- School of Future Environments, Auckland University of Technology, Auckland, New Zealand
The construction industry’s inherent fragmentation fuels Construction Supply Chain (CSC) complexity. Logistics form an essential part of the CSC in terms of costs and project management. In turn, transportation accounts for more than half the logistics costs due to construction materials being low-cost/high-volume, and most other logistics processes being business processes and not physical ones. Construction transport is almost entirely road bound, one of the least sustainable modes with externalities far out of proportion to its numbers. Ergo, its optimisation presents substantial greening (de-carbonisation) opportunity, with potentially far-reaching sustainability impacts. The ASI (Avoid-Shift-Improve) concept considers activity, modal structure, and energy intensity/efficiency as transport carbon components, providing a fundamental optimisation framework. 93% of New Zealand’s freight (a third of it construction related) is road bound, with 99% fossil-fuel (diesel) dependence. Government adoption of ASI elements viz Supply Chain (SC) efficiency, transport optimisation, data/information sharing, and collaboration for transport decarbonisation aligns with Industry 5.0, but seeks further research for concrete implementation pathways. This paper aims to bridge the gap by proposing a research framework for greening of construction transport as a sustainability enabler for New Zealand.
Introduction
The construction sector typically contributes circa 13% to the global GDP (McKinsey and Company, 2017). It generates employment, creates and improves infrastructure, and supports business. Contributing substantially to socio-economic development, the sector’s operations exemplify significant resource consumption. As of 2019, the construction industry represented 35% of global energy consumption, in turn contributing 38% to global emissions (UNEP, 2020). These stem from upstream contributors viz embodied, including construction related energy (circa 5–36% for conventional buildings, and 10–83% for low-energy buildings) (Chastas et al., 2016), the rest being downstream recipients (Built Environment operation and maintenance) of the construction process. Figure 1 illustrates the Life Cycle Energy Analysis of a built asset.
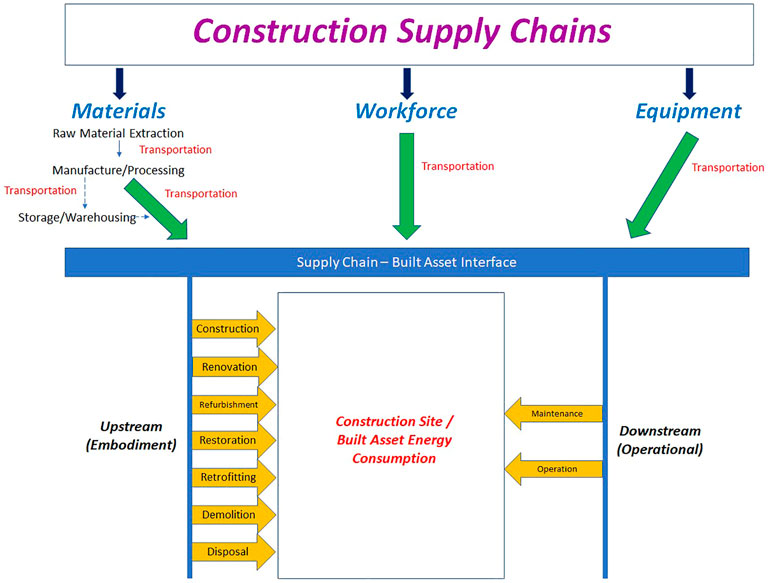
FIGURE 1. Life Cycle Energy Analysis of a built asset (Authors’ conception based on Cox and Ireland, 2002; Ramesh et al., 2010; Thomsen and van der Flier, 2011; Chastas et al., 2016).
The sector exhibits deep rooted fragmentation (Shakantu and Emuze, 2012; Guerlain et al., 2019b; Riazi et al., 2020; Jones et al., 2021). Fragmentation manifests at two levels viz at the Industry level through segregation from a relatively large number of small firms, and Project level due to disintegration of construction process and entities (Alashwal and Abdul-Rahman, 2014; Alashwal and Fong, 2015). Logistics is an interdisciplinary domain that forms a substantial component of the complex Construction Supply Chain (CSC) both in terms of project management and costs (Ying and Tookey, 2014). Precluding continuity and an integrated approach, fragmentation manifests as limited coordination and integration from ambiguous division of responsibility, leading to process inefficiencies (Alashwal and Fong, 2015). The resultant increased resource overheads create sustainability concerns. Comprised of diverse elements from transportation to warehousing and management of stocks (Szymonik, 2012, p. 12), logistics present substantial optimisation opportunity for the sector, through both strategic as well as operational mechanisms.
Transportation is the single largest element of logistics (Bowersox et al., 2002, pp. 32–34; Madadi et al., 2009), as a result of most other logistics processes except warehousing being business processes and not physical ones (Szymonik, 2012, p. 12). Construction materials being low cost/high volume compared to other industries (Lovell et al., 2006; Ying and Roberti, 2013; Balm and Ploos van Amstel, 2017; The Bellona Foundation, 2020), transportation forms a significant component of construction logistics. In addition to energy consumption, emissions, and costs (Smith et al., 2003; Szymonik, 2012; Ying et al., 2014), other transport externalities across sustainability domains may be direct (e.g., pollution, noise, congestion), or indirect (e.g., loss of ecosystems, health impacts, reduced quality of life) (Chatziioannou et al., 2020). Improved sustainability can, therefore, be achieved by optimising construction logistics through its transportation function.
Based on assessments undertaken by various agencies in the area of climate change, it is anticipated that transportation will contribute to approximately 60% of emissions by 2050 (IPCC, 2014a). Analysis also shows that freight transport is one of the most difficult sectors to decarbonise, not for lack of options, rather for challenges in deploying at the scale needed to achieve significant carbon reductions (Guerin et al., 2014; McKinnon, 2018).
New Zealand has peculiar physical attributes (Dani et al., 2022) viz its geographical isolation (Naismith et al., 2016), a deregulated market (Khan and Lockhart, 2019), sparse population, a low-density urban development strategy, and a resultant elongated sprawl with “urban villages” (Silva, 2019) strung out longitudinally along the two major islands. Logistics is the single most important domain keeping the country ticking, the end of an international logistics chain at its entry points only marking the beginning of extensive internal ones. The peculiar constraints typified in New Zealand restrict circa 93% freight movement to roads (a third of it construction related) with 99% fossil fuel (diesel) dependency. Market dynamics constrain improved technology, compounding the issue.
Freight transport decarbonisation is a high priority in the government’s climate change response for achieving New Zealand’s 2030/2050 carbon targets. The government’s strategy seeks 25% reduction in emissions from freight transport by 2035, amongst other objectives, towards greening/decarbonising freight transport (Ministry for the Environment, New Zealand Government, 2021). The strategy adopts the Avoid-Shift-Improve framework (Transformative Urban Mobility Initiative, 2019) and develops three themes to group together opportunities within this framework, with one of the three focussing on freight transport. It identifies Supply Chain (SC) efficiency, transport optimisation, data/information sharing, collaboration, and legislation/regulation as decarbonisation enablers, however, falling short of specific pathways, seeking further research to arrive at concrete implementation measures (Ministry of Transport, New Zealand Government, 2021). It is this gap that has provided the opportunity for the formulation of a research framework for implementation of the government’s strategy.
This study aims at suggesting a research framework for greening/decarbonisation of construction transport aligned to New Zealand realities based on the government’s adopted strategy. It is driven by the preponderance of road transport in construction logistics that supports optimisation, and the implicit onus of resolving construction logistics issues on the construction industry, rather than on those ordinarily responsible for managing city logistics (Janné and Fredriksson, 2019). Construction transport presents substantial sectoral sustainability improvement opportunities across a wide spectrum of initiatives from restructuring logistics at the strategic end to the introduction of cleaner transport technologies at the operational boundary.
Construction Logistics and Transport
The CSC adopts its characteristics from the nature of the construction industry viz Convergence of the SC directing all materials to the construction site, where the product (the built asset) is assembled; Setting up of the “manufacturing plant” around the single product (the built asset), unlike manufacturing, where multiple products pass through the manufacturing facility; A temporary SC involving repetitive reconfiguration of project organisations (causing instability, fragmentation, and delineation of design and construction) (Wegelius-Lehtonen, 2001; Dubois and Gadde, 2002; Naismith et al., 2016); and, A one-off and unique product, therefore, a “make-to-order” SC (Seth et al., 2018; Guerlain et al., 2019b; Riazi et al., 2020). The primary actors in the CSC are Owner, Architect or Consultant, Contractor, Sub-contractors, Suppliers, and User (Vrijhoef and Koskela, 2000).
Logistics provide a decision-making framework integrating inventory, transportation, warehousing, materials handling and industrial packaging, manifesting through physical flow, and management of goods/services and accompanying information, in an interdisciplinary environment. Major logistics activities are transportation (movement), storage (warehousing), industrial packaging, manipulation of materials, control of stocks, order fixing, demand forecasting, production planning, procurement, customer service, location of facilities, and management of waste. Logistics processes enable physical performance of logistics functions. “A logistics process may be considered to be an orderly time sequence of successive conditions and changes; a set of logically related activities and tasks, to achieve a business result; or, transformation of input data into output leading to change, considering value addition to a product or service. Logistics processes are specified temporally and spatially, in the field of the physical flow of goods/services, flow of associated information, and associated risks” (Szymonik, 2012, p. 23). Logistics, peculiar to the construction domain, may be defined as:-
- “…a process of strategically managing the procurement, movement and storage of materials, parts and finished inventory (and the related information flows) through the organisation and its marketing channels in such a way that current and future profitability are maximised through the cost-effective fulfilment of orders” (Wegelius-Lehtonen, 2001).
- “The scope of construction logistics concerns all supply and disposal shipments of building materials, construction equipment and construction personnel to and from the construction site. Construction logistics is aimed at getting the right equipment, the right materials and the right workers with the right level of quality to the right construction site at the right moment and at minimum cost” (Quak et al., 2011 (in Dutch) cited Balm and Ploos van Amstel, 2017).
- “Construction logistics management may be defined as the management of the process of delivering materials and resources required at a construction site in a productive way. It is not only the management of the flow of material and information, but includes also delivering quality, ensuring safety, and providing an environment that facilitates construction activities” (Ghanem et al., 2018).
Figure 2 illustrates the construction logistics domain in relation to the main actors.
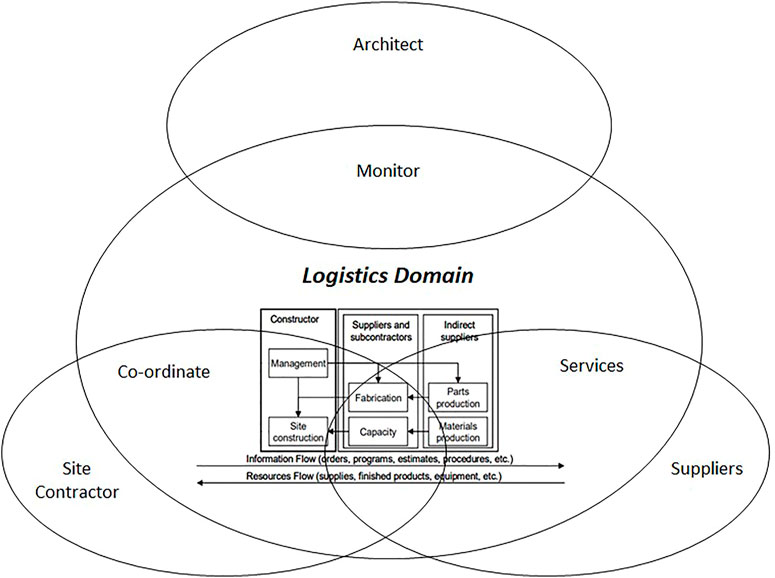
FIGURE 2. The Construction Logistics domain (Authors’ conception based on Vrijhoef and Koskela, 2000; Salagnac and Yacine, 1999).
Construction logistics involve the preparation, management, coordination, and control of product flow from raw material processing to final utilisation in the finished project and reverse logistics of waste removal and disposal (Agapiou et al., 1998; Ying and Tookey, 2014). The components of construction logistics are (Jang et al., 2003; Sobotka et al., 2005; Ying et al., 2018; Janné, 2020):-
- Whole-project Logistics Sees the building site through the lens of a production system. It is a member of many logistics chains, executing complex processes within the constraints of budget, space, and time.
- Supply Logistics Delivery of products from sources external to the construction site viz material and human resources for building activities. Supply logistics are comprised of supplier management, transportation, material resource procurement planning, processing, and maintenance of procured resources.
- On-site Logistics On-site material flow coordination internal to the construction site.
Construction logistics form part of complex systems with multiple stakeholders, and a wide range of simultaneous activities, processes, and systems in action, on- or off-site. A generic construction logistics model groups together planning and organisation (inter-organisational relationships), transportation, and site activities (Janné, 2020). Activities within these domains typically perform the roles of establishing clear SC - construction site interfaces, possible integration of the construction site with the SC, increasing efficiencies of the SC and site processes/activities, local stakeholder coordination, and value addition. Optimisation requires a very high degree of awareness and coordination/integration. Some of the major logistics challenges in construction are: Unclear division of responsibilities between the SC and the Site; SC inefficiency; Inefficient on-site logistics; and, Lack of coordination (Construction in the Vicinities Innovative Co-creation, 2018).
Construction characteristics distinctively influence logistics viz Each site, being unique and temporary, needs a new logistics setup (Wegelius-Lehtonen, 2001; Dubois and Gadde, 2002; Naismith et al., 2016); Sites being material intensive and supplied on an irregular basis (Kim and Nguyen, 2018); Construction activities being sequential, transmit delays, errors, and inefficiencies through all activities; and, Varying operational and management philosophies, and inefficient resource utilisation as a result of fragmentation of the industry (many construction companies, suppliers and Logistics Service Providers (LSPs) working in different temporary construction consortia) (Construction in the Vicinities Innovative Co-creation, 2018).
Transport is the largest component of logistics (Bowersox et al., 2002), a result of most other involved processes (except warehousing) being business processes and not physical ones (Szymonik, 2012, p. 12). The volume of construction material required for a project, its sustained delivery aligned to the site requirements, absence of planning and coordination/communication, typically small deliveries, low cost/high volume nature of construction materials, and transport externalities (Lovell et al., 2006; Ying and Roberti, 2013; Balm and Ploos van Amstel, 2017; The Bellona Foundation, 2020) present significant challenges.
An overview of freight transport with specific reference to urban goods distribution is essential as a primer to construction transport. The systemic framework of Urban Freight Transport (UFT) comprises three components viz Demand (for goods produced at places other than at the demand location, and requiring transportation), Supply (for meeting the demand by supplying logistics viz facilities and transport), and Context (logistics operations in facilities, and actual vehicle movements resulting from the interaction between demand and supply). The physical environment, in which demand and supply take place, defines the contexts and domains in which different SCs operate. Each of these components can be linked to one or more stakeholders viz Receivers (generators of demand for goods and, therefore for transport on the demand side), Shippers (send goods to fulfil this demand), LSPs (undertake logistics operations and actual deliveries as a direct result of the interaction between Receivers and Shippers). Contextual movements are regulated by Local Authorities, and the physical environment where all this takes place, is inhabited by Citizens (Kin et al., 2017; Guerlain et al., 2018; City Vitality and Sustainability, 2020; Diana et al., 2020).
Deliveries to construction sites are distinctly different from consumer goods movement, distribution, and delivery. Construction sites are material intensive and, irrespective of their size, need supplies to be aligned to their requirements, which may be irregular (Kim and Nguyen, 2018). Carriers attempt to be as service-oriented as possible, ignoring the negative social and environmental impacts this method of working might have. European Union (EU) transport data estimates the contribution of construction material transportation as circa 50% to European freight transportation (Balm and Ploos van Amstel, 2017), while another estimate places construction transport as circa 30% of urban freight transport (Guerlain et al., 2019b; Muerza and Guerlain, 2021), benchmarks for understanding the contribution of transportation to construction logistics. Illustration of the CSC in Figure 1 evidences the significance of the transport function in the construction domain (Fredriksson et al., 2020).
Construction Transport and Sustainability
Construction transportation being road dominant, is achieved primarily with motorised vehicles. By virtue of the sheer volume of the material to be transported, it consumes significant amounts of energy, and generates large amounts of emissions, a substantial negative environmental impact of construction logistics. While contributing to only 20–30% of road traffic, goods transport consumes 40% of urban oil, emitting 80–90% logistics related carbon and 16–50% of overall air pollutants (McKinnon, 2010; Lindholm and Behrends, 2012; Khaled and Alam, 2016). Construction materials being low cost/high volume compared to other industries (Lovell et al., 2006; Ying and Roberti, 2013; Balm and Ploos van Amstel, 2017; The Bellona Foundation, 2020), transportation forms a significant component of construction logistics, typically contributing to more than half the logistics costs (Szymonik, 2012), a fifth of the energy consumption (Smith et al., 2003), and a tenth of the greenhouse gases of the construction sector (Ying et al., 2014).
Other transport externalities across sustainability domains may be direct (e.g., pollution, noise, congestion, etc.), or indirect (e.g., loss of ecosystems, health impacts, reduced quality of life, etc.) (Kohn and Brodin, 2008; Browne and Allen, 2011; Bretzke, 2013; Morana et al., 2014; Behrends, 2015; Vrijhoef, 2015; Kin et al., 2017; Janné and Fredriksson, 2019; Chatziioannou et al., 2020). Freight transportation is road dominant and based on motorised vehicles, which have externalities far out of proportion to their numbers, and form one of the least sustainable transport modes (Khaled and Alam, 2016). Improved sustainability can, therefore, be achieved by optimising the transportation function of construction logistics through the lens of the following attributes:-
- Externalities “Transport externalities refer to a situation in which a transport user either does not pay for the full costs (e.g., including the environmental, congestion or accident costs) of his/her transport activity or does not receive the full benefits from it” (Director General for Transport, European Union, 1995, p. 4); “The crucial importance of transport externalities arises from the fact that, in a market economy, (economic) decisions are heavily dependent on market prices. However, when market prices fail to reflect existing scarcities (clean air, absorptive capacity of the environment, infrastructure etc.), the individual decisions of consumers and producers no longer add up to an outcome that provides maximum benefits to society as a whole. Thus, pricing on the basis of full social costs is a key element of an efficient and sustainable transport system” (Director General for Transport, European Union, 1995, p. 5). The relationship between transport and sustainability is paradoxical; while supporting mobility, transport is invariably and intricately linked to externalities e.g., Emissions, Use of Non-renewable Fuels, Waste, Loss of Ecosystems, Congestion, Noise, Accident Risk, Gridlock, Air/Water Pollution, Public Health, Damage to infrastructure, and Reduction in QoL of Life, to name a few (Kohn and Brodin, 2008; Browne and Allen, 2011; Bretzke, 2013; Morana et al., 2014; Behrends, 2015; Vrijhoef, 2015; Balm and Ploos van Amstel, 2017; Kin et al., 2017; Janné and Fredriksson, 2019). Table 1 illustrates the externalities associated with road freight transport and their inter-se associations.
- The extent of externalities of freight transport is reflected in an illustration of their monetisation per annum in the EU, and a comparison of externalised costs between Heavy Delivery Vehicles (HDVs) and other modes at Figure 3. The graphs give an idea of the unsustainability of freight transport.
- Costs Transportation costs may be upto two-thirds of the logistics costs (Bowersox et al., 2002, pp. 32–34; Building Research Establishment, 2003), though this does not directly imply that transport contributes as much to the negative impacts. However, considering that construction materials are generally of low cost and high volume as compared to other industries (Lovell et al., 2006; Ying and Roberti, 2013; Balm and Ploos van Amstel, 2017; The Bellona Foundation, 2020), and that the other processes involved in construction logistics are business processes (except warehousing) and not physical processes like transportation (Szymonik, 2012), it may be concluded that transportation may actually be responsible for more than half the costs of construction logistics as a whole. With materials usually accounting for approximately 30–50% of a building project cost (Agapiou et al., 1998; Ying et al., 2014), transportation costs constitute circa 39–58% of the total logistics costs and between 4 and 10% of the selling price of the building (Shakantu et al., 2003). As per another estimate, transportation costs can vary between 10 and 20% of construction costs (Building Research Establishment, 2003).
- Infrastructure In addition to being a cost component of construction logistics, the requirement of transport for the construction industry creates additional transport flows and a competition with other traffic users for existing infrastructure, creating an infrastructural deficit, or overloading of the road network in most urbanised areas. Establishment of infrastructure invariably lags the demand, leading to difficult accessibility and congestion in most cases (DG MOVE European Commission, 2012; Kin et al., 2017). The size of construction vehicles and the immense loads they carry can cause considerable damage to infrastructure, in comparison to other lighter freight vehicles (Director General for Transport, European Union, 1995).
- Management Construction materials delivery and waste removal are usually distinct businesses having different SCs with divergent orientations without integration. These are uncoordinated activities (McKinnon and Ge, 2006; Shakantu et al., 2008; Shakantu et al., 2009).
- Material delivery transport is managed for its own best efficiency, completely ignoring the impact of such localised efficiency-based operations on the transportation system at large and the negative impacts on the area of operations (Crainic et al., 2004). Delivery transport movement, therefore, consists of a very high level of empty trips between in-bound and out-bound traffic flows (Crainic et al., 2004; DG MOVE, European Commission, 2012).
- Activities of adjacent construction sites are not synchronised. In addition, waste collection vehicles are employed over and above the material delivery vehicles. Construction transport, therefore, competes with other road users to cause congestion in the road transport network (DG MOVE, European Commission, 2012; Kin et al., 2017). This leads to increase in road traffic and knock-on effects such as reduction in labour productivity, decrease in asset utilisation, increase in inventories, increase in distribution costs, increase in transportation times and delayed/omitted deliveries, and potential underutilisation of transport, amongst other impacts (Sankaran et al., 2005).
- This is accentuated by unidirectional loading of vehicles (onward trip for delivery and return trip for waste removal vehicles) in addition to non-utilisation of full vehicle capacity (part loading) (Berden, 2017) as a result of most material suppliers and Construction and Demolition Waste (C&DW) carriers operating with their dedicated vehicle fleet (Shakantu et al., 2009).
- A paradox in the current scenario exists, where fully loaded delivery vehicles may lead to overstocking of material on construction sites, causing congestion of the work environment and uncalled for material damage and waste, where-as actually, most vehicles travelling to site are not fully loaded, and further travel fully empty from site, leading to congestion and other externalities (Lundesjo, 2011). Low load factors, typically less than 50% (Vrijhoef, 2015), lead to very high per unit carbon emissions.
- Transport efficiency critically depends on vehicle capacity utilisation measured across the onward and return trips, finding backloads for returning vehicles being a major logistical challenge (McKinnon and Ge, 2006). Non-optimal capacity utilisation causes increased per unit carbon emissions and externalities vis-à-vis tonnage handled (Lundesjo, 2011). Empty running, earlier identified as a wasted resource, is now considered an environmental liability. Most sustainable distribution strategies focus acutely on reducing empty running of vehicles (McKinnon and Ge, 2006).
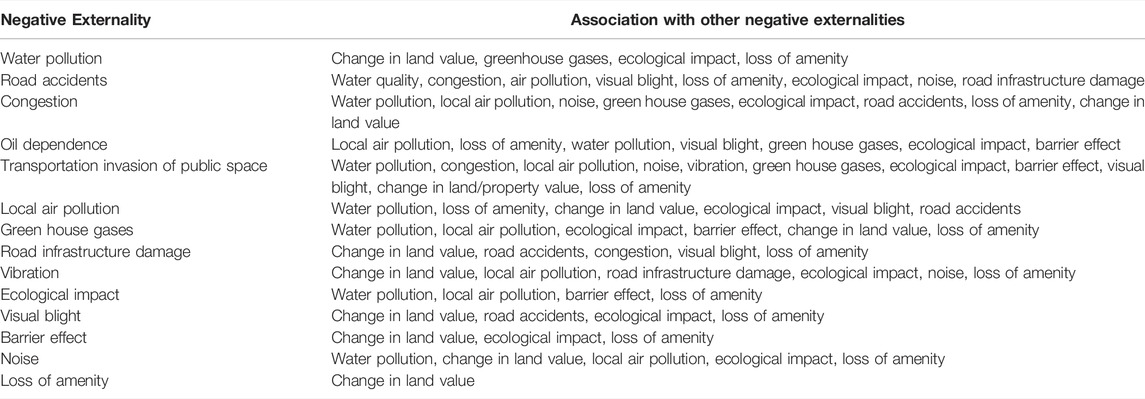
TABLE 1. Freight transport externalities and their inter-se interlinking (Chatziioannou et al., 2020).
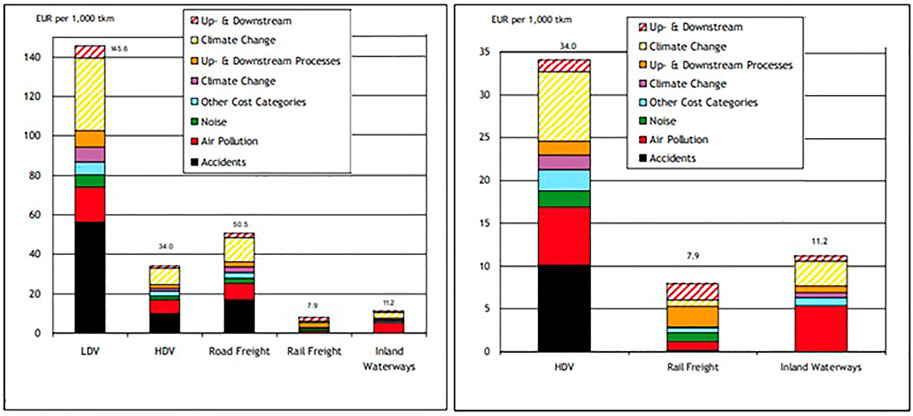
FIGURE 3. Monetised externalities of various forms of goods transport in the EU in 2008 (Left) and a comparison of the monetised externalities of HDVs with other modes (Right) (Van Essen et al., 2011).
In addition to the objectives of maximising of profits along with minimising of costs and lead times, logistics systems have evolved from minimising the Total Environmental Impact (Kohn and Brodin, 2008) to minimising the Total Impact (which includes Safety and Security inducing direct and short-time impacts, e.g., Accidents; Environmental impacts, both medium- and long-term; System characteristics impacting the management of the system and transport operation processes; System support in terms of infrastructure, SCs, upstream and downstream processes that impact environment and society; and, Unsustainable resource use) (Rohács and Rohács, 2020).
From the discussion so far, three aspects of construction transport support its optimisation i.e., manner of its employment, characteristics of the SC, and technology.
The direction of discussion being sustainable logistics, with the transport component forming its primary unsustainable element, it is pertinent to define a sustainable transport system viz “A sustainable transport system is one that throughout its full life-cycle operation, allows generally accepted objectives for health and environmental quality to be met, for example, those concerning air pollutants and noise proposed by the World Health Organisation (WHO); is consistent with ecosystem integrity, for example, it does not contribute to exceedance of critical loads and levels as defined by WHO for acidification, eutrophication and ground level ozone; and does not result in worsening of adverse global phenomena such as climate change and stratospheric ozone depletion” (OECD, 2002, p. 16). Figure 4 illustrates the key elements of a sustainable transport system.
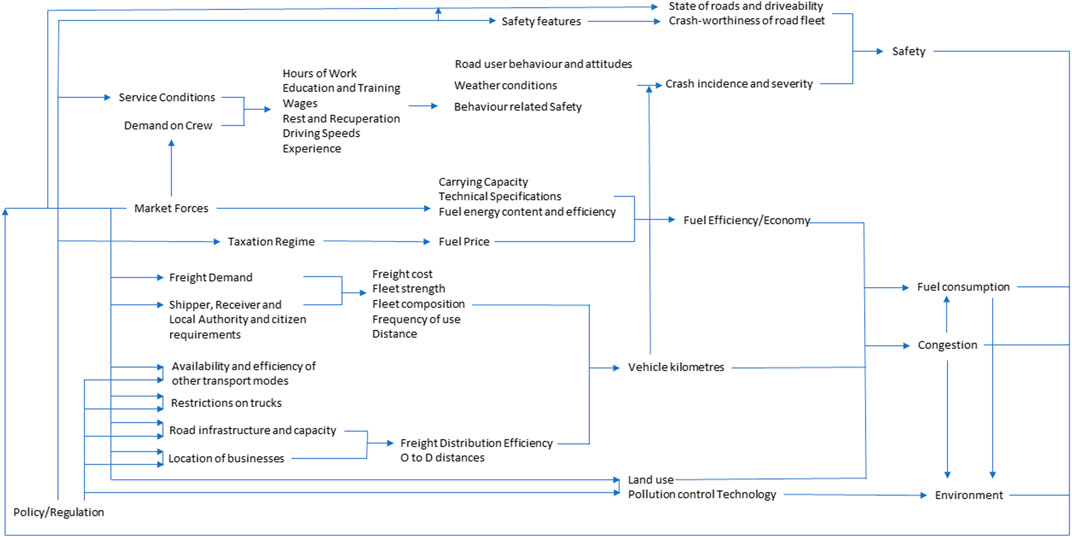
FIGURE 4. Key elements of a sustainable freight transport system (based on Richardson, 2005).
Greening Construction Logistics Through Transport
UNEP defines a green economy as “one that results in improved human well-being and social equity, while significantly reducing environmental risks and ecological scarcities. In its simplest expression, a green economy can be thought of as one which is low carbon, resource efficient and socially inclusive” (Fedrigo-Fazio and ten Brink, 2012, p. 4). The OECD Green Growth Report defines green growth as “fostering economic growth and development, while ensuring that natural assets continue to provide the resources and environmental services on which our well-being relies” (OECD, 2011, p. 4).
Both operate on the basis of development within environmental limits, which is also the universally understood meaning of the term “Greening.” In the transport domain, it is invariably construed as consisting of measures which reduce emissions from the tail pipe, therefore, localising focus on transport technologies. The concept is deeper and more involved than just technology, though technology does form a significant part of it. “Greening” implies “redesigning systems so that–in their functioning–they require less energy and materials, and produce less emissions while improving wider well-being goals” (OECD, 2021, p. 11). This discussion will consider “greening” as any measure which reduces emissions from freight transport and consider it synonymous with decarbonisation strategies.
Four fundamental frameworks for freight transport de-carbonisation are the Green Logistics Framework, the A-S-I/A-S-I-F Framework, the TIMBER Framework, and the IF-TOLD Framework. A discussion of these frameworks is essential to align their dimensions to the three aspects of construction transport presenting the “greening” opportunity.
Green Logistics Framework
This framework was developed for specifically greening freight transport operations. To understand the dimensions of greening, it is pertinent to first examine the key determinants impacting freight transport emissions. These are presented at Figure 5.
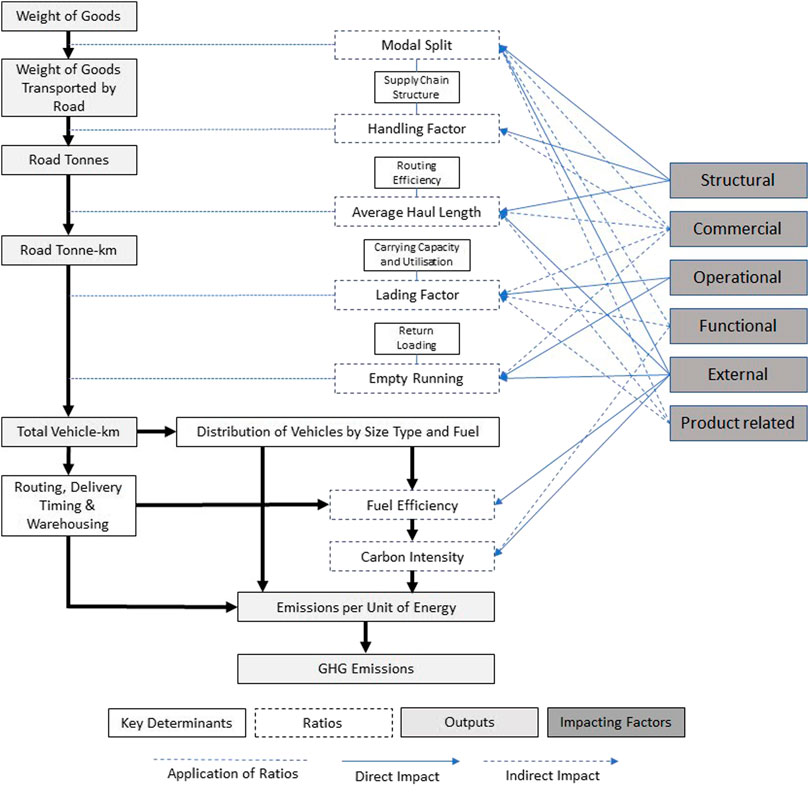
FIGURE 5. Key determinants of freight transport emissions (Based on Piecyk and McKinnon, 2010; McKinnon et al., 2014; McKinnon, 2018).
The model consists of the following key parameters (McKinnon, 2018, pp. 21–23):-
- Modal split The proportion of freight carried by different transport modes.
- Handling factor The ratio of the weight of the goods to the freight tonnes (weight) lifted. It is a measure of the number of links in an SC.
- Length of Haul The mean length of each link in an SC. Converts tonnes-lifted into tonne-km.
- Empty running Total vehicle-kms run empty.
- Load factor Measured in terms of weight.
- Energy efficiency Ratio of the distance travelled to the energy consumed. This is a function of vehicle characteristics, driving behavior, and traffic conditions.
- GHG emissions per unit of energy Depends on the carbon content of fuel burned in the vehicle, and in case of electric vehicles, fuel burnt at the power generation facility. These should ideally be measured on a “well-to-wheel” basis.
The “handling factor” and “length of haul” may be combined into a “freight transport intensity” parameter and “empty running” and “load factor” an “asset utilisation” parameter.
The main levers determining GHG emissions under this framework are (United Nations Conference on Trade and Development, 2017):-
- The SC Structure Relates to the location of the nodes and links through which freight moves, and which determine the geographical patterns of cargo/freight flows. Under this lever, key parameters include the average handling factor and the average length of haul.
- Freight Modal Split Refers to the allocation of cargo/freight traffic among transport modes, which is critical to any sustainable freight transport strategy. Some modes are “cleaner” than others in terms of the externalities they generate per ton-kilometre of cargo/freight carried. Modes also differ in their functionality and face different infrastructural, operational and technical constraints. Key parameters include the modal split.
- Vehicle Routing Within a fixed SC chain, vehicles can follow different routes between nodes, some of which may generate more externalities because of their length, traffic conditions and/or the environmental, cultural or historical sensitivity of the areas through which they pass. Key parameters include length of haul.
- Capacity and Utilisation Many freight transport vehicles are under- or over-loaded. Under-loading leads to the requirement of more trips, generating more traffic, using more fuel, and causing more pollution. Overloading reduces the number of trips required, but reduces fuel efficiency, increases emissions, and damages road infrastructure. Optimised vehicle loading is a major goal of any sustainable freight transport policy. Load factor is the key parameter.
- Traffic Congestion Operating freight transport vehicles on congested roads carries a fuel penalty. Rescheduling deliveries to off-peak periods and optimising freight transport planning/volume/operations contribute to cutting fuel consumption and emissions and ease the level of congestion for all types of traffic. Relevant parameters include average load on laden trips and average % empty running.
- Energy Efficiency A more efficient operation of freight transport vehicles translates directly into lower energy consumption, lower emissions, and greater resource conservation. A set of mutually reinforcing technological, operational, and behavioural measures can be deployed to improve energy efficiency.
- Carbon and Pollutant Content of the Energy Source Switching to cleaner fuels is key. Electrifying freight transport and using other clean technologies such as alternative fuels and hybrid propulsion systems can generate environmental benefits. However, alternative and cleaner energy sources or and/or the required refueling/recharging infrastructure might not be readily available and accessible. The cost differential can also hinder an effective fuel switch. A key parameter relates to the emission intensity or emission per unit of energy.
- Other Externalities Other effects such as noise, vibration, and accidents are not functions of energy consumption; these can be expressed with respect to vehicle-km. A key parameter would be externalities per vehicle kilometre.
The A-S-I/A-S-I-F Framework
Avoid-Shift-Improve (A-S-I) was first developed in Germany in the early 1990s as vermeiden, verbessern, verlagern. The term was first published in a 1994 report by the German Parliament’s Enquete Commission (Transformative Urban Mobility Initiative, 2019). The three key components of this framework are (Transport Policy Advisory Services, 2019):-
- Avoid/Reduce The need to improve the efficiency of the transport system as a whole. This may be achieved through integrated land-use management and transport demand management.
- Shift/Maintain instruments seek to improve individual trip efficiency. A modal shift from the most energy consuming and polluting transport mode towards more environmentally friendly modes addresses challenges of transport systems substantially.
- Improve focuses on vehicle and fuel efficiency as well as optimisation of the operational efficiency of transport. Additionally, improvement of the energy sources required for their operation is key, with inclination towards introducing renewable energy sources into the transport sector.
The literature also sees frequent reference to the A-S-I-F framework, which has a separate Fuel parameter to differentiate the energy efficiency from the level of emissions per unit of energy (Schipper et al., 2009). Emissions in the freight transport sector are dependent on the level of travel activity (A) in tonne-km across all modes, the modal structure (S), the fuel intensity of each mode (I), in litres per tonne-km, and a fuel’s carbon content, which yields a per unit emission factor (F). The relationship between these parameters is represented mathematically by the A-S-I-F equation, while the framework assesses opportunities for:-
- Avoid Reducing the level of transport Activity.
- Shift Altering the modal Structure of the transport system.
- Improve Reducing the energy Intensity of transport operation.
- Fuel Cutting the carbon content of the Fuel.
The A-S-I framework subsumes the Fuel attribute of the A-S-I-F framework into the Intensity parameter. Figure 6 illustrates the A-S-I-F equation and the A-S-I framework.
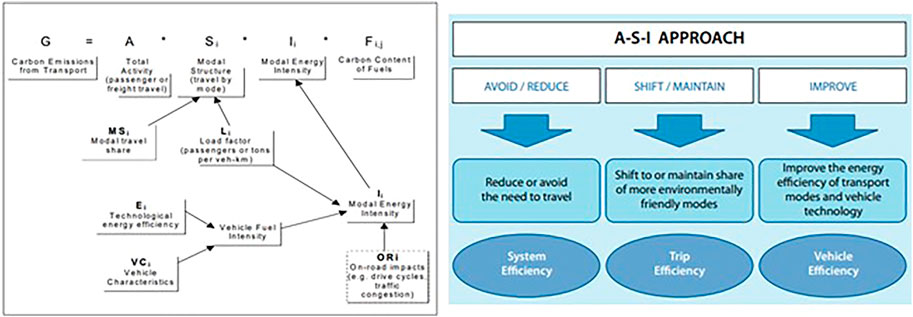
FIGURE 6. The A-S-I-F Equation (Schipper et al., 2009) and the A-S-I Framework (Ministry of Transport, New Zealand Government, 2021).
The freight transport sector encompasses a diverse set of activities connected by the common purpose of moving goods. The relative importance of each of the components to total changes in emissions varies with project type. The transportation system is highly interconnected and various policies, programs, and projects can directly and indirectly affect one or more of these components.
The TIMBER Framework
Logistics operations also need to take cognizance of factors extraneous to the organisation while formulating a freight transport de-carbonisation strategy. Alignment of these to the internal factors can accentuate the impacts positively, while if they are counteracting, internal efforts may either need to be intensified or de-carbonisation targets may need to be revised pragmatically downwards. The factors, acronymised as “TIMBER,” are as follows (McKinnon, 2018, p. 24):-
- Technology includes advances in transport, warehousing and materials handling technology.
- Infrastructure is predominantly transport infrastructure, comprising networks and terminals and covering all the main transport modes, but can also include energy and communication infrastructures.
- Market changes in the structure of the logistics services market, the way logistics services are traded and the nature of the demand for these services.
- Behaviour implies industry and employee levels and, at the latter, includes driver training and certification programmes.
- Energy comprising the nature of electricity generation, the availability of alternative fuels and the carbon intensity of the range of fuels used.
- Regulation at multi-national, national and local levels can include construction and use regulations on trucks, regulatory controls on the road haulage industry and restrictions on vehicle access at particular times of day. It can also be extended to cover fiscal policy measures.
The IF-TOLD Framework
This framework is similar to the Green Logistics Framework and involves reconfiguring freight transport technologies, operations, and infrastructure across six dimensions viz Intermodal Infrastructure; Fuel Alternatives; Technological Alternatives; Operational Practices; Logistical Realignment; and, Demand changes for goods and packaging.
Figure 7 illustrates the interrelationship between Sustainable Freight Transport, and ASI, Green Logistics, TIMBER, and IF-TOLD frameworks.
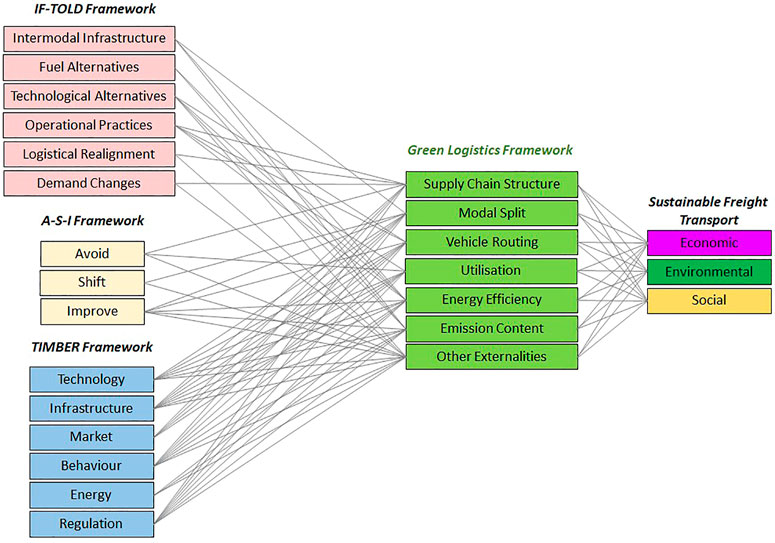
FIGURE 7. Interrelationship between Sustainable Freight Transport, and ASI, Green Logistics, TIMBER, and IF-TOLD frameworks (Authors’ conception based on United Nations Conference on Trade and Development, 2017; Mckinnon, 2018).
The Solution Space
Out of the above four frameworks for freight transport de-carbonisation, the A-S-I framework presents itself as the most overarching framework. Minimum attribute specificity makes it flexible and versatile to circumscribe more than one de-carbonisation dimensions within each of its attributes, at the same time associating more than one attributes with a particular dimension of the other frameworks, resulting in a multi-dimensional application matrix.
Based on an integration of the four frameworks, a solution space consisting of five focus areas emerges viz management of freight demand, smart use and combination of transport modes, sharing and maximum utilisation of assets, energy efficiency of assets, and use of lowest energy source to the extent feasible. The individual elements comprising each one of these are as follows (McKinnon, 2018; Punte et al., 2019):-
- Freight demand Reducing the freight transport intensity of economic activity (SC restructuring, localisation and nearshoring, decentralisation of production and stocking, dematerialisation, and influencing consumer behaviour).
- Transport modes Shifting to low intensity transport modes (Increased use of rail, waterways, and low emission modes, multimodal optimisation, and synchromodality).
- Asset utilisation Load optimisation, consolidation and asset sharing, modular packaging, backhauling, and open warehouses and transportation networks.
- Energy efficient assets Reducing energy consumption relative to Freight-Tonne-Km (Cleaner and efficient technologies and fleet, fleet operation, telematics, and low energy infrastructure).
- Low energy intensity Reducing the carbon content of energy used in logistics (Optimising diesel systems, CNG/LNG, biofuels, hydrogen, electric/hybrid vehicles).
The major stakeholders, (as in the case of smart city initiatives of which smart transport with de-carbonisation as a subset, is an integral part) form the Quadruple Helix (Borkowska and Osborne, 2018) for de-carbonisation/greening delivery. Each one of the above focus areas needs to be seen in a different perspective by the four major stakeholders in the Quadruple Helix (Punte et al., 2019) i.e.:-
- Government Regulation, pricing, subsidy, technology induction, emission/energy norms, infrastructure, and alternative mode development.
- Business SC restructuring, material and source alternatives, transparency in informing impacts, actively seeking lower emission modes, investment in infrastructure improving connectivity and complementarity, inclusion of fleet and energy efficiency criteria in logistics procurement, freight exchange platforms, open warehouses, and encourage energy efficiency through technology and investment.
- Research and Development Institutions Developing explanatory/collaborative/integrated models, logistics design concepts, network redesign, support industry research initiatives, consolidation/warehousing/transshipment requirements, freight flow analysis, technology impact analysis, and development of cleaner energy sources/technologies.
- Civil society Collaboration between players, advocacy, campaigning and promotion of cleaner transportation models and technology.
This aspect is of significance in the construction transport domain, since the implicit onus of managing construction logistics falls on the construction industry, (unlike city logistics whose responsibility is assumed by city managers), while the benefits are reaped by the city as a whole (Janné and Fredriksson, 2021).
The Peculiarities of Construction Transport
Before the New Zealand context of freight transport is discussed, the peculiarities of construction transport need to be emphasised to provide context to the development of a research framework. Construction transport is a sub-set of freight transport however, it differs in terms of the impact of the nature of the CSC (primarily fragmentation and bespoke nature) (Alashwal and Fong, 2015; Guerlain et al., 2019b; Jones et al., 2021), peculiarities of employment patterns on a construction project (Ying et al., 2014; Sezer and Fredriksson, 2021), the spread of transport configuration peculiar to the construction industry (Guerlain et al., 2019a), and the onus of managing construction logistics being on the construction industry and not those managing city logistics (Janné and Fredriksson, 2019).
Impact of the Nature of the Construction Supply Chain
The fundamental differences between a CSC and other common SCs impact the transportation function associated with a construction project (Guerlain et al., 2019b). A comparative analysis between commonly known urban SCs and CSCs with specific reference to transportation/material delivery is at Table 2.
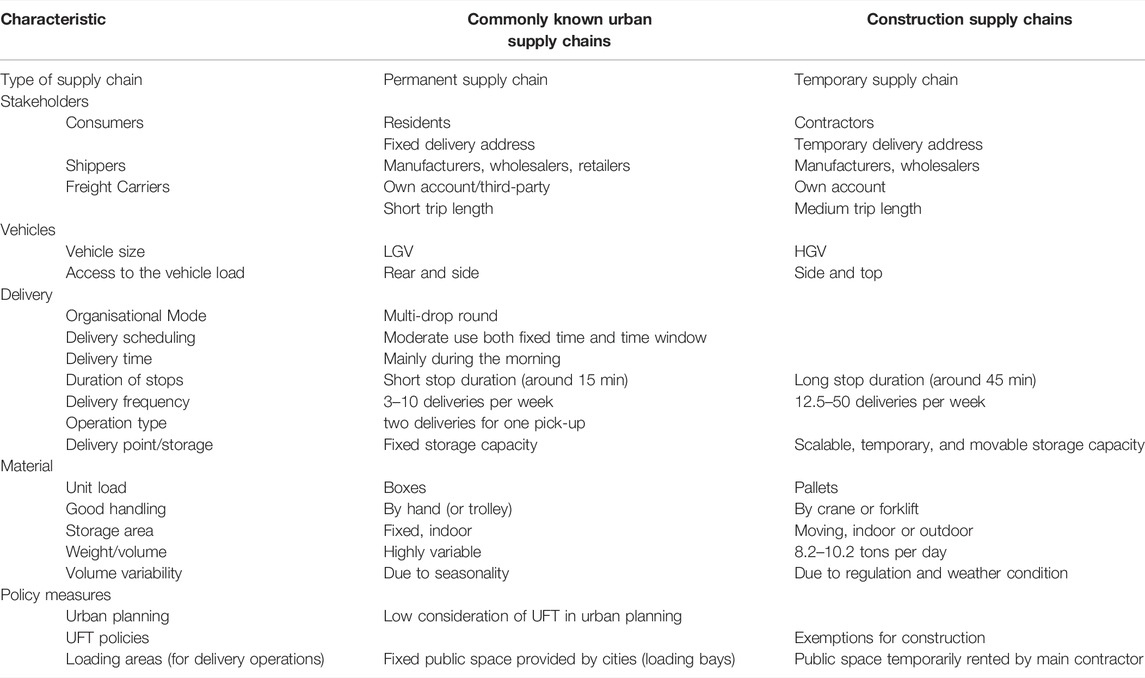
TABLE 2. Comparison of Common Urban Supply Chains and Construction Supply Chains specific to transportation/material delivery (Guerlain et al., 2019b).
Transport Utilisation Pattern and Types
Deliveries to construction sites are distinctly different from consumer goods movement, distribution, and delivery. Construction sites are material intensive and, irrespective of their size, need supplies to be aligned to their requirements, which may be irregular (Kim and Nguyen, 2018). Construction deliveries during a day are invariably numerous, small in quantity and ad-hoc, since material needs to be delivered on time and in precise quantities (Balm and Ploos van Amstel, 2017; Construction in the Vicinities Innovative Co-creation, 2018).
A typical construction project may be divided into four cycles viz Site preparation, excavation, and ground works; Framing; Installation of services; and, Interior work. These cycles lead to the following pattern of employment of transport on a construction project: 1) Excavation works lead to a large proportion of transports at the beginning of projects, which decrease significantly after the first cycle; 2) Waste collection related transport increases from the second cycle; 3) Concrete is delivered mainly during the second and third cycles; 4) Transports delivering small packages mainly take place during the fourth cycle; and, 5) Transport related to support services are distributed in the first three cycles (Sezer and Fredriksson, 2021).
Typically, the types of transport corresponding to the project cycles are: 1) Trucks plus trailers are used most commonly during the first and fourth cycles of a project but are used less during the second and third cycles; 2) Delivery vans are used more often during the fourth cycle, and least during the first cycle; 3) Trucks are used more commonly during the fourth cycle; 4) Semi-trailers are used evenly throughout the projects’ lifecycles.
Construction transport in New Zealand typically exhibiting characteristics discussed above is substantiated by a case study in literature (Ying et al., 2014).
The New Zealand Context
New Zealand’s freight logistics are driven by its peculiar spatial, market, regulatory, and economic attributes (inter alia geographical isolation, deregulated market, import dependency, small regional extent, long transits, small market/economy, non-implementation of vehicle emission norms/standards etc.) (Ying et al., 2014; Khan & Lockhart, 2019; Chapman and Howden-Chapman, 2020). While various implementation methodologies have worked effectively in de-carbonising freight transport in Europe, North America, and Asia, New Zealand’s peculiarities prevent their wholesale adoption.
93% of annual freight tonnages are moved by road-based transport. Diesel-powered freight transport (including Light Commercial Vehicles) produces 42% of New Zealand’s transport GHG emissions while accounting for only 26.1% vehicle-kilometres. A third of New Zealand’s freight transport is construction related. With a 99% fossil-fuel dependency, it exhibits about three times higher emissions intensity than other forms of road transport. Construction loads contribute to about a third of the freight tonnages (Ministry of Transport, New Zealand Government, 2020a, 2020c). To meet the 2050 Climate Change targets, de-carbonisation or greening of the road transport sector is critical (Ministry for the Environment, New Zealand Government, 2021). Freight transport, including construction transport, forms an important component of this de-carbonisation endeavour.
The Climate Change Commission in its “Advice to the New Zealand Government on the first three emission budgets” recommends uptake of electric vehicles (EVs), a shift to low carbon fuels (including hydrogen as a fuel), improving the efficiency of vehicles and freight movement, adoption of the A-S-I framework, optimising existing systems, end-to-end integrated transport planning, and formulation of a “National Low Emissions Freight Strategy” for investment and infrastructure to deliver a low-emissions freight system as strategic directions for de-carbonising the heavy transport fleet. It also emphasises policy to support co-funding pilot and demonstration projects for unproven technologies, sharing learnings with industry, and supporting adoption at scale of successful pilot projects (Climate Change Commission, New Zealand, 2021).
The Ministry for the Environment, New Zealand Government, in its “Transitioning to a low-emissions and climate-resilient future” consultation document seeks 25% reduction in emissions from freight transport by 2035, reduction in the emissions intensity of transport fuel by 15 per cent by 2035, integrating land use, urban development and transport planning and investments to reduce transport emissions, implementing mode-shift plans for urban areas, accelerating de-carbonisation of trucks, and developing a “Freight and Supply Chain Strategy” as strategic/policy directions for de-carbonising freight transport (Ministry for the Environment, New Zealand Government, 2021).
The Ministry of Transport in its document titled “Transport Emissions: Pathways to Net Zero by 2050,” adopt the A-S-I framework to identify opportunities to reduce emissions across the transport system by addressing the four key elements of the transport system viz transport activity (number of trips and kilometres travelled), mode share (percentage share of different modes), energy intensity (quantity of fuel used per kilometre) and carbon intensity (emissions from quantity of fuel per kilometre). The Ministry has developed three themes to group together opportunities within this framework, with one of the three focussing on freight. The key focus areas for de-carbonising or greening freight transport are improving SC efficiency, optimising freight routes, equipment and vehicles, improving the efficiency of freight payloads, using data and support information sharing and collaboration, and improving resilience and reliability of less carbon intensive transport modes. While the overarching strategy is identified, it falls short of specific implementation measures, and seeks further research and work with industry for these (Ministry of Transport, New Zealand Government, 2021).
The preceding discussion considers freight transport, however, all attributes discussed have a more acute impact on construction transport primarily because of it being a major component of the construction logistics domain, the endemic fragmentation exacerbating the adverse sustainability impacts of construction transport, and the onus of managing construction logistics being on the construction industry unlike that of city logistics.
A Research Framework for Greening Construction Transport in New Zealand
Research Framework Development Methodology
The Design Science approach was adopted for development of the research framework as design science focusses on development and validation of prescriptive knowledge. An abridged design framework (Johannesson and Perjons, 2014, p. 77) was used to include three activities to arrive at the desired artefact (in this case, a research framework as a “model” manifestation of design science artefact). The three activities are described as follows:-
- Problem Explication This activity was based on existing explicit descriptive knowledge (Johannesson and Perjons, 2014, p. 25) through an exhaustive literature review pertaining to emissions and their impact on climate in New Zealand, freight transport as a contributor to emissions in general and in New Zealand in particular, the transport function in construction, frameworks for freight transport emissions reduction, strategies and methodologies adopted overseas (Europe and North America) to reduce transport emissions, and the New Zealand status quo. Following is the statement of the problem arrived at:-
“Emissions from transport are an identified climate change contributor. Mechanisms ranging from strategic initiatives to operational methodologies have been applied overseas, particularly in Europe and North America, to reduce freight transport emissions. Extremely high domestic dependence on road transport for freight movement presents a fertile opportunity for overall emissions reduction. New Zealand’s unique circumstances such as geographical isolation, import dependency, small regional extent, long transits, small market/economy, and regulatory voids, impede direct adoption of overseas freight transport initiatives. Lack of contiguous regional tracts, physical connectivity, a large market and economy, and technology availability demands a solution reflecting domestic conditions. Customisation of overseas freight transport initiatives to New Zealand realities is a potential solution” (Authors).
- The problem explication points to a new solution (prescriptive knowledge) for a known problem from existing descriptive knowledge, manifesting Exaptation as a research contribution. The novelty of the proposed research lies in instantiation of known artefacts (models, paradigms, techniques) under circumstances they have not been designed for.
- Requirement Definition Based on descriptive knowledge available from New Zealand Government reports and other similar documents, the government’s strategy identifies SC efficiency, transport optimisation, data/information sharing, collaboration, and legislation/regulation as de-carbonisation enablers, using the A-S-I de-carbonisation framework. The strategy, however, falls short of specific pathways, seeking further research to arrive at concrete implementation pathways (Ministry for the Environment, New Zealand Government, 2021; Ministry of Transport, New Zealand Government, 2021). The overall spread of the strategy was considered too wide to be undertaken in one research programme, therefore, the requirement definition was limited to “optimisation of the transport function, and re-design of the supply chain with specific reference to logistics.”
- Design and Development of the Artefact The artefacts in the instant case were the research framework (research objectives), and a research plan based on understanding of preceding work available, building up of research on it, and the potential succeeding actions. The research framework artefact itself will include research objectives, potential research outputs, and a research plan.
Figure 8 illustrates the research framework development methodology from the design science approach.
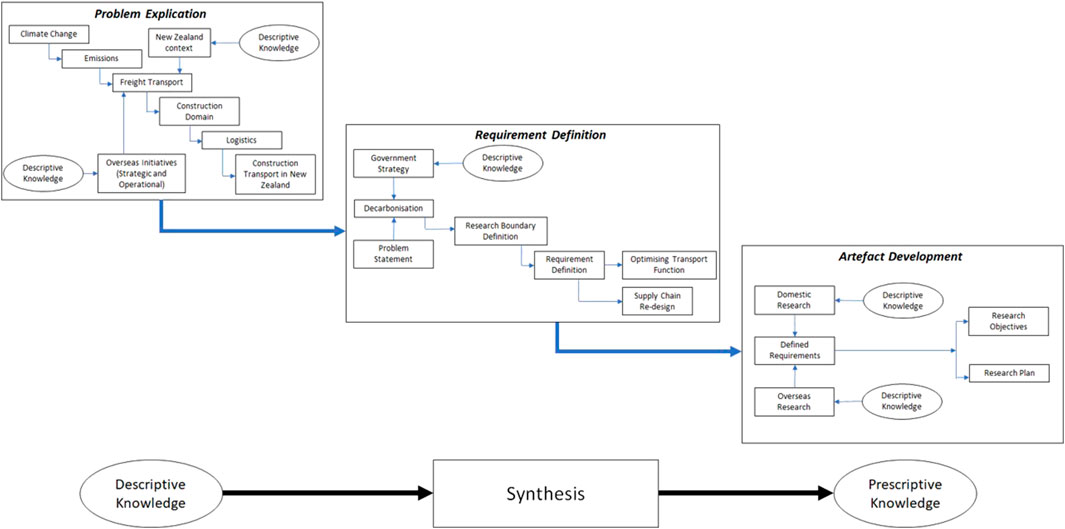
FIGURE 8. The Research Framework development methodology (Authors’ conception based on Johannesson and Perjons, 2014).
The Research Framework
With the current focus on 2050 carbon targets, and a climate emergency having been declared in New Zealand (Ministry of Transport, New Zealand Government, 2021), an objective of 25% reduction in freight emissions by 2035 has been adopted, as part of the overall transport de-carbonisation strategy.
93% of freight in New Zealand is road-bound, a third of it, construction related (Ministry of Transport, New Zealand Government, 2020a). New Zealand freight’s 99% fossil fuel (diesel) reliance is well above the world average, and when combined with the country’s attributes, exacerbates the situation. Freight transport de-carbonisation is particularly challenging in New Zealand owing to the sector’s continual growth offsetting any technological advancements. Because they are symptomatic, stand-alone transport solutions are unlikely to be effective. Rather than merely transport demand/flow-based solutions, an emphasis on composite logistics-transport solutions is required. Within the A-S-I framework adopted by the New Zealand Government for freight transport de-carbonisation specific pathways/measures need to be worked out to achieve the targeted objectives (Ministry of Transport, New Zealand Government, 2021). While sufficient overseas examples of strategy formulation and implementation are available (Browne et al., 2005; Allen et al., 2007; Browne et al., 2012), the uniqueness of New Zealand’s disposition prevents wholesale adoption of overseas implementations. Research is required to benchmark New Zealand specific criteria to overseas strategies/initiatives in formulating logistical solutions tailored to New Zealand reality.
Towards achieving the above, the following research objectives have been synthesised considering international research and New Zealand realities:-
- Analyse international initiatives to create a referrable repository of implementation variables/parameters and their inter-relationships.
- Identify domestic freight transport A-S-I (Avoid-Shift-Improve) opportunities considering logistics issues such as loading patterns, capacity utilisation, movement reduction, integrated forward/reverse logistics, technology etc.
- Benchmark domestic planning parameters to international implementation.
- Synthesise New Zealand specific schemes/models for re-organising logistics and transitioning to cleaner transport technology.
- Validate alternatives using analytical/computational tools.
- Build and create implementation scenarios by combining alternatives with spatial-operational-technological parameters to assess carbon reduction and long-term sustainability/economic impacts.
Figure 9 illustrates the research framework to include Research Objectives (ROs), their comprising elements, and linkages between these. The potential outputs of the ROs is at Table 3, while the research plan is at Figure 10.
The proposed research framework aligns with “New Zealand Energy Strategy to 2050” (Ministry for Economic Development, 2007), “Emissions Reduction Plan discussion document” (Ministry for the Environment, New Zealand Government, 2021), “2020 Green Freight Strategic Working Paper” (Ministry of Transport, New Zealand Government, 2020a), “Government Policy Statement on Land Transport 2021-22/2030-31” (Ministry of Transport, New Zealand Government, 2020b), “Transport Emissions: Pathways to Net Zero by 2050” (Ministry of Transport, New Zealand Government, 2021), “Our Plan for the Land Transport System 2021-31 Version 2” (New Zealand Transport Agency, 2020), “A Low Emissions Future for Aotearoa” (Climate Change Commission, New Zealand, 2021), [8]; and Reports of the Intergovernmental Panel on Climate Change (IPCC, 2014a; IPCC, 2014b; IPCC, 2019a; IPCC, 2019b).
Conclusion
The construction industry exhibits endemic fragmentation in its operations. With transport forming a major component of construction logistics, and construction material being low-cost and high-volume in nature requiring large quantum of transport, the adverse impacts of construction transport are invariably more acute than freight transport in general. Sustainability now forming an integral dimension of construction operations, there is an urgent need for “greening” construction transport.
New Zealand exhibits certain peculiar traits, mainly due to its geographical isolation from the world inter alia geographical isolation, import dependency, small regional extent, long transits, small market/economy, non-existence of vehicle emission norms/standards etc. 93% of freight movement is by means of diesel-based freight transport, a third of it, construction related. The Climate Change objectives of 2050 make it incumbent that freight transport “greening” (or de-carbonisation) measures be put in place within the overall ambit of freight transport.
Government strategy does identify SC efficiency, transport optimisation, data/information sharing, collaboration, and legislation/regulation as de-carbonisation enablers under the A-S-I framework, however, it falls short of concrete implementation pathways, seeking further research. The requirement of research is substantiated by the ineptitude for adopting successful European and North American strategic and operational initiatives freight transport de-carbonisation, in (New Zealand) circumstances, they are not designed for.
This paper aims to bridge the implementation gap by suggesting a research framework for “greening” construction transport, limiting the scope to optimisation of the transport function, and re-design of the supply chain with specific reference to logistics, with the understanding that construction transport requires a higher intricacy and depth of analysis owing to the nature of construction logistics, and the onus of its management being on the construction industry. The results will also enable “greening” freight transport in general, since measures applicable to freight transport are expected to be a subset of those applicable to construction transport, as a consequence of the peculiarity of construction operations.
Author Contributions
KD, JT, AlG and AmG all the above authors have contributed equally to the manuscript.
Conflict of Interest
The authors declare that the research was conducted in the absence of any commercial or financial relationships that could be construed as a potential conflict of interest.
Publisher’s Note
All claims expressed in this article are solely those of the authors and do not necessarily represent those of their affiliated organizations, or those of the publisher, the editors and the reviewers. Any product that may be evaluated in this article, or claim that may be made by its manufacturer, is not guaranteed or endorsed by the publisher.
References
Agapiou, A., Flanagan, R., Norman, G., and Notman, D. (1998). The Changing Role of Builders Merchants in the Construction Supply Chain. Construction Management Econ. 16, 351–361. doi:10.1080/014461998372376
Alashwal, A., and Abdul-Rahman, H. (2014). Developing a Conceptual Framework of Fragmentation in Construction. Available at: https://www.library.auckland.ac.nz/external/finalproceeding/Files/Papers/46530final001 76 .pdf.
Alashwal, A., and Fong, P. (2015). Empirical Study to Determine Fragmentation of Construction Projects. J. Construction Eng. Management. 141, 04015076. doi:10.1061/(ASCE)CO.1943-7862.0000986
Allen, J., Thorne, G., and Browne, M. (2007). Best Urban Freight Solutions (BESTUFS), GoodPractice Guide on Urban Freight Transport. Brussels, Belgium: European Commission.
Balm, S., and Ploos van Amstel, W. (2017). “Exploring Criteria for Tendering for Sustainable Urban Construction Logistics,” in City Logistics 1: New Opportunities and Challenges. Editors E. Taniguchi, and R. Thompson (Hoboken, USA: John Wiley & Sons), 251–263. doi:10.1002/9781119425519.ch13
Behrends, S. (2015). “Factors Influencing the Performance of Urban Consolidation Schemes,” Proceedings of the 2nd Interdisciplinary Conference on Production, Logistics and Traffic 2015. Editors U. Clausen, H. Friedrich, C. Thaller, and C. Geiger, 351–367. doi:10.1007/978-3-319-21266-1_23
Berden, M. (2017). Analysis of Construction Logistics Calculation Models and Factors that Obstruct Their Development. Available at: https://www.civic-project.eu›files›2017_06_01_d.2.1_final_anoniem.pdf.
Borkowska, K., and Osborne, M. (2018). Locating the Fourth Helix: Rethinking the Role of Civil Society in Developing Smart Learning Cities. Int. Rev. Educ. 64, 355–372. doi:10.1007/s11159-018-9723-0
Bowersox, D., Closs, D., and Cooper, M. (2002). Supply Chain Logistics Management. New York, USA: The McGraw-Hill Companies.
Bretzke, W.-R. (2013). Global Urbanization: a Major challenge for Logistics. Logist. Res. 6, 57–62. doi:10.1007/s12159-013-0101-9
Browne, M., and Allen, J. (2011). Enhancing the Sustainability of Urban Freight Transport and Logistics. Transport Commun. Bull. Asia Pac. 80, 1–19.
Browne, M., Allen, J., Nemoto, T., Patier, D., and Visser, J. (2012). Reducing Social and Environmental Impacts of Urban Freight Transport: A Review of Some Major Cities. Proced. - Soc. Behav. Sci. 39, 19–33. doi:10.1016/j.sbspro.2012.03.088
Browne, M., Sweet, M., Woodburn, A., and Allen, J. (2005). Urban Freight Consolidation Centres: Final Report. London, United Kingdom: Transport Studies Group, Ministry of Transport.
Building Research Establishment (BRE) (2003). Construction Site Transport: The Next Big Thing. Watford, UK: BRE. Available at: https://www.bre.co.uk/pdf/constructiontraffic.pdf.
Chapman, R., and Howden-Chapman, P. (2020). Transforming Transport and Cities in NZ: A Note for the Climate Change Commission’s Engagements on Transport and Urban Form. Wellington, New Zealand: New Zealand Centre for Sustainable Cities.
Chastas, P., Theodosiou, T., and Bikas, D. (2016). Embodied Energy in Residential Buildings-Towards the Nearly Zero Energy Building: A Literature Review. Building Environ. 105, 267–282. doi:10.1016/j.buildenv.2016.05.040
Chatziioannou, I., Alvarez-Icaza, L., Bakogiannis, E., Kyriakidis, C., and Chias-Becerril, L. (2020). A Structural Analysis for the Categorization of the Negative Externalities of Transport and the Hierarchical Organization of Sustainable Mobility's Strategies. Sustainability. 12, 6011. doi:10.3390/su12156011
City Vitality and Sustainability (CIVITAS) (2020). Smart Choices For Cities: Making Urban Freight Logistics More Sustainable (CIVITAS Policy Note). Brussels, Belgium: CIVITAS, European Commission.
Climate Change Commission, New Zealand (CCC) (2021). Ināia Tonu Nei: A Low Emissions Future for Aotearoa. Advice to the New Zealand Government on its First Three Emissions Budgets and Direction for its Emissions Reduction Plan 2022 – 2025. Wellington, New Zealand: Climate Change Commission.
Construction in the Vicinities Innovative Co-creation (CIVIC) (2018). Smart Construction Logistics. Sweden: CIVIC.
Cox, A., and Ireland, P. (2002). Managing Construction Supply Chains: The Common Sense Approach. Eng. Construction Architectural Management. 9 (5), 409–418. doi:10.1108/eb021235
Crainic, T. G., Ricciardi, N., and Storchi, G. (2004). Advanced Freight Transportation Systems for Congested Urban Areas. Transportation Res. C: Emerging Tech. 12, 119–137. doi:10.1016/j.trc.2004.07.002
Dani, A. A., Roy, K., Masood, R., Fang, Z., and Lim, J. B. P. (2022). A Comparative Study on the Life Cycle Assessment of New Zealand Residential Buildings. Buildings. 12, 50. doi:10.3390/buildings12010050
DG MOVE European Commission (2012). Study on Urban Freight Transport. Available at: https://civitas.eu/sites/default/files/2012_ec_study_on_ urban_freight_transport_0.pdf.
Diana, M., Pirra, M., and Woodcock, A. (2020). Freight Distribution in Urban Areas: A Method to Select the Most Important Loading and Unloading Areas and a Survey Tool to Investigate Related Demand Patterns. Eur. Transp. Res. Rev. 12 (1), 14. doi:10.1186/s12544-020-00430-w
Director General for Transport, European Union (DG Transport EU) (1995). Towards Fair and Efficient Pricing in Transport: Policy Options for Internalising the External Costs of Transport in the European Union. Brussels, Belgium: European Commission.
Dubois, A., and Gadde, L.-E. (2002). The Construction Industry as a Loosely Coupled System: Implications for Productivity and Innovation. Construction Management Econ. 20, 621–631. doi:10.1080/01446190210163543
Fedrigo-Fazio, D., and ten Brink, P. (2012). Green Economy. Nairobi, Kenya: United Nations Environmental Programme.
Fredriksson, A., Flyen, C., Fufa, S., Venås, C., Hulthén, K., Brusselaers, N., et al. (2020). Construction Logistics Scenarios And Stakeholder Involvement: Scenarios of Construction Logistics. Sweden: Minimizing Impact of Construction Material Flows in Cities: Innovative Co-creation (MiMiC). Linköping, Sweden: Linköping University.
Ghanem, M., Hamzeh, F., Seppänen, O., and Zankoul, E. (2018). “A New Perspective of Construction Logistics and Production Control: An Exploratory Study,” in Proceedings of the 26th Annual Conference of the International Group for Lean Construction (IGLC). Editor V. González (Chennai, India, 992–1001. doi:10.24928/2018/0540
Guerin, E., Waisman, H., and Mas, C. (2014). Pathways to Deep Decarbonisation. New York, United Nations: Sustainable Development Solutions Network (SDSN) and Institute for Sustainable Development and International Relations. (IDDRI).
Guerlain, C., Renault, S., and Ferrero, F. (2019a). “Understanding Construction Logistics: Comparative Analysis,” in The 11th International Conference on City Logistics. Dubrovnik, Croatia.
Guerlain, C., Renault, S., and Ferrero, F. (2019b). Understanding Construction Logistics in Urban Areas and Lowering its Environmental Impact: A Focus on Construction Consolidation Centres. Sustainability. 11, 6118. doi:10.3390/su11216118
Guerlain, C., Renault, S., and Ferrero, F. (2018). “Urban Freight: What about Construction Logistics?,” in Proceedings of 7th Transport Research Arena TRA 2018, April 16-19, 2018. Vienna, Austria. Available at: https://www.list.lu/fileadmin/files/news/TRA2018_10859_GUERLAIN_v03.00.pdf.
Intergovernmental Panel on Climate Change (IPCC) (2014a). Fifth Assessment Report: Summary for Policy Makers. Geneva, Switzerland: IPCC.
Intergovernmental Panel on Climate Change (IPCC) (2014b). Climate Change 2014: Synthesis Report. Contribution of Working Groups I, II and III to the Fifth Assessment Report of the Intergovernmental Panel on Climate Change. Geneva, Switzerland: Intergovernmental Panel on Climate Change.
Intergovernmental Panel on Climate Change (IPCC) (2019a). Climate Change and Land: An IPCC Special Report on Climate Change, Desertification, Land Degradation, Sustainable Land Management, Food Security, and Greenhouse Gas Fluxes in Terrestrial Ecosystems. Geneva, Switzerland: Intergovernmental Panel on Climate Change.
Intergovernmental Panel on Climate Change (IPCC) (2019b). Global Warming of 1.5°C. An IPCC Special Report on the Impacts of Global Warming of 1.5°C above Pre-industrial Levels and Related Global Greenhouse Gas Emission Pathwaysthe Context of Strengthening the Global Response to the Threat of Climate Change, Sustainable Development, and Efforts to Eradicate Poverty. Geneva, Switzerland: Intergovernmental Panel on Climate Change.
Jang, H., Russell, J. S., and Yi, J. S. (2003). A Project Manager's Level of Satisfaction in Construction Logistics. Can. J. Civ. Eng. 30, 1133–1142. doi:10.1139/l03-068
Janné, M. (2020). Construction Logistics in a City Development Setting. Sweden: Doctoral dissertation, Linköping University.
Janné, M., and Fredriksson, A. (2019). Construction Logistics Governing Guidelines in Urban Development Projects. Construction Innovation 19, 89–109. doi:10.1108/CI-03-2018-0024
Janné, M., and Fredriksson, A. (2021). Construction Logistics in Urban Development Projects - Learning From, or Repeating, Past Mistakes of City Logistics? Int. J. Logistics Management 33 (5), 49–68. doi:10.1108/IJLM-03-2020-0128
Johannesson, P., and Perjons, E. (2014). An Introduction to Design Science. Cham, Switzerland: Springer.
Jones, K., Mosca, L., Whyte, J., Davies, A., and Glass, J. (2021). Addressing Specialization and Fragmentation: Product Platform Development in Construction Consultancy Firms. Construction Management Econ., 1–16. doi:10.1080/01446193.2021.1983187
Khaled, A., and Alam, Z. (2016). Location Factors and Their Performance Measures for a Sustainable Urban Freight Consolidation Centre (UFCC). SIU J. Management. 6 (1), 7–30.
Khan, M., and Lockhart, J. (2019). “Embedding Corporate Social Responsibility into Business Practice: Lessons Learned from New Zealand,” in Clean, Green and Responsible? CSR, Sustainability, Ethics and Governance. Editors G. Eweje, and R. Bathurst (Switzerland: Springer), 87–105. doi:10.1007/978-3-030-21436-4_6
Kim, S.-Y., and Nguyen, V. T. (2018). A Structural Model for the Impact of Supply Chain Relationship Traits on Project Performance in Construction. Prod. Plann. Control. 29, 170–183. doi:10.1080/09537287.2017.1398846
Kin, B., Verlinde, S., and Macharis, C. (2017). “Towards a Sustainable Urban Freight Transport System - Axes for Intervention,” in Paper presented at the Logistics Research Network Annual Conference. Southampton, United Kingdom. London, UK: Chartered Institute of Logistics and Transport).
Kohn, C., and Brodin, M. H. (2008). Centralised Distribution Systems and the Environment: How Increased Transport Work Can Decrease the Environmental Impact of Logistics. Int. J. Logistics Res. Appl. 11, 229–245. doi:10.1080/13675560701628919
Lindholm, M., and Behrends, S. (2012). Challenges in Urban Freight Transport Planning - A Review in the Baltic Sea Region. J. Transport Geogr. 22, 129–136. doi:10.1016/j.jtrangeo.2012.01.001
Lovell, A., Saw, R., and Stimson, J. (2005). Product Value‐density: Managing Diversity through Supply Chain Segmentation. Int. J. Logistics Management. 16, 142–158. doi:10.1108/09574090510617394
Lundesjo, G. (2011). Using Construction Consolidation Centres to Reduce Construction Waste and Carbon Emissions. London, UK: Waste and Resources Action Programme WRAP. Available at: http://www.wrap.org.uk/sites/files/wrap/CCC%20combined.pdf.
Madadi, A., Kurz, M. E., and Ashayeri, J. (2010). Multi-level Inventory Management Decisions with Transportation Cost Consideration. Transportation Res. E: Logistics Transportation Rev. 46, 719–734. doi:10.1016/j.tre.2009.12.012
McKinnon, A. C., and Ge, Y. (2006). The Potential for Reducing Empty Running by Trucks: A Retrospective Analysis. Int. J. Phys. Distribution Logistics Management. 36, 391–410. doi:10.1108/09600030610676268
McKinnon, A. (2018). Decarbonising Logistics: Distributing Goods in a Low-Carbon World. London, New York: Kogan Page Limited.
McKinnon, A. (2010). Green Logistics: The Carbon Agenda. Electron. Scientific J. Logistics. 6, 1–8. Available at: http://www.logforum.net/pdf/6_3_1_10.pdf.
McKinnon, A., Piecyk, M., and Validi, S. (2014). The Role of External Factors in the Decarbonisation of Companies’ Freight Transport Operations: German and UK Perspectives. Logistics Research Network 2014 Annual Conference. Huddersfield, England: University of HuddersfieldUniversity of Huddersfield.
McKinsey and Company (2017). Reinventing Construction: A Route to Higher Productivity. Shanghai, China: McKinsey Global Institute.
Ministry for Economic Development (Now Ministry of Business, Innovation and Employment), New Zealand Government (2007). New Zealand Energy Strategy to 2050. Wellington, New Zealand: Ministry for Economic Development.
Ministry for the Environment, New Zealand Government (MfE) (2021). Te Hau Mārohi Ki Anamata | Transitioning to a Low-Emissions and Climate-Resilient Future: Have Your Say and Shape the Emissions Reduction Plan. Wellington, New Zealand: Ministry for the Environment.
Ministry of Transport, New Zealand Government (MoT) (2020a). 2020 Green Freight Strategic Working Paper. Wellington, New Zealand: Ministry of Transport.
Ministry of Transport, New Zealand Government (MoT) (2020b). Government Policy Statement on Land Transport 2021. Wellington, New Zealand: Ministry of Transport.
Ministry of Transport, New Zealand Government (MoT) (2020c). Road Transport: Vehicle Kms Travelled (VKT). Available at: https://www.transport.govt.nz/statistics-and-insights/road-transport/sheet/vehicle-kms-travelled-vkt.
Ministry of Transport, New Zealand Government (MoT) (2021). Hīkina te Kohupara – Kia mauri ora ai te iwi Transport Emissions: Pathways to Net Zero by 2050. Wellington, New Zealand: Ministry of Transport. Available at: https://www.transport.govt.nz/assets/Uploads/Discussion/DiscussiondocumentHikinateKohuparaKiamaurioraaiteiwiTransportEmissionsPathwaystoNetZeroby2050.pdf
Morana, J., Gonzalez-Feliu, J., and Semet, F. (2014). “Urban Consolidation and Logistics Pooling,” in Sustainable Urban Logistics: Concepts, Methods and Information Systems EcoProduction (Environmental Issues in Logistics and Manufacturing). Editors J. Morana, J. Gonzales-Feliu, and F. Semet (Berlin: Springer), 187–210. doi:10.1007/978-3-642-31788-0_10
Muerza, V., and Guerlain, C. (2021). Sustainable Construction Logistics in Urban Areas: A Framework for Assessing the Suitability of the Implementation of Construction Consolidation Centres. Sustainability 13, 7349. doi:10.3390/su13137349
Naismith, N., Monaghan, B., Zhang, T., Doan, D., GhaffarianHoseini, A., and Tookey, J. (2016). Examining the Productivity in the NZ Construction Industry. Kuala Lumpur: The 2nd NZAAR International Event Series on Natural and Built Environment, Cities, Sustainability And Advanced Engineering.
New Zealand Transport Agency (NZTA) (2020). Arataki to Tātou Mahere Mō Te Pūnaha Waka Whenua: Our Plan for the Land Transport System 2021-31 Version 2. Wellington, New Zealand: New Zealand Transport Agency.
Organisation for Economic Cooperation and Development (OECD) (2002). OECD Guidelines towards Environmentally Sustainable Transport. Paris, France: OECD.
Organisation for Economic Co-operation and Development (OECD) (2011). Towards Green Growth: A Summary for Policy Makers May 2011. Paris, France: OECD. Available at: https://www.oecd.org/greengrowth/48012345.pdf.
Organisation for Economic Co-operation and Development (OECD) (2021). Transport Strategies for Net-Zero Systems by Design. Paris, France: OECD.
Piecyk, M. I., and McKinnon, A. C. (2010). Forecasting the Carbon Footprint of Road Freight Transport in 2020. Int. J. Prod. Econ. 128, 31–42. doi:10.1016/j.ijpe.2009.08.027
Punte, S., Tavasszy, L., Baeyens, A., and Liesa, F. (2019). Roadmap towards Zero Emissions Logistics 2050. Brussels, Belgium: Alliance for Logistics Innovation through Collaboration in Europe (ALICE-ETP). Available at: https://www.etp-logistics.eu/wp-content/uploads/2019/12/Alice-Zero-Emissions-Logistics-2050-Roadmap-WEB.pdf.
Ramesh, T., Prakash, R., and Shukla, K. K. (2010). Life Cycle Energy Analysis of Buildings: An Overview. Energy and Buildings 42, 1592–1600. doi:10.1016/j.enbuild.2010.05.007
Riazi, S., Zainuddin, M., Nawi, M., Musa, S., and Lee, A. (2020). A Critical Review of Fragmentation Issues in the Construction Industry. Talent Development Excell. 12 (2s), 1510–1521. doi:10.13169/intejcubastud.12.1.0155
Richardson, B. C. (2005). Sustainable Transport: Analysis Frameworks. J. Transport Geogr. 13, 29–39. doi:10.1016/j.jtrangeo.2004.11.005
Rohács, J., and Rohács, D. (2020). Total Impact Evaluation of Transport Systems. Transport 35, 193–202. doi:10.3846/transport.2020.12640
Salagnac, J., and Yacine, M. (1999). “Logistics: A Step towards Lean Construction,” in Proceedings of the 7th Annual Conference of the International Group on Lean Construction (IGLC) (Berkley, California, USA: IGLC), 121–132.
Sankaran, J. K., Gore, A., and Coldwell, B. (2005). The Impact of Road Traffic Congestion on Supply Chains: Insights from Auckland, New Zealand. Int. J. Logistics Res. Appl. 8, 159–180. doi:10.1080/13675560500166780
Schipper, L., Fabian, H., and Leather, J. (2009). Transport and Carbon Dioxide Emissions: Forecasts, Options Analysis, and Evaluation. Manila, Philippines: Asian Development Bank.
Seth, D., Nemani, V. K., Pokharel, S., and Al Sayed, A. Y. (2018). Impact of Competitive Conditions on Supplier Evaluation: A Construction Supply Chain Case Study. Prod. Plann. Control. 29, 217–235. doi:10.1080/09537287.2017.1407971
Sezer, A. A., and Fredriksson, A. (2021). Paving the Path towards Efficient Construction Logistics by Revealing the Current Practice and Issues. Logistics 5, 53. doi:10.3390/logistics5030053
Shakantu, W., and Emuze, F. (2012). “Improving H&S by Limiting Transport Externalities in South Africa,” in Proceedings for the 20th Annual Conference of the International Group for Lean Construction (IGLC) (San Diego, USA: IGLC).
Shakantu, W., Muya, M., Tookey, J., and Bowen, P. (2009). Conceptualising Reverse Logistics in a Construction Context: Re-Defining its Contours. J. Contemp. Management 6, 81–96. doi:10.10520/EJC51059
Shakantu, W., Muya, M., Tookey, J., and Bowen, P. (2008). Flow Modelling of Construction Site Materials and Waste Logistics. Eng. Construction Architectural Management 15 (5), 423–439. doi:10.1108/09699980810902721
Shakantu, W., Tookey, J. E., and Bowen, P. A. (2003). The Hidden Cost of Transportation of Construction Materials: An Overview. J. Eng. Des. Tech. 1, 103–118. doi:10.1108/eb060892
Silva, C. (2019). Auckland's Urban Sprawl, Policy Ambiguities and the Peri-Urbanisation to Pukekohe. Urban Sci. 3, 1. doi:10.3390/urbansci3010001
Smith, R., Kersey, J., and Griffiths, P. (2003). The Construction Industry Mass Balance: Resource Use, Wastes and Emissions {Vridis Report VR4 (Revised)}. UK: Vridis.
Sobotka, A., Czarnigowska, A., and Stefaniak, K. (2005). Logistics of Construction Projects. Foundations of Civil and Environmental Engineering 6, 203–216.
Szymonik, A. (2012). Logistics and Supply Chain Management. LodzPoland: Technical University of Lodz Press.
The Bellona Foundation (2020). Efficient Transportation to and from Construction Sites Can Reduce CO2 Emissions by 50%. Oslo, Norway: The Bellona Foundation.
Thomsen, A., and van der Flier, K. (2011). Obsolescence and the End of Life Phase of Buildings. Management and Innovation for a Sustainable Built Environment, 20 – 23 June 2011. AmsterdamDelft, Netherlands: OTB Research Institute for the Built Environment.
Transformative Urban Mobility Initiative (TUMI) (2019). Sustainable Urban Transport: Avoid-Shift-Improve (A-S-I). Bonn/Eschborn, Germany: Deutsche Gesellschaft für Internationale Zusammenarbeit (GIZ) GmbH.
Transport Policy Advisory Services (Germany) (2019). Sustainable Urban Transport: Avoid-Shift-Improve (A-S-I). Berlin, Germany: Federal Ministry for Economic Cooperation and Development.
United Nations Conference on Trade and Development (UNCTAD) (2017). UNCTAD Framework for Sustainable Freight Transport. New York and Geneva: UNCTAD.
United Nations Environment Programme (UNEP) (2020). 2020 Global Status Report for Buildings and Construction: Towards a Zero-Emission, Efficient and Resilient Buildings and Construction Sector. Nairobi, Kenya: UNEP.
Van Essen, H., Schroten, A., Otten, M., Sutter, D., Schreyer, C., Zandonella, R., et al. (2011). External Costs of Transport in Europe, Update Study for 2008. Netherlands: CE Delft.
Vrijhoef, R., and Koskela, L. (2000). The Four Roles of Supply Chain Management in Construction. Eur. J. Purchasing Supply Management. 6, 169–178. doi:10.1016/S0969-7012(00)00013-7
Vrijhoef, R. (2015). “Reducing the Environmental Impact and Improving Efficiency of Construction Transport,” in Proceedings of the Joint CIB Symposium Going North for Sustainability: Leveraging Knowledge and Innovation for Sustainable Construction and Development. London, UK: London South Bank University), 23–25.
Wegelius-Lehtonen, T. (2001). Performance Measurement in Construction Logistics. Int. J. Prod. Econ. 69, 107–116. doi:10.1016/s0925-5273(00)00034-7
Ying, F., and Roberti, H. (2013). Improving Construction Logistics. BUILD 134(February/March 2013). Available at: https://www.buildmagazine.org.nz/assets/PDF/Build-134-80-Research-Improving-Construction-Logist ics.pdf?
Ying, F., and Tookey, J. (2014). Managing Logistics Cost – A Missing Piece in Total Cost Management. Milano, Italy: International Cost Engineering Council IX World Congress.
Ying, F., Tookey, J., and Roberti, J. (2014). Addressing Effective Construction Logistics through the Lens of Vehicle Movements. Eng. Construction Architectural Management. 21, 261–275. doi:10.1108/ECAM-06-2013-0058
Keywords: construction logistics, construction transport, green transport, sustainable construction, transport decarbonisation
Citation: Dhawan K, Tookey JE, GhaffarianHoseini A and GhaffarianHoseini A (2022) Greening Construction Transport as a Sustainability Enabler for New Zealand: A Research Framework. Front. Built Environ. 8:871958. doi: 10.3389/fbuil.2022.871958
Received: 08 February 2022; Accepted: 21 March 2022;
Published: 25 May 2022.
Edited by:
Muhammad Jamaluddin Thaheem, Deakin University, AustraliaReviewed by:
Sikandar Bilal Khattak, University of Engineering and Technology, Peshawar, PakistanMaria Ghufran, National University of Sciences and Technology (NUST), Pakistan
Copyright © 2022 Dhawan, Tookey, GhaffarianHoseini and GhaffarianHoseini. This is an open-access article distributed under the terms of the Creative Commons Attribution License (CC BY). The use, distribution or reproduction in other forums is permitted, provided the original author(s) and the copyright owner(s) are credited and that the original publication in this journal is cited, in accordance with accepted academic practice. No use, distribution or reproduction is permitted which does not comply with these terms.
*Correspondence: Kamal Dhawan, a2FtYWwuZGhhd2FuQGF1dC5hYy5ueg==