- 1Department of Civil Engineering, Jordan University of Science and Technology, Irbid, Jordan
- 2Civil Engineering Program, Al Ain University-Abu Dhabi Campus, Abu Dhabi, United Arab Emirates
- 3Department of Civil Engineering, Prince Mohammad Bin Fahad University, Khobar, Saudi Arabia
- 4Department of Civil Engineering, Al-Zaytoonah University of Jordan, Amman, Jordan
The use of hydrophilic bentonite nanoclay to improve the geotechnical properties of expansive soils was studied. Sonic waves and manual mixing for two expansive soils (taken from two different locations in Jordan) were studied; thereafter, a comparison between the results obtained from both methods was carried out. Different percentages of nanoclay were added to the two soils: 0, 0.1, 0.3, 0.5, 0.8, 1, 1.5, and 2.5% by dry weight of soil. Their impacts on compaction, unconfined compression strength (UCS), and free swell index (FSI) were investigated. For both methods of mixing, adding nanoclay to the soils resulted in a significant enhancement in strength and a reduction in swell properties of the soils. The UCS results of sonicated samples treated with 0.5% of nanoclay addition showed an increase of 27%–57% and a reduction in FSI by 41%–46.5%. Nevertheless, the UCS of non-sonicated samples treated with 1% of nanoclay showed an increase of 19.3–28.5% and a reduction in FSI by 37.3–44.3%.
Introduction
Soil is a heterogeneous material that is extremely complex and exposed to many changes in nature. Properties of soil not only change from one place to another but also change as a function of depth, climate, and the case of drainage (Gupta and Sharma, 2017), which increase the element of uncertainty and make predicting its behavior in a highly demanding proposition, especially when dealing with expansive soils. Expansive soils are found in arid and semi-arid regions of the world where there is a change in water content (%w) over different seasons (Nelson and Miller, 1997) or where annual evaporation exceeds annual precipitation (Abduljauwad, 1993). Expansive soils are a world-wide problem for civil engineers because of their unpredictable behavior. These soils are considered as a high natural hazard, which can cause extensive damage to structures such as foundations, roads, highways, buildings, airport runways, and earth dams (Gupta and Sharma, 2017).
Expansive soil tends to expand by volume upon wetting and decrease or shrink by volume when the water evaporates. The increase and decrease in volume as a result of moisture changing cause instability of foundations that have been built on the expansive soil (Fattah et al., 2015; Irshayyid and Fattah, 2019; Sharo and Taamneh, 2020; Sharo et al., 2022).
To overcome the weakness of expansive soils, adding a foreign agent or using a mechanical stabilization method can be used to improve the properties of soil through the so-called “soil stabilization”. Soil stabilization can improve the performance of soil represented by an increase in strength, bearing capacity, and a decrease in swell potential (Fattah and Al-Lami, 2017). Expansive soils always take a big part in researchers’ minds, time, and efforts to reach to the best applicable stabilization method. However, one of the rapid, low-cost, eco-friendly, and recent techniques is using nanomaterials as soil-stabilizing agents (Kajbafvala et al., 2013), such as nanoclay, nano-CuO, nano-MgO, nano-SiO2, and others, which belong to the nanotechnology world (Ghasabkolaei et al., 2017).
The idea of nanotechnology was proposed in 1959 by Richard Feynman in his lecture titled “There’s Plenty of Room at the Bottom” (Feynman, 1960). At that time, the term “nanotechnology” has not existed yet, but along the years, it had a significant rapid spread. Each branch in science has its own definition of “nanotechnology”, but the National Nanotechnology Initiative (NNI) defined it by the control, comprehension, and reformation matters based on the hierarchy of nanometers to develop matters with new uses (NSTC, 2007). The main goal of nanotechnology in geotechnical engineering is to improve soil properties by adding very small amounts of materials at the nanoscale, which are called “nanomaterials”. These nanomaterials are known to be 100 nanometers (nm) lower in terms of their dimensions (Majeed et al., 2014). Based on this fact, the presence of these nanomaterials in the soil can influence the physical and chemical behavior significantly due to the very high specific surface area, surface charges, and their morphologic properties (Shahin et al., 2015).
Using nanomaterials in the literature as stabilizing agents for coarse-grained and fine-grained soils confirmed that using them, even at low doses, could improve the expansive soil properties. For example, colloidal silica increased the unconfined compressive strength (UCS) of the sand soil (Miwa and Yonekura, 1994) and increased the liquefaction resistance (Towhata and Kabashima, 2001; Díaz-Rodríguez and Antonio-Izarraras, 2004; and Kodaka et al., 2005). However, it decreased the hydraulic conductivity as noticed by Noll et al., 1992 and Persoff et al. (1996). Moreover, using nano-Al2O3 to stabilize clay soil increased the strength properties (Luo et al., 2012) and decreased the plasticity index and the swelling potential (Naval et al., 2017). Additionally, using nanoclay material as a stabilizing agent for clay soils increased the strength properties as noticed by Arabani et al., 2012, Majeed and Taha (2013), Nikookar et al., 2013, and Zahedi et al. (2014). It also increased plastic and liquid limit values, which reflected a decrease in the plasticity index as noticed by Bahari et al., 2013, Mohammadi and Niazian (2013), and Tabarsa and Hosseini (2015). Using nanoclay decreased the swelling properties according to the literature, and this was shown clearly by Sharo and Alawneh (2016) and Naval et al. (2017).
Bentonite is mainly composed of montmorillonite clay mineral (Al-Omari et al., 2016). Because the soil swelling is considered as a major factor to evaluate the foundation performance resting on it (Fattah and Al-Lami, 2017), several attempts aimed to stabilize bentonite were made. Fattah et al. (2021) and Fattah et al. (2022) reported that the adding of 50% of sand to bentonite leads to a reduction in the swell percent from 14 % to 2.4%.
Many studies that cover the effect of using nanoclay as a soil-stabilizing agent are available in the literature, few of which cover enhancing expansive soil properties using its cause (montmorillonite) manipulated at the nanoscale. In addition, the literature did not account for the impact of mixing procedures used in soil stabilization using nanoclay in field applications. In addition to the effect of stabilizing expansive soil using nanoclay (treatment with a cause), the advantages of using the sonic wave procedure, which is usually used to disperse the interparticles of nanomaterials, over the use of manual mixing in soil stabilization using nanoclay will also be elaborated in this study.
This study aimed to investigate the effect of adding of nanoclay bentonite powder on the engineering properties of two types of expansive soils in Jordan, Irbid, and Shmeissani soils. These soils are classified as highly expansive soils. There are several studies aimed to stabilize Irbid soil by the addition of solid and liquid chemicals like lime, cement, and cement–lime blend (Sharo et al., 2019); dehydrated calcium chloride (Sharo et al., 2018); and disposed formalin aqueous (Sharo and Taamneh, 2020). The stabilizing method presented in this paper is almost different from the previous methods by treating with a cause.
Materials and experimental program
Soil properties
Two types of soil from different locations in Jordan were collected: the eastern district of Irbid (Soil A) and Shmeissani area at the northwest area of Amman (Soil B), as indicated in Figure 1. Samples were taken from 1.5 m to 2.5 m depth from the ground surface. Disturbed soil samples were collected in bags and transported to the laboratory to be prepared to undergo laboratory testing.
Table 1 summarizes the natural soil properties, while the grain-size distribution curves for natural soils are shown in Figure 2. The clay-size fractions were 74.8% and 57.2% for Soil A and Soil B, respectively. Moreover, the collected soil samples exhibited a plasticity index of 35.2% for Soil A and 33.9% for Soil B. Referring to Figure 3, the soils are located above the A-line on the plasticity chart in the zone of inorganic clay with high-plasticity CH with a high degree of expansiveness. Based on free swell index (FSI) values, the soils are classified as “highly expansive soils” according to Chen (1975) as indicated in Table 2.
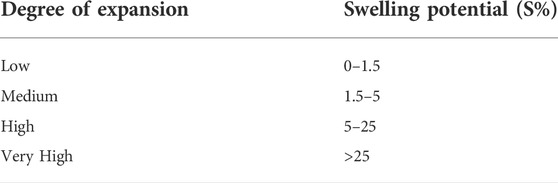
TABLE 2. Classification of expansive soils (Chen, 1975).
Additive properties
The nanoclay used in this study is a light-brown soluble hydrophilic bentonite powder, with a specific gravity of 2.6 and an average crystallite size (Dp) of 8.04 nm. It is commercially available and produced by Sigma Aldrich, Darmstadt/Germany. The fabric arrangements of nanoclay particles are shown in Figure 4. Figure 5 shows the X-Ray diffraction pattern of the additive that indicates a clear presence of the predominant mineral of montmorillonite, while Table 3 shows the X-Ray fluorescence analysis of the additive.
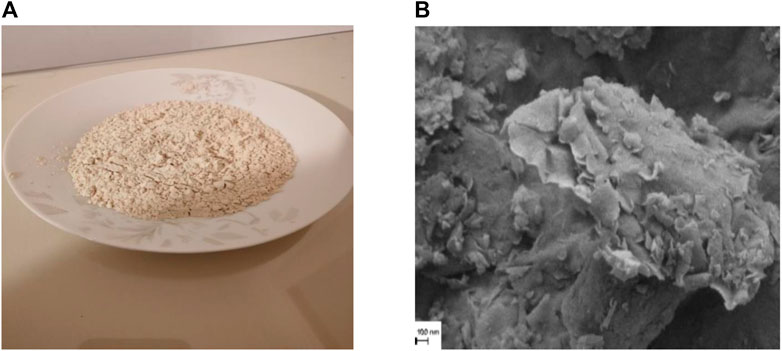
FIGURE 4. Commercial nanoclay used in this study: (A) nanoclay, hydrophilic bentonite, in the state of powder and light tan in color. (B) Scanning electron microscopy (SEM) image of nanoclay.
Experimental program
Sample preparation and mixing methods
Before applying the additive treatment to the soil, both collected soil samples were subjected to oven drying and fine crushing in preparation for their use in the experimental testing. Eight percentages of the nanoclay (0%, 0.1%, 0.3%, 0.5%, 0.8%, 1%, 1.5%, and 2.5%) by the dry weight of soil were mixed with the amount of water required to perform each test. Thereafter, the solution of water and each percentage of nanoclay was added to the soil material passing sieve No. 40, and the blend was then processed according to test standards. In this study, the remolded specimens, which were tested for unconfined compression and swelling, were shaped by adding the optimum water content of the untreated soils and attaining the maximum dry density as obtained from the results of the Harvard miniature compaction test.
As the fundamental core of this research is to investigate the effect of mixing methods on the efficiency of using nanoclay as a stabilizing agent, two series of experimental testing were carried out by employing two mixing methods. In the first series, the mixture of nanoclay and water was stirred manually in a beaker to reach a relatively distributed state. However, for the second series, the sonication process was applied to disperse and homogenize the mixture by using a sonicator of a frequency of nearly 20 kHz for about 10 min before adding the solution to the soil subsequently.
Testing methods
A set of laboratory experiments were performed to judge the effectiveness of nanoclay in stabilizing the soil. The Harvard miniature compaction test, unconfined compression test, swell test, and Atterberg limits tests were carried out following Wilson (1970), ASTM D2166 (2007), ASTM D4546-08 (2008), and ASTM D4318 (2000), respectively.
The compaction test was conducted on both soils treated with different proportions of nanoclay to demonstrate the effect of the nanoclay addition on the maximum dry density and the optimum water content. Compaction curves were established for all cases using sonication and manual methods following (Wilson, 1970). This compaction method consists of packing the soil-additive blend into the Harvard miniature mold of known dimensions (an inner diameter of 3.3 cm and a length of 7.1 cm) within three equal layers. Each layer was compacted by 25 blows using a spring hammer.
Performing the unconfined compression test was essential in this research as the test may efficiently indicate the effect of the nanoclay addition on the strength of the soils studied. Treated by both sonication and manual mixing, untreated soil specimens were prepared by compacting the soil-additive blend within three equal layers using the Harvard miniature mold. Then, the unconfined molded specimens were vertically subjected to compression within a constant rate (1.270 mm/min per ASTM D2166) until failure had occurred, and the compressive strength was recorded per as the standard. Figure 6 shows the unconfined compression testing device that was used during this study.
The one-dimensional swelling test was also conducted in this study to present the impact of the nanoclay addition on the swell–shrink behavior of the soils. All treated and untreated soil samples were compacted in a mold of 50 mm in diameter and 20 mm in thickness. Then, the mold was placed on a flexible oedometer, submerged in water and allowed to swell freely (Figure 7). The increase in the height as a percentage of the original thickness of the sample was noted as the free swell percentage of the sample. The swell pressure was recorded by loading the swelled soil sample gradually until the soil was once more compressed to its initial height.
The Atterberg limits tests were conducted on all treated and untreated soil samples to evaluate the effect of the nanoclay addition on the plasticity of soils. The tests were performed following the standards as the liquid limit was obtained by dropping the brass cup in the Casagrande device with a rate of 2 rev/sec and the plastic limit was determined by rolling the soil with a rate of 80 strokes/min.
Results and discussion
Compaction
Figures 8, 9 show the results of the maximum dry unit weight of soils and the optimum moisture content (OMC) variation upon increasing the stabilizer quantity.
Figures 8, 9 indicate that the compaction results for the sonicated sample of Soil A at 0.5% stabilizer exhibited an increase in the maximum unit weight of 14.5% and a 35% decrease in OMC compared to natural soil, while for manual mixing at 1% stabilizer, the increase of maximum unit weight reached 11.6% with 19.2% decrease in OMC compared to natural soil.
The same figures showed the results of compaction tests of the sonicated sample of Soil B at 1% stabilizer. Under these conditions, the maximum unit weight increase reached 13.5% with 28% decrease in OMC compared to natural soil, while for manual mixing at 1% stabilizer, the increase of maximum unit weight reached 8.1% with 18.3% decrease in OMC compared to natural soil.
There is an obvious increase in the maximum dry unit weight values when adding the stabilizer to the soil samples. When adding the stabilizer to the soil, it enhances the packing density of soil particles and decreases the space between them; henceforth, the free water decreases, resulting in an increase in the internal friction between solid particles (Bahmani et al., 2014). By the filling effect of nanoclay, the nanoparticles reduced the porosity between soil particles and bonded the particles together (Majeed et al., 2014).
Based on the results, the optimum content of the stabilizer is 0.5% for sonicated samples and 1% for non-sonicated samples for Soil A and 1% stabilizer for both methods of mixing for Soil B. After the optimum content of the stabilizer, the increase will lead to the agglomeration of the nanoparticles, which in turn may cause an increase in the void ratio then a decrease in density and hence an increase in water content, especially for manual mixing. According to Ferkel and Hellmig (1999), the agglomeration of nanoscaled powders increases the number of necks between particles and therefore decreases the density of the associated framework.
Unconfined compressive strength
Figure 10 shows the results of the UCS of the soils and the variation upon increasing the stabilizer quantity.
The results of the UCS for the soils increased significantly after the treatment with the stabilizer. For Soil A, there is a peak present at 0.8% of the stabilizer addition (for sonicated samples with 47% increase compared to natural soil) and 1% (for non-sonicated samples with 28.5% increase). However, for Soil B, the peaks were observed at 0.5% (for sonicated samples with 57.4% increase) and 1% (for non-sonicated samples with 19.3% increase).
It can be observed that nanoclay is a very effective additive for enhancing the strength of soils. Sharo and Alawneh (2016) referred that to the fact that nanoclay acts as a fastening agent that ties soil particles together. It could be summarized that the nanoclay particles under moisture conditions can cause soil stabilization by bonding the particles together. However, the addition of a higher percentage of nanoclay (greater than 0.5% for sonicated samples and 1% for non-sonicated samples) led to lower strength gain, which can be attributed to the interaction and bonding developed between nanoparticles with the soft soil matrix (Khalid et al., 2015).
One-dimensional swelling
Figures 11, 12 show the results of the FSI, the swell pressures of soils, and the variation upon increasing the stabilizer quantity.
A distinguished decrease was shown in FSI of Soil A (Figure 11); at 0.5% stabilizer, the FSI of sonicated samples decreased by 46% from the natural soil and 21.7% for the non-sonicated sample. However, for 1% of the stabilizer addition, the reductions in FSI were 59% and 44%, respectively. The same figure shows similar behavior for Soil B.
The influence of nanoclay addition on swell pressure is shown in Figure 12 for Soil A; for 0.5% of the stabilizer, a decrease for sonicated and non-sonicated samples was observed by 36% and 13%, respectively. However, for 1% stabilizer addition, the decrease was 63% and 41%, respectively. The same trend was noticed for Soil B as indicated in the same figure (Figure 12).
A noticeable decrease was shown in the values; this decrease meant that there was a significant effect when using nanoclay as a stabilizing agent. Even low doses could improve the expansive soil properties (Taha, 2009).
The results showed that the addition of stabilizer particles at a nanoscale level would significantly reduce the amount of swelling of expansive soils, and this, in turn, reduced the required loads needed to return the soil to its original state. This behavior could be explained by the process of filling the intraparticle voids of the soil by the stabilizer particles, and when adding water to the mixture, the stabilizer will absorb most of the water and expand in the intraparticle voids. Therefore, only less amount of water can reach soil particles, resulting in a slight amount of soil expansion. Besides, it was also found by Naval et al. (2017) that when nanomaterials are added to the soil, the nanomaterials fill the tiny pores of the mineral and they allow less water to penetrate these units and thus decrease the swelling of the minerals.
Plasticity index
Atterberg limits tests were conducted on soil samples according to ASTM D4318. Figures 13–15 show the results of these tests: plastic limit (PL), liquid limit (LL), and plasticity index (PI), respectively. The figures indicate that as the stabilizer content increases, LL and PL increase, resulting in a decrease in the PI.
It can be seen in the aforementioned curves that upon mixing 1% of the stabilizer with the expansive soils, the PL increased by 42% for Soil A and 41% for Soil B. Moreover, the LL increased by 31% for Soil A and 17% for Soil B compared to the untreated soil samples.
Figure 15 shows a slight decrease in PI with the increase of stabilizer content. The PI values decreased by 4.8% for Soil A and 1.7% for Soil B. The increase in PL and LL of soils when adding the stabilizer can be attributed to the ability of its particles to absorb water due to its high specific surface area, which is in line with Zhang et al. (2004), who explained that the presence of nanoparticles with intraparticle porosity in the soil shows more plastic and liquid limits. Furthermore, Taha (2009) mentioned that when increasing the specific surface area of nanoparticles, the water required to cover all the particles also increases. Furthermore, Majeed and Taha (2013) added explanations that the presence of nanopores can cause an accumulation of water in these pores, resulting in an increase in the available water capacity in the soil.
This decrease in PI values expresses a predictable improvement in the behavioral actions of soils, which is beneficial for the geotechnical structure, for soil liners and fill caps, as stated by Khalid et al. (2015). It is clear that increasing the stabilizer additive after being added to the expansive soil resulted in a decrease in the PI, which led to a lower ability of the soil to expand and shrink, consequently leading to considerable effects on the performance of structures resting on these types of soil mixes.
Scanning electron microscopy
The fabric arrangement of soil samples was studied under a scanning electron microscope. The obtained images (Figure 16) showed that natural soils were flaky and platy sheets and the dispersion of the particles had an irregular shape with more open spacing between them, which is an indication of the presence of smectite and kaolinite minerals.
The micrographs show a comparison between natural and treated soils with the stabilizer (nanoclay) material using both methods of mixing. The nanostructure of the expansive soils mixed manually with the stabilizer at 0.5% showed that the soil particles agglomerated like a coherent mass. The stabilizer filled the pores and coated the soil grains, resulting in a uniform smooth surface, which increased the density of the treated soils and provided the desired improvement in soil properties. The stabilizer produced denser filling, smaller pores, and more lineament structure in sonicated samples compared with non-sonicated samples. In addition, from Figure 16, it can be observed that the nanoclay additive filled the pores within the natural soil.
Statistical analysis
A summary of the statistical analysis of the test results is shown in Tables 4, 5. The analysis shows the effect of adding the stabilizer on various soil properties to natural soils at different percentages (0.1%, 0.3%, 0.5%, 0.8%, 1%, 1.5%, and 2.5% by weight) using both mixing methods. These tables summarize the comparison between sonicated samples and non-sonicated samples for Soil A and Soil B, respectively, for UCS, maximum dry unit weight, OMC, PI, FSI, and SP, which were evaluated based on the increase and decrease in absolute percent between the natural values and modified soil with different percentages of nanoclay (addition by weight).
For example, it is obvious that when more nanoclay was added to soil samples, higher improvement occurred in UCS and maximum dry unit weight. The results showed that the improvement in soil properties improved when using the sonicator apparatus for the mixing process. For Soil A, at 0.5% of nanoclay, the UCS through manual mixing resulted in a value of 161.4 kPa. While using sonication, the value reached 184 kPa with an increase of 27.8%. However, using nanoclay of 1% for non-sonicated samples resulted in UCS of 185.1 kPa with an improvement of 28.54%.
For Soil B, at 0.5% of nanoclay, the UCS through manual mixing resulted in a value of 190.7 kPa. While using the sonication process, the improvement reached 273 kPa with 57.4% increase. However, using nanoclay of 1% for non-sonicated samples produced a compressive strength of 207.1 kPa with 19.23% increase.
Conclusion
Stabilizing expansive soil with the nanoclay additive shows significant effects on the properties. Based on the results of the study, the following conclusions can be drawn:
1. A controlled additive amount of nanoclay to expansive soil absorbs water effectively and expands in the pores within the soil matrix, leading to a reduction in the swelling behavior.
2. Adding nanoclay to expansive soil (<2.5% by weight) increased the maximum dry unit weight of the soil and UCS. However, it decreased the corresponding optimum moisture content, FSI, and swell pressure.
3. Exceeding nanoclay content to certain limits resulted in an undesirable effect on the mechanical properties of expansive soil due to the agglomeration of the nanoparticles, resulting in a reduction of surface free energy by increasing their size and decreasing their surface area, which led to a decrease in the maximum dry unit weight and UCS.
4. The additive (nanoclay) optimum content to both expansive soils was 0.5% for sonicated samples and 1% for non-sonicated samples.
Data availability statement
The raw data supporting the conclusions of this article will be made available by the authors, without undue reservation.
Author contributions
DA did the experimental work under the supervision of AS. All the authors listed have made a substantial, direct, and intellectual contribution to work and approved it for publication. All authors contributed to the writing.
Funding
The work presented herein was funded by the deanship of research at Jordan University of Science and Technology grant No. 434-2019.
Acknowledgments
The work presented in this paper is part of a master thesis presented by DA at the civil engineering department.
Conflict of interest
The authors declare that the research was conducted in the absence of any commercial or financial relationships that could be construed as a potential conflict of interest.
Publisher’s note
All claims expressed in this article are solely those of the authors and do not necessarily represent those of their affiliated organizations or those of the publisher, the editors, and the reviewers. Any product that may be evaluated in this article or claim that may be made by its manufacturer is not guaranteed or endorsed by the publisher.
Abbreviations
γd,max is the maximum dry density; %w is the water content; CEC is the cation exchange capacity; Dp is the average crystallite size; FSI is the free swell index; LL is the liquid limit; NNI is the National Nanotechnology Initiative; PI is the plasticity index; PL is the plastic limit; S% is the swelling potential; SEM is scanning electron microscopy; Soil A denotes the soil samples taken from Irbid area; Soil B denotes the soil samples taken from Shmeissani area; SP is the swell pressure; UCS is the unconfined compression strength; USCS is the unified soil classification system.
References
Abduljauwad, S. N. (1993). Treatment of calcareous expansive clays. Fly ash for soil improvement, 36, 100–115. New York, NY: ASCE Geotechnical Special Publication. Available at: http://worldcat.org/isbn/0872629864.
Al-Omari, R. R., Fattah, M. Y., and Ali, H. A. (2016). Treatment of soil swelling using geogrid reinforced columns. Italian J. Geosciences 135 (1), 83–94. doi:10.3301/ijg.2014.54 |
Arabani, M., Haghi, A. K., Sani, A. M., and Kamboozia, N. (2012). “Use of nanoclay for improvement the microstructure and mechanical properties of soil stabilized by cement,” in Proceedings of the 4th international conference on nanostructures (ICNS4) (IR Iran: Kish Island).
ASTM D2166 (2007). Standard test method for unconfined compressive strength of cohesive soils. West Conshohocken, PA: ASTM International.
ASTM D421–85 (2007). Standard practice for dry preparation of soil samples for particle-size analysis and determination of soil constants. West Conshohocken, Pa: ASTM International. doi:10.1520/D0421-85r07 |
ASTM D422-63 (2007). Standard test method for particle-size analysis of soils. West Conshohocken, PA: ASTM International.
ASTM D4318 (2000). Standard test methods for liquid limit, plastic limit, and plasticity index of soils, 4. West Conshohocken, PA: ASTM Annual Book of Standards.
ASTM D4546-08 (2008). Standard test method for one-dimensional swell or collapse of cohesive soils. West Conshohocken, PA: ASTM International.
ASTM D854-02 (2005). Standard test method for specific gravity of soil solids by water pycnometer. American Society for Testing Materials.
Bahari, M., Nikookar, M., Arabani, M., Haghi, A. K., and Khodabandeh, H. (2013). “Stabilization of silt by nano-clay,” in Proceedings of the 7th national congress on civil engineering, 7–8.
Bahmani, S. H., Huat, B. B., Asadi, A., and Farzadnia, N. (2014). Stabilization of residual soil using SiO2 nanoparticles and cement. Constr. Build. Mater. 64, 350–359. . doi:10.1016/j.conbuildmat.2014.04.086 |
Chen, F. H. (1975). “Foundation on expansive soils,” in Development in geotechnical engineering (New York: Elsevier Scientific Publishing Company), 12.
Díaz-Rodríguez, J. A., and Antonio-Izarraras, V. M. (2004). “Mitigation of liquefaction risk using colloidal silica stabilizer,” in 13th world conference on earthquake engineering paper (509). Expansive clayey soil using nano-clay material. In geo-chicago, 451–457.
Fattah, M. Y., Al-Lami, A. H., and Ahmed, M. D. (2015). Effect of initial water content on the properties of compacted expansive unsaturated soil. J. Eng. 21 (3), 93–108.
Fattah, M. Y., and Al-Lami, A. H. (2017). Behavior and characteristics of compacted expansive unsaturated bentonite-sand mixture. J. Rock Mech. Geotechnical Eng. 8 (5), 629–639. doi:10.1016/j.jrmge.2016.02.005 |
Fattah, M. Y., Salim, N. M., and Irshayyid, E. J. (2022). Influence of soil suction on swelling pressure of bentonite-sand mixtures. Eur. J. Environ. Civ. Eng. 26 (7), 2554–2568. doi:10.1080/19648189.2017.1320236 |
Fattah, M. Y., Salim, N. M., and Irshayyid, E. J. (2021). Swelling behavior of unsaturated expansive soil. Transp. Infrastruct. Geotech. 8 (1), 37–58. doi:10.1007/s40515-020-00112-z |
Ferkel, H., and Hellmig, R. J. (1999). Effect of nanopowder deagglomeration on the densities of nanocrystalline ceramic green bodies and their sintering behaviour. Nanostructured Mater. 11 (5), 617–622. doi:10.1016/S0965-9773(99)00348-7 |
Feynman, R. P. (1960). There's plenty of Room at the bottom. Pasadena, CA: California Institute of Technology, Engineering and Science Magazine.
Ghasabkolaei, N., Choobbasti, A. J., Roshan, N., and Ghasemi, S. E. (2017). Geotechnical properties of the soils modified with nanomaterials: A comprehensive review. Archives Civ. Mech. Eng. 17 (3), 639–650. doi:10.1016/j.acme.2017.01.010 |
Gupta, H., and Sharma, M. (2017). A review of soil stabilization using polypropylene and wheat husk fiber. Int. J. Innovative Res. Adv. Eng. 11 (4).
Irshayyid, E. J., and Fattah, M. Y. (2019). The performance of shear strength and volume changes of expansive soils utilizing different additives IOP Conference Series: Materials Science and Engineering. IOP Conf. Ser. Mat. Sci. Eng. 518 (2), 022043. doi:10.1088/1757-899x/518/2/022043 |
Kajbafvala, A., Li, M., Bahmanpour, H., Maneshian, M. H., and Kauffmann, A. (2013). Nano/microstructured materials: Rapid, low-cost, and eco-friendly synthesis methods. J. Nanoparticles, 1–3. doi:10.1155/2013/530170 |
Khalid, N., Mukri, M., Kamarudin, F., Ghani, A. H. A., Arshad, M. F., Sidek, N., et al. (2015). “Effect of nano-clay in soft soil stabilization,” in Inciec 2014. 905-914 (Singapore: Springer). doi:10.1007/978-981-287-290-6_79 |
Kodaka, T., Oka, F., Ohno, Y., Takyu, T., and Yamasaki, N. (2005). “Modeling of cyclic deformation and strength characteristics of silica treated sand,” in Geomechanics: Testing, modeling, and simulation, 205–216. Available at: https://ascelibrary.org/doi/abs/10.1061/40797(172)11. |
Luo, H. L., Hsiao, D. H., Lin, D. F., and Lin, C. K. (2012). Cohesive soil stabilized using sewage sludge ash/cement and nano aluminum oxide. Int. J. Transp. Sci. Technol. 1 (1), 83–99. doi:10.1260/2046-0430.1.1.83 |
Majeed, Z. H., and Taha, M. R. (2013). A review of stabilization of soils by using nanomaterials. Aust. J. Basic Appl. Sci. 7 (2), 576–581.
Majeed, Z. H., Taha, M. R., and Jawad, I. T. (2014). Stabilization of soft soil using nanomaterials. Res. J. Appl. Sci. Eng. Technol. 8 (4), 503–509. doi:10.19026/rjaset.8.999 |
Miwa, M., and Yonekura, R. (1994). Fundamental properties of sodium silicate based grouts. Soils Found. 34 (3), 113–121. doi:10.3208/sandf1972.34.3_113 |
Mohammadi, M., and Niazian, M. (2013). Investigation of nano-clay effect on geotechnical properties of rasht clay. J. Adv. Sci. Technol. 3 (3), 37–46.
Naval, S., Cmanualan, K., and Sharma, D. (2017). “Stabilization of expansive soil using nanomaterials,” in Proceedings of the international interdisciplinary conference on science (Singapore: Technology Engineering Management Pharmacy and Humanities), 22–23.
Nelson, J., and Miller, D. J. (1997). Expansive soils: Problems and practice in foundation and pavement engineering. John Wiley & Sons.
Nikookar, M., Bahari, M., Nikookar, H., and Arabani, M. (2013). “The strength characteristics of silty soil stabilized using nano-clay,” in Proc. of 7th symposium on advances in science & Technology. 7thsastech.7-8.
Noll, M. R., Bartlett, C., and Dochat, T. M. (1992). “In situ permeability reduction and chemical fixation using colloidal silica,” in Proceeding of the sixth national outdoor action conference on aquifer restoration, ground water monitoring, and geophysical method (Las Vegas, NV: National Ground Water Association), 443–457.
NSTC (2007). The national nanotechnology initiative - strategic plan. Office of Science and Technology, Washington D.C.: Executive Office of the President of the United States.
Persoff, P., Apps, J. A., and Moridis, G. J. (1996). Effect of dilution and contaminants on strength and hydraulic conductivity of sand grouted with colloidal silica gel (No. LBNL-39347; CONF-970208-16). CA (United States): Lawrence Berkeley Lab.
Shahin, S. S., Fayed, L. A. E. M., and Ahmad, E. H. (2015). “Review of nano additives in stabilization of soil,” in Seventh international conference on Nano Technology in construction (Sharm El-Sheikh, Egypt: NTC).
Sharo, A. A., Alawneh, A. S., Al Zghool, H. N., and Rabab’ah, S. R. (2022). Effect of alkali-resistant glass fibers and cement on the geotechnical properties of highly expansive soil. J. Mat. Civ. Eng. 34 (2), 04021417. doi:10.1061/(asce)mt.1943-5533.0004058 |
Sharo, A. A., and Alawneh, A. S. (2016). “Enhancement of the strength and swelling characteristics of expansive clayey soil using nano-clay material,” in Geo-chicago, 451–457. |
Sharo, A. A., Alhouidi, Y. A., and Al-Tawaha, M. S. (2018). Feasibility of Calcium Chloride dehydrate as stabilizing agent for expansive soil. J. Eng. Sci. Technol. Rev. 11 (6), 156–161. doi:10.25103/jestr.112.19 |
Sharo, A. A., Alhowaidi, Y. A., and Al-Tawaha, M. S. (2019). Improving properties of expansive soil using cement, quick lime and cement-lime blend. Int. Rev. Civ. Eng. (IRECE) 10 (2), 176. doi:10.15866/irece.v10i3.17767 |
Sharo, A. A., and Taamneh, M. O. (2020). Optimizing the use of formalin aqueous by using disposed formalin aqueous to improve properties of expansive soil. Procedia Manuf. 44, 44–51. doi:10.1016/j.promfg.2020.02.203 |
Tabarsa, A., and Hosseini, S. (2015). Effect of adding nano-clay on the mechanical behaviour of fine-grained soil reinforced with polypropylene fibers. J. Struct. Eng. Geo-Techniques 5 (2), 59–67.
Taha, M. R. (2009). “Geotechnical properties of soil-ball milled soil mixtures,” in Nanotechnology in construction (Berlin, Heidelberg: Springer), 3, 377–382. doi:10.1007/978-3-642-00980-8_51 |
Towhata, I., and Kabashima, Y. (2001). “Mitigation of seismically-induced deformation of loose sandy foundation by uniform permeation grouting,” in Proc. Earthquake geotechnical engineering satellite conference, XVth international conference on soil Mechanics and geotechnical engineering, (Turkey: Istanbul), 313–318.
Wilson, S. D. (1970). “Suggested method of test for moisture-density relations of soils using harvard compaction apparatus,” in Special procedures for testing soil and Rock for engineering purposes. Fifth Edition (West Conshohocken, PA: ASTM International), 101–103.doi:10.1520/STP38484S |
Zahedi, M., Sharifipour, M., Jahanbakhshi, F., and Bayai, R. (2014). Nanoclay performance on resistance of clay under freezing cycles. J. Appl. Sci. Environ. Manag. 18 (3), 427–434.
Keywords: expansive soil, nanoclay, stabilizer, sonic waves, swell index
Citation: Sharo AA, Khasawneh MA, Bani Baker M and Al Tarawneh DM (2022) Sonicated waves procedure effect on stabilizing expansive soil by nano-clay: Treat with cause. Front. Built Environ. 8:975993. doi: 10.3389/fbuil.2022.975993
Received: 22 June 2022; Accepted: 08 August 2022;
Published: 15 September 2022.
Edited by:
Domenico Lombardi, The University of Manchester, United KingdomReviewed by:
Mohammed Y. Fattah, University of Technology, IraqShivanshi, Manipal University Jaipur, India
Copyright © 2022 Sharo, Khasawneh, Bani Baker and Al Tarawneh. This is an open-access article distributed under the terms of the Creative Commons Attribution License (CC BY). The use, distribution or reproduction in other forums is permitted, provided the original author(s) and the copyright owner(s) are credited and that the original publication in this journal is cited, in accordance with accepted academic practice. No use, distribution or reproduction is permitted which does not comply with these terms.
*Correspondence: Abdulla A. Sharo, YWFzaGFyb0BqdXN0LmVkdS5qbw==