- 1University of Sarajevo-Faculty of Civil Engineering, Sarajevo, Bosnia and Herzegovina
- 2Department of Structures for Engineering and Architecture, University of Naples “Federico II”, Naples, Italy
- 3Department of Civil Engineering, ISISE, University of Minho, Guimarães, Portugal
The European building stock presents pertinent issues needing suitable strategies to be solved. In detail, this regards seismic safety and energy efficiency of buildings to ensure more liveable and safe cities, which represents an important goal for modern societies. Energy performance includes both comfort and saving, the latter required for a more responsible resource consumption within the building sector, having a considerable contribution to the total demand. Current strategies do not seem to be able to simultaneously solve such aspects satisfactorily, both for higher economic requirements and poor technical feasibility implied by separated and disharmonious interventions. Thus, a holistic perspective should be adopted to pursue such an ambitious objective, which has recently gained increasing attention among researchers. Current requirements are firstly highlighted to show the need for an integrated approach to building retrofit. Secondly, a critical review of integrated strategies combining seismic and energy refurbishment proposed in the literature is reported. Then, special issues are discussed, including the feasibility of an integrated approach applied to heritage buildings. This study revealed that a fully efficient strategy for integrated retrofit has not still been proposed in the literature and there are several open issues to be solved. However, current solutions may be further developed to improve them and the several options currently available demonstrated the increasing attention and importance of the topic. Finally, concluding remarks on this research topic have been drawn to promote future studies.
1 Introduction
In the last few decades, the construction sector has been experiencing a crucial change towards new perspectives and challenges. While in the past attention was focused on new buildings, nowadays the refurbishment of the existing building stock is of primary importance involving a rethinking of procedures to be adopted. This is especially true in Europe, where aged constructions present an increasing need for retrofitting intervention to solve their many deficiencies. As a matter of fact, several requirements are not currently satisfied by existing buildings, thus needing for innovative and appropriate strategies. Solutions already used in practice did not demonstrate to be adequate for current needs, since they are applied in a separated way, resulting poorly compatible with each other and with inefficient results (Marini et al., 2017). So, an integrated approach can represent an effective answer to such a complex and urgent problem.
Before defining a proper solution, it is necessary to clarify the requirements that should be met and satisfied. Energy efficiency and seismic safety are crucial and demanding issues presented by the European building stock. Currently, thermal comfort and energy saving are fundamental conditions for a building, as required by European legislation as well (Directive EU, 2018). Their importance is not only limited to inhabitants’ welfare but also to reduce costs and the environmental impact of constructions. Indeed, existing buildings and especially ancient ones are responsible for a great amount of the total energy and carbon emissions in Europe (Marini et al., 2014), so a solution to improve their performance would result in a reduction of such effects, as well as for their management cost.
Moreover, the seismic safety of existing constructions is a fundamental issue to be guaranteed for people and heritage safeguarding. A great part of European countries fall into seismic-prone areas and recent earthquakes have shown the vulnerability of existing buildings (Cattari et al., 2012). Even if retrofitting techniques are already adopted for seismic strengthening, such interventions are performed in a disharmonious way, not considering the advantages of closer coordination with the thermal function. Such a holistic integration may result in higher efficiency and lower costs of the refurbishment, allowing for a decrese in the payback period of the investment (Menna et al., 2022).
Suitable indicators have also to be defined to assess the effectiveness of a strategy and to establish the most appropriate solutions for each case. Performance targets need to be fixed for each aspect of the refurbishment, namely for the energy and seismic parts, as well as for costs and the technical feasibility of the approach. Most of such targets come from current regulations, both national and European, but different ones may descend from owners and stakeholders. It is worth mentioning that retrofitting of ordinary buildings may not result in the unique or best option from economical or performance points of view. When the conditions of a building are too poor either from the energy or seismic perspective, or even both, refurbishment may not represent a valid choice, and partial or total demolition with consequent reconstruction can lead to higher benefits and advantages (Power, 2010).
Historical buildings deserve a special mention since their cultural value and unicity need to be preserved, but at the same time, their proper use has to be guaranteed to avoid their abandonment. This is particularly important in small and ancient centres, where poor conditions and comfort of buildings represent an important obstacle for the survival and conservation of these towns (De Berardinis et al., 2014). Therefore, there is a need for a delicate balance between preserving the historical value of these constructions and their rehabilitation to allow their use.
This paper is organized as follows: Section 1 provides the description and framework of the research topic; Section 2 deals with the several reasons that make an integrated approach to retrofit existing buildings a convenient and urgent measure, not limiting the analysis only to practical advantages, but also to national and international policies requiring such types of solutions; Section 3 presents a critical review of methods and strategies proposed in the literature, highlighting their benefits and drawbacks, as well as possible further developments; Section 4 discusses specific issues, such as the approach to retrofit heritage buildings and the decision-making process criteria for demolition or refurbishment of existing constructions; Section 5 shows the concluding remarks.
2 Current needs for existing buildings
2.1 European commission policies
The development of energy policies was at first connected to the Oil Embargo back in the 1970s (Alpanda and Peralta-Alva, 2010). As the oil crisis affected the countries belonging to the Organisation for Economic Cooperation and Development (OECD), energy efficiency became a domineering policy response for the development of security in the oil sector in the following few years (Papadopoulos, 2016; Brown and Huntington, 2017). Bilgin (Bilgin, 2010) elaborated in detail on the comprehensive analysis conducted by Schollnberger in 1998 regarding the consumption of energy in relation to geological and other factors. The scenario marked as “C” was represented as an energy mix that featured an intermediate demand for hydrocarbons and increasing demand for alternative resources. The scenario was based on fifteen assumptions and the focus was not only on the oil sector but moved to other energy sources, like natural gas, environmentally friendly hydroelectric power plants, renewable energy, etc. (Bilgin, 2010). Ten out of fifteen of Schollnberger’s assumptions became reality, while the rest are on the road to be implemented but in various degrees.
The oil crisis was the trigger for the adoption of the Resolution for the promotion of energy savings in 1974 by the European Council (Council of the European Union, 1974). From that point forward, several Council Resolutions, Regulations, Directives, and Communications have been adopted and published until today. For details, the reader can refer to (Economidou et al., 2020).
The Energy Efficiency Directive (Directive 2012/27/EU, 2012) defined the 20% energy efficiency of the Union’s primary energy consumption by 2020 and paved the way to further improvements after 2020, becoming a part of the European Energy and Climate package (Rosenow et al., 2017). For the targets to be reached, the Directive defined compulsory energy efficiency policy measures and devoted several articles specifically affecting buildings (Article 4: Long-term renovation strategies; Article 5: Central government buildings; Article 9: Metering; Article 10: Billing information; Article 11: Cost of access to metering and billing information; Article 18: Energy services, Article 19: Split incentives). The EED, 2012/27/EU Directive 2012/27/EU (2012)-Energy efficiency directive EED, 2012/27/EU has been amended in 2018 by 2018/844/EU (Directive EU, 2018), extending the duration of the policy and setting the targets until 2030.
2.2 Energy efficiency and seismic safety
The trend of seismic strengthening and energy efficiency improvement has increased by 20% compared to the 1980s, and most probably will continue to increase in the future (Joint Research Center (JRC) et al., 2015), due to the characteristics of the existing building stock and respecting the sustainable use of natural resources, as defined in the EU Regulation on Construction Products (CPR 305/2011/EU, 2011). Research conducted by the Buildings Performance Institute Europe (Buildings Performance Institute Europe (BPIE) and Economidou, 2011) revealed that 40% of all residential buildings (18.75 billion m2 of useful floor space) constructed in 27 EU member states plus Switzerland and Norway were built before the 1960s. This all indicates a rather high insufficiency of seismic capacity and energy efficiency and an increase in material degradation.
In the seismic-prone zones (Wössner et al., 2015) (a significant part of Europe) the issue of energy efficiency cannot be analysed solely but in conjunction with seismic safety. In the European Union, more than one-third (36%) of CO2 emissions are caused by buildings (Directive EU, 2018), while 50% of the EU energy consumption is taken by heating and cooling (Rojs, 2017). In 2011, European households were responsible for 68% of the total energy use in buildings (Buildings Performance Institute Europe (BPIE) and Economidou, 2011). The three analysed sectors in Europe (South Europe with six counties having a total population of 129 million; North and West Europe consisting of 13 counties and a population of 281 million; and Central and East Europe with ten countries and a population of 102 million) revealed that in all three regions the majority (in the range of 35%–42%) of the existing buildings were constructed before any kind of energy efficiency policies (before the 1960s). The construction of new buildings going from 1991 to 2010 is in the range from 14% in Southern Europe to a maximum of 19% in North and West European countries. The remaining percentage in the range from 39% to 49% belongs to buildings constructed from 1961 to 1990, which can be considered the second construction boom (Mazzarella, 2015) with the application of some kind of energy efficiency measures.
The development of the seismic codes followed some major earthquake activity in certain regions of Europe (Crowley et al., 2021), and four categories of seismic design were marked. From no seismic design (CDN), low code (CDL), moderate code (CDM), to high code (CDH). The buildings of design class CDN were mainly built before the 1960s, which can be connected to the first major group discussed ahead. Depending on the earthquake ground motions, in certain parts of Europe, the design class CDL was introduced from the 1950s to the 1990s. While moderate codes (CDM) were introduced at end of the 20th century and high code (CDH) was introduced in several European countries like Greece, Cyprus, Spain, and Italy (Crowley et al., 2021). Having in mind that more than 40% of all structures in Europe were constructed before the 1960s, possessing limited seismic and energy efficiency regulations, some questions about their structural safety and energy performance should arise. In the last decades, various researchers have investigated the vulnerability of existing buildings, many times motivated by catastrophic seismic events (Donaire-Avila et al., 2012; Indirli et al., 2013; Fiorentino et al., 2017; De Luca et al., 2018; Chieffo and Formisano, 2019; Ademović et al., 2020; Chieffo and Formisano, 2020; Ademovic et al., 2022; Aguilar-Meléndez et al., 2022; Avila-Haro et al., 2022).
It is only in the 80s and 90s that the application of the modern codes for seismic and energy design started in different countries in Europe. In this respect, the vulnerability of structures in both segments is more than evitable. As a majority of the building stock was constructed before the implementation of the seismic codes, these buildings, made of either masonry or reinforced concrete (RC), do not comply with the requirements set in the Eurocodes. The inadequacy of seismic performance is connected to poor material quality and/or aging and material degradation, inadequate reinforcement detailing, and neglecting the influence of infill walls. As mentioned ahead, the inclusion of the first energy codes resulted in the 90s, when 66% of the current EU building stock had already been built (IEA-UNDP, 2013).
Three construction periods can be recognized in Europe regarding the implementation of energy efficiency methods. For buildings that were constructed before World War II (before 1945), some kind of energy efficiency measures were implemented (i.e., bioclimate design). A 35-year period, from 1945 to 1980, saw some kind of energy-efficiency codes exist in some European countries, even if they were not strict and most of the countries did not check for their compliance (IEA-UNDP, 2013). These buildings are the least efficient in relation to energy efficiency. A third period started in 1980, when building energy codes started to be introduced with the aim to reduce the energy consumption of new buildings. Energy code provisions have to be applied to existing structures that undergo major renovation since 2002, according to the Energy Performance of Buildings (Directive EU, 2018).
It is only in recent years that seismic and energy demands started to be looked at jointly. A certain part of Europe being at risk from earthquakes, on one hand, and the need for improvement of energy efficiency and increase of comfort of existing deteriorated buildings, on the other hand, have promoted research trying to lead to an integrated approach. (Calvi et al., 2016) proposed an integrated (energy efficiency and earthquake resilience) assessment of buildings and proposed a common indicator named green and resilient indicator (GRI), which takes into account seismic resilience and energy efficiency parameters. Several case studies proved that the joint renovation procedure is economically more acceptable in comparison to sole interventions (Calvi et al., 2016; Mastroberti et al., 2018). Triantafillou et al. (2017) established that joint application of Textile Reinforced Mortar (TRM) and thermal insulating materials is better than a sole application of TRM. The strengthening of infill walls with a combination of new RC structures was elaborated by Manfredi and Masi (2018) for typical RC frame structures designed only to carry vertical loads in Italy.
It is only in the 2018 Directive regarding Energy Performance of Buildings (Directive EU, 2018) that, besides energy efficiency in the long-term renovation strategies of buildings, the seismic aspect was considered as well. Bournas (2018) proposed TRM jacketing and thermal insulation materials for seismic strengthening and energy efficiency for old RC buildings. La Greca and Margani (2018) provided a very detailed analysis of buildings in Italy, indicated barriers that are affecting the implementation of such integrated procedures, and provided some possible solutions which may be implemented in other countries. Formisano et al. (2019) conducted a case study on a masonry building located in Venticano (Italy) and applied a combined procedure of seismic, energetic, and economic measures for its retrofitting. Georgescu et al. (2018) investigated buildings in Italy and Romania. For both countries, energy efficiency procedures are more welcome when compared to seismic strengthening, as the work is conducted on the envelope of the structure. A difficulty identified is that the funding is provided by two different funds and connected to separate legal frameworks. Mistretta et al. (2019) proposed six retrofitting techniques for a single masonry wall taking into account different cities by which various combinations of low/high seismic loads and low/high thermal requirements are covered. Pohoryles et al. (2020) expanded the work regarding the mutual assessment of buildings exposed to various seismic hazards (five seismic zones) and four climate zones, covering twenty European cities in different countries. These authors analysed stone, masonry, and infilled reinforced concrete buildings of various heights. As in the works of previous researchers, they also indicated the economic benefits of a combined retrofit in comparison to sole retrofit procedures.
2.3 Performance indicators
To evaluate the seismic and energy response of a structure or structural element, performance indicators have to be defined, such as the flexural and shear capacity of a wall, or the thermal transmittance (U-value) of the envelope for the thermal aspect. In order to prevent failure under seismic forces, special detailing for boundary elements of reinforced concrete (RC) walls has to be conducted. Different codes provide various guidelines, for example, Wallace and Orakcal (2002) in the ACI 318–08 based the detailing of the boundary elements on the displacement-based concept, while the ductility of RC coded in the Japanese code (Simo, 2003) is connected to the ratio of the neutral axis depth to the wall thickness. The available ductility ratio, used in the earthquake-resistant design, can be defined as the ability of a structural element or the whole system to exhibit big amplitude cyclic deformations, once exposed to earthquake activity. Ductility ratios are expressed as relations between different response parameters related to deformations, which can be presented in the forms of displacements, rotations, and curvatures. For example, the displacement ductility ratio in cyclic loading is based on the envelope curve of the hysteretic loops indicating the connection between the strength and displacement of a structural element or the whole system. Displacement ductility due to its association with the strength reduction factor owing to the nonlinear hysteretic behavior is recognized as a seismic performance indicator.
Various factors affect the displacement ductility capacity of masonry and reinforced concrete structures. Besides the geometry of the structures, height to length ratio, the factors that affect the displacement ductility capacity are the concrete type, web reinforcement ratio, type of reinforcement ratio, boundary type, and axial load ratio. On the other hand, it is necessary to define the maximum displacement capacity which has been proposed in the literature. It is necessary to define the displacement at the peak strength, at 20%–50% degradation from peak strength, and at the moment when the transverse reinforcement fails. Priestley (2000) indicated that it is not easy to determine the displacement ductility capacity of structures due to large differences in the accepted percentages of peak strength deterioration. Park (1988) indicated that the acceptable post-peak displacement would be the one in which a reduction of carrying capacity is in the range of 20% or 25%. Azizinamini et al. (1990) chose the displacement ductility ratio for the assessment of the ductility of lap-spliced RC beam specimens. RC structures that have a flexure deformation have better energy dissipation capacities and ductility compared to low-rise shear walls with traditional steel reinforcement (longitudinal and transverse) in the web (Hsu and Mansour, 2005). Rakhshanimehr et al. (2014) showed that concrete strength and the amount of transverse reinforcement over the splice have major effects on ductility. Changing the amount of transverse reinforcement can have a beneficial effect in obtaining a satisfactory ductility response in relation to the various concrete strengths.
Concerning energy performance, thermal transmittance takes into account heat losses due to conduction, convection, and radiation. Mutual features in the assessment of seismic and energy efficiency analysis are the construction period, structural system or material, and the number of stories (Ademović et al., 2020; Pohoryles et al., 2020; Ademovic et al., 2022). The mutual denominator is age. Construction age is indirectly connected to the code regulations in force at that time of building construction (Ademović et al., 2020) and, based on that in Europe, four categories of seismic design were marked, from no seismic design (CDN), low code (CDL), moderate code (CDM), to high code (CDH) (Crowley et al., 2021). The U-value of the envelope elements is a function of age (Boermans and Petersdorff, 2007).
Manfredi and Masi (2018) showed that with the application of the joint strengthening methodology for an RC frame structure with masonry infill, a structure which is widely spread in entire Europe, the inclusion of new infills and new RC frames significantly increased the seismic performance. This was noted in the decrease of the fundamental period by 30%–40%, and the increase in bending and shear capacity for various seismic hazard levels, from low to high seismic activity, while one climate zone was evaluated, and the thermal insulation value, U, after rehabilitation, was lower than the one prescribed in a selected climate zone. The climate zone has been defined according to the parameter named “Gradi Giorno” (GG)-degree days, which is calculated as the sum throughout a year of the daily difference between the mean value of the expected external temperature and the standard room temperature. Based on this, the territory of Italy is divided into six climate zones.
Pohoryles et al. (2020) conducted a joint retrofitting procedure for twenty cities in Europe considering different seismic hazard zones defined by Peak Ground Acceleration (PGA) for a return period of 475 years and various climate zones defined by heating degree-days (HDD), which is obtained as a difference between the base temperature (taken as 18°C) and the day’s mean temperature. The main parameter for the assessment of thermal performance was again the thermal transmittance of its envelope elements. The performance level of the structures was examined as a measure of the seismic upgrade, meaning that structures constructed with no seismic design would have better performance (one or two levels higher) after the retrofitting measures.
Corrado et al. (2011), Fragoso and Baptista (2016), Urquizo et al. (2019) illustrated an example of the change in the minimum value of the thermal transmittance of envelope elements in relation to the change in the standards in the United Kingdom (Table 1), Italy (Table 2), and two cities in Portugal (Table 3). Existing buildings have to comply with minimum seismic and energy requirements, making it more difficult to perform separated interventions to meet both requirements, so an integrated approach makes rational sense.
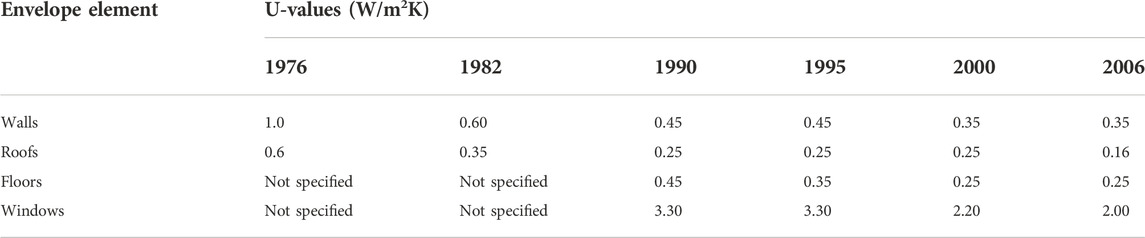
TABLE 1. U-values (W/m2K) in the United Kingdom building regulation (Urquizo et al., 2019).
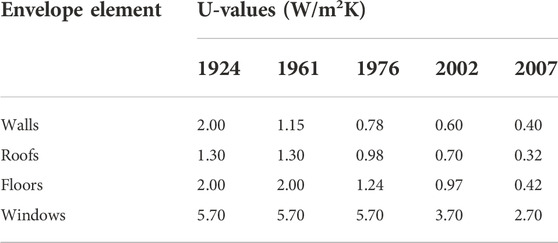
TABLE 2. U-values (W/m2K) in the Italian building regulation (Corrado et al., 2011; Bournas, 2018).
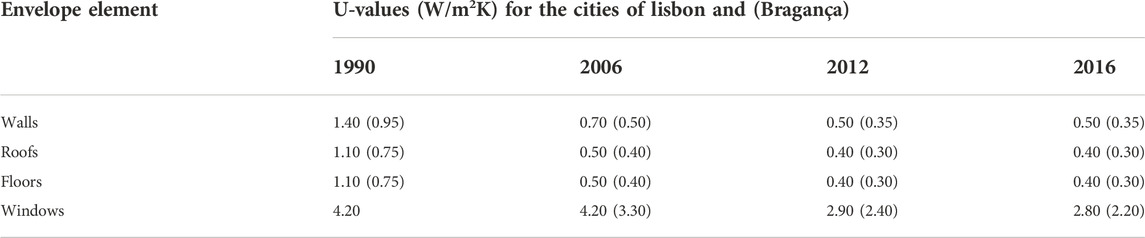
TABLE 3. U-values (W/m2K) in the Portuguese building regulation in Lisbon and Bragança (Fragoso and Baptista, 2016).
Another performance indicator that can be considered for the thermal part is the energy performance certificate (EPC), which is seen as the property’s energy efficiency. Generally, the EPC depends on the amount of energy used per m2 and the level of carbon dioxide emissions (given in tonnes per year). This certificate includes reference values enabling consumers to compare and evaluate energy performance. Information regarding the EPC is important for both the owner of the property and the potential renter.
It may be argued that investing in the better energy performance of buildings may lead to higher rent but to better conform conditions. The analysis was conducted in several countries: Austria, Belgium, France, Ireland, and the United Kingdom; in all countries, excluding the United Kingdom the effects of energy efficiency were clear and positive. However, each country sets its own EPC scheme connected to the regulations of each country, so one has to be cautious when conducting assessments and comparisons between countries (Intelligent Service and Lyons, 2013). A comparative analysis regarding the EPCs schemes within the European Union was conducted by Atanasiu and Constantinescu (Bogdan and Tudor, 2011). The development of the EPC schemes within the European Union and data regarding the seven ongoing Horizon 2020 projects are provided in (Lițiu and Hogeling, 2021). The German Energy Agency GmbH developed a prototype of a nationwide energy certificate entitled “Energy Passport for Existing Residential Buildings” (Energieeinsparverordnung EnEV, 2009). The energy classes are in the range from A+ to H, in which the value of the energy efficiency coefficient is <30 kWh/m2 and <250 kWh/m2, respectively.
Italy has complied with the fundamental European directives 2002/91/EC and 2006/32/EC, producing a classification in categories called Energy efficiency classes of buildings (Classi di efficienza energetica degli edifici) (Costanzo et al., 2016; interm, 2015). Currently, there are 10 classes in the range from class A4 (maximum saving) to class G (minimum saving), as indicated in Figure 1. The calculation is based on the ratio between the total energy consumed in a year by the building per m2 of surface (kWh/m2 year). This is indicated as EPgl (Global Energy Performance). The description of the classes is given in Table 4.
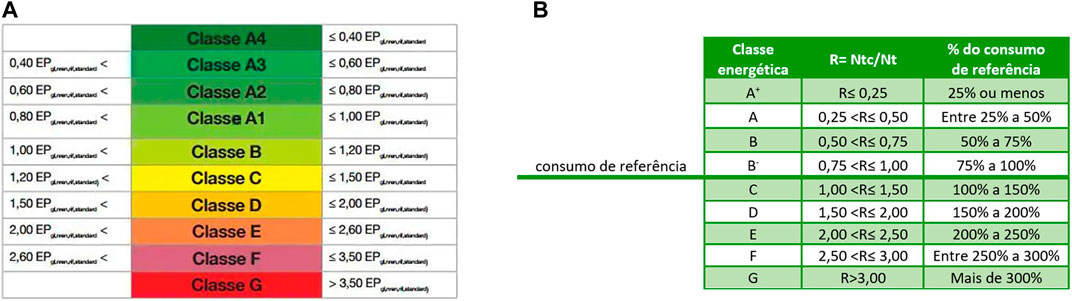
FIGURE 1. Classification scale of residential buildings according to the global Energy Performance (EP) index: (A) Italian ranking (interm, 2015); (B) energy classes in Portugal (adapted from ADENE, 2022).
The Energy Certification System was created in Portugal in 2006, but it has been updated to meet the European Union’s impositions on building requirements, which are becoming increasingly demanding (Fragoso and Baptista, 2016). Buildings Energy Certification System was implemented as mandatory since 2009, in all, new or existing, residential and service buildings (Vaquero, 2020). There are nine energy classes in the range from A+ to F, as shown in Figure 3. The energy efficiency scale results from the ratio between the Ntc primary energy needs of a building and the respective threshold value Nt. New buildings must have a class higher than B-, which represents the consumption of reference (100%). Existing buildings can be of any class; the various classes represent the percentage range consumption, e.g., a class C property consumes between 100% and 150% (between 1 and 1.5 times) of the reference consumption (ADENE, 2022).
An additional parameter crucial for structural engineering is the cost optimization of the strengthening procedure. Various techniques are available for strengthening the existing buildings, taking into account all specific features, aiming at applying the most cost-effective strengthening procedure (Gkournelos et al., 2021). A detailed procedure for RC structures is provided in (Gkournelos et al., 2021). A simplified method was developed by Vitiello et al. (2017) to evaluate the economic performance of an RC structure that may likely be exposed to earthquakes. This approach targets the most cost-effective strengthening procedures for evaluating existing buildings. It should be noted that the PGA level will have an impact on the most-effective intervention procedure. In a study conducted by Vitiello et al. (Vitiello et al., 2017), it was indicated that, for the mean seismicity zone (PGA = 0.168 g), the most effective strengthening procedure was base isolation, while FRP strengthening was in a very acceptable financial range and RC jacketing and construction of new shear walls was not economically acceptable. On the other hand, for low seismicity zones (PGA = 0.071 g) the only economically acceptable procedure would be based on a FRP-base strategy, while base isolation was ineffective, highlighting that the strengthening procedure in structural and economical view depends on the level of the seismic hazard.
Motra and Paudel (2021) conducted a cost-effective analysis of the strengthening procedure of an existing masonry school. Four strengthening methods were proposed. Once the structural and cost-effective analysis was performed, it was decided to strengthen the structure with new RC piers and connections with existing brick walls, extending the interventions only up to the first-storey slab. This procedure was economically the most effective even though it enhanced the building’s capacity slightly, but the strengthening can be extended to other floors if needed later. It was suggested that several features play an important role in the selection of the most acceptable strengthening procedure and one that may play a crucial role was the cost optimization of the intervention.
3 Review of integrated strategies
Current vulnerabilities and deficiencies of existing buildings justify the need for integrated seismic and energy retrofitting strategies. In the last few years, researchers have paid increasing attention to the topic, proposing innovative approaches to face this crucial challenge, but a unique and effective solution does not exist. Specific systems to improve separately the energy and seismic behaviour of buildings have been studied and represent the current approach to retrofitting in practice. However, separated and disharmonious interventions are not an efficient solution for building stock renovation (Caprino et al., 2021). The opportunity for a holistic cost- and resource-saving technique exists (Belleri and Marini, 2016), as shown by recent research involving integrated strategies (Bournas and Pohoryles, 2021).
A further advantage of an integrated retrofit is indeed due to the lower costs achievable by the combined energy and seismic intervention. In addition to many incentives currently provided for the improvement of the energy and seismic performance of existing buildings (e.g., European Green Deal (COM/2019/640, 2019), Italian EcoBonus, and SismaBonus (Law, 2020)), another benefit deriving from an integrated refurbishment is the lower payback time of the investment, which can be considered as an estimation of its economic efficiency (Menna et al., 2022). Such reduction is mainly due to the energy performance improvement given by thermal refurbishment, which translates into higher energy efficiency with lower consumption and building management costs. However, even the decrease in seismic risk due to the structural retrofit can be interpreted from an economic point of view as a reduction in expected losses in the event of an earthquake (Belleri and Marini, 2016), thus making the economic benefit of the integrated retrofit twofold.
3.1 Exoskeletons
Some authors studied the possible use of steel exoskeletons, already adopted for structural retrofit purposes (Formisano et al., 2020a; Di Lorenzo et al., 2020), combined with thermal and façade systems for energy and aesthetic rehabilitation (Marini et al., 2017; Passoni et al., 2020), see Figure 2. Exoskeletons are based on parametric design (Kerdan et al., 2015), an architectural concept already used for energy and functional refurbishment, allowing a flexible solution to achieve the target performance. More recently, such a strategy has been proposed for integrated retrofit to foster its benefits in a unique system (D'Urso and Cicero, 2019). Among the advantages of this solution, it emerges the possibility to perform an intervention from the outside of the building, thus reducing the occupancy disruption involved by restoration works. Moreover, it allows a certain architectural freedom in new façades design, as well as a good level of adaptability and reparability due to the modularity of such structures. However, steel exoskeletons seem suitable for suburbs and less usable in high-density areas like city centres, since they require a considerable additional space around the building. Also, their application is not acceptable for cultural heritage buildings as the façades of the buildings have to be preserved. In addition, the new structure involves a significant quantity of material, making higher the costs and the embodied carbon entailed by the intervention. Steel exoskeletons have been also combined with other types of refurbishments, such as indoor climatic conditioning systems, as proposed by Ferrante et al. (2018). In this study, an integrated renovation based on exoskeletons also provided a new high-efficient heating ventilation air conditioning (HVAC) system that has been suggested to considerably enhance the energy performance and thermal comfort of existing buildings. This is an example of how many benefits can be obtained from the combination of active and passive techniques for energy retrofit with seismic strengthening systems. This strategy has been mainly studied for reinforced concrete (RC) buildings, whose characteristics allow to perform connections between concrete members and the steel exoskeleton. As for masonry structures, it may imply some technical issues, depending on the actual conditions, and lower effectiveness of the solution due to the reduced ductility of masonry.
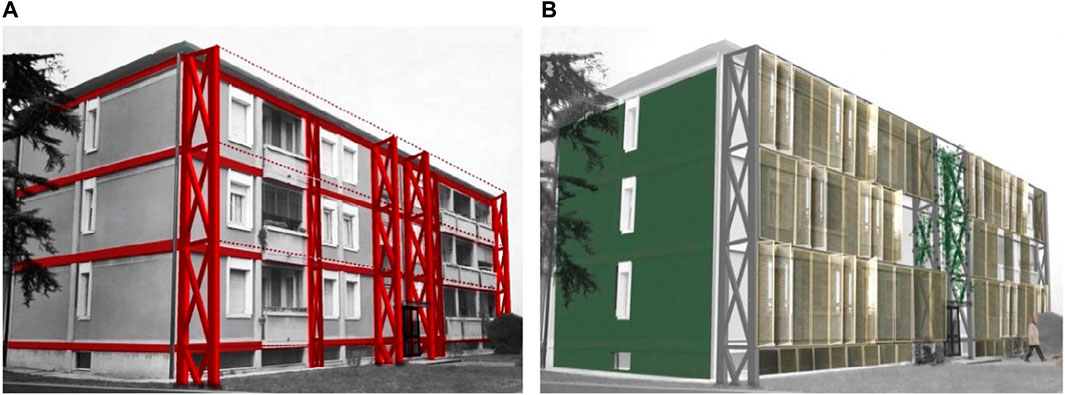
FIGURE 2. Application of a steel exoskeleton system for integrated seismic and energy refurbishment of buildings: (A) external structure for seismic retrofitting; (B) façade systems for energy efficiency and aesthetic renovation (Marini et al., 2017).
3.1.1 Responsive exoskeletons
To overcome the issue related to low ductility structural elements, responsive exoskeletons have been proposed; such structures can be passive, with the adoption of sacrificial elements, or active, with actuators implemented. Taking inspiration from nature, bio-inspired adaptive building skins (Loonen, 2015) enhance the system’s adaptability, as well as its multi-ability and evolvability according to climatic conditions. Similarly, responsive exoskeletons can be designed to change their behaviour depending on the earthquake intensity to reduce the solution dimensions and further optimize the structural response of the system.
Considering that base shear forces are typically high for traditional non-dissipative solutions hinged at the base, involving consequent problems for ordinary foundations, Labò et al. (2016) proposed adaptive-responsive diagrids (see Figure 3). With this system, a controlled soft-storey mechanism of the existing building is imposed, conceiving the structure to change its boundary conditions depending on the earthquake intensity. In this way, for higher levels of seismic loading, special supports at the base are activated allowing a fixed amount of sliding to reduce seismic forces and increase the building’s displacements. Despite the advantages of this technique for stiff constructions, such as masonry ones, preliminary interventions are required on the existing elements, involving higher costs and time for the retrofit. A similar concept has been developed by Scuderi (2016), who proposed adaptive exoskeletons connected to the building with shape memory alloys-based dampers (SMADs). However, the high costs of such innovative devices compared to ordinary construction technologies and the need for further research concerning their employment in civil engineering applications make them a much less affordable retrofitting solution. Nonetheless, due to their advantages, they may represent a suitable strategy in the future for the integrated seismic and energy retrofit of specific types of buildings.
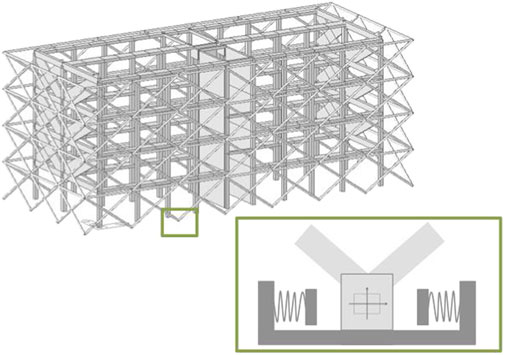
FIGURE 3. Schematic representation of a responsive exoskeleton for seismic and energy retrofit with a simplified scheme of the special sliding supports (adapted from (Labò et al., 2016)).
In another work, Feroldi et al. (2014) proposed the idea of developing dissipative connectors between the exoskeleton and the existing structure, namely by employing buckling restrained braces. By concentrating the possible damage induced by earthquake actions in correspondence with the connections, it is possible to minimize seismic consequences affecting the existing elements. So, post-earthquake repairing works can be limited to the replacement of a lower number of damaged components of the new structure, resulting in a more cost- and time-effective intervention. Nonetheless, incorrect design or installation of the connectors may produce an unexpected structural response, with even catastrophic consequences for the retrofitted construction.
3.1.2 RC exoskeletons
Proposals based on RC exoskeletons for integrated renovation can be found in the literature, such as an RC shell structure ((Pertile et al., 2021), see Figure 4) or an external RC frame ((Pozza et al., 2021), see Figure 5), combined with EPS insulation panels. These solutions have the clear advantage of being applied from the outside, limiting invasiveness and interruption of usage. However, they have drawbacks comparable to steel exoskeletons, as well as longer intervention time and less reversibility, as the proposed RC elements are typically not made of precast concrete. On the contrary, Manfredi et al. (Manfredi et al., 2021) proposed a high-performance dissipating frame (HPDF) system based on external precast RC frames and supplied with damper devices for energy dissipation capacity. Trying to reduce some disadvantages of previous RC-based strategies, this solution has been proposed for seismic strengthening only, but a possible integration with energy retrofitting systems may further increase its benefits.
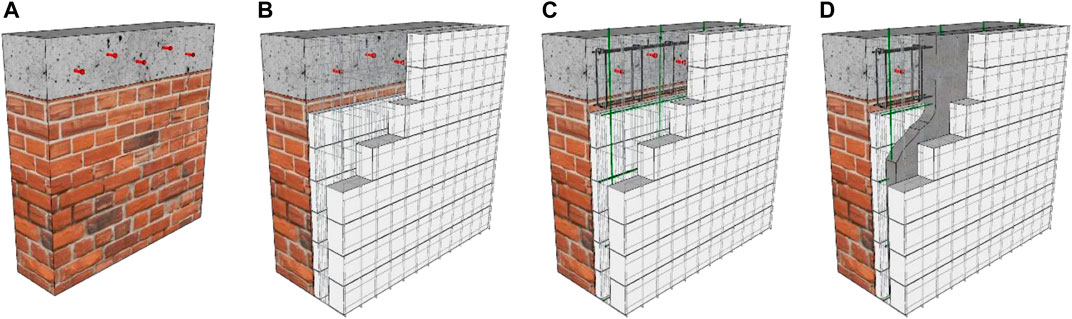
FIGURE 4. Construction phases of the integrated solution based on RC shell structures: (A) anchoring installation; (B) disposition of insulation panels; (C) placing of the steel reinforcement for RC elements; (D) concrete casting (adapted from (Pertile et al., 2021).
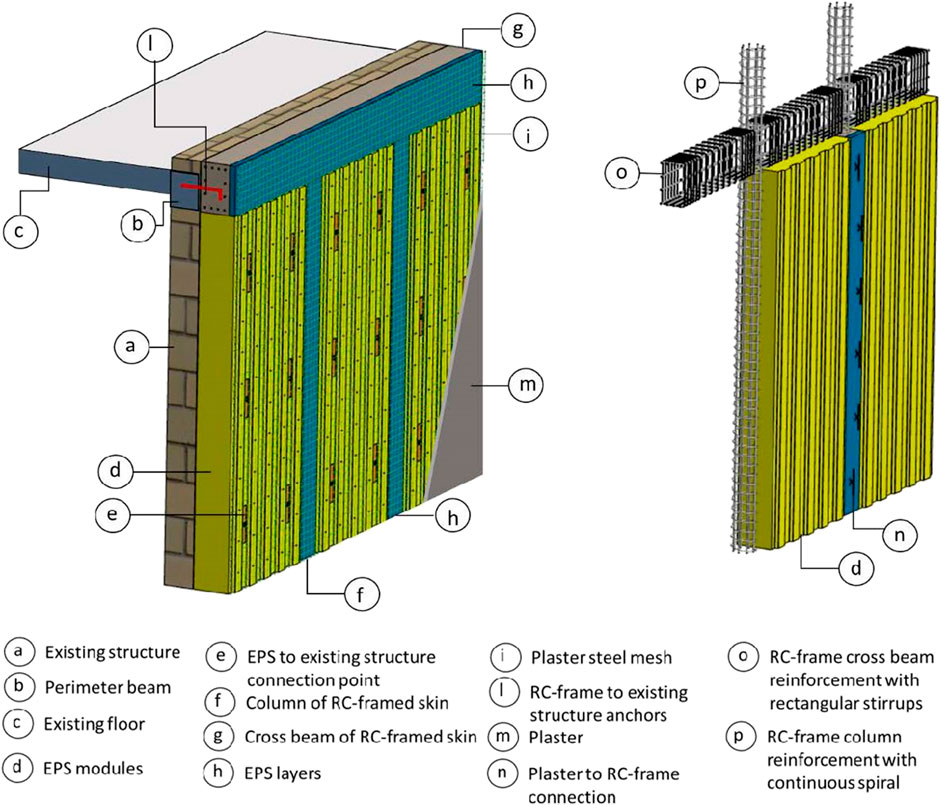
FIGURE 5. RC frame exoskeleton: global view (left); detail of EPS insulation panel and steel reinforcement (right) (Pozza et al., 2021).
3.2 Timber based solutions
3.2.1 Cross laminated timber panels
A sustainable strategy has been proposed by adopting cross laminated timber (CLT) panels, an engineered wood product combining lightweight and good mechanical properties with low thermal conductivity. It is a prefabricated solution that can be externally applied without requiring a long intervention time. CLT panels have been developed in the last few decades (Brandner et al., 2016) allowing the creation of two-dimensional structural elements with considerable dimensions, depending on production machine size and transport limitations. Studies on CLT construction systems have been mainly focused on its use for new structures (Izzi et al., 2018), but more recently CLT boards have been proposed for structural retrofit (Stazi et al., 2019) and, in some cases, for integrated renovation (Dalla Mora et al., 2015; Margani et al., 2020) (see Figures 6, 7). In this framework, Smiroldo et al. (2021) proposed two possible strategies employing CLT panels: the first one is based on the replacement of the external layer of infill masonry walls using CLT boards, while the second one involves a less invasive intervention through the application of CLT panels from the outside without removing infills. In both solutions, CLT panels are provided with insulating layers placed on the inner and outer sides of the timber panel. Regarding seismic response, CLT panels avoid brittle mechanisms of RC members due to infill walls and prevent out-of-plane mechanisms of masonry infills. Therefore, the results demonstrated that the proposed integrated intervention approach can significantly reduce both the seismic vulnerability and the energy consumption of RC buildings.
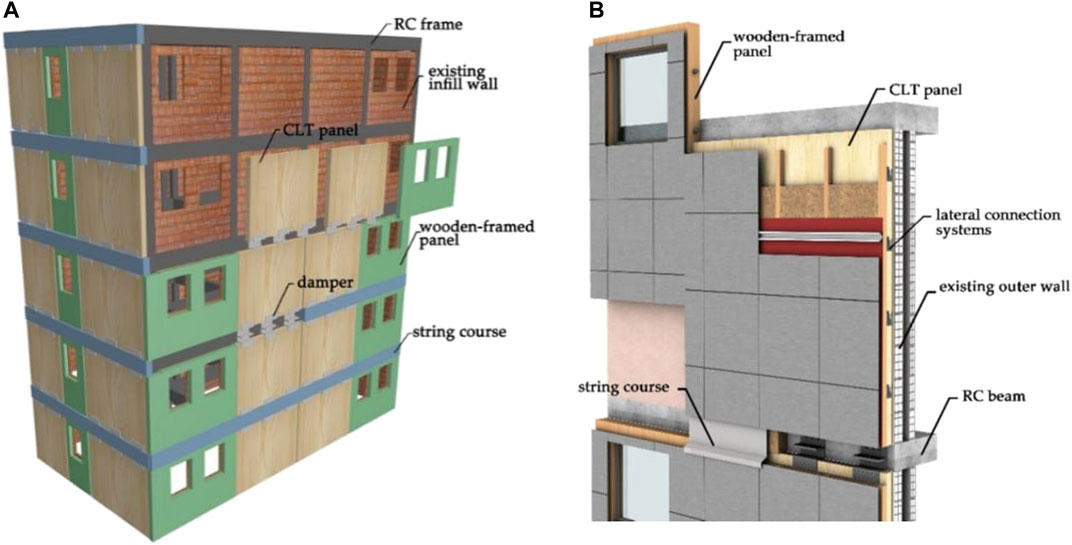
FIGURE 6. CLT retrofitting solution: (A) conceptual scheme; (B) detailing of the prefabricated timber panels combined with ventilated façade system (Margani et al., 2020).
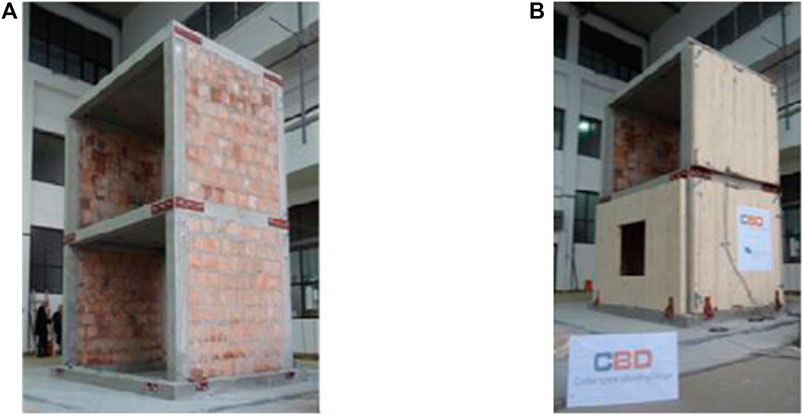
FIGURE 7. RC frame with masonry infill walls: (A) not retrofitted and (B) retrofitted structures with CLT panels (adapted from (Sustersic and Dujic, 2014)).
Among the advantages of the employment of CLT panels for integrated retrofit, it should be considered the possibility to limit the additional masses due to the intervention. This implies a better seismic behavior of the retrofitted building when compared to the application of more massive additional elements such as exoskeletons. However, connections between new and existing elements may constitute a critical drawback depending on the building conditions, especially for masonry structures, even if innovative systems have been proposed (Sustersic and Dujic, 2014). To assess the seismic capacity improvement due to the employment of CLT panels and the effectiveness of the connecting system, it is necessary to perform real-scale tests like those presented in Figure 7, which involve considerable costs and time to be carried out. Moreover, the thermal properties of CLT panels could be insufficient to guarantee adequate performance and comfort levels, thus requiring additional insulation layers of higher-performing materials, with an increase in the overall thickness and a reduction of the solution advantages. On the other side, CLT panels, like most timber-based solutions, are natural and sustainable products, which allow reducing the environmental impact of the retrofitting intervention, in accordance with current European policies (COM/2019/640, 2019).
Borri et al. (2021) proposed to use CLT panels combined with steel wire ropes for the in-plane strengthening of rubble stone masonry walls in historic structures (Figure 8), improving at the same time the energy performance of the existing envelope. Besides the possible inadequacy of the thermal improvement due to CLT properties, such an approach causes moderate increments of the wall shear behaviour together with induced out-of-plane deformations, limiting the intervention effectiveness. Moreover, a careful evaluation concerning the compatibility of this technique with historical substrates should be considered.
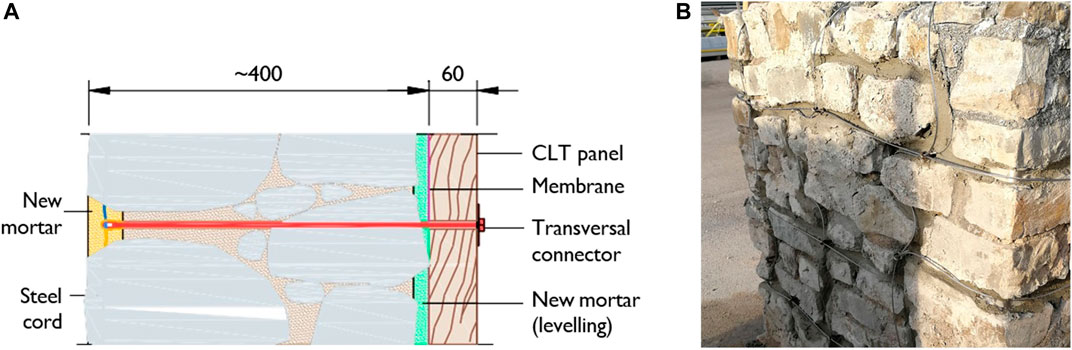
FIGURE 8. CLT panels combined with steel wire ropes for combined seismic and energy retrofit of buildings: (A) vertical cross-section; (B) detail of the steel wire ropes at one side of the wall (adapted from (Borri et al., 2021)).
3.2.2 “Nested buildings” technique
CLT panels have been also applied for internal retrofit interventions in buildings with listed façades (Lucchini et al., 2014; Valluzzi et al., 2021) to create a totally new internal CLT structure with improved structural safety and thermal efficiency while maintaining the external envelope (see Figure 9). Such a type of technique also called “nested buildings”, allows to achieve performances comparable to new structures, but implies a very invasive intervention with the demolition of internal finishing and slabs, possibly not feasible for historic heritage buildings. Despite the high improvements that can be achieved both in terms of thermal efficiency and seismic capacity, this technique is limited to a restricted set of buildings on which it can be successfully applied, preserving their cultural value.
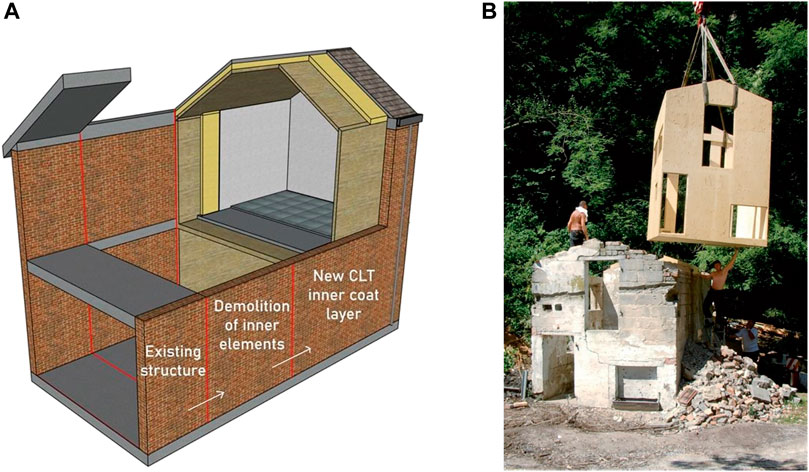
FIGURE 9. Nested building retrofitting intervention through CLT panels: (A) conceptual representation of work phases (Valluzzi et al., 2021); (B) example of the insertion of the new CLT structure inside the existing envelope (Lucchini et al., 2014).
3.2.3 Alternative timber-based strategies
Different timber-based products other than CLT panels have been studied and proposed in the literature for the retrofit of existing buildings. Iuorio et al. (2021) studied the application of oriented strand board (OSB) panels to improve the out-of-plane flexural capacity of masonry walls. The use of OSB panels alone without the presence of an additional timber frame, as usual in timber light frame structures, is a considerable advantage for this strategy since it allows for a reduction of the solution thickness. In addition, thanks to the lower costs involved by OSB panels compared to CLT ones, this retrofitting system may represent a cheaper option yet with good performance. However, the study has been limited to the out-of-plane behaviour of retrofitted walls, while the in-plane response of masonry wallets strengthened with OSB panels has not been assessed. Moreover, no investigations concerning the thermal performance of this solution have been carried out, thus requiring further research to understand whether this system can be suitable for the integrated refurbishment of buildings.
An integrated retrofitting solution has been studied by Busselli et al. (2021), which investigated both the structural and energetic performance achievable utilizing timber-based panels, namely CLT and laminated veneer lumber (LVL), applied to masonry walls. For the latter, veneers oriented transversally to the main direction of the element have been considered. Moreover, both traditional and state-of-the-art (i.e. aerogel) thermal insulators have been included in the solution to improve its thermal performance. Several configurations have been considered in the analysis, also adopting vertical timber elements, called strong-backs, to improve the out-of-plane behaviour of the retrofitted wall and hybrid solutions with both panels and vertical timber members. It has been found that a hybrid solution with LVL panels and strong-backs may lead to a substantial improvement of the seismic capacity and a considerable reduction in the thermal transmittance of the retrofitted wall, depending on the type of thermal insulator used. It should be noted that such results have been mainly obtained from numerical analysis, thus requiring extensive experimental validation to confirm such promising performance increments. Despite the high thermal benefits, the use of aerogel for thermal insulation represents a strong limitation of the solution due to its high costs. Moreover, the combination of timber panels and strong-backs involves a considerable thickness of the solution, so reducing either the usable floor area or possibly conflicting with surrounding constraints for internal or external applications, respectively.
3.3 Composite materials
3.3.1 Textile reinforced mortar
Textile Reinforced Mortar (TRM), also termed Fabric Reinforced Cementitious Matrix (FRCM) composites (Triantafillou, 2016) has been recently applied coupled with insulation panels for seismic and energy retrofit purposes (Triantafillou et al., 2018; Gkournelos et al., 2020). The first experimental results demonstrated that this combined solution increases the seismic safety level of the retrofitted construction, even considering the application on one side only and with different dispositions of the TRM system with respect to the insulation layers (see Figure 10). In this way, it is possible to apply the system either externally, avoiding excessive occupancy disruption, or internally, to preserve listed façades. However, one-side applications involve an asymmetric disposition of the retrofitting with possibly not sufficient seismic capacity increments for highly vulnerable structures. Moreover, buckling effects in the TRM in-plane response may be an issue for this strategy requiring specific solutions such as proper anchorage systems (Bournas et al., 2015).
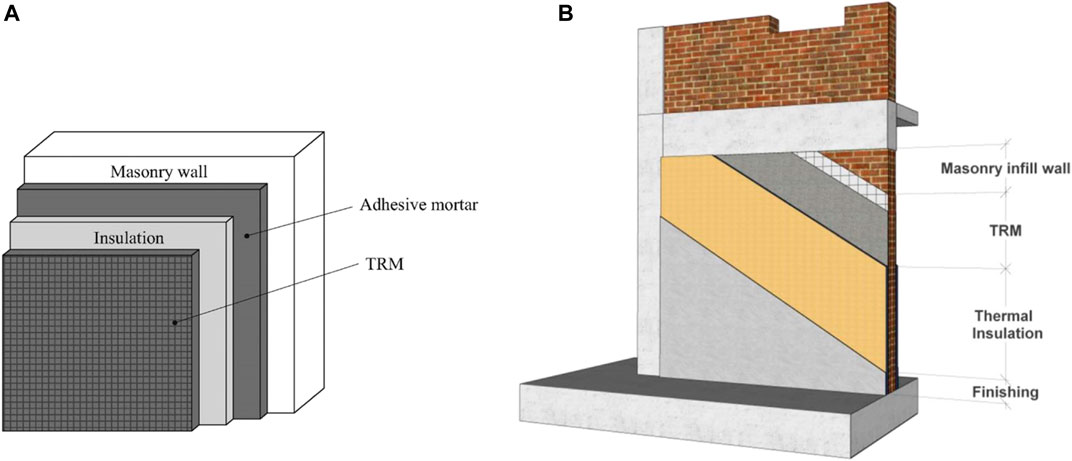
FIGURE 10. Scheme of the TRM structural and energy retrofitting solution: (A) masonry wall (Triantafillou et al., 2018); (B) RC frame (Pohoryles et al., 2020).
Concerning the seismic response of the TRM technology, it mainly depends on the TRM system, even if some studies in the literature have investigated possible structural benefits derived from the application of thermal insulation panels on masonry walls (Manos et al., 2021). Among the advantages, their durability and lightweight make TRM a suitable solution for seismic retrofit. Furthermore, specifically for TRM, the use of lime-based mortars allows their application in masonry buildings thanks to the higher compatibility between existing and new materials when compared to cement-based mixtures. Concerning the local seismic response of structural elements such as masonry walls, TRM systems can greatly improve both their in-plane and out-of-plane behaviour (Minotto et al., 2020). On the other hand, the application of TRM only externally may not be sufficient to achieve an adequate seismic capacity improvement for an entire building in global terms. In this case, it may be required the employment of the TRM-based solution on the inner side as well, thus making it a more invasive intervention. So, the application of TRM-based solutions on the envelope of buildings is a very good, fast, and efficient option regarding the in-plane and out-of-plane seismic behavior of façades, but in some cases, it may be necessary to couple this strategy with other techniques or to apply TRM also inside the building.
No detailed investigations have been carried out to assess the thermal performance of this solution, being previous studies focused on the structural response of retrofitted walls. Also, the proposed applications mainly consider the use of Expanded Polystyrene (EPS) or Extruded Polystyrene (XPS) panels, non-eco-friendly materials yet nowadays commonly used in ordinary applications for their good thermal properties and relatively low cost. Some theoretical considerations concerning the use of different products have been done in (Bournas, 2018), whose proposals deserve further attention and validation by employing specific experimental studies.
3.3.2 Enhanced mortars
Following a different approach, Borri et al. (2015) considered the adoption of mortars with improved thermal properties, namely reducing their thermal conductivity. Made of low-strength lime-based mortars, such a TRM inorganic matrix has a twofold function, since it can combine mechanical and thermal benefits into the same material, reducing the solution thickness by avoiding the insertion of an additional insulation layer. Furthermore, lime-based mortars are more compatible with masonry substrates than cement ones in terms of chemical composition, mechanical performance, and stiffness. However, it is difficult to obtain mortars with a balanced performance as their mechanical properties are usually not sufficient for TRM applications, as also demonstrated by Longo et al. (2019). Therefore, it is necessary to find a proper equilibrium between the mechanical and thermal properties of mortars, as well as to consider a supplementary layer with good insulation properties. However, inserting an additional insulator involves a greater thickness and longer time for the intervention, thus reducing the advantages of such an approach.
Illampas et al. (2021) studied the incorporation of Phase Change Materials (PCMs) into mortars to improve their thermal performance and obtain a suitable matrix for integrated structural and energy retrofit of building envelopes. As for thermal mortars, the addition of microencapsulated PCM causes a reduction of the mechanical properties due to the increase of mortar porosity. However, in this case, the benefit in terms of thermal performance is the increment of the material thermal mass, resulting in the reduction and time-shifting of temperature peaks. Although this is a beneficial result for the energy behaviour of the building, it aims to a target that is different from current regulation requirements, which are set in terms of U-value. Moreover, PCM performance strongly depends on the environmental conditions of the building location, considerably affecting the strategy’s effectiveness according to the specific climate.
3.3.3 Geopolymers
The adoption of geopolymer mortars within the framework of building retrofit has been proposed in (Longo et al., 2020a; Longo et al., 2020b). The term geopolymer is applied to a class of solid materials that are obtained by the reaction of an aluminosilicate powder within an alkaline solution (Provis and van Deventer, 2009). In this way, it is possible to generate binders alternative to Portland cement by alkaline activation, commonly using fly ash to reduce the carbon footprint of concrete, thus obtaining an environmentally friendly product (Gkournelos et al., 2022). Thanks to their good thermal properties, geopolymers exhibit a considerable potential for application as thermal insulators, thus allowing them to achieve acceptable mechanical properties and reduce thermal conductivity. However, the latter is not comparable to that of thermal insulators, thus requiring the addition of better-performing materials, even if it allows the reduction of the overall thickness of the retrofitting system given the better thermal properties of geopolymers compared to those of ordinary mortars.
However, geopolymer mortars exhibit lower workability when compared to cement ones, typically requiring the addition of admixtures. The setting time is also very short, making more difficult the application procedure on-site. Secondly, the geopolymerization process is very sensitive, greatly influencing the material final performance. Increased quality control is thus fundamental, as well as a proper selection of the geopolymer components. Moreover, the curing process of geopolymer may produce a considerable quantity of salts if the chemical constituents are not carefully evaluated (Miranda et al., 2017). Such salts have a damaging impact not only on the existing substrate, especially for historic masonry, but may also involve hazards to human health. Such risk should be prevented by accurate dosage both in the design and execution phases.
3.4 Other solutions
Other possible approaches for the integrated renovation of the existing building stock can be found in literature, where most of them have features that can be further developed and improved to increase their benefits in practical applications. Nevertheless, in some cases, such proposals are aimed at specific types of buildings, making them difficult to be applied to a wide set of constructions.
Instead of additional structures, a possible retrofitting intervention from the outside of the building with reduced invasiveness can be performed by substituting infill masonry walls in RC frames with panels made of Autoclaved Aerated Concrete (AAC) blocks having a dry density between 4 and 6 kN/m3 with a correspondent thermal conductivity between 0.100 and 0.150 W m−1K−1, respectively (Artino et al., 2019). From a seismic viewpoint, these panels have a deadweight lower than that of bricks, so providing a reduction of the seismic mass. However, compared to traditional infill brick walls, the dynamic response of RC buildings with AAC panels was different due to the diverse increase of the base RC structure stiffness and, therefore, leading to a different vibration period and stress concentrations on both beam-to-column joints and foundations. From energy viewpoints, the low thermal conductivity and high fire resistance of AAC block panels make this solution very attractive for infill walls of RC framed buildings. However, this technique allows achieving slight improvements only in terms of seismic capacity and thermal efficiency, so not always satisfactory for ordinary RC buildings.
Similarly, Manfredi and Masi (2018) studied the replacement of the external layers of existing infill walls made of hollow bricks with cored bricks panels and an additional insulator, namely glass wool. This solution, specifically developed for RC infilled frames, allowed to achieve a thermal transmittance of the envelope lower than the threshold limit for the climatic zone in which the considered building was located. Furthermore, thanks to the better mechanical properties of cored bricks compared to hollow ones, the new infill walls presented a higher resistance against horizontal seismic loads with respect to the original configuration. However, as for AAC blocks, the thermal efficiency and seismic capacity improvement may not be sufficient to achieve minimum requirements, according to the building type and the current conditions of the construction, as well as the thermal transmittance minimum values and the seismic hazard due to the specific location.
As an alternative, de Sousa et al. (2021) proposed that infill masonry walls can be substituted by composite sandwich panels made of steel fibre reinforced micro-concrete layers and a polystyrene core to be adopted for seismic and energy purposes, respectively. With this latter solution, the obtained structural and thermal performances may allow achieving the targets set by current standards, but such an approach still presents some limitations. As a matter of fact, it involves partial demolition of existing elements with consequent production of additional waste. Moreover, being specifically developed for RC frames, it does not seem feasible for masonry buildings.
4 Current challenges and open issues
Among general needs for integrated refurbishment, there are specific aspects that a retrofitting solution should be able to address. Depending on the context and the involved requirements, special care should be paid for instance to the possible heritage value of historic constructions. Moreover, an accurate assessment of the costs and benefits of a retrofitting intervention compared to demolition and reconstruction of the building is necessary to find the best solution in terms of performance and sustainability as to define the most suitable choice.
4.1 European building stock needs
A fundamental aspect of the renovation of existing buildings is their characterization before planning the intervention. A preliminary assessment of current conditions is essential not only for specific constructions but also for the building stock in general. To study and develop a suitable retrofitting solution, it is necessary to draw a comprehensive picture of existing construction typologies and their deficiencies from the structural and energy points of view. In this way, a proper and calibrated strategy for their refurbishment can address deficiencies in an effective and targeted manner, promoting at the same time a deep renovation towards a safer and more sustainable building stock (Gladushenko, 2021; Jiménez-Pulido et al., 2021).
Based on these premises, to evaluate the seismic and energy performances of European buildings, the first thing to do is to classify the building stock in terms of structural technology and envelope. This has been done through a comprehensive analysis of literature data (Landolfo et al., 2022), leading to the maps presented in Figure 11. From a structural viewpoint, it has been noticed that the highest percentages of European building stocks are represented by reinforced concrete framed and masonry brick and rubble stone constructions. On the other hand, the distribution of European-built heritage envelopes in terms of infill walls, depicted in Figure 11B11, has shown that the most diffused infill wall type is represented by hollow brick masonry walls.
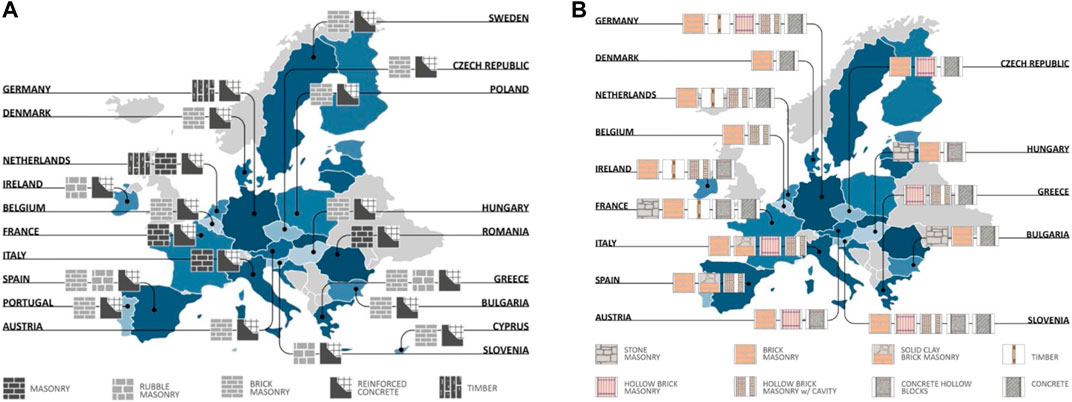
FIGURE 11. European building stock: (A) Building construction technologies map based on quantitative and qualitative data; (B) Typical infill walls (Landolfo et al., 2022).
4.2 Heritage buildings
Conservation of European cultural heritage buildings and historical centres with artistic-cultural and/or historic value is a pressing need for designers and researchers. This issue is very important to give the necessary attention to the cultural heritage, which represents a token of human artistic and social identity, and to allow for the repopulation of minor historical centres, which are subjected to abandonment in the last years. Therefore, the resistance against seismic actions and energy adequacy are the main issues considered by the European Union for the preservation, requalification, and upgrading of historic built heritage.
The earthquake safety of existing built heritage is increasingly important, especially in the European countries with higher seismic hazard, like Italy, where recent earthquakes of large magnitudes occurred in the last recent years (Mw 6.3 L’Aquila earthquake in 2009; Mw 6.0 Emilia-Romagna earthquake in 2012; Mw 6.6 Central Italy earthquake in 2016) and caused injuries and loss of human lives Cattari et al. (2012), Indirli et al. (2013), Fiorentino et al. (2017). In this country, the number of victims was higher than that occurred in other areas subjected to earthquakes of greater magnitude due to the presence of ancient and degraded buildings with historic value, which were designed without anti-seismic criteria.
Energy efficiency is also considered a relevant task for the sustainable conservation of historic heritage. In this framework, it is noteworthy to mention the revised version of the European Directive 2012/27/EU, whose directives proposed predefined energy targets aimed at amending the Energy Performance of Buildings Directive (EPBD) devoted to addressing energy efficacy and low-carbon measures to reduce climate change effects on the historic buildings, which are responsible for about 38% of the final energy demand (Directive EU, 2018; Artino et al., 2019; Basiricò and Enea, 2018).
Historic constructions need dedicated solutions, where each case is unique, due to problems faced, conservation issues, and the variety of building fabric. It is worth noting that heritage building safeguarding is the main goal of any retrofitting intervention to be carried out on such a type of construction while achieving a high performance after refurbishment is a secondary aspect, but both should be harmonized as much as possible. Besides several multidisciplinary approaches for the combined assessment of historic buildings that can be found in the literature (Ascione et al., 2017; Güleroğlu et al., 2020), Milone et al. (2015) proposed an interesting concept for the decision-making process based on Best Availability Technologies (BAT) and Allowed Best Technologies (ABT). A compromise between the best performance that can be achieved with the best solutions provided by the state-of-.the-art and the need for preservation and compatibility with the cultural heritage should be found.
Since most historic buildings have bad performances from seismic and energy perspectives, an integrated renovation approach is often strongly recommended. Instead, independent approaches are usually employed to cover the energy and structural lacks of buildings. Interventions like these may result in time- and cost-consuming and/or poorly effective. Contrary, with a unique intervention, the requalification of the built heritage from seismic and energy viewpoints can be attained.
One of the most effective integrated interventions applied outside cultural heritage buildings is given by TRM (Longo et al., 2021), although it may be considered as a borderline technique for some historic constructions. In the past, materials made of Fibre Reinforced Polymers (FRP) emerged as a valuable structural option for the retrofitting of buildings, but FRP materials showed incapacity to be applied on wet substrates, poor fire resistance, and low reversibility, the latter being responsible for inapplicability on masonry cultural heritage substrates (Valluzzi et al., 2014). Another possibility for historical construction is given by steel exoskeletons, which can be applied from the outside of the buildings with minimum invasiveness and allow to preserve the heritage value of the construction as much as possible. Nevertheless, as already mentioned, the use of exoskeletons entails the increase of the global dimensions of the existing building, while urban regulations often do not allow it, and this is particularly important for building aggregates in historical city centres (Angiolilli et al., 2021), where this solution is hardly feasible.
4.3 Refurbishment vs. demolition
Once identified the most recurrent structural technologies of buildings, together with their envelope types, there are two possible intervention strategies to reduce energy deficits and seismic vulnerabilities of the existing built heritage: (a) retrofitting operations; (b) total or partial demolition and reconstruction. Generally, the first option implies lower embodied energy and global warming potential impacts, so it is preferable both from a sustainability point of view and to avoid interruption of use. Contrary, demolition and reconstruction should be preferred only when retrofitting alternatives are not economically and technically convenient (Fiore et al., 2017; Pozza et al., 2021).
In terms of environmental sustainability, the demolition and disposal phases make the reconstruction interventions more burdensome than recovery interventions of existing buildings. However, in the case of destruction and reconstruction, it is necessary to consider the possible benefits deriving from recycling and the reuse of materials resulting from demolition. In recent years the Life Cycle Assessment (LCA) methodology, initially used to assess the environmental impact of materials or individual building components, has been extended to the analysis of entire buildings. One of the most successful results (Asdrubali et al., 2013) indicates that the most impactful phase of the building life cycle is related to its use, with an impact from 77% to 85%. Contrary, the impact of the construction phase ranges from 14% to 21%, while the end-of-life phase contributes up to an impact maximum percentage of 4%, with values usually in the range from 1% to 2%. A modification of this latter percentage could come from recycling and reusing of waste materials. Nevertheless, although recovering of aggregate wastes can give greater sustainability to the building substitution processes, at present, this benefit is still much limited. In fact, recycled aggregates are mostly used either for filling operations, in order to replace the drainage material of quarries, or for screeds and substrates, while reuse for quality applications is still at an unsatisfactory level, despite the objectives set by the European directives and the important research initiatives carried out in recent years (Formisano et al., 2020b; Davino et al., 2022).
Contrary, the convenience of demolition and reconstruction instead of retrofit interventions in seismic terms is a more complicated issue. In fact, structural reinforcement is usually more complex than the ex-novo design for several reasons, such as often limited knowledge of the structure, difficulties of logistical nature during diagnosis, doubtful presence of the effects of past earthquakes or other accidental actions and errors, and/or negligence that occurred during work execution.
The extent of the interventions on the structural parts is linked to both the current state of damage and the seismic vulnerability degree of buildings, as well as to the structural safety level to be achieved in compliance with the requirements imposed by the technical standards. It should also be noted that the seismic risk has strong implications on the environmental sustainability of buildings. Belleri and Marini (2016) showed that, especially in medium-high seismic hazard areas, integrated approaches towards energy requalification and structural upgrading are more sustainable than alternatives of either demolition-reconstruction or energy requalification only of the building. In fact, in the latter case, the advantages both in terms of cost and environmental impact could be completely nullified from the extent of the expected damage because of seismic events.
5 Concluding remarks
Seismic and energy retrofit of aged constructions has become a priority in modern societies to make the existing building stock safer and improve the interior conditions for inhabitants’ comfort, as well as reducing the environmental impact associated with structures. In the past, several solutions have been used in practice but the integration among the two components has limited their effectiveness and made them more expensive. Starting from the current need for a holistic approach to refurbishment, this study aimed at highlighting the possible benefits of an integrated solution combining seismic and energy retrofit in a unique intervention. Besides possible higher performances involved by a harmonious combination of seismic and energy retrofits, it is possible to perform less time-demanding interventions and reduce retrofitting costs, even considering the lower payback period of the investment.
However, some limitations of integrated strategies have prevented their application in practice, thus fostering the study and development of better solutions to solve such issues. It has been shown that this research topic is taking increasing attention among researchers, who are trying to integrate such aspects apparently incompatible and usually conflicting into a holistic solution. Even if many difficulties and problems still remain, this work provides an overview of open issues and possible promising solutions that can be further improved to overcome the defects and obstacles that are still preventing their extensive implementation in practical applications. Different proposals have tried to fill in the research gaps that still exist by adopting several techniques and materials, either more traditional or innovative. Since this is a really emerging research field, new solutions and ideas are being continuously proposed thus enriching the current overview and the possible available options.
Specific questions have been discussed as well, given the several difficulties that certain conditions may involve in the design process of a retrofitting intervention. The refurbishment of heritage buildings has been considered to underline the importance of their preservation, as well as their management for future use, making necessary a careful balance between such aspects in the designer’s choices.
In the case of ordinary buildings, another option is available instead of retrofitting, namely demolition and reconstruction. In the latter case, economic and technical reasons may lead to preferring this one over more complex refurbishment interventions. However, most of the time such a drastic decision involves high environmental impacts, producing more waste, and using more resources. The environmental issue is becoming increasingly important also in the construction sector given the unacceptable price that is being paid worldwide, being necessary to address it in retrofitting processes.
Author contributions
DO contributed to the conception and design of the study, being approved by other authors (NA, AF, LP). All authors (NA, AF, LP, DO) were involved in the paper preparation, from the draft, final version of the paper, revision, reading, corrections, approval, and submitted version.
Funding
Authors acknowledge the support through project NORTE-01-0145-FEDER-000058, cofounded by the European Regional Development Fund (FEDER) through Norte Regional Operational Programme, and grant agreement 2022.11827.BD, provided by the Portuguese Foundation for Science and Technology. This work was partly financed by FCT/MCTES through national funds (PIDDAC) under the R&D Unit Institute for Sustainability and Innovation in Structural Engineering (ISISE), under reference UIDB/04029/2020.
Conflict of interest
The authors declare that the research was conducted in the absence of any commercial or financial relationships that could be construed as a potential conflict of interest.
The handling editor declared a past collaboration with the author AF.
Publisher’s note
All claims expressed in this article are solely those of the authors and do not necessarily represent those of their affiliated organizations, or those of the publisher, the editors and the reviewers. Any product that may be evaluated in this article, or claim that may be made by its manufacturer, is not guaranteed or endorsed by the publisher.
References
Ademovic, N., Hadzima-Nyarko, M., and Zagora, N. (2022). Influence of site effects on the seismic vulnerability of masonry and reinforced concrete buildings in Tuzla (Bosnia and Herzegovina). Bull. Earthq. Eng. 20, 2643–2681. doi:10.1007/s10518-022-01321-2
Ademović, N., Hadzima-Nyarko, M., and Zagora, N. (2020). Seismic vulnerability assessment of masonry buildings in Banja Luka and Sarajevo (Bosnia and Herzegovina) using the macroseismic model. Bull. Earthq. Eng. 18 (8), 3897–3933. doi:10.1007/s10518-020-00846-8
ADENE (2022). Guia Prático do Certificado Energético da Habitação. greenplan.Pt. Availableat: http://www.greenplan.pt/ficheiros_artigos/Guia%20Pr%C3%A1tico%20do%20Certificado%20Energ%C3%A9tico.pdf (Accessed June 1, 2022).
Aguilar-Meléndez, A., Pujades, L. G., Barbat, A. H., Monterrubio-Velasco, M., de la Puente, J., and Lantada, N. (2022). Comparative analysis of a new assessment of the seismic risk of residential buildings of two districts of Barcelona. Nat. Hazards (Dordr). 110, 1649–1691. doi:10.1007/s11069-021-05006-y
Alpanda, S., and Peralta-Alva, A. (2010). Oil crisis, energy-saving technological change and the stock market crash of 1973–74. Rev. Econ. Dyn. 13 (4), 824–842. doi:10.1016/J.RED.2010.04.003
Angiolilli, M., Lagomarsino, S., Cattari, S., and Degli Abbati, S. (2021). Seismic fragility assessment of existing masonry buildings in aggregate. Eng. Struct. 247, 113218. doi:10.1016/j.engstruct.2021.113218
Artino, A., Evola, G., Margani, G., and Marino, E. M. (2019). Seismic and energy retrofit of apartment buildings through autoclaved aerated concrete (AAC) blocks infill walls. Sustainability 11, 3939. doi:10.3390/su11143939
Ascione, F., Ceroni, F., De Masi, R. F., de’ Rossi, F., and Pecce, M. R. (2017). Historical buildings: Multidisciplinary approach to structural/energy diagnosis and performance assessment. Appl. Energy 185 (2), 1517–1528. doi:10.1016/j.apenergy.2015.11.089
Asdrubali, F., Baldassarri, C., and Fthenakisb, V., (2013). “Life cycle analysis in the construction sector: Guiding the optimization of conventional Italian buildings”, Energy Build. 64, 73–89. doi:10.1016/j.enbuild.2013.04.018
Avila-Haro, J. A., Gonzalez-Drigo, R., Vargas-Alzate, Y. F., Pujades, L., and Barbat, A. (2022). Probabilistic seismic assessment of a high-rise URM building. J. Build. Eng. 45, 103344. doi:10.1016/j.jobe.2021.103344
Azizinamini, A., Pavel, R., Hatfield, E., and Gosh, S. K. (1990). Behavior of lap-spliced reinforcing bars embedded in high strength concrete. ACI Struct. J. 96 (5), 826–836.
Basiricò, T., and Enea, D. (2018). Seismic and energy retrofit of the historic urban fabric of Enna (Italy). Sustainability 10 (4), 1138. doi:10.3390/su10041138
Belleri, A., and Marini, A. (2016). Does seismic risk affect the environmental impact of existing buildings? Energy Build. 110, 149–158. doi:10.1016/j.enbuild.2015.10.048
Bilgin, M. (2010). New energy order and FAST principles: Premises of equitable and sustainable energy security in the 21st century. Int. J. Glob. Energy Issues 33 (1), 4–21. doi:10.1504/IJGEI.2010.033012
Boermans, T., and Petersdorff, C. (2007). U-Values for better energy performance of buildings. Eurima, Brussels: Eurima/Ecofys.
Bogdan, A., and Tudor, C. (2011). A comparative analysis of the energy performance certificates schemes within the European Union: Implementing options and policy recommendations. ECEEE 2011 Summer Study Conf. Energy Effic. first Found. a low-carbon Soc, 595–604.
Borri, A., Corradi, M., Sisti, R., Buratti, C., Belloni, E., and Moretti, E. (2015). Masonry wall panels retrofitted with thermal-insulating GFRP reinforced jacketing. Mat. Struct. 49 (10), 3957–3968. doi:10.1617/s11527-015-0766-4
Borri, A., Sisti, R., and Corradi, M. (2021). Seismic retrofit of stone walls with timber panels and steel wire ropes. Proc. Institution Civ. Eng. - Struct. Build. 174 (5), 359–371. doi:10.1680/jstbu.19.00100
Bournas, D., and Pohoryles, D. (2021). iRESIST+ - innovative seismic and energy retrofitting of the existing building stock: numerical simulations and impact assessment. Publ. Off. 24, 1544. doi:10.2760/496388
Bournas, D. A. (2018). Concurrent seismic and energy retrofitting of RC and masonry building envelopes using inorganic textile-based composites combined with insulation materials: A new concept. Compos. Part B Eng. 148, 166–179. doi:10.1016/j.compositesb.2018.04.002
Bournas, D. A., Pavese, A., and Tizani, W. (2015). Tensile capacity of FRP anchors in connecting FRP and TRM sheets to concrete. Eng. Struct. 82, 72–81. doi:10.1016/j.engstruct.2014.10.031
Brandner, R., Flatscher, G., Ringhofer, A., Schickhofer, G., and Thiel, A. (2016). Cross laminated timber (CLT): Overview and development. Eur. J. Wood Prod. 74, 331–351. doi:10.1007/s00107-015-0999-5
Brown, S. P. A., and Huntington, H. G. (2017). OPEC and world oil security. Energy Policy 108, 512–523. doi:10.1016/J.ENPOL.2017.06.034
Buildings Performance Institute Europe (Bpie) (2011). “Europe’s buildings under the microscope. A country-by-country review of the energy performance of buildings,” in Buildings performance Institute Europe: Brussels, Belgium. Editor M. Economidou. Available: https://bpie.eu/wp-content/uploads/2015/10/HR_EU_B_under_microscope_study.pdf (Accessed May 28, 2022).
Busselli, M., Cassol, D., Prada, A., and Giongo, I. (2021). Timber based integrated techniques to improve energy efficiency and seismic behaviour of existing masonry buildings. Sustainability 13, 10379. doi:10.3390/su131810379
Calvi, G. M., Sousa, L., and Ruggeri, C. (2016). Energy efficiency and seismic resilience: A common approach. Multi-Hazard Approaches Civ. Infrastructure Eng., 165–208. doi:10.1007/978-3-319-29713-2_9
Caprino, A., Lorenzoni, F., Carnieletto, L., Feletto, L., De Carli, M., and da Porto, F. (2021). Integrated seismic and energy retrofit interventions on a URM masonry building: The case study of the former courthouse in Fabriano. Sustainability 13, 9592. doi:10.3390/su13179592
Cattari, S., Degli Abbati, S., Ferretti, D., Lagomarsino, S., Ottonelli, D., Rossi, M., et al. (2012). The seismic behaviour of ancient masonry buildings after the earthquake in Emilia (Italy) on May 20th and 29th. Ing. Sismica 29 (2), 87–119.
Chieffo, N., and Formisano, A. (2020). Induced seismic-site effects on the vulnerability assessment of a historical centre in the Molise region of Italy: Analysis method and real behaviour calibration based on 2002 earthquake. Geosciences 10 (1), 21. doi:10.3390/geosciences10010021
Chieffo, N., and Formisano, A. (2019). The influence of geo-hazard effects on the physical vulnerability assessment of the built heritage: An application in a district of Naples. Buildings 9 (1), 26. doi:10.3390/buildings9010026
COM/2019/640 (2019). Communication from the commission to the European parliament, the European Council, the Council, the European economic and social committee and the committee of the regions: The European green deal. Brussels: Publications Office. Available at: https://eur-lex.europa.eu/legal-content/EN/TXT/?qid=1576150542719&uri=COM%3A2019%3A640%3AFIN.
Corrado, V., Ballarini, I., Corgnati, S. P., and Talà, N. (2011). Building typology brochure – Italy. Fasc. sulla Tipologia Edil. Ital. Politecnico Torino, 1–117.
Costanzo, E., Federici, A., Martino, A., Antinucci, M., and Varalda, G. (2016). Implementation of the EPBD in Italy. Available: https://epbd-ca.eu/ca-outcomes/outcomes-2015-2018/book-2018/countries/italy (Accessed June 1, 2022).
Council of the European Union (1974). Council Resolution of 17 December 1974 concerning Community energy policy objectives for 1985. (OJ C, C/153, 09.07.1975, p. 2, CELEX: https://eur-lex.europa.eu/legal-content/EN/TXT/?uri=CELEX:31975Y0709(02) (Accessed May 28, 2022)).
CPR 305/2011/Eu (2011). Regulation (EU) No 305/2011 of the European Parliament and of the Council of 9 March 2011 laying down behavior conditions for the marketing of construction products and repealing Council Directive 89/106/EEC. Off. J. Eur. Union 88, 5–43. Available at: http://data.europa.eu/eli/reg/2011/305/oj (Accessed June 2, 2022).
Crowley, H., Despotaki, V., Silva, V., Dabbeek, J., Romao, X., Pereira, N., et al. (2021). Model of seismic design lateral force levels for the existing reinforced concrete European building stock. Bull. Earthq. Eng. 19 (7), 2839–2865. doi:10.1007/s10518-021-01083-3
D'Urso, S., and Cicero, B. (2019). From the efficiency of nature to parametric design. A holistic approach for sustainable building renovation in seismic regions. Sustainability 11, 1227. doi:10.3390/su11051227
Dalla Mora, T., Righi, A., Peron, F., and Romagnoni, P. (2015). Functional, energy and seismic retrofitting in existing building: An innovative system based on xlam technology. Energy Procedia 82, 486–492. doi:10.1016/j.egypro.2015.11.851
Davino, A., Meglio, E., and Formisano, A. (2022). Lime-based plaster reinforced with hemp braids as sustainable building product. Architecture 2, 135–156. doi:10.3390/architecture2010008
De Berardinis, P., Rotilio, M., Marchionni, C., and Friedman, A. (2014). Improving the energy-efficiency of historic masonry buildings. A case study: A minor centre in the Abruzzo region, Italy. Energy Build. 80, 415–423. doi:10.1016/j.enbuild.2014.05.047
De Luca, F., Woods, G. E. D., Galasso, C., and D’Ayala, D. (2018). RC infilled building performance against the evidence of the 2016 EEFIT central Italy post-earthquake reconnaissance mission: Empirical fragilities and comparison with the FAST method. Bull. Earthq. Eng. 16, 2943–2969. doi:10.1007/s10518-017-0289-1
de Sousa, C., Barros, J. A. O., Correia, J. R., and Valente, T. D. S. (2021). Development of sandwich panels for multi-functional strengthening of RC buildings: Characterization of constituent materials and shear interaction of panel assemblies. Constr. Build. Mater. 267, 120849. doi:10.1016/j.conbuildmat.2020.120849
Di Lorenzo, G., Colacurcio, E., Di Filippo, A., Formisano, A., Massimilla, A., and Landolfo, R. (2020). State of the Art on steel exoskeletons for seismic retrofit of existing RC buildings. Ing. Sismica 37 (1), 33–50.
Directive EU. (2018). Directive (EU) 2018/2002 of the European parliament and of the Council of 11 december 2018 amending directive 2012/27/EU on energy efficiency”, Available: http://data.europa.eu/eli/dir/2010/31/2021-01-01 (Accessed May 25, 2022).
Directive 2012/27/EU. (2012). Directive 2012/27/EU of the European Parliament and of the Council of 25 October 2012 on energy efficiency. Amend. Dir. 2009/125/EC 2010/30/EU repealing Dir. 2004/8/EC and 2006/32/EC, 2012”, Available: http://data.europa.eu/eli/dir/2012/27/oj (Accessed May 23, 2022).
Donaire-Avila, J., Benavent-Climent, A., Escobedo, A., Oliver-Saiz, E., Ramírez-Márquez, A. L., and Feriche, M. (2012). Damage assessment on building structures subjected to the recent near-fault earthquake in Lorca (Spain) 15th World Conference on Earthquake Engineering. Lisbon.
Economidou, M., Todeschi, V., Bertoldi, P., Agostino, D., Zangheri, P., and Castellazzi, L. (2020). Review of 50 years of EU energy efficiency policies for buildings. Energy Build. 225, 110322. doi:10.1016/j.enbuild.2020.110322
Feroldi, F., Marini, A., Belleri, A., Passoni, C., Riva, P., Preti, M., et al. (2014). Sustainable seismic retrofit of modern RC buildings through an integrated structural, energetic and architectural approach adopting external engineered double skin façades. Progett. Sismica 5, 31–47.
Ferrante, A., Mochi, G., Predari, G., Badini, L., Fotopoulou, A., Gulli, R., et al. (2018). A European project for safer and energy efficient buildings: Pro-GET-onE (proactive synergy of inteGrated efficient technologies on buildings’ envelopes). Sustainability 10, 812. doi:10.3390/su10030812
Fiore, P., Donnarumma, G., and Sicignano, C. (2017). “Refurbishment vs. demolition and reconstruction: Analysis and evaluation in order to choose the intervention, Proceedings of the 2017 Colloqui.AT.e Conference “Demolition or Reconstruction?”, Ancona, 28-29 September 2017. ”Edicom editor. Editors G. Bernardini, and E. Di Giuseppe (Gorizia, Italy, 964–975.
Fiorentino, G., Forte, A., Pagano, E., Sabetta, F., Baggio, C., Lavorato, D., et al. (2017). Damage patterns in the town of Amatrice after august 24th, 2016 central Italy earthquakes. Bull. Earthq. Eng. 16 (3), 1399–1423. doi:10.1007/s10518-017-0254-z
Formisano, A., Chiumiento, G., and Dessì, E. J. (2020). Laboratory tests on hydraulic lime mortar reinforced with jute fibres. Open Civ. Eng. J. 14 (1), 152–162. doi:10.2174/1874149502014010152
Formisano, A., Massimilla, A., Di Lorenzo, G., and Landolfo, R. (2020). Seismic retrofit of gravity load designed RC buildings using external steel concentric bracing systems. Eng. Fail. Anal. 111, 104485. doi:10.1016/j.engfailanal.2020.104485
Formisano, A., Vaiano, G., and Fabbrocino, F. (2019). A seismic-energetic-economic combined procedure for retrofitting residential buildings: A case study in the province of Avellino (Italy). AIP Conf. Proc. 2116, 420008–420018. doi:10.1063/1.5114435
Fragoso, R., and Baptista, N. (2016). Implementation of the EPBD in Portugal. Available: https://epbd-ca.eu/ca-outcomes/outcomes-2015-2018/book-2018/countries/portugal (Accessed June 1, 2022).
Georgescu, E. S., Georgescu, M., Macri, Z., Marino, E., Margani, G., Meita, V., et al. (2018). Seismic and energy renovation: A review of the code requirements and solutions in Italy and Romania. Sustainability 10 (5), 1561. doi:10.3390/su10051561
Gkournelos, P. D., Azdejkovic, L. D., and Triantafillou, T. C. (2022). Innovative and eco-friendly solutions for the seismic retrofitting of natural stone masonry walls with textile reinforced mortar: In- and out-of-plane behavior. J. Compos. Constr. 26 (1), 04021061. doi:10.1061/(ASCE)CC.1943-5614.0001173
Gkournelos, P. D., Triantafillou, T. C., and Bournas, D. A. (2020). Integrated structural and energy retrofitting of masonry walls: Effect of in-plane damage on the out-of-plane response. J. Compos. Constr. 24 (5), 04020049. doi:10.1061/(ASCE)CC.1943-5614.0001066
Gkournelos, P. D., Triantafillou, T. C., and Bournas, D. A. (2021). Seismic upgrading of existing reinforced concrete buildings: A state-of-the-art review. Eng. Struct. 240, 112273. doi:10.1016/j.engstruct.2021.112273
Gladushenko, R. (2021). Deep renovation: The new normal. Euractive, 36. Available: https://www.euractiv.com/section/energy-environment/opinion/deep-renovation-the-new-normal/(Accessed June 3, 2022).
Güleroğlu, S. K., Karagüler, M. E., Kaharaman, I., and Umdu, E. S. (2020). Methodological approach for performance assessment of historical buildings based on seismic, energy and cost performance: A mediterranean case. J. Build. Eng. 31, 101372. doi:10.1016/j.jobe.2020.101372
Hsu, T., and Mansour, M. (2005). Stiffness, ductility, and energy dissipation of RC elements under cyclic shear. Earthq. Spectra 21 (4), 1093–1112. doi:10.1193/1.2044828
IEA-UNDP (2013). Modernising building energy codes to secure our global energy future. Available at: https://www.iea.org/reports/policy-pathway-modernising-building-energy-codes-2013 (Accessed May 28, 2022).
Illampas, R., Rigopoulos, I., and Ioannou, I. (2021). Influence of microencapsulated Phase Change Materials (PCMs) on the properties of polymer modified cementitious repair mortar. J. Build. Eng. 40, 102328. doi:10.1016/j.jobe.2021.102328
Indirli, M., Kouris, L. A. S., Formisano, A., Borg, R. P., and Mazzolani, F. M. (2013). Seismic damage assessment of unreinforced masonry structures after the Abruzzo 2009 earth-quake: The case study of the historical centers of L′Aquila and Castelvecchio Subequo. Int. J. Archit. Herit. 7, 536–578. doi:10.1080/15583058.2011.654050
Intelligent Service, B., and Lyons, R. (2013). Energy performance certificates in buildings and their impact on transaction prices and rents in selected EU countries. Final Rep. Prep. Eur. Comm. (DG Energy), 377. Available: https://energy.ec.europa.eu/document/download/64dce784-0bbe-40af-b141-924a7ecf7ac8_en?filename=20130619-energy_ performance_certificates_in_buildings.pdf (Accessed May 25, 2022).
Interm, D. (2015). Adeguamento linee guida nazionali per la certificazione energetica degli edifici. Available at: https://www.mise.gov.it/index.php/it/normativa/decreti-interministeriali/2032968-decreto-interministeriale-26-giugno-2015-adeguamento-linee-guida-nazionali-per-la-certificazione-energetica-degli-edifici (Accessed June 3, 2022).
Iuorio, O., Dauda, J. A., and Lourenço, P. B. (2021). Flexural resistance of masonry wall retrofitted with timber panel under out-of-plane loading. Int. Conf. SAHC 180, 577. doi:10.23967/sahc.2021.114
Izzi, M., Casagrande, D., Bezzi, S., Pasca, D., Follesa, M., and Tomasi, R. (2018). Seismic behaviour of cross-laminated timber structures: A state-of-the-art review. Eng. Struct. 170, 42–52. doi:10.1016/j.engstruct.2018.05.060
Jiménez-Pulido, C., Jiménez-Rivero, A., and García-Navarro, J. (2021). Strategies to promote deep renovation in existing buildings. Rethink. Sustain. Towards a Regen. Econ. Future City 15, 377–394. doi:10.1007/978-3-030-71819-0_21
Joint Research Center (Jrc) (2015). New European technical rules for the assessment and retrofitting of existing structures. in New European technical rules for the assessment and retrofitting of existing structures. Editors S. Dimova, A. Pinto, P. Luechinger, and S. Denton (Brussels, Belgium: Joint Research Center). doi:10.2788/095215
Kerdan, I. G., Raslan, R., and Ruyssevelt, P. (2015). “Parametric study and simulation-based exergy optimization for energy retrofits in buildings”, Proceedings of the ECOS 2015 Conference, Pau, France, March 12 2015.
La Greca, P., and Margani, G. (2018). Seismic and energy renovation measures for sustainable cities: A critical analysis of the Italian scenario. Sustainability 10, 254. doi:10.3390/su10010254
Labò, S., Passoni, C., Marini, A., Belleri, A., Camata, G., Riva, P., et al. “Diagrid solutions for a sustainable seismic, energy, and architectural upgrade of European RC buildings”, Proceedings of the XII International Conference on Structural Repair and Rehabilitation, Porto Portugal, 2016.
Landolfo, R., Formisano, A., Di Lorenzo, G., and Di Filippo, A. (2022). Classification of European building stock in technological and typological classes. J. Build. Eng. 45, 103482. doi:10.1016/j.jobe.2021.103482
Law, D. (2020). Incentives for energy efficiency, sisma bonus, photovoltaic and electric vehicle charging stations. Italy. Available at: https://www.gazzettaufficiale.it/eli/id/2020/05/19/20G00052/sg.
Lițiu, A. V., and Hogeling, A. (2021). The evolution of building energy performance assessment and certification schemes in Europe. REHVA J., 82–86.
Longo, F., Cascardi, A., Lassandro, P., and Aiello, M. A. (2020). A new fabric reinforced geopolymer mortar (FRGM) with mechanical and energy benefits. Fibers 8 (8), 49. doi:10.3390/fib8080049
Longo, F., Cascardi, A., Lassandro, P., and Aiello, M. A. (2021). Thermal and seismic capacity improvements for masonry building heritage: A unified retrofitting system. Sustainability 13, 1111. doi:10.3390/su13031111
Longo, F., Cascardi, A., Lassandro, P., Sannino, A., and Aiello, M. A. (2019). Mechanical and thermal characterization of FRCM-matrices. Key Eng. Mat. 817, 189–194. doi:10.4028/www.scientific.net/KEM.817.189
Longo, F., Lassandro, P., Moshiri, A., Phatak, T., Aiello, M. A., and Krakowiak, K. J. (2020). Lightweight geopolymer-based mortars for the structural and energy retrofit of buildings. Energy Build. 225, 110352. doi:10.1016/j.enbuild.2020.110352
Loonen, R. C. G. M. (2015). Bio-inspired adaptive building skins. Biotechnol. Biomimetics Civ. Eng., 115–134. doi:10.1007/978-3-319-09287-4_5
Lucchini, A., Mazzucchelli, E. S., Mangialardo, S., and Persello, M. (2014). Façadism and CLT technology: An innovative system for masonry construction refurbishment. IAHS World Congr. Hous. Portugal: Funchal. doi:10.13140/2.1.3510.8163
Manfredi, V., and Masi, A. (2018). Seismic strengthening and energy efficiency: Towards an integrated approach for the rehabilitation of existing RC buildings. Buildings 8 (3), 36. doi:10.3390/buildings8030036
Manfredi, V., Santarsiero, G., Masi, A., and Ventura, G. (2021). The high-performance dissipating frame (HPDF) system for the seismic strengthening of RC existing buildings. Sustainability 13, 1864. doi:10.3390/su13041864
Manos, G. C., Melidis, L., Katakalos, K., Kotoulas, L., Anastasiadis, A., and Chatziastrou, C. (2021). Masonry panels with external thermal insulation subjected to in-plane diagonal compression. Case Stud. Constr. Mater. 14, e00538. doi:10.1016/j.cscm.2021.e00538
Margani, G., Evola, G., Tardo, C., and Marino, E. M. (2020). Energy, seismic, and architectural renovation of RC framed buildings with prefabricated timber panels. Sustainability 12, 4845. doi:10.3390/su12124845
Marini, A., Passoni, C., Belleri, A., Feroldi, F., Preti, M., Metelli, G., et al. (2017). Combining seismic retrofit with energy refurbishment for the sustainable renovation of RC buildings, a proof of concept. Eur. J. Environ. Civ. Eng. 26, 2475–2495. doi:10.1080/19648189.2017.1363665
Marini, A., Passoni, C., Riva, P., Negro, P., Romano, E., and Taucer, F. (2014). Technology options for earthquake resistant, eco-efficient buildings in Europe: Research needs. Report EUR 26497 EN. JRC87425. Luxembourg: Publications Office of the European Union. doi:10.2788/68902
Mastroberti, M., Bournas, D., Vona, M., Manganelli, B., and Palermo, V. (2018). Combined seismic plus energy retrofitting for the existing RC buildings: Economic feasibility. 16th Eur. Conf. Earthq. Eng. Thessaloniki, Greece, 18–21.
Mazzarella, L. (2015). Energy retrofit of historic and existing buildings. The legislative and regulatory point of view. Energy Build. 95, 23–31. doi:10.1016/j.enbuild.2014.10.073
Menna, C., Felicioni, L., Negro, P., Lupíšek, A., Romano, E., Prota, A., et al. (2022). Review of methods for the combined assessment of seismic resilience and energy efficiency towards sustainable retrofitting of existing European buildings. Sustain. Cities Soc. 77, 103556. doi:10.1016/j.scs.2021.103556
Milone, D., Peri, G., Pitruzzella, S., and Rizzo, G. (2015). Are the Best Available Technologies the only viable for energy interventions in historical buildings? Energy Build. 95, 39–46. doi:10.1016/j.enbuild.2014.11.004
Minotto, M., Verlato, N., Donà, M., and da Porto, F. (2020). Strengthening of in-plane and out-of-plane capacity of thin clay masonry infills using textile- and fiber-reinforced mortar. J. Compos. Constr. 24 (6), 4020059. doi:10.1061/(ASCE)CC.1943-5614.0001067
Miranda, T., Silva, R. A., Oliveira, D. V., Leitão, D., Cristelo, N., Oliveira, J., et al. (2017). ICEBs stabilised with alkali-activated fly ash as a renewed approach for green building: Exploitation of the masonry mechanical performance. Constr. Build. Mater. 155, 65–78. doi:10.1016/j.conbuildmat.2017.08.045
Mistretta, F., Stochino, F., and Sassu, M. (2019). Structural and thermal retrofitting of masonry walls: An integrated cost-analysis approach for the Italian context. Build. Environ. 155, 127–136. doi:10.1016/j.buildenv.2019.03.033
Motra, G. B., and Paudel, S. (2021). Performance evaluation of strengthening options for institutional brick masonry buildings: A case study of Pulchowk Campus. Prog. Disaster Sci. 10, 100173. doi:10.1016/j.pdisas.2021.100173
Papadopoulos, A. M. (2016). Forty years of regulations on the thermal performance of the building envelope in Europe: Achievements, perspectives and challenges. Energy Build. 127, 942–952. doi:10.1016/j.enbuild.2016.06.051
Park, R. (1988). Ductility evaluation from laboratory and analytical testing. Proc. 9th World Conf. Earthq. Eng. - 9WCEE, Tokyo, Japan 8, 605–616.
Passoni, C., Guo, J., Christopoulos, C., Marini, A., and Riva, P. (2020). Design of dissipative and elastic high-strength exoskeleton solutions for sustainable seismic upgrades of existing RC buildings. Eng. Struct. 221, 111057. doi:10.1016/j.engstruct.2020.111057
Pertile, V., Stella, A., De Stefani, L., and Scotta, R. (2021). Seismic and energy integrated retrofitting of existing buildings with an innovative ICF-based system: Design principles and case studies. Sustainability 13, 9363. doi:10.3390/su13169363
Pohoryles, D. A., Maduta, C., Bournas, D. A., and Kouris, L. A. (2020). Energy performance of existing residential buildings in Europe: A novel approach combining energy with seismic retrofitting. Energy Build. 223, 110024. doi:10.1016/j.enbuild.2020.110024
Power, A. (2010). Housing and sustainability: Demolition or refurbishment? Proc. Institution Civ. Eng. - Urban Des. Plan. 163 (4), 205–216. doi:10.1680/udap.2010.163.4.205
Pozza, L., Degli Espositi, A., Bonoli, A., Talledo, D., Barbaresi, L., Semprini, G., et al. (2021). Multidisciplinary performance assessment of an eco-sustainable RC-framed skin for the integrated upgrading of existing buildings. Sustainability 13, 9225. doi:10.3390/su13169225
Priestley, M. (2000). Performance based seismic design. Proceedings of the 12th World Conference on Earthquake Engineering - 12WCEE. New Zealand: Auckland, 2831. doi:10.5459/bnzsee.33.3.325-346
Provis, J. L., and van Deventer, J. S. J. (2009). Geopolymers - structures, processing, properties and industrial applications. Woodhead Publ. Mater. 376, 10075.
Rakhshanimehr, M., Esfahani, M. R., Kianoush, M. R., Mohammadzadeh, A. B., and Roohollah Mousavi, S. (2014). Flexural ductility of reinforced concrete beams with lap-spliced bars. Can. J. Civ. Eng. 25, 594–604. doi:10.1139/cjce-2013-0074
Rojs (2017). Heat roadmap Europe. Heat. Cool. facts figures. Available: https://heatroadmap.eu/wp-content/uploads/2019/03/Brochure_Heating-and-Cooling_web.pdf (Accessed May 28, 2022).
Rosenow, J., Cowart, R., Bayer, E., and Fabbri, M. (2017). Assessing the European Union’s energy efficiency policy: Will the winter package deliver on ′efficiency first′? Energy Res. Soc. Sci. 26, 72–79. doi:10.1016/j.erss.2017.01.022
Scuderi, G. (2016). Building exoskeletons for the integrated retrofit of social housing. Civ. Eng. J. 2 (6), 226–243. doi:10.28991/cej-2016-00000029
Simo, E. (2003). National Institute for land and infrastructure management. Des. Guidel. High-Rise RC Frame-Wall Struct. Tokyo, Japan: Kaibundo, 53–85.
Smiroldo, F., Paviani, I., Giongo, I., Zanon, S., Albatici, R., and Piazza, M. (2021). An integrated approach to improve seismic and energetic behaviour of RC framed buildings using timber panels. Sustainability 13, 11304. doi:10.3390/su132011304
Stazi, F., Serpilli, M., Maracchini, G., and Pavone, A. (2019). An experimental and numerical study on CLT panels used as infill shear walls for RC buildings retrofit. Constr. Build. Mater. 211, 605–616. doi:10.1016/j.conbuildmat.2019.03.196
Sustersic, I., and Dujic, B. (2014). Seismic strengthening of existing concrete and masonry buildings with crosslam timber panels. Mater. Joints Timber Struct. RILEM Bookseries 9, 713–723. doi:10.1007/978-94-007-7811-5_64
Triantafillou, T. C., Karlos, K., Kapsalis, P., and Georgiou, L. (2018). Innovative structural and energy retrofitting system for masonry walls using textile reinforced mortars combined with thermal insulation: In-plane mechanical behavior. J. Compos. Constr. 22 (5), 04018029. doi:10.1061/(ASCE)CC.1943-5614.0000869
Triantafillou, T. C., Karlos, K., Kefalou, K., and Argyropoulou, E. (2017). An innovative structural and energy retrofitting system for URM walls using textile reinforced mortars combined with thermal insulation: Mechanical and fire behavior. Constr. Build. Mater. 133, 1–13. doi:10.1016/j.conbuildmat.2016.12.032
Triantafillou, T. C. (2016). Textile fibre composites in civil engineering. Woodhead Publ. Ser. Civ. Struct. Eng. 60. doi:10.1016/C2014-0-01415-3
Urquizo, J., Calderón, C., and James, P. (2019). Technical options for retrofitting the building stock. A case study from the United Kingdom. Proceedings of the ECOS 2019 - the 32nd International Conference on Efficiency, Cost, Optimization, Simulation and Environmental Impact of Energy Systems. June 23-28, 2019. Wroclaw, Poland. Available at: https://www.researchgate.net/publication/333132639_Technical_options_for_retrofitting_the_building_stock_A_case_study_from_the_United_Kingdom (Accessed June 1, 2022).
Valluzzi, M. R., Modena, C., and de Felice, G. (2014). Current practice and open issues in strengthening historical buildings with composites. Mat. Struct. 47, 1971–1985. doi:10.1617/s11527-014-0359-7
Valluzzi, M. R., Saler, E., Vignato, A., Salvalaggio, M., Croatto, G., Dorigatti, G., et al. (2021). Nested buildings: An innovative strategy for the integrated seismic and energy retrofit of existing masonry buildings with CLT panels. Sustainability 13, 1188. doi:10.3390/su13031188
Vaquero, P. (2020). Buildings energy certification system in Portugal: Ten years later. Energy Rep. 6, 541–547. doi:10.1016/j.egyr.2019.09.023
Vitiello, U., Asprone, D., Di Ludovico, M., and Prota, A. (2017). Life-cycle cost optimization of the seismic retrofit of existing RC structures. Bull. Earthq. Eng. 15 (5), 2245–2271. doi:10.1007/s10518-016-0046-x
Wallace, J. W., and Orakcal, K. (2002). ACI 318-99 provisions for seismic design of structural walls. ACI Struct. J. 99 (4), 499–508.
Keywords: integrated retrofit, seismic safety, energy efficiency, European building stock, heritage buildings, buildings environmental impact
Citation: Ademovic N, Formisano A, Penazzato L and Oliveira DV (2022) Seismic and energy integrated retrofit of buildings: A critical review. Front. Built Environ. 8:963337. doi: 10.3389/fbuil.2022.963337
Received: 07 June 2022; Accepted: 18 October 2022;
Published: 17 November 2022.
Edited by:
Luigi Sorrentino, Sapienza University of Rome, ItalyCopyright © 2022 Ademovic, Formisano, Penazzato and Oliveira. This is an open-access article distributed under the terms of the Creative Commons Attribution License (CC BY). The use, distribution or reproduction in other forums is permitted, provided the original author(s) and the copyright owner(s) are credited and that the original publication in this journal is cited, in accordance with accepted academic practice. No use, distribution or reproduction is permitted which does not comply with these terms.
*Correspondence: Naida Ademovic, naidadem@gmail.com