystems. Avaliable At: https://youmatter.world/en/definition/ecosystem-definition-example/(Accessed October 1, 2022).
Zabihi, H., Habib, F., and Mirsaeedie, L. (2012). Sustainability in building and construction: Revising definitions and concepts. Int. J. Emerg. Sci. 2 (4), 570–578.
Zang, H., Zhang, S., and Hapeshi, K. (2010). A review of nature-inspired algorithms. J. Bionic Eng. 7 (4), S232–S237. doi:10.1016/s1672-6529(09)60240-7
Zang, S., Wu, C., Liu, H., and Na, X. (2011). Impact of urbanization on natural ecosystem service values: A comparative study. Environ. Monit. Assess. 179 (1), 575–588. doi:10.1007/s10661-010-1764-1
Zhang, X., Wang, Y., Gu, M., Wang, M., Zhang, Z., Pan, W., et al. (2020). Molecular engineering of dispersed nickel phthalocyanines on carbon nanotubes for selective CO2 reduction. Nat. Energy 5 (9), 684–692. doi:10.1038/s4156-020-0667-9
Keywords: biomimicry, biomimicry thinking, built environment, climate change, nature, sustainable construction, sustainability
Citation: Oguntona OA and Aigbavboa CO (2023) Nature inspiration, imitation, and emulation: Biomimicry thinking path to sustainability in the construction industry. Front. Built Environ. 9:1085979. doi: 10.3389/fbuil.2023.1085979
Received: 31 October 2022; Accepted: 13 January 2023;
Published: 26 January 2023.
Edited by:
Izuru Takewaki, Kyoto University, JapanReviewed by:
Saeed Reza Mohandes, The University of Manchester, United KingdomYunpeng Zhu, Queen Mary University of London, United Kingdom
Copyright © 2023 Oguntona and Aigbavboa. This is an open-access article distributed under the terms of the Creative Commons Attribution License (CC BY). The use, distribution or reproduction in other forums is permitted, provided the original author(s) and the copyright owner(s) are credited and that the original publication in this journal is cited, in accordance with accepted academic practice. No use, distribution or reproduction is permitted which does not comply with these terms.
*Correspondence: Olusegun Aanuoluwapo Oguntona, YXJjaGl0ZWN0b2d1bnRvbmExMkBnbWFpbC5jb20=, b29ndW50b25hQHdzdS5hYy56YQ==