- Institute of Energy Engineering and Chemical Machinery, Faculty of Mechanical Engineering and Informatics, University of Miskolc, Miskolc, Hungary
Municipal solid waste management systems use several techniques for municipal solid waste at the end-of-life stage. However, to take the major differences to identify good waste management practices and the optimal actions, more complex comparisons need to be discussed. This perspective article discusses the advances and future directions of the given specific research area from the viewpoint of the author with complex review of professional literature and presentation of other authors’ work. This research work assesses and compares the environmental impacts of two end-of-life scenarios (landfilling and conventional incineration) in the European Union including the practical life cycle assessment. To find the research answers, eight main environmental impact categories, emissions, and primary energies were analyzed using the GaBi 8.0 software. Based on the results, it can be concluded that in the case of incineration, the emissions and the electricity power credit are higher. These research results can be used to compare waste treatment processes with lower environmental impacts, and to perform further research on these processes.
Introduction
The current situation of waste management worldwide is characterized by a sustainability transformation influenced by the circular economy (CE). Sustainable waste management has come to represent a main topical issue to be addressed to achieve the environmentally friendly implementation of different waste management techniques. One of the significant challenges to waste management (WM) is the municipal solid waste (MSW), whose volume has been increasing due to rapid development and industrialization. The global annual production of MSW is expected to grow to 3.4 billion tons by 2050 (Kaza et al., 2018; Xu et al., 2022). In accordance with integrated waste management, the principle of reduction aims to minimize waste production, however, the principle of waste prevention has not become an organic attitude among economic operators (Ghisellini et al., 2016; Geueke et al., 2018). Many research studies (Stahel, 2016; Grosso et al., 2017; Andersen et al., 2022; Avató and Mannheim, 2022) suppose that the CE supports the reduction of the production of various wastes by taking advantage of the opportunities provided by the Life Cycle Assessment (LCA). The concept of LCA was born in the 1960s, when environmental degradation and narrow access to resources began to cause concern (Bjørn et al., 2018). LCA tools should point to technical feasibility that produces economic and energetic benefits with lower environmental burdens. The whole life cycle of a product can be divided into three lifecycle phases (production, use/consumption, and end-of-life) and many environmental indicators must be recognized. The idea of applying a complex life cycle model for solid municipal waste fractions was already posited by Civancik-Uslu et al. (2018) and Civancik-Uslu et al. (2019). The results of Taşkın and Demir. (2020) provide low emission/low energy models for WM systems. The European Green Deal (EGD) and the Sustainable Development Goals (SDGs) require a more holistic approach to production processes (Guerrero et al., 2013; Hoang and Fogarassy, 2020). Consequently, sustainable decision-making models should combine LCA results with the technological, energetic, and economic data (Giannetti et al., 2013; Brancoli and Bolton, 2019).
Instead of waste reduction, the proper selection of WM procedures at the end of waste’s life cycle was emphasized. More and more research works are available (Finnveden et al., 2009; Evangelisti et al., 2015; Baldowska-Witos et al., 2019; Demetrious and Crossin, 2019; Mannheim, 2021; Ouedraogo et al., 2021; Avató and Mannheim, 2022; Lara-Topete et al., 2022) for the evaluation of EoL scenarios based on the LCA between the possible waste treatments contrasting the impacts of different methods. Landfilling and incineration are the most common treatment methods for MSW (Voběrková et al., 2017; Koda et al., 2017; Asase et al., 2019; Mehr et al., 2021). However, landfilling is the third largest source of human methane emissions. According to Genovesi at al. (2022), the overall impact of fossil-based systems is closely related to landfill in the categories of water with human toxicity and water with chronic ecotoxicity. Incineration has the advantage of being located close to MSW generation sources reducing transportation costs. The improvements can be achieved in thermic treatment processes if we consider other scenarios of the investigated system, such as energy recovery. Waste-to-Energy (WtE) technologies may facilitate the use of new waste flows (Lausselet et al., 2016; Okati et al., 2022) for energy purposes. Several research studies (Panepinto et al., 2015; Indrawan et al., 2018; Oliveira et al., 2022) suggest alternative thermic treatment techniques, such as gasification or plasma-based technology. Voss et al. (2021) and Keller et al. (2022) introduce an integrated life cycle model that compares pyrolysis and gasification for MSW, finding that gasification shows greater emission reductions than pyrolysis. My previous LCA results (Mannheim and Siménfalvi, 2012; Mannheim, 2014) showed that gasification and plasma-based technology have a better environmental performance in terms of all examined impact categories compared to incineration. Compared to traditional thermal technologies, electricity can be produced more efficiently by plasma gasification where no dioxins and furans are produced (Mohai and Szépvölgyi, 2005; Oliveira et al., 2022). In recent times, also anaerobic digestion became the center of attention. In Canada, Norouzi and Dutta. (2022) analyzed several anaerobic digestion apparatuses. Several studies (Li and Feng, 2022; Morsink-Georgali et al., 2022) investigated the impact of sludge through LCA and highlighted the environmentally friendly nature of the anaerobic digestion process.
To realize waste handling process optimization, LCA can be used effectively to investigate the environmental loads of end-of-life stages (Guerrero et al., 2013; Di Maria et al., 2018). If system boundaries are well-defined, we can apply Life Cycle Inventory (LCI) and life cycle impact assessment (LCIA) for EoL stage with a suitable LCA software. The variety of developed software programs keeps the chance to devise environmental load decrease solutions at the end-of-life cycle stage (Alwaeli, 2016). Many research studies (Guinée et al., 2002; Steve, 2015; Lettner et al., 2018) have defined sustainable decision-making models, focusing on end-of-life waste management technologies. The most important viewpoint is that the applied tools provide environmental, economic, and energetic indicators for decision-makers at the same time. That is, the applied aspect for optimal decision-making is a holistic approach, which includes the product and technological procedure lifecycles and removes the possibility of changing practices that have a direct effect on the environment between different phases of the WMS. In recent years, the integration of different accounting methods has also come to the fore (Arbault et al., 2014; Kharrazi et al., 2014). Kharazi et al. (2014) integrated emergy, exergy, and ecological footprint. The integration between LCA and different information-based approaches, such as exergy analysis (ExA), emergy accounting (EmA), and BIM-based environmental and economic LCA tools has also come to the fore (Santos et al., 2019, 2020).
This work compared two treatment processes (landfilling and incineration) and examined environmental loads of the MSW product in the EU. Life cycle models are completed with leachate, sludge treatments, and landfill gas utilization. The functional unit was defined as the distribution of 1 kg of municipal solid waste. In addition to the analyses and evaluations of the results, this study presents a review of the application of life cycle assessment in waste management with the help of the reviewed professional literature.
Methodology
Life cycle assessment and life cycle inventory methodology
Life cycle assessment method evaluates the environmental loads associated with the waste product. It is standardized by ISO 14040 and ISO 14044 (International Organisation for Standardization, 2006). In recent years, the growing importance of environmental and waste management has enlarged interest in the LCA. Researchers worldwide have been expanding this methodology because of rising demand for more environmental information (Borodin et al., 2015; Curran, 2016). The applied methodology includes life cycle inventory, life cycle impact assessment (LCIA) by applying GaBi 8.0 software with the database of 2020, and interpretation of the results. The consistent LCI includes and quantifies input-output material flows and energy supplies for all examined processes. In the modelling of waste treatment systems, we used product-specific input information. The input wastes are disposed of as municipal solid waste and the output energy is recovered. The LCI method allocates energy requirements and environmental emissions to treated waste products with the allocation of mass. In addition to the main waste product, the landfilling process produces recycled sludge and wastewater. Sewage sludge is treated, and the wastewater is managed in wastewater treatment plant.
System boundaries, functional unit, and allocation
The system boundaries are developed gate-to-grave and included a dataset of unit single operation processes. The examined life cycle stage starts after the use stage of the product, depending on the choice of the product’s end-of-life scenario. The elementary and system flows to the treatment sites are allocated to the elementary content in the waste input. Included within the system environment, energy supply, and waste treatment are depending on system boundaries. Auxiliary systems such as produced electric power, the landfill gas utilization, the leachate treatment, and the sewage sludge treatment processes were calculated. The equipment, machines, and trucks have gone beyond the boundaries of the system. The energy amount for heating, cooling, and lighting was not included in the system boundaries. The dataset did not include incoming transports. The functional unit (FU) was described as the distribution of 1 kg of municipal solid waste (average composition from EU in 2020). For the power production, allocation by energetic content was applied.
Life cycle impact assessment method
With the help of applied CML 2016 (Centrum voor Milieukunde Leiden) method, resources, emissions, and impact categories for EoL processes in terms of the functional unit were estimated (Kupfer et al., 2021). Eight main environmental impact potentials—photochemical ozone creation (POCP), marine and terrestrial ecotoxicity (MAETP, TETP), human toxicity (HTP), global warming (GWP), eutrophication (EP), acidification (AP), and abiotic depletion for fossils (ADPF)—were calculated. The normalization and weighting methods were the same for both end-of-life models.
Results
This work enables primary energy, resources, and environmental impacts associated with the EoL scenarios of MSW. Table 1 summarizes the calculated values for the treatment scenarios in the EU and presents that the emissions to air and freshwater, and the material resources are much higher for incineration. Figure 1 describes the examined impacts in nanograms for Scenario 1. Figure 2 shows the environmental categories in percent for Scenario 2. Figure 3 presents the main calculated impact categories in nanograms for the two examined end-of-life scenarios.
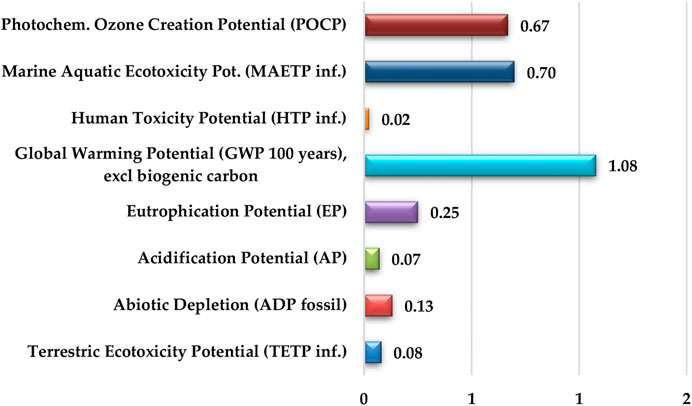
FIGURE 1. Normalized and weighted values of the environmental impact categories for landfill in nanograms. Functional unit: 1 kg of municipal solid waste, EU-28. Normalization method: CML 2016, EU 25 + 3, year 2000, excl. biogenic carbon. Weighting method: thinkstep LCIA Survey 2012, Europe, CML 2016, excl. biogenic carbon. (Source: own analyzes with GaBi 8.0 software).
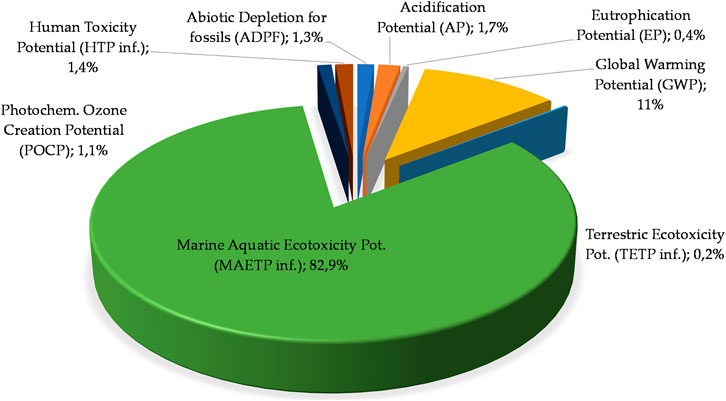
FIGURE 2. Percentage distribution of impact categories for incineration in percent (functional unit: MSW of 1 kg). Normalisation reference: CML 2016, EU 25 + 3, year 2000, excl. biogenic carbon. Weighting method: thinkstep LCIA Survey 2012, Europe, CML 2016, excl. biogenic carbon). In Scenario 1, 0.476 MJ of waste heat, 0.016 MJ of unused primary energy, 0.081 kg of used air, 0.248 kg of processed water to groundwater, 0.005 kg of cooling water to a river, 0.728 kg of collected rainwater, 0.011 kg of turbid water to a river, 0.216 kg of carbon dioxide to the air, and 0.297 MJ of electric power (electricity from landfill gas utilization) were generated from 1 kg of MSW. In Scenario 2, 1.23 MJ of electric power was generated, and energy feedback can be reused in a specifically designed plant. (Source: own analyzes with GaBi 8.0 software).
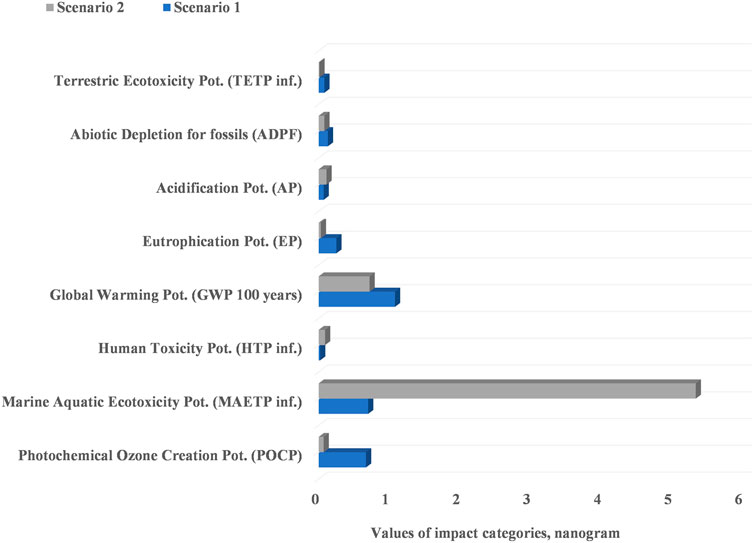
FIGURE 3. Main environmental impact category values for the two examined end-of-life scenarios in nanograms. (Source: own analyzes with GaBi 8.0 software).
Discussion
Europe is trying to achieve climate neutrality by 2050 in the context of the EGD, SDGs and Circular Economy Strategy (Kaczmarczyk and Urych, 2022). For this purpose, avoiding municipal solid waste is an important factor, and for sustainability, it is essential to evaluate the EoL stage. Regarding this, values of environmental impacts and primary energy were examined to identify which EoL scenario is more optimal.
According to my results, the values of emissions to air and freshwater are higher in the case of incineration. Values of POCP, ADPF, EP, GWP, and TETP are higher for the landfilling, however, the total load of incineration is twice that of landfilling. Marine ecotoxicity of incineration (82,9%) is remarkably high compared to landfilling. LCA studies mentioned in the introduction similarly concluded that landfilling had a lower impact on the environment than incineration. However, in the case of incineration, the value of the electricity power credit is five times higher, which means that we can now talk about a treatment method, that is, associated with energy recovery and energetic utilization.
This developed LCA model can be integrated with additional environmental factors. The integrated studies for MSW management with LCA (Lara-Topete et al., 2022), and the integration of Life Cycle Sustainability Assessment (LCSA) with environmental risk assessment are increasingly used (Walker et al., 2021; De Luca Peña et al., 2021; Hackenhaar et al., 2022) for decision-making related to the efficient use of natural resources. The combination of LCA and CE can be combined with economic indicators. Loizia et al. (2021) established factors to evaluate the environmental performance, which cover the waste compositional, SWOT and PESTEL analysis. Rimano et al. (2021) illustrated methodological choices commonly encountered in an Organizational Life Cycle Consumption Assessment. The increasing energy costs require more detailed and systematic life cycle decision-making (Sanyé-Mengual and Sala, 2022).
Conclusion
This perspective presents and compares the environmental impacts of two EoL scenarios by gate-to-grave LCA in the EU. The functional unit was defined as the distribution of 1 kg of MSW. In addition to the analyses and evaluations of the results, this study presents a review of the application of life cycle assessment in waste management with the help of the reviewed professional literature.
To improve the results of conclusions supporting decision-making, uncertainty analysis of technological characteristics and measuring of long-term effects can serve as an advantage. To assess the uncertainty two approaches can be taken with the help of LCA: sensitivity analysis on hotspots or Monte Carlo simulation. By measuring long-term effects, the resources and emissions can show us what are the weak points and possibilities in waste management.
These results consequence from LCA can be used to compare the environmental impacts of different waste treatment technologies and help to focus efforts on making environmental improvements to the treatment enterprises.
Data availability statement
The raw data supporting the conclusion of this article will be made available by the author, without undue reservation.
Author contributions
All authors listed have made a substantial, direct, and intellectual contribution to the work and approved it for publication.
Funding
The research was conducted at the University of Miskolc as part of the “Developments aimed at increasing social benefits deriving from more efficient exploitation and utilization of domestic subsurface natural resources” project supported by the Ministry of Innovation and Technology from the National Research, Development and Innovation Fund according to the Grant Contract issued by the National Research, Development and Innovation Office (Grant Contract reg. no. TKP-17-1/PALY-2020).
Conflict of interest
The author declares that the research was conducted in the absence of any commercial or financial relationships that could be construed as a potential conflict of interest.
Publisher’s note
All claims expressed in this article are solely those of the authors and do not necessarily represent those of their affiliated organizations, or those of the publisher, the editors and the reviewers. Any product that may be evaluated in this article, or claim that may be made by its manufacturer, is not guaranteed or endorsed by the publisher.
Abbreviations
ADPF, abiotic depletion potential for fossils; AP, acidification potential; CE, circular economy; CES, circular economy strategy; EGD, European Green Deal; EoL, end-of-life; EP, eutrophication potential; EU, European Union; FU, functional unit; GWP, global warming potential; HTP, human toxicity potential; LCA, life cycle assessment; LCI, life cycle inventory; LCIA, life cycle impact assessment; MAETP, marine aquatic ecotoxicity potential; MSW, municipal solid waste; POCP, photochemical ozone creation potential; SDGs, sustainable development goals; TETP, terrestric ecotoxicity potential; WM, waste management.
References
Alwaeli, M. (2016). End-of-life vehicles recovery and recycling and the route to comply with eu directive targets. Environ. Prot. Eng. 42, 191–202. doi:10.37190/epe160114
Andersen, S. C., Birgisdottir, H., and Birkved, M. (2022). Life cycle assessments of circular economy in the Built environment—a scoping review. Sustainability 14, 6887. doi:10.3390/su14116887
Arbault, D., Rugani, B., Barna, L., and Benetto, E. (2014). A semantic study of the emergy sustainability index in the hybrid lifecycle-emergy framework. Ecol. Indic. 43, 252–261. doi:10.1016/j.ecolind.2014.02.029
Asase, M., Yanful, E. K., Mensah, M., Stanford, J., and Amponsah, S. (2019). Comparison of municipal solid waste management systems in Canada and Ghana: A case study of the cities of london, ontario, and kumasi, Ghana. Waste Manag. 29, 2779–2786. doi:10.1016/j.wasman.2009.06.019
Avató, J. L., and Mannheim, V. (2022). Life cycle assessment model of a catering product: Comparing environmental impacts for different end-of-life scenarios. Energies 15, 5423. doi:10.3390/en15155423
Baldowska-Witos, P., Kruszelnicka, W., Kasner, R., Tomporowski, A., Flizikowski, J., and Mrozinski, A. (2019). Impact of the plastic bottle production on the natural environment. Part 2. Analysis of data uncertainty in the assessment of the life cycle of plastic beverage bottles using the Monte Carlo technique. Przem. Chem. 98, 1668–1672. doi:10.15199/62.2019.10.28
Bjørn, A., Owsianiak, M., Molin, C., and Laurent, A. (2018). Main characteristics of LCA. Life Cycle Assess. 9, 16. doi:10.1007/978-3-319-56475-3_2
Borodin, Y. V., Aliferova, T. E., and Ncube, A. (2015). Waste management through life cycle assessment of products. IOP Conf. Ser. Mat. Sci. Eng. 81, 012085. doi:10.1088/1757-899X/81/1/012085
Brancoli, P., and Bolton, K. (2019). Life cycle assessment of waste management systems. Sustain. Resour. Recovery Zero Waste Approaches 23, 33. doi:10.1016/B978-0-444-64200-4.00002-5
Civancik-Uslu, D., Ferrer, L., Puig, R., and Fullana-i-Palmer, P. (2018). Are functional fillers improving environmental behavior of plastics? A review on LCA studies. Sci. Total Environ. 626, 927–940. doi:10.1016/j.scitotenv.2018.01.149
Civancik-Uslu, D., Puig, R., Ferrer, L., and Fullana-i-Palmer, P. (2019). Influence of end-of-life allocation, credits and other methodological issues in LCA of compounds: An in-company circular economy case study on packaging. J. Clean. Prod. 212, 925–940. doi:10.1016/j.jclepro.2018.12.076
Curran, M. (2016). “Life Cycle Assessment,” in Kirk-Othmer Encyclopedia of Chemical Technology. Editor A. Seidel (Wiley), 359–366. doi:10.1016/B978-0-12-409548-9.09700-1
De Luca Peña, L. V., Taelman, S. E., Préat, N., Boone, L., Van der Biest, K., Custódio, M., et al. (2022). Towards a comprehensive sustainability methodology to assess anthropogenic impacts on ecosystems: Review of the integration of life cycle assessment, environmental risk assessment and ecosystem services assessment. Sci. Total Environ. 808, 152125. doi:10.1016/j.scitotenv.2021.152125
Demetrious, A., and Crossin, E. (2019). Life cycle assessment of paper and plastic packaging waste in landfill, incineration, and gasification-pyrolysis. J. Mat. Cycles Waste Manag. 21, 850–860. doi:10.1007/s10163-019-00842-4
Di Maria, F., Lovat, E., and Caniato, M. (2018). Comparing waste management in developed and developing countries: The case study of Umbria region (Italy) and of west bank (Palestine). Detritus 3, 171–180. doi:10.31025/2611-4135/2018.13690
Evangelisti, S., Tagliaferri, C., Clift, R., Lettieri, P., Taylor, R., and Chapman, C. (2015). Life cycle assessment of conventional and two-stage advanced energy-from-waste technologies for municipal solid waste treatment. J. Clean. Prod. 100, 212–223. doi:10.1016/j.jclepro.2015.03.062
Finnveden, G., Hauschild, M. Z., Ekvall, T., Guinée, J., Heijungs, R., Hellweg, S., et al. (2009). Recent developments in life cycle assessment. J. Environ. Manage. 91, 1–21. doi:10.1016/j.jenvman.2009.06.018
Genovesi, A., Aversa, C., Barletta, M., Cappiello, G., and Gisario, A. (2022). Comparative life cycle analysis of disposable and reusable tableware: The role of bioplastics. Clean. Eng. Technol. 6 (2022), 100419. doi:10.1016/j.clet.2022.100419
Geueke, B., Groh, K., and Muncke, J. (2018). Food packaging in the circular economy: Overview of chemical safety aspects for commonly used materials. J. Clean. Prod. 193, 491–505. doi:10.1016/j.jclepro.2018.05.005
Ghisellini, P., Cialani, C., and Ulgiati, S. (2016). A review on circular economy: The expected transition to a balanced interplay of environmental and economic systems. J. Clean. Prod. 114, 11–32. doi:10.1016/j.jclepro.2015.09.007
Giannetti, B. F., Bonilla, S. H., and Almeida, C. M. V. B. (2013). An emergy-based evaluation of a reverse logistics network for steel recycling. J. Clean. Prod. 46, 48–57. doi:10.1016/j.jclepro.2012.05.024
Grosso, M., Niero, M., and Rigamonti, L. (2017). Circular economy, permanent materials and limitations to recycling: Where do we stand and what is the way forward? Waste Manag. Res. 35 (8), 793–794. doi:10.1177/0734242x17724652
Guerrero, L. A., Maas, G., and Hogland, W. (2013). Solid waste management challenges for cities in developing countries. Waste Manag. 33, 220–232. doi:10.1016/j.wasman.2012.09.008
Guinée, J. B., Gorrée, M., Heijungs, R., Huppes, G., Kleijn, R., de Koning, A., et al. (2002). Handbook on life cycle assessment: Operational guide to the ISO standards’, series: Eco-efficiency in industry and science. Dordrecht, The Netherlands: Kluwer Academic Publisher.
Hackenhaar, I., Alvarenga, R. A. F., Bachmann, T. M., Riva, F., Horn, R., Graf, R., et al. (2022). A critical review of criticality methods for a European Life Cycle Sustainability Assessment. Procedia CIRP 105, 428–433. doi:10.1016/j.procir.2022.02.071
Hoang, N. H., and Fogarassy, C. S. (2020). Sustainability evaluation of municipal solid waste management system for hanoi (vietnam) - why to choose the ‘waste-to-energy’ concept. Sustainability 12, 1085. doi:10.3390/su12031085
Indrawan, N., Thapa, S., Bhoi, P. R., Huhnke, R. L., and Kumar, A. (2018). Electricity power generation from co-gasification of municipal solid wastes and biomass: Generation and emission performance. Energy 162, 764–775. doi:10.1016/j.energy.2018.07.169
International Organisation for Standardization (2006). ISO 14040:2006, environmental management—life cycle assessment—principles and framework. Geneva, Switzerland: ISO. Available At: https://www.iso.org/standard/37456.html (accessed on June 6, 2019).
Kaczmarczyk, B., and Urych, I. (2022). Perception of the transition to a zero-emission economy in the opinion of polish students. Energies 15, 1102. doi:10.3390/en15031102
Kaza, S., Yao, L., Bhada-Tata, P., and Van Woerden, F. (2018). “What a waste 2.0: A global snapshot of solid waste management to 2050,” in Urban development series (Washington, DC: Word Bank). doi:10.1596/978-14648-1329-0
Keller, F., Voss, R. L., Lee, R. P., and Meyer, B. (2022). Life cycle assessment of global warming potential of feedstock recycling technologies: Case study of waste gasification and pyrolysis in an integrated inventory model for waste treatment and chemical production in Germany. Resour. Conservation Recycl. 179 (2022), 106106. doi:10.1016/j.resconrec.2021.106106
Kharrazi, A., Kraines, S. B., Hoang, L., and Yarime, M. (2014). Advancing quantification methods of sustainability: A critical examination emergy, exergy, ecological footprint, and ecological information-based approaches. Ecol. Indic. 37, 81–89. doi:10.1016/j.ecolind.2013.10.003
Koda, E., Miszkowska, A., and Sieczka, A. (2017). Levels of organic pollution indicators in groundwater at the old landfill and waste management site. Appl. Sci. 7, 638. doi:10.3390/app7060638
Kupfer, T., Baitz, M., Colodel, C. M., Kokborg, M., Schöll, S., Rudolf, M., et al. (2021). “GaBi databases & modeling principles,” in Sphera solutions GmbH (Chicago, IL, USA: Sphera). Available at: https://sphera.com/wp-content/uploads/2020/04/Modeling-Principles-GaBi-Databases-2021.pdf.
Lara-Topete, G. O., Yebra-Montes, C., Orozco-Nunnelly, D. A., Robles-Rodríguez, C. E., and Gradilla-Hernández, M. S. (2022). An integrated environmental assessment of MSW management in a large city of a developing country: Taking the first steps towards a circular economy model. Front. Environ. Sci. 10, 838542. doi:10.3389/fenvs.2022.838542
Lausselet, C., Cherubini, F., del Alamo Serrano, G., Becidan, M., and Strømman, A. H. (2016). Life-cycle assessment of a Waste-to-Energy plant in central Norway: Current situation and effects of changes in waste fraction composition. Waste Manag. 58, 191–201. doi:10.1016/j.wasman.2016.09.014
Lettner, M., Solt, P., Rößiger, B., Pufky-Heinrich, D., Jääskeläinen, A.-S., Schwarzbauer, P., et al. (2018). From wood to resin—identifying sustainability levers through hotspotting lignin valorisation pathways. Sustainability 10, 2745. doi:10.3390/su10082745
Li, H., and Feng, K. (2022). Life cycle assessment of the environmental impacts and energy efficiency of an integration of sludge anaerobic digestion and pyrolysis. J. Clean. Prod. 195, 476–485. doi:10.1016/j.jclepro.2018.05.259
Loizia, P., Voukkali, I., Zorpas, A. A., Pedreño, J. N., Chatziparaskeva, G., Inglezakis, V. J., et al. (2021). Measuring the level of environmental performance in insular areas, through key performed indicators, in the framework of waste strategy development. Sci. Total Environ. 753, 141974. doi:10.1016/j.scitotenv.2020.141974
Mannheim, V. (2014). Examination of thermic treatment and biogas processes by LCA. Ann. Fac. Eng. Hunedoara Int. J. Eng. 12, 225–234. Available at: https://annals.fih.upt.ro/pdf-full/2014/ANNALS-2014-2-37.pdf.
Mannheim, V. (2021). Life cycle assessment model of plastic products: Comparing environmental impacts for different scenarios in the production stage. Polymers 13 (5), 777. doi:10.3390/polym13050777
Mannheim, V., and Siménfalvi, Z. (2012). “Determining A priority order between thermic utilization processes for organic industrial waste with LCA,” in Waste management and the environment VI (Southampton, UK: WIT Press), 153–166. doi:10.2495/WM120151
Mehr, J., Haupt, M., Skutan, S., Morf, L., Adrianto, L. R., Weibel, G., et al. (2021). The environmental performance of enhanced metal recovery from dry municipal solid waste incineration bottom ash. Waste Manage. 119, 330–341. doi:10.1016/j.wasman.2020.09.001
Mohai, I., and Szépvölgyi, J. (2005). Treatment of particulate metallurgical wastes in thermal plasmas. Chem. Eng. Process. Process Intensif. 44 (2), 225–229. doi:10.1016/j.cep.2004.04.008
Morsink-Georgali, P.-Z., Kylili, A., Fokaides, P. A., and Papadopoulos, A. M. (2022). Compost versus biogas treatment of sewage sludge dilemma assessment using life cycle analysis. J. Clean. Prod. 350, 131490. doi:10.1016/j.jclepro.2022.131490
Norouzi, O., and Dutta, A. (2022). The current status and future potential of biogas production from Canada’s organic fraction municipal solid waste. Energies 15, 475. doi:10.3390/en15020475
Okati, A., Reza Khani, M., Shokri, B., Rouboa, A., and Monteiro, E. (2022). Optimizing the operating conditions for hydrogen-rich syngas production in a plasma co-gasification process of municipal solid waste and coal using Aspen Plus. Int. J. Hydrogen Energy 47, 26891–26900. doi:10.1016/j.ijhydene.2022.06.058
Oliveira, M., Ramos, A., Ismail, T. M., Monteiro, E., and Rouboa, A. (2022). A review on plasma gasification of solid residues: Recent advances and developments. Energies 15 (4), 1475. doi:10.3390/en15041475
Ouedraogo, A. S., Frazier, R. S., and Kumar, A. (2021). Comparative life cycle assessment of gasification and landfilling for disposal of municipal solid wastes. Energies 14 (21), 7032. doi:10.3390/en14217032
Panepinto, D., Tedesco, V., Brizio, E., and Genon, G. (2015). Environmental performances and energy efficiency for MSW gasification treatment. Waste Biomass Valorization 6, 123–135. doi:10.1007/s12649-014-9322-7
Rimano, M., Simboli, A., Taddeo, R., Del Grosso, M., and Raggi, A. (2021). The environmental impact of organizations: A pilot test from the packaging industry based on organizational life cycle assessment. Sustainability 13 (20), 11402. doi:10.3390/su132011402
Santos, R., Aguiar Costa, A., Silvestre, J. D., and Pyl, L. (2020). Development of a BIM-based environmental and economic life cycle assessment tool. J. Clean. Prod. 265, 121705. doi:10.1016/j.jclepro.2020.121705
Santos, R., Aguiar Costa, A., Silvestre, J. D., and Pyl, L. (2019). Integration of LCA and LCC analysis within a BIM-based environment. Automation Constr. 103, 127–149. doi:10.1016/j.autcon.2019.02.011
Sanyé-Mengual, E., and Sala, S. (2022). Life cycle assessment support to environmental ambitions of EU policies and the sustainable development Goals. Integr. Environ. Assess. Manag. 00, 1–12. doi:10.1002/ieam.4586
Steve, S. (2015). Reducing energy demand: A review of issues, challenges and approaches. Renew. Sustain. Energy Rev. 47, 74–82. doi:10.1016/j.rser.2015.03.002
Taşkın, A., and Demir, N. (2020). Life cycle environmental and energy impact assessment of sustainable urban municipal solid waste collection and transportation strategies. Sustain. Cities Soc. 61, 102339. doi:10.1016/j.scs.2020.102339
Voběrková, S., Vaverková, M. D., Burešová, A., Adamcová, D., Vršanská, M., Kynický, J., et al. (2017). Effect of inoculation with white-rot fungi and fungal consortium on the composting efficiency of municipal solid waste. Waste Manag. 61, 157–164. doi:10.1016/j.wasman.2016.12.039
Voss, R., Lee, R. P., Seidl, L., Keller, F., and Fröhling, M. (2021). Global warming potential and economic performance of gasification-based chemical recycling and incineration pathways for residual municipal solid waste treatment in Germany. Waste Manag. 134, 206–219. doi:10.1016/j.wasman.2021.07.040
Walker, T. R., McGuinty, E., and Hickman, D. (2021). Marine debris database development using international best practices: A case study in Vietnam. Mar. Pollut. Bull. 173 Part A, 112948. doi:10.1016/j.marpolbul.2021.112948
Keywords: municipal solid waste, life cycle assessment, end-of-life stage, environmental impacts, incineration, landfill, circular economy
Citation: Mannheim V (2022) Perspective: Comparison of end-of-life scenarios of municipal solid waste from viewpoint of life cycle assessment. Front. Built Environ. 8:991589. doi: 10.3389/fbuil.2022.991589
Received: 11 July 2022; Accepted: 22 August 2022;
Published: 14 September 2022.
Edited by:
Sudhakar Babu Thanikanti, Chaitanya Bharathi Institute of Technology, IndiaReviewed by:
Natalia Shushunova, Moscow Institute of Civil Engineering, RussiaCopyright © 2022 Mannheim. This is an open-access article distributed under the terms of the Creative Commons Attribution License (CC BY). The use, distribution or reproduction in other forums is permitted, provided the original author(s) and the copyright owner(s) are credited and that the original publication in this journal is cited, in accordance with accepted academic practice. No use, distribution or reproduction is permitted which does not comply with these terms.
*Correspondence: Viktoria Mannheim, dmlrdG9yaWEubWFubmhlaW1AdW5pLW1pc2tvbGMuaHU=