- 1Department of Civil and Architectural Engineering, College of Engineering, Qatar University, Doha, Qatar
- 2Institute of Structural Analysis and Antiseismic Research, School of Civil Engineering, National Technical University of Athens, Athens, Greece
- 3School of Engineering and Applied Sciences, University of the District of Columbia, Washington, DC, United States
Blockchain is a technology that allows the recording of information in a way that it is difficult or practically impossible to alter, hack, or cheat. It is a new, promising technology, considered by many as a general-purpose technology (GPT). GPTs are technologies that have the potential to affect an entire economy, impacting economic growth and transforming both everyday life and the ways in which we conduct business. We present a bibliometric analysis of the relevant literature, followed by a discussion about monetary mediums and the evolution of bitcoin, as the first digital medium managing to solve the “double-spending” problem and the first successful implementation of blockchain technology. The computational operations involved in blockchain are presented, together with the cryptographic technologies associated with it, its unique characteristics, and the advantages it offers as a technology. A comprehensive literature review is provided, of the current state of the art in blockchain in the fields of civil engineering, architecture and the construction industry. Six important application areas are identified, and the relevant literature is investigated. Namely, building information modelling and computer aided design, contract management and smart contracts, construction project management, smart buildings and smart cities, construction supply chain management, and real estate. Finally, we discuss the future applications, the challenges and the opportunities that blockchain technology brings to these fields.
1 Introduction
Construction is arguably one of the largest industries in the world, creating infrastructure which is the backbone of productivity and economic growth. The Architecture, Engineering, and Construction (AEC) industry has entered a period of major disruption caused by a host of new and more mature digital technologies, such as Artificial Intelligence (AI), the Internet of Things (IoT), Virtual Reality (VR), Geographic Information Systems (GIS), digital photogrammetry, Building Information Modelling (BIM), 3D printing, laser scanning, global positioning systems (GPS), radio frequency identification devices (RFID), augmented reality (AR), sensors, robotics, big data management, and others (Wang et al., 2020). These technologies have been proven helpful due to the numerous benefits they offer to project stakeholders, such as increased productivity, reduction in building waste, sustainable performance, enhanced visualization, safety improvement and improved data sharing.
Lately, another innovative digital technology, blockchain, has appeared, promising to change the way people do transactions, keep records, validate data and much more. For many, blockchain is a new general-purpose technology that can transform both our everyday lives and the ways we do business. Bitcoin was the first successful application of this technology and its first description as an algorithmic idea can be found in the work by (Nakamoto 2008). Blockchain was the technology which allowed Bitcoin to transfer value in a decentralized network, for the very first time in history. Many desirable characteristics of blockchain already exist in Bitcoin, even though Bitcoin was only the first application of the technology and further developments and improvements have been made since. For this reason, the present study will start with discussing monetary mediums, problems related to transferring value using digital currency and how blockchain technology managed to solve the well-known “double spending” problem in digital currency, opening horizons for other important applications of the technology.
Although blockchain is still at its early stages today, it probably has the potential to play a significant role in construction industry in the future, or even reshape it drastically to the better (Shojaei 2019). explored the applications of blockchain in improving information management systems in the construction industry. The author concludes that blockchain has the potential of addressing common problems of the construction industry, while it can be adaptable to the construction industry structure and the way it is practiced (Nawari and Ravindran 2019b). reviewed blockchain and how it is related to the built environment. They explored potential applications in the AEC industry, focusing mainly on Building Information Modeling (BIM) and highlighting its potential and its limitations.
(Tezel et al., 2022) examined blockchain opportunities and related issues in the Built Environment, with particular emphasis on its potential influence on trust, transparency and cybersecurity. The authors also provide directions for future research, contributing to the cyber-physical convergence in Construction 4.0 (Li et al., 2019). identified recent challenges in the construction industry and explored blockchain as a potential solution to some of these challenges. They performed a review of blockchain uses in the built environment and identified seven distinct areas of applications, namely 1) Smart Cities and the Sharing Economy, 2) Smart Energy, 3) Smart Homes, 4) Smart Government, 5) Intelligent Transport, 6) BIM and Construction Management, and 7) Business Models and Organizational Structures.
(Cheng et al., 2021) reviewed the present status and investigated the benefits, challenges, and future research opportunities of blockchain meeting the AEC industry. Their results show that relevant research on blockchain remains new and fragmented. Nevertheless, they managed to identify five relevant areas of benefit, namely 1) supply chain management, 2) information management, 3) contract management, 4) integration management, and 5) stakeholder management (Shojaei et al., 2021). investigated blockchain as a promising technology for the facilitation of a circular economy (CE) in the built environment. They presented and tested a blockchain model through a synthetic case study to provide a proof of concept as to the feasibility of blockchain as an enabler of a CE in the built environment. They conclude that blockchain is shown to be a feasible and novel approach for employing CE concepts in the built environment domain as it can provide full material and energy traceability, enabling the user to make predictions for the recycling and reuse of materials and goods used in the built environment.
The aim of this study is to formulate a picture of the current state and practice of the use of blockchain technology in fields related to the built environment such as civil engineering, architecture and construction industry areas, to identify the current state of the art and to examine the challenges and opportunities ahead. The study also summarizes and highlights specific application areas related to the AEC industry, where blockchain has the potential to provide new solutions, and how these solutions can be adopted to improve performance, sustainability, and safety in the future. The structure of the paper is as follows: Section 1 is the introduction, followed by the bibliometric analysis of Section 2. Section 3 presents the idea of monetary mediums, from bartering to digital money, the double spending problem and how it was first solved using blockchain technology. Section 4 focuses on technical details of the technology, while Section 5 discusses the applications of blockchain in the civil, architectural and construction industry, where six application areas as identified and discussed in detail. The conclusions and a relevant discussion are provided in Section 6.
2 Bibliometric Analysis
2.1 Papers Published in the Field
A simple look at the most recent scientific literature can reveal how important blockchain has become for the scientific community lately. The word “blockchain” returns 28,600 document results in Scopus when searching within “Article title, Abstract and Keywords” (Query string: “TITLE-ABS-KEY (blockchain)”). 28,355 of these results (99.14%) have a publication year of 2017 or later (i.e. last 5 years), as only 245 papers were published in the topic between 1990–2016. Even more interestingly, 85.77% of the results (24,531 documents) have a publication year of 2019 or later, i.e. published in the last 3 years. The same search, limited within the “Engineering” field only (Query string: “TITLE-ABS-KEY (blockchain) AND (LIMIT-TO (SUBJAREA,"ENGI”))”) returns 11,969 document results. These queries were made on 6 March 2022. Figure 1 shows these search results, per year, for the years from 2013 to 2021. Year 2022 was excluded from the plot as it is still a year in progress. It has to be noted that even for year 2021 the process of indexing and adding papers is still a work in progress in Scopus. The exponential growth in the production of scientific papers is clearly visible, showing the latest impact of blockchain in all scientific fields, engineering included.
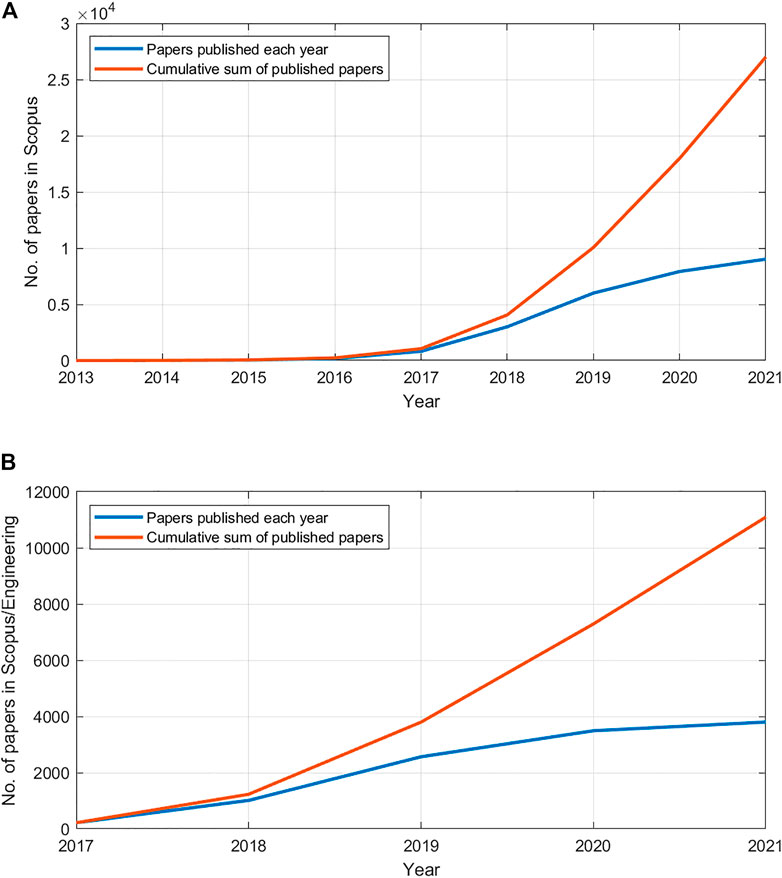
FIGURE 1. Papers in “blockchain” in Scopus database, per year: (A) General search (all fields), (B) Search limited within the “Engineering” field.
2.2 Top Keywords
We performed a co-occurrence analysis of the top keywords, including author keywords and index keywords from the Scopus database. For this, we search Scopus with the word “blockchain” within “Article title, Abstract and Keywords” and we limit the search to the “Engineering” field and also to the years 2017–2022 (6 years in total). The full query string, performed on 7 March 2022, is therefore “TITLE-ABS-KEY(blockchain) AND (LIMIT-TO ( SUBJAREA,“ENGI”) ) AND (LIMIT-TO ( PUBYEAR, 2022) OR LIMIT-TO (PUBYEAR, 2021) OR LIMIT-TO (PUBYEAR, 2020) OR LIMIT-TO (PUBYEAR, 2019) OR LIMIT-TO (PUBYEAR, 2018) OR LIMIT-TO (PUBYEAR, 2017))”. The query returned 11,926 documents. Within this result, we found the top-30 keywords of the papers. Similar keywords needed to be merged manually, as follows: blockchain (block-chain, blockchains, blockchain technology), internet of things (iot, internet of things (iot), internet of thing (iot)), smart contract (smart contracts), supply chain (supply chains, supply chain management), building information model (bim, building information model - bim, building information modeling, building information modelling), where the word in bold is the main keyword and the words in parentheses are the different variations that have been merged within the main keyword. The network visualization of the co-occurrence of the top-30 keywords is presented in Figure 2, generated using the VOSviewer software (van Eck and Waltman 2007), with 5 clusters presented with different colors, and minimum strength equal to 60.
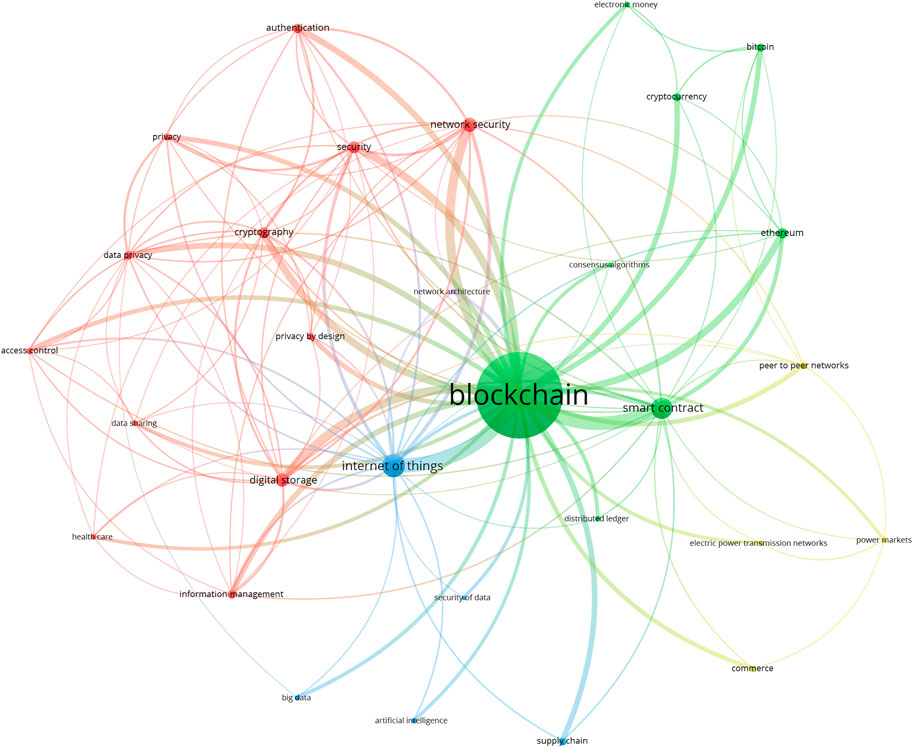
FIGURE 2. Bibliometric map of the co-occurrence of top-30 keywords of documents including “blockchain” in the “Engineering” field, in Scopus (2017–2022).
In this map, the links (lines) between keywords express the frequency of co-occurrence of the keywords in the documents, while the size of each bubble (keyword) expresses the number of occurrences of a specific keyword. As expected, the keyword “blockchain” appears in the center of the network as the strongest keyword (10,135 occurrences), followed by “internet of things” (2072), “smart contract” (1873), “network security” (1,168), “digital storage” (1,058) and “security” (928). Interestingly, the keyword “bitcoin” appears as a small bubble on the top-right, which shows that despite it being the first application of blockchain, it is now somehow isolated as simply one of the numerous applications of the broader technology.
A clear cluster related to engineering applications is the yellow one, having to do with power markets and electric power transmission networks. Interestingly, keywords related to the civil engineering and the construction industry are not present in this network visualization, although the search has been limited to the “engineering” field. This shows that blockchain applications in these areas are still at a very premature state. It also confirms that the fact that the construction industry has been traditionally slow in adapting new digital technologies.
Next, we limit the search to areas related to civil engineering and the construction industry. For this, we search within the “Article title, Abstract and Keywords” with the query “blockchain AND (“construction industry” OR “civil engineering” OR “construction management” OR “building information modelling” OR “smart buildings” OR “real estate")”, which returns 365 document results. We apply the same technique for merging similar keywords as we did before. Setting a minimum strength of connections equal to 10, and 5 clusters, we obtain the bibliometric map of Figure 3.
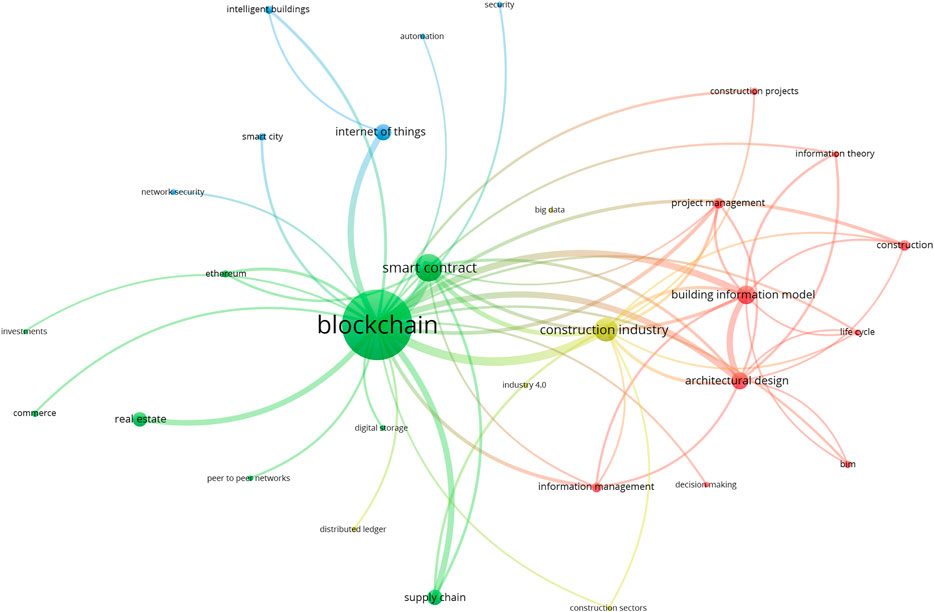
FIGURE 3. Bibliometric map of the co-occurrence of the top-30 keywords of documents including blockchain in the civil engineering and the construction industry, in Scopus.
Again, the keyword “blockchain” is in the center of the network as the strongest keyword (280 occurrences), followed by “smart contract” (91), “construction industry” (76), “building information model” (56), “architectural design” (50), “internet of things” (49), “supply chain” (45) and “project management” (28). This map reveals some trends and the most important application areas of the technology in the civil engineering and the construction industry fields. It also shows the connections of different applications, for example the construction industry (as a central node) with BIM, architectural design, smart contract, information management and others.
2.3 Top Countries
Figure 4 is based on the same data from Scopus as Figure 3, but now the focus is on the co-authorship of the top countries in the field. Setting the minimum documents for a country to 2, the largest set of connected countries is found to be 27. The map is presented in Figure 4 with the minimum connection strength set equal to zero.
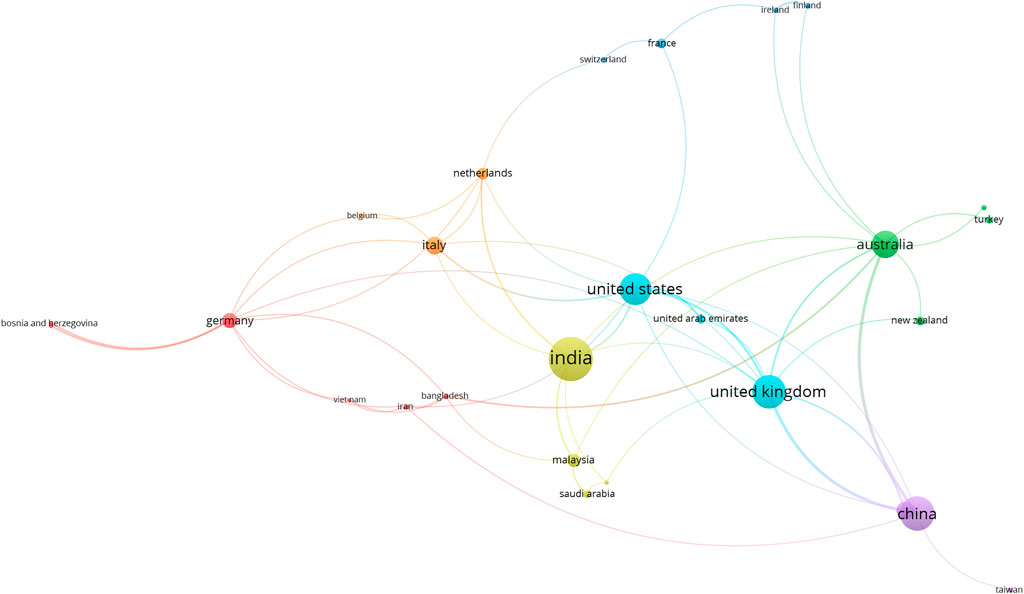
FIGURE 4. Bibliometric map of the co-authorship of the top countries with documents including blockchain in the civil engineering and the construction industry, in Scopus.
In this map, the links (lines) between countries represent the frequency of co-authorship between the countries, while the size of each bubble (country) expresses the number of publications in the field by a specific country. In terms of the number of documents published in the field, the most active country is India with 47 documents and 157 citations, followed by China (35, 446), the United Kingdom (34, 388), the US (32, 505), Australia (26, 440), Hong Kong (16, 135) and Italy (16, 44). It is not surprising that India leads this map, as in India IT is a vast industry comprising information technology services, consulting, and outsourcing, accounting for 8% of India’s GDP in 2020.
3 Monetary Mediums: From Bartering to Digital Money
In the long past, there were no monetary mediums. People in primitive societies would simply exchange goods using a fair barter arrangement. Bartering has to do with the exchange of services and goods between two people or parties without the use of money. It is based on equivalent estimates of the value of services and goods. This approach has significant limitations, one of the most important ones being not being able to store wealth in the long term.
Then came money, a monetary medium in the form of a metal coin, a piece of paper or even a shell or other object, that people could use as a medium of exchange, a unit for measuring value and a means to store wealth. The value of money depends mostly on the importance that the people place on it. The invention of money had an important role in the evolution and development of human societies as it allowed people to trade services and goods indirectly, providing also a very effective and direct way to store wealth. Historians believe that objects made of metal were first used as money as early as 5,000 BC. Around the second half of the 7th century B.C., the Lydians were the first western civilization to make coins. The Lydian stater was the first coin officially issued by a state in the world history and was the model for virtually all subsequent coinage (Kroll 2012). Later on, other civilizations began to mint their own coins, having specific properties and values. Around 700 B.C., the Chinese moved to paper money. In Europe, the first banknotes were issued in 1,666 by the Bank of Stockholm while in the US, the Massachusetts Bay Colony was said to have issued the first paper money in 1,690.
3.1 Digital Money and the Need for a Trusted Third Party
The 21st century and the evolution of computers and digital technology gave rise to a new form of currency, digital money, allowing digital, mobile payments between different parties. Mobile payments can be used to buy a service or product through the use of a portable electronic device, such as a smartphone, tablet, laptop or desktop computer, to name a few. Money nowadays needs not have a physical form. It can merely sit in bank accounts, waiting to be used online for whatever necessary. Mobile payments offer the advantage that the parties involved in a transaction do not have to be in the same physical location for a successful transaction to take place. Unlike hand-to-hand physical payments by cash, digital payments can be made remotely, through an institution (usually a bank). The bank acts as an intermediary or a “trusted third party”. When Alice pays Bob the amount of $100 remotely, she makes the relevant request at a bank online. The bank needs to verify and process the transaction. First, it checks if Alice has $100 at her disposal, in her account. If yes, it proceeds with the payment to Bob. For the bank this is simply a couple of records in the transactions’ ledger. The bank will debit Alice’s account with the amount of $100 and simultaneously credit Bob’ account with the same amount. Everything happens at the level of the bank ledger which is a “book” in which account transactions are recorded. In such bookkeeping system with double entries, credits and debits are simply entries that are made in the account ledgers to record changes in value, as a result of transactions.
As mentioned, such online payments need a trusted third party to act as an intermediary. Unlike cash transactions, that are truly “peer-to-peer” from one party to the other, traditional online payments need to go through a bank. This causes problems and difficulties in certain cases. First of all, the bank needs to be online, it needs to be working during the transaction and it needs to be there to validate the payment. Although these may seem to be small problems in the real world, there are other more significant problems and difficulties arising from the fact that an intermediary is always necessary. For example, banks may delay or even censor transactions, while in some cases transaction fees may be too high. This is the case with international payments between two banks in different, distant countries and especially when a third world country is involved. In addition, one needs to open a bank account to send or receive such payments. All banks have specific regulations. There are certain requirements for opening an account and rules on who can pay or who can get paid. According to the 2019 National Survey of Household Use of Banking and Financial Services by FDIC (Federal Deposit Insurance Corporation 2020), 5.4% of households in the US had no access to a checking or savings account at a bank or credit union. This represents approximately 7 million U.S. households or a total of approximately 14 million American adults who are literally unbanked. Interestingly, nearly half of them reported that they did not have a bank account due to lack of enough money to meet minimum requirements, while approximately 1/3 of unbanked households reported that they did not have an account because they do not trust banks (Federal Deposit Insurance Corporation 2020). Privacy is a major concern as a lot of personal information is revealed in every transaction and the bank or a bank employee have access to this sensitive information.
3.2 The “Beauty” of Cash, Digital Cash and the “Double-Spending Problem”
Cash payments do not have these issues. The “beauty” of cash lies in its simplicity and on the fact that it is truly “peer-to-peer”, without the need of any intermediaries. But cash has other problems: For example, when Alice gives Bob a bank note, the two parties need to be in the same place at the same time for the physical object (the bank note) to be handed from one person to the other. In addition, cash is bulky and therefore it cannot be easily used for big transactions. At this point the question arises: Could we have online, digital payments that are just like cash, i.e. peer-to-peer, with no need for intermediaries, no banks, no delays, no censorship and no privacy issues? Is there a way to have digital money that would behave just like cash?
Many researchers had tried to deal with this issue, but they faced the so called “double-spending problem.” Unlike physical currencies that cannot be easily replicated, digital currencies can be easily reproduced digitally. Double spending has to do with the problem of a digital currency or token being spent twice, which should not be allowed in a fair system. In other words, if somebody tries to use a digital token for a second time, after it has been already spent, the system should be able to detect it and reject the second transaction.
3.3 The Solution to the Double-Spending Problem
In 2008, Satoshi Nakamoto introduced Bitcoin to the world. In his novel work (Nakamoto 2008) the brilliant idea of a system for electronic transactions which does not rely on a “trusted third party” is explained. This system was the first successful application managing to solve the double-spending problem and the first implementation of blockchain technology. It is a peer-to-peer electronic cash system which uses cryptography and the concept of “proof-of-work” (PoW) to record the history of transactions. The real identity of Satoshi Nakamoto remains a matter of dispute until today.
The code of Bitcoin was released in 2009, after the original paper was published in 2008. In Bitcoin, there is no bank, no central system, no single institution in the middle playing a special role. That’s why it is called a “decentralized network”. All parties in this network are equal and there is no central authority or special player. The technology that enabled Bitcoin to make this breakthrough, is called blockchain. Bitcoin and other cryptocurrencies, all based on blockchain technology, offer peer-to-peer transactions with privacy for any amount of money, just like cash.
The system uses a decentralized approach which is based on the creation of blocks which are linked together, forming a chain of blocks, the so-called “block chain”. This is what we nowadays call blockchain technology. In the blockchain, every transaction and every block have a timestamp and blocks are linked together with their hash values and “proof-of-work”. The record of a transaction is distributed among many nodes in the system which makes it practically impossible for a bad actor to gain control of the system and manipulate the ledger to their advantage. Using proof of work, the amount of computational power needed to reverse or change a transaction is enormous. This technology allows bitcoin to transfer value in a decentralized way without the need for any intermediary, or a trusted third party such as a bank. In addition, the system uses public-key cryptography which allows users to transact anonymously or more accurately, pseudonymously.
Bitcoin has a market capitalization of more than $700 billion (as of 6 March 2022) and it is the largest application of blockchain technology until today. Despite its widespread fame and its high value, Bitcoin itself remains a controversy. For many, it is the ultimate democratic tool and the currency of the future. They highlight its advantages, such as payment freedom, availability, total control of one’s money, security, transparency and low fees. On the other hand, some economists and other experts have characterized Bitcoin as a speculative bubble or even an advanced Ponzi scheme. They criticize Bitcoin for its use in illegal transactions, large carbon footprint, price volatility, scalability problems and low speed.
4 Blockchain
4.1 Technical Details
4.1.1 Asymmetric Cryptography
Asymmetric cryptography, also known as public-key cryptography uses a pair of keys, a Public and a Private key to encrypt and decrypt a message. Each user has a pair of keys. The two keys are mathematically related. The private key cannot be shared with others and works similarly to a password, while the public key can be shared with and is visible to everyone. Both keys are needed to perform an operation. A message which is encrypted with the private key can only be decrypted with the public key, and vice-versa: a message or data encrypted with the public key can only be decrypted with the private key. Based on which key is used first, there are two main uses of asymmetric cryptography, as shown schematically in Figure 5.
In the first case depicted in Figure 5, called Digital signature or Sender authentication, data is encrypted with the private key of the sender and can be decrypted with the corresponding public key. This ensures that the message came from the stated sender. Example: Alice sends a message to Bob and she encrypts it with her private key. Bob receives the message. He decrypts it using the public key of Alice and that guarantees that the message came indeed from Alice.
In the second case, called Digital envelope or Receiver authentication, data is encrypted with the public key of the recipient and can be decrypted with the corresponding private key. This ensures that the message can only be read by the intended recipient. Example: Alice sends a message to Bob and she encrypts it using the public key of Bob. Then Bob is the only one who can decrypt and read the message as only he has access to the pairing private key. This ensures confidentiality of a message.
Together, the two keys help ensure the security of the exchanged data. Asymmetric encryption has many applications such as in key exchange, email security and web security. In Bitcoin and other blockchain applications, asymmetric encryption is used to ensure the integrity of transactions. Bitcoin uses the ECDSA algorithm (Elliptic Curve Digital Signature Algorithm) and in particular the Secp256k1 elliptic curve and the double-SHA hash to implement its public key cryptography.
When a person creates a crypto wallet, the system generates a pair of keys. The private key can generate the public key, but the public key cannot be converted back into the private key. The public key itself is not a bitcoin address. The address of a wallet, which is similar to a bank account number (where one can receive payments) is derived from the public key by putting the public key into a hash function (see next subsection). Bitcoin addresses are 34-digit alphanumeric. An example Bitcoin address is “1J7mdg5rbQyUHENYdx39WVWK7fsLpEoXZy”. Such an address appears most commonly as the recipient of funds. Note that several of the characters in an address are used as a checksum. This way typographical errors can be automatically found and rejected by the system.
On the other hand, the private key is used for the digital signature of the transactions, and it provides access to the funds in the wallet. In other words, it is equivalent to a password or PIN code that provides control over a bank account. Mathematically speaking, a signature is generated from a hash of something to be signed, plus a private key. When Alice sends Bob an amount of Bitcoin, she presents her public key and a signature (transaction fingerprint). The signature, which is different each time, is created with her private key and can only be produced by someone who has access to Alice’s private key. However, anyone in the network can verify the signature if they have access to the public key and the transaction fingerprint. In other words, it is ensured that only Alice, having the private key, can spend the bitcoin she has in her account, but anyone in the network, having her public key, can easily verify that it is Alice who made the payment.
4.1.2 Cryptographic Hash Functions
A hash function is a one-way function that maps data of an arbitrary size to fix-sized values (Estébanez et al., 2014). The return of a hash function is called the hash value, digest, hash code, or simply hash. A hash function is deterministic, meaning that for a given input value it should always generate the same output. Hash functions are designed to be irreversible, a property which is usually referred to as pre-image resistance, which means that it is not possible to generate the input from the hash, and therefore a hash function is essentially a one-way function.
A cryptographic hash function has additionally some desired special properties:
• Quick computation: The hash value is computationally inexpensive to compute, for a given message.
• It is practically impossible to generate a message that will give a given hash
• Collision resistance: Practically, it is impossible to find two messages giving the same hash value.
• Avalanche effect: A slight change in a message will result to a drastic change in the hash value, so that the new hash appears to be completely different and uncorrelated to the old one.
Bitcoin uses the SHA-256 hashing algorithm, which belongs to the SHA-2 set of cryptographic hash functions designed by the US NSA (National Security Agency). The algorithm was first published in 2001 (Penard and van Werkhoven 2008). Irrespective of the size of the message, the hash values of SHA-256 will always be 256 bits and they are represented by 64 hexadecimal “digits” (each one taken from the set “0123456789abcdef” containing 16 such “digits”).
The value set of SHA-256 contains 2256 = 1664 ≈ 1077 different message digests, an unfathomably large number considering that, for example, it is estimated that there are between 1078 to 1082 atoms in the known, observable Universe. Examples of SHA-256 hash values can be found in Table 1. One can see that the hash value of the message “This is a message” is completely different than the one of “This is a message.” with an added dot in the end, which shows the avalanche effect. Also, it is seen that the hash value remains always the same length (64 digits), even for longer messages.
Other than Bitcoin, the SHA algorithm is used in many applications, such as Digital Signature Verification, Secure Sockets Layer (SSL) Handshake, Integrity Checks, Password Hashing and others. For example, using password hashing, the password of a user need not to be stored in a website’s database. Instead, the hash value of the password is stored. This is enough to validate a given password when a user tries to log in. On the other hand, if a hacker gets access to the database, all they will find is the hashes of the passwords and not the passwords themselves, which increases security.
4.1.3 Blockchain Definition, Characteristics and Structure
Distributed Ledger Technology (DLT) or simply blockchain is a technology able to simplify and secure transactions among parties and record keeping in general. It has to do with a growing number of blocks containing records. These records are usually transaction data and timestamps, but it can be virtually anything that can be recorded. The blocks are linked together using hash values created with a cryptographic hash function. The innovation lies in the connection of each new block of data with the previous one, using the cryptographic hash. In particular, the hash value of a given block is part of the information stored in the next block. Any small change in a block would lead to a new hash value for the block, which would automatically invalidate all subsequent blocks. In addition, the ledger is not stored in a central location, but it is distributed in thousands of copies among the nodes of the network, which are also asked to validate the blocks containing the transactions. Blockchain technology uses a decentralized architecture based on distributed computing, crypto-chain block structures to store data, node consensus algorithms to verify data and smart contracts to program data (Xu et al., 2021).
The structure of a blockchain is depicted in a simplistic way in Figure 6. Note that in this example, for illustration purposes, we use simple hash values with 8 digits instead of real SHA-256 hashes with 64 digits. After several transactions have taken place within a predefined time interval, a miner node will pack the transaction data into a new block, together with some additional information, with a timestamp and a signature. As shown, each block contains the hash value of the previous block, making a chain of blocks. The miner node will send the package to all the other nodes of the network. After the block is validated by the other nodes, it is added to the main chain and all the nodes of the network are synchronized with the latest main chain. Each block has its unique hash value, which is not included in the block itself (it can be easily calculated anytime), but it is added to the next block in the chain.
In Bitcoin and similar systems relying on blockchain to transfer value, the steps needed for a transaction to get into the blockchain are the following:
• A peer-to-peer transaction is requested by a user.
• Each user has a private key and a public key. A transaction is signed with the private key of the user, for authentication purposes.
• The transaction is sent first to the closest node of the network, and it is verified and propagated to other nodes.
• The transaction waits until a miner picks it up to include it in the next block to be mined
• The transaction, together with a number of other transactions are packed together in a block by a miner. Other miners may try to create other blocks using probably different combinations of transactions.
• The first miner achieving to solve the “Proof of Work” cryptographic puzzle adds the block to the main chain and receives a reward in cryptocurrency
• The update in the blockchain is distributed across the network
• Other nodes verify the result and propagate the block
• The transaction is complete.
If a bad actor goes back in time and tries to change a transaction record in a given old block, this will cause a change in the hash of the block and the block will be no longer connected to the next block in the chain. Such an attempt will be easily spotted and be denied by the decent nodes of the blockchain network.
4.1.4 Proof of Work
As mentioned, calculating the hash function of a block would be a computationally easy operation as SHA-256 hashes are easy to calculate on any modern computer. In other words, generating any hash for a given set of transactions is trivial. The bitcoin protocol and other similar blockchain networks make this harder by introducing a level of difficulty in the hashing operation. In particular, a miner has to add some special info into the header of the block, an integer number known as a nonce (“number used once”), to achieve a hash that has a value which is lower than a predefined threshold value, or in other words it has a number of leading zeros. Since hash values cannot be predicted and the outcome is completely “random” (although deterministic), a miner node has to try many times with different nonces until it finds the right nonce that will give the hash with the desired properties.
In Bitcoin, this mechanism aims to add a new block to the blockchain every 10 min, on average. To do so, it adjusts the difficulty of the cryptographic puzzle depending on how quickly miner nodes are adding blocks. If miners have high computational power and add blocks too quickly, the difficulty increases, and hash computations become harder. In contrast, if blocks are added too slowly, hash computations become easier. This concept, called “Proof of work” (PoW) is a consensus mechanism requiring members in a decentralized network to do some computational work in order to prevent bad actors from gaming the system.
In the simple example of Figure 6, we see that the hash values of the blocks, all have 3 leading zeros, which means that some “work” was needed for finding the proper nonce that would provide the hash for each block. As a practical example and a case study, Table 2 shows some properties of Block 712,650 of the Bitcoin blockchain, mined on 05 December 2021 at 7:45 AM (GMT+3). We see that the block has a hash value with 19 leading zeros. To achieve this number of leading zeros, one would need to try on average approximately 1619 ≈ 7.55579∙1022 hashes with different nonces. A more accurate estimation for the needed number of tries is in fact given by formula D∙232, where D is the difficulty of the block, shown in Table 2 for Block 712,650. In our case, this formula will result to 22335659268936.39*232 = 9.59309∙1022 hashes. Since a block is created every 10 min (600 s) on average. In Table 2, we see that the hash value of the block has 19 leading zeros (in bold), as a result of mining and the relevant difficulty. The computational power of the bitcoin network for the time period related to the generation of block 712,650 can be calculated as 9.59309∙1022 hashes/600 s ≈ 160 million TH/s (tera hash per second) = 160 EH/s (exa hash per second). This shows how computationally demanding Bitcoin mining has become lately. It also has a huge effect on the carbon footprint of Bitcoin.
Miners race and compete to solve the cryptographic puzzle first, i.e. to find the nonce that produces a target hash that is below the threshold set by the block difficulty. The winning miner receives two kinds of reward: 1) in the form of newly mined crypto coins (currently 6.25 BTC per block), and 2) transaction fees. In the example of Block 712,650, the miner will receive 6.25 + 0.20322136 = 6.45322136 BTC as the total reward.
The first reward is set by the bitcoin protocol. The rate at which new Bitcoins are generated (mined) is reduced over time, as rewards are halved every approximately 4 years. Today, there have been three halving events. When bitcoin started in 2008, the reward was 50 BTC which was halved to 25 BTC in 2012, 12.5 BTC in 2016 and lately 6.25 BTC in 2020. Bitcoin last halved on 11 May 2020, around 3 p.m. EST, resulting in a block reward of 12.5/2 = 6.25 BTC. Halving occurs after every 210,000 blocks are mined. Given that a block is produced every 10 min on average, 210,000 blocks require 2,100,000 min which is 1,458.33 days or 3.99 years (considering each year equal to 365.25 days). The maximum supply of BTC coins will be 21 million coins, after all bitcoins have been “mined”.
Eventually, after all bitcoins have been generated, miners will keep receiving only the second reward, in the form of transaction fees. Transaction fees are therefore used to 1) avoid spam transactions in the bitcoin network, and 2) give incentive to the miners to keep mining even after the generation of new bitcoin has come to end. Transaction fees also reflect the speed with which one would like their transaction to be validated in the system. Transactions with higher fees are more likely to be processed first, as miners have the incentive to include them in their blocks, in order to get a higher cumulative mining reward.
PoW is an efficient mechanism which protects the network against malicious and fraudulent actors. In a hypothetic scenario concerning Block 712,650, we suppose that after 30 min another 3 blocks have been added to the chain (i.e. blocks 712,651, 712,652, 712,653) and at that time everybody is working on making the next block, i.e. block 712,654. Bill, a bad actor, instead of building the next block wants to alter a transaction in block 712,650 to his favor. If Bill does that, then the hash of the block will be changed and the link of the blockchain will be broken. The community will simply reject the copy of the blockchain presented to them, unless Bill manages to properly “redo” the next blocks, i.e. 712651, 712,652, 712,653 before the other nodes manage to generate block 712,654. This is the only way that Bill can cheat the network and alter an existing transaction in the blockchain. To do so, Bill must be fast enough, which means he needs to have at least 51% of the computational power of the whole network, which is very difficult to achieve. If 51% or more of the computational power remains in good hands, then this assures that the network will work in a decent way, rejecting such fraudulent attempts.
4.1.5 Proof of Stake: An Alternative to PoW
Validators in a blockchain network have to carry out the task of appending a block of transactions to the blockchain. In most cases, they will receive a reward for doing so. For security purposes, the blockchain protocol must have a mechanism to prevent a bad actor from taking over the majority of validation. PoW systems use validation based on computational power to verify transactions, which incentivizes consuming huge quantities of energy. Although PoW offers some advantages, such as a decentralized way of verifying transactions, high security level, and allowing miners to earn rewards, it also has disadvantages and limitations. Its main drawbacks are high energy usage, slow transaction speeds, and scalability problems. PoW has mathematical limitations on scalability, as the block size and the block creation frequency need to be within certain bounds, to maintain security. For Bitcoin, these bounds set a limit of a few transactions per second (TPS), far below requirements for worldwide adaption and practical applications at a global scale. At this time, Bitcoin executes around 5 TPS, compared, for example, to VISA’s 24,000 TPS. To make things even worse, the energy needed for a single Bitcoin transaction is equivalent to the one needed for several hundreds of thousands of VISA transactions.
Another promising approach is Proof of Stake (PoS), which extends the voting power to the stakeholders of the system. In PoS, participants owning crypto coins can stake them, which will give them the right to check new blocks, validate them and add them to the blockchain. The first functioning implementation of a PoS cryptocurrency was Peercoin, introduced in 2012. PoS is tremendously more energy-efficient than PoW (Bach et al., 2018). did a comparative analysis of typical consensus mechanisms and some of their contemporaries that are in use in modern blockchains. The analysis focused on the algorithms, scalability issues, reward mechanisms and security risks.
In PoS, when a block is ready, the system chooses a node to act as its reviewer and validator. The validator will check if the transactions are accurate. In this case, they will add the block to the blockchain and they will receive a reward. The probability of being selected to act as a validator is proportional to the number of staked coins one has. The more coins one has staked, the more probable it is to be selected as validator. In case a validator validates a block which has inaccurate information in it, there will be a penalty and the validator will lose part of their staked coins. In such a system, the mining power is proportional to the number of staked coins one has. Unlike PoW, which uses a difficult computational puzzle, requiring tremendous amounts of computing power and electricity with a huge carbon footprint, PoS is simpler, faster and more eco-friendly.
Consensus mechanisms, such as PoW and PoS, usually deal with the trilemma of decentralization, scalability and security. Both PoW and pure PoS have a decentralized nature where all participants have the right to participate in validation. An alternative approach is the so-called “Delegated PoS”, which is a more centralized system where only a limited number of people with known identities have the power to validate transactions and generate blocks.
4.1.6 Merkle Tree
A Merkle tree is an inverse tree structure where every leaf node has a label which is the cryptographic hash of a transaction (or any other data) and every non-leaf node has a label which is the hash of the labels of its child nodes. A simple Merkle tree is depicted in Figure 7.
This structure allows for an efficient and secure validation of records of large data structures. The validation that a leaf node is part of the tree requires the computation of a number of hashes which is proportional to the logarithm of the number of the leaf nodes in the tree. Merkle trees are used in Bitcoin and other crypto networks. An average Bitcoin block contains over 1,000 transactions, so the Merkle tree is in fact much larger than the one presented in Figure 7. A full (“thick”) node of the network has full, complete blocks. On the other hand, a “thin” node has only headers but still needs to be able to verify transactions. In thin nodes, the Merkle tree is used to verify a specific transaction without the need to download the whole blockchain. For example, in Figure 7, to verify that Transaction B is included in the Block, we have to query the network about HA and HCD, only. Given HB and HA, we calculate HAB. Then, given HAB and HCD, we calculate HABCD and we compare it with the Merkle root of the tree, which is part of the header. The transaction is proved to be valid, unaltered and part of the block if the final hash is equal to the Merkle root of the tree, on top of it. In a Merkle tree with 1,000 transactions, one would need to have only about 10 hash outputs to validate a given transaction.
4.2 General Purpose Technologies
General Purpose Technologies (GPTs) can affect an entire economy. They can impact economic growth and transform both our everyday lives and the ways we do business. Such technologies have the inherent potential for technical improvements, and innovational complementarities, giving rise to increasing returns-to-scale (Bresnahan and Trajtenberg 1995). Thus, they can drastically alter societies and foster generalized productivity gains through their impact on pre-existing economic and social structures. Examples of GPTs include electricity, the electric motor, the steam engine, the computer and the internet. These technologies fundamentally impacted how we live, expanded our lives (physically and emotionally), helped build our cities and changed how people interact with the world.
4.3 Blockchain as GPT
Given its unique characteristics, such as immutability, transparency, and distribution (Sandner and Schulden 2019), blockchain is recognized by many as a new form of GPT. Naturally, it takes time for a GPT to diffuse through the economy. Although blockchain is still at the infrastructure building stage, it is expected to unleash several applications across different verticals within the next 5–15 years. Like the internet in its first years, blockchain is difficult to predict or even understand well, but in the future, it could become ubiquitous in the exchange of physical and digital goods, record keeping, information, and online platforms. According to an article by Harvard Business Review, “Blockchain is the first native digital medium for value, just as the internet was the first native digital medium for information.”
4.4 The Properties of Distributed Ledger Technology
A distributed ledger has some specific properties. In particular, it is:
• Programmable. For example, it can be programmed to run specific smart contracts
• Secure. All the records are encrypted
• Immutable. The validated records cannot be deleted or changed as they are irreversible.
• Anonymous. The identity of participants can be either fully anonymous or pseudonymous.
• Unanimous. The participants agree to the validity of the records.
• Distributed. Copies of the ledger are distributed to all participants, for complete transparency.
• Time-stamped. Every block and every transaction have a timestamp.
4.5 Decentralized and Centralized Ledgers
In a fully decentralized blockchain, e.g. Bitcoin, anyone can participate and transact on the ledger. There are no “privileged” users, and a distributed consensus protocol is used. As a result of this system design, there should be mechanisms in place to combat the vulnerabilities arising from it. These mechanisms prevent people from corrupting the system and ensure that transactions are correct. Bitcoin uses Proof-of-Work (PoW) and “mining” for this.
In a centralized blockchain, not anyone can transact on the ledger. There are a few trusted centralized authorities that have the right to validate transactions and modify the ledger. In this case the blockchain can still be distributed, meaning that many parties can again hold copies of the ledger. Yet, the validity of the system comes from the fact that only some credible and reputable participants can modify the ledger. And because participants’ identities are known, their transactions can therefore be audited.
Centralization can undermine the technology’s purpose as a shared ledger. Too much power can be placed in the control of a single entity or a few special “players”. The past has shown that when a single entity takes too much power then it may no longer operate for the benefit of the society. Fully decentralized distributed systems can mitigate risk and prevent attacks while centralized systems are more prone to them. A centralized ledger would essentially act like a third-party and thus the concept of peer-to-peer, fully distributed network without intermediaries would no longer be valid.
5 Blockchain in the Civil, Architectural and Construction Industry
In this section we identify several key areas of the civil, architectural and construction industry where blockchain can be applied, and we investigate the current state of the art, the benefits that blockchain technology can offer and the challenges and opportunities ahead. The summarized results are presented in Table 3, while the following sub-sections provide further details and a relevant discussion for each application area.
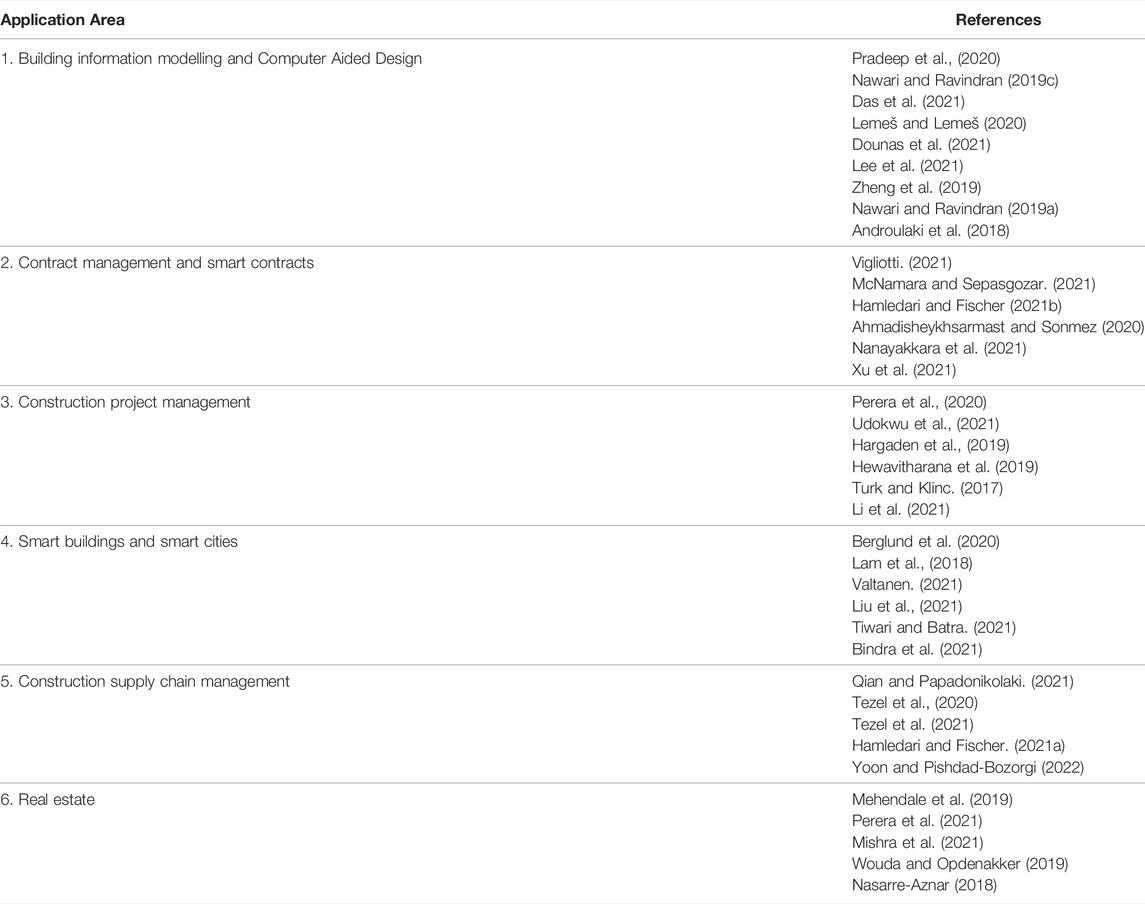
TABLE 3. Key areas of the civil, architectural and construction industry where blockchain can be applied.
5.1 Building Information Modelling and Computer Aided Design
Blockchain can be used to provide live and trustworthy information for BIM, by information sharing among present and future information owners. Furthermore, it can help enhance the benefits of BIM by allowing architects and engineers to design on the same BIM model with clear ownership, while design and construction decisions can be recorded on the blockchain for future analysis and liability.
Information exchange in BIM is critical yet complex due to the multi-party collaboration nature of a construction project. Bimchain, funded in 2017, is a blockchain technology software aiming at accelerating the BIM revolution in the Building Industry by integrating the BIM software and processes to create a binding traceability of data exchanges. As paper-based solutions are often insufficient, (Pradeep et al., 2020), investigated the use of blockchain technology and in particular the commercial software Bimchain for improving Trust in BIM Data Exchange. Their work showed that Bimchain manages to accomplish most of its objectives, such as improving data reliability, limiting the scope of liability, and clarifying stakeholder responsibilities, among others. However, the legal validity of the tool’s proofs is still untested and therefore it is still not able to establish a global acceptance for real-world applications.
(Nawari and Ravindran 2019c) proposed the use of blockchain in a BIM workflow environment. They presented an overview of the blockchain and discussed its integration with the Building information process, focusing on how blockchain can help in improving the BIM working environment by providing reliable data storage and management of permissions, reinforcing network security, and ensuring data ownership and change tracing (Das et al., 2021). presented a comprehensive study on the requirements of BIM security, claiming that although the technologies to support BIM security are available in research and on the market, they are not customized in existing collaborative BIM platforms to support security. They proposed a conceptual encryption strategy for securing BIM data distribution and a distributed blockchain-based framework for BIM change recording.
(Lemeš and Lemeš 2020) presented a work on using blockchain technology in Distributed and collaborative CAD (Computer Aided Design) environments, such as BIM and Geographical Information Systems (GIS). They argue that blockchain can provide answers to key issues such as data integrity and confidence in information stored in information systems (Dounas et al., 2021). introduced a framework for decentralized architectural design BIM and blockchain integration in the context of the 4th industrial revolution. The authors examined the constraints of BIM regarding collaboration and trust. Then they introduced a blockchain solution for creating new operational and business models for architectural design, through scaling collaboration, project governance, and shifting trust to the infrastructure. They focused on the design process and validated the framework with a prototype of BIM design optimization integrated with blockchain.
(Lee et al., 2021) proposed an integrated digital twin and blockchain solution to support accountable information sharing in construction projects. In this implementation, the digital twin updates the BIM in nearly real-time using sensors and internet of things, while the blockchain has the role of authentication and adding confidence to the transaction data. The framework was tested with a case study where virtual positioning data from a prefabricated brick was transmitted to a digital twin in real-time and recorded on the blockchain using time stamps (Zheng et al., 2019). presented a novel BIM system called bcBIM to facilitate BIM data audit for historical modifications by blockchain in mobile cloud with big data sharing. The authors proposed a method of BIM data organization based on private or public blockchains. Using blockchain, the system can trace, authenticate and prevent tampering of historical data related to BIM (Nawari N. and Ravindran S. 2019). proposed a new framework with the integration of BIM and blockchain to improve the efficiency of building permit processes in post-disaster events, with the application of smart contracts and Hyperledger Fabric (HLF) (Androulaki et al., 2018).
5.2 Contract Management and Smart Contracts
A Smart Contract is a computer program that works based on an “if/then” principle. Smart contracts can identify accountabilities and trigger payments based on milestones (Vigliotti 2021). They are executed automatically reducing the necessity of intermediaries and as a result time and money can be saved. They can be used to automate agreements, thus revolutionizing construction contracts and payments which usually rely on traditional methods.
(McNamara and Sepasgozar 2021) discussed and investigated the use of blockchain and intelligent contracts (iContracts) for the digitalization of the construction industry. The authors identified 9 influencing factors based on 46 studies and presented a conceptual three-dimensional model for iContract system adoption. The study aims to identify key considerations for such contracts, develop a theoretical adoption model and offer an agenda of 6 research directions for the future (Hamledari and Fischer 2021b). investigated the role of smart contracts in the automation of construction progress payments. Current computerized payment applications cannot support reliable automation of progress payments due to the fact that they rely on centralized control mechanisms and no guaranteed execution. The authors argue that decentralized smart contracts based on blockchain can address these limitations in an effective way. They explore the conceptual underpinning for the design of an automated payment system and investigate the role of smart contracts in enabling reliable and autonomous conditioning of cash flow on product flow status. They also use a test case for payments based on progress and smart contracts in the context of unmanned aerial vehicle-based progress monitoring.
(Ahmadisheykhsarmast and Sonmez 2020) proposed the use of smart contracts for securing the payments in construction contracts. This can guarantee payments while eliminating administrative costs and burdens related to trusted intermediaries, by employing an automated protocol running on a decentralized blockchain. On the other hand (Nanayakkara et al., 2021), investigated the suitability of blockchain and smart contracts for dealing with payment issues in the construction industry. They concluded that solutions based on blockchain and smart contracts can mitigate the payment and the related financial issues in the construction industry, including non-payments, partial payments, long payment cycle, cost of finance, retention, security of payments, among others.
In the structural engineering field, very powerful capabilities are available today for the simulation and analysis of structures, given the development of computational methods, numerical analysis software and hardware during the last decades (Plevris and Tsiatas 2018). In this area (Xu et al., 2021), presented a work on the application of a blockchain network and smart contracts in structural health monitoring (SHM). Their results showed that such a system can provide several advantages, such as monitoring authority verification, generation of abnormal alerts, data immutability, resistance to attacks, and traceability query.
5.3 Construction Project Management
Construction project management (CPM) can potentially benefit from an agile and more decentralized approach based on blockchain, with high transparency, and the parties being compensated for outcomes and for work performed. Given that the construction industry has been historically reported as one of the slowest sectors in the adaptation of information technology, the question of whether blockchain as a technology is hype or real in the construction industry was addressed by (Perera et al., 2020). Their work aimed at analyzing the potential of blockchain applications in construction through case analyses and a comprehensive literature review. According to the study, blockchain has indeed a credible potential in the construction industry, due to its exponential general use, the investments involved, and a number of start-up businesses contributing to Industry 4.0.
In the work of (Udokwu et al., 2021), a blockchain-based CPM platform implementing smart contract technologies was presented for facilitating the peer-to-peer collaboration between parties in the construction industry, leading to improved information flow, cost and time reduction, and improvement in the quality of the services. The system relies on diligent up-front requirement studies with a coherent system architecture and the use of cooperation protocols (Hargaden et al., 2019). examined the role of blockchain technologies in CPM, providing insights into the performance of blockchain in construction and investigating the feasibility of its potential adoption with case studies. The authors claim that blockchain can increase the efficiency of processes within the construction industry and eliminate current issues related to trust, verification and transparency (Hewavitharana et al., 2019). examined how blockchain can address the project management perspectives in the construction industry regarding the guidelines mentioned in the Project Management Body of Knowledge (PMBOK). Five criteria were selected for the analysis using the relevant guidelines, namely 1) contract management, 2) purchase management, 3) finance management, 4) asset and inventory management, and 5) subcontractor management. It was identified that blockchain can indeed assist in all these areas.
(Turk and Klinc 2017) presented an investigation on the potential of blockchain for construction management. The authors highlighted that blockchain can improve the trustworthiness and reliability of logbooks in construction, while it can also help secure storing of sensitive data. They concluded that blockchain can offer solutions to various problems in construction information management while decentralizing the construction processes (Li et al., 2021). proposed the use of a 2-layer adaptive supervision model based on blockchain for off-site modular housing production, where the 1st layer includes the adaptive private sidechains of participants and the 2nd is the main blockchain for trading and communication among all participants. The blockchain-based methodology has the benefit of avoiding tampering of the operation records, while driving the participants to promptly publish their operation records, without any privacy risks.
5.4 Smart Buildings and Smart Cities
As urbanization is increasing rapidly, offering improved livability and a higher standard of living, the concept of “smart cities” are one of the main focus areas of many governments across the globe. Many countries attempt to establish special strategies for transforming their cities into smart cities, utilizing the potential opportunities and limiting any relevant threats arising from urbanization. Smart cities enable operational efficiency, maximize environmental sustainability efforts and create new citizen services. Blockchain innovation can be utilized to make smarter cities. Blockchain-based solutions can be utilized to enhance our cities and provide for better economic development and livability, by offering enhanced security, immutability, resilience and transparency.
(Berglund et al., 2020) investigated the role of civil engineering in smart cities and smart infrastructures. They examined a number of smart technologies that can be used for infrastructure management, such as crowdsourcing and citizen science, sensors, data transmission, actuators, big data analytics, data visualization, Internet of Things, and blockchain. They identified the gaps in the application of such technologies for infrastructure systems and they highlighted how civil engineering can adopt new roles toward the development of applications related to smart cities (Lam et al., 2018). investigated the use of a blockchain system in smart cities, claiming that malpractices related to civil engineering can be avoided if there are transparent, timely, and unalterable records of the relevant activities, based on a blockchain (Valtanen 2021). identified several design challenges regarding the development of blockchain-enabled capabilities of a smart home. They analyzed and classified these challenges and did an organized literature review to identify the best practices and find possible solutions (Liu et al., 2021). explored the impact of integrating BIM and blockchain into a smart city environment, on making more sustainable buildings. They investigated the relationships between BIM, blockchain, and sustainable building throughout the life cycle of a construction project.
(Tiwari and Batra 2021) examined the application of blockchain-based solutions for the reparations in smart buildings, proposing a prototype simulating the system architecture and discussing how blockchain can further expedite security, automation, and transparency in smart buildings. The work focused on the use of smart contracts in smart buildings, for repairs and service. On the other hand (Bindra et al., 2021), investigated the use of blockchain technology and smart contracts for the flexible, decentralized access control of smart buildings. According to the study, visitor and occupant access to equipment and spaces within the buildings continue to be managed in a conservative, old-fashioned, and inflexible way, through inefficient, unsystematic, and human-intensive processes. Their work describes a methodology relying on blockchain and smart contracts that can securely and flexibly manage building access privileges for both short-term visitors and long-term occupants, taking into consideration the risk associated with accessing a space in the building, in an efficient, decentralized way.
5.5 Construction Supply Chain Management
The construction industry is characterized by fragmentation in processes, operations and services. One of its major problems is the disconnect between construction and design, due to the lack of trustworthy and open information across the supply chain. Blockchain has the potential to adverse these issues using open and transparent transactions. It can be used to trace physical objects from the origin to the destination. It can also improve payment settlements, compliance management and material planning, while smart contracts can be implemented to automatically purchase, track, and verify items in the supply chain, in real-time.
According to the study of (Qian and Papadonikolaki 2021), supply chain management (SCM) has long been committed to the reduction of cost and increase of efficiency, while trying to reduce fragmentation and optimize resources. Since trust has always been a significant factor in managing SCM relationships, the study aimed at examining how trust can be affected by introducing blockchain technology in the construction SCM. Based on semi-structured interviews and information from experts, the study suggests that blockchain can help enhance trust in SCM and provide supply chain partners with proper protection mechanisms to avoid the risks and costs associated with opportunistic behaviour in collaboration. This can shift trust from relational to system-based and cognition-based. However, the authors highlight that the extent to which blockchain can develop and spread will ultimately depend on the readiness of the social capital to accept decentralised governance schemes.
(Tezel et al., 2020) examined the potential and future directions of blockchain applied to construction supply chains. For this, the authors collected empirical data through semi-structured interviews with seventeen experts in the field. They used SWOT analysis to present the strengths, weaknesses, opportunities and threats involved and they also exhibited the requirements for and steps toward a construction supply structure facilitated by blockchain technology. The same group (Tezel et al., 2021) later investigated the implementation of blockchain in construction, presenting discussions on SCM applications of blockchain for construction by collecting feedback for 3 models based on blockchain: reverse auction-based tendering for bidding, project bank accounts for payment purposes, and asset tokenization for the financing of projects. A set of general and model-specific challenges and opportunities were identified for the implementation of blockchain in construction.
(Hamledari and Fischer 2021a) presented the implementation of crypto assets based on blockchain for the integration of the physical and financial supply chains in the construction industry. The paper demonstrated how blockchain-based crypto assets used for payments made conditionally on the flow of products can address the limitations of physical and financial supply chains due to high fragmentation and relying on financial institutions. The study also highlights the problem of price volatility and examines potential solutions (Yoon and Pishdad-Bozorgi 2022). aimed to explore the applications of blockchain in addressing issues related to CSC. They identified the main problems in CSC as related to collaboration, information sharing and sustainability. Although these issues have been dealt with individually in the past, they are essentially coupled and interconnected. Blockchain technology can provide a holistic system view approach to address all of them together, i.e., enhance sustainability, promote collaboration, and facilitate information sharing, all at once.
5.6 Real Estate: Property Ownership, Land Titles, Asset Management and Maintenance
Real estate is known as one of the most important sectors of the economy, playing a crucial role in the lives of people across the world. The size of the global real estate assets managed professionally was estimated at $8.5 trillion in 2017. Real estate investments provide better returns than the stock market without as much volatility, providing also tax benefits in many cases. Although real estate is so important and despite the technological advancements that have affected other sectors, it has not changed much during the last decades. It is still a “pen and pencil” business, relying on archaic methods for keeping records and doing transactions. The industry suffers from various problems, such as limited participation due to barriers to enter, slow and costly verification procedures involving a lot of intermediaries and very limited foreign investments, to name only a few.
Figure 8 presents the real estate ecosystem today and depicts the number of different parties involved and the relevant interactions. This traditional model has several drawbacks and limitations:
• There are a lot of intermediaries, that increase the cost and reduce the transactions’ speed.
• The current approach requires significant time and effort for due diligence and financial verification.
• Foreign investments are difficult, expensive, and slow. International bank accounts, accreditation, financing, credit score, cash requirements, access to sponsors, fund managers, even citizenship, might be needed for investing in real estate in a foreign country. As a result, the real estate business remains very “local”, in geographical terms.
• Real estate transactions are done via wire transfers and require costly and slow verification processes with increased likelihood or error.
• Real estate investment can be very expensive and as a result there is limited participation. Although everybody is interested in housing, real estate is the investment choice of the rich and not open to all. People with small amounts of money are not allowed to invest in expensive real estate assets as the current system does not support fractional ownership. One needs to either buy a whole building/apartment or nothing.
• Low liquidity. Real estate assets are traditionally difficult to trade or convert to cash because of their high value and the cost of the intermediaries, each time a transaction is made.
Real estate is entering the blockchain era and it can benefit from the numerous advantages that the technology can offer. Traditionally, real estate transaction records are housed in central servers controlled by a single administration point. With Blockchain, all real estate ownership and transaction records can be stored securely as tamper-proof digital records on the blockchain, in a decentralized way. Such records are fully accurate, safe, and immutable. Blockchain immutability proves ownership and facilitates transactions. In addition, with the use of blockchain, verification becomes an easy task which does not require lengthy procedures, high cost and intermediaries (Mehendale et al., 2019). discussed how blockchain can revolutionize the real estate industry, by reducing the paperwork and the time needed for property assessment, document collection, the preparation of contracts, and others (Perera et al., 2021). highlighted the potential of blockchain in real estate, with respect to property transactions, where businesses depend on the reliability of transaction records and blockchain can be user to enhance trust and ensure ownership. Their paper demonstrates a methodology for developing a blockchain system starting from problem analysis, selection of blockchain platform, system modelling, prototype development, and evaluation. Their findings provide the foundation for developing proofs of concept for other potential applications of blockchain in the built environment.
Information and data related to the building or structure need to be tracked at every stage of its life cycle. Blockchain can provide a living ledger that records everything happening with the asset. Blockchain can allow tracking and access to all the necessary information and data through the life cycle of the asset. In case of any refurbishments or other improvements to a building, these changes can be documented and recorded, and the whole repository can be transferred to new owners when the property is sold. In the future, each property will come with a universally shared data set, which will include background information such as past sales, repairs and amenities. This digital history of transactions will help every stakeholder prove their ownership, increase transparency and eliminate fraud attempts. This alone will have tremendous consequences in countries where one cannot rely on public authorities because of corruption. The transactions can be made easy, safe, and inexpensive due to the use of blockchain technology which offers quick and costless verification (Mishra et al., 2021). discussed the digitalization of land records using blockchain technology. According to the authors, the immutable, auditable and traceable features of blockchain entice governments around the world to implement decentralized technology within the process of land registration.
In addition, real estate assets can be fully tokenized in the blockchain system, allowing participation to people having limited amounts of savings. Real estate tokenization is the process of creating a digital asset that represents a property on the blockchain. The tokenization process addresses various challenges in capital formation and liquidity, although it requires a legal wrapper around the property, to securitize and create an investment vehicle. The use of intermediaries can be minimized, while the system can facilitate foreign real estate investments and liquidity as anyone will be able to buy or sell even tiny shares of real estate assets (Wouda and Opdenakker 2019). investigate the application of a blockchain solution for improving the transaction process of an office building in the Netherlands. The authors highlight the problems of the current system, such as lack of market transparency, slow speed and inefficiency (Nasarre-Aznar 2018). investigate the relationship between collaborative housing and blockchain. The authors conclude that the technology has the potential to facilitate access to housing through the reduction of cost and time and minimizing the role of intermediaries.
6 Conclusion and Discussion
We are moving to a digital economy where financial and physical assets will increasingly have digital representations. According to the World Economic Forum, by 2025, blockchains will store around 10% of the world’s GPD. Countries are trying to make the necessary legislative and regulatory changes to adapt to the new environment and make this change a reality. New opportunities arise. Looking towards the future, it appears that blockchain is something that we will be hearing a lot more of. Although nobody can predict the degree to which it will affect the economy, our lives and every single sector particularly in the long run, most experts agree that it has the potential to play a significant role in the future, in a wide range of fields across different verticals. This is evidenced by the fast-growing occurrence of blockchain-related articles in the scientific literature during the very recent years, in several scientific areas, including engineering.
The present study briefly examined the technical details, main concepts and aspects of blockchain technology and aimed at formulating a picture of the current state and practice of its use in fields related to civil, architectural and construction engineering. The study also summarized and highlighted specific application areas related to the Architecture, Engineering, and Construction (AEC) industry where blockchain has the potential to provide new solutions, and how they can be adopted to improve performance, sustainability, and safety in the future. We identified six important application areas and examined the relevant challenges and opportunities ahead, namely in: 1) Building information modelling (BIM) and Computer Aided Design (CAD); 2) Contract management and smart contracts; 3) Construction project management; 4) Smart buildings and smart cities; 5) Construction supply chain management; and 6) Property ownership, land titles, asset management and maintenance in real estate. The conclusion of the study is that although blockchain technology is new and there are certainly several early challenges to tackle, it has great potential to become an extremely positive force of change in the construction industry. As engineers, it is our inherent responsibility to facilitate the digital transformation of the AEC industry and to make it ready for the challenges and opportunities of the future, and blockchain is bound to play a pivotal role in this transformation.
Author Contributions
All authors listed have made a substantial, direct, and intellectual contribution to the work and approved it for publication.
Conflict of Interest
The authors declare that the research was conducted in the absence of any commercial or financial relationships that could be construed as a potential conflict of interest.
Publisher’s Note
All claims expressed in this article are solely those of the authors and do not necessarily represent those of their affiliated organizations, or those of the publisher, the editors and the reviewers. Any product that may be evaluated in this article, or claim that may be made by its manufacturer, is not guaranteed or endorsed by the publisher.
References
Ahmadisheykhsarmast, S., and Sonmez, R. (2020). A Smart Contract System for Security of Payment of Construction Contracts. Automation in Construction 120, 103401. doi:10.1016/j.autcon.2020.103401
Androulaki, E., Barger, A., Bortnikov, V., Muralidharan, S., Cachin, C., Christidis, K., et al. (2018). “Hyperledger Fabric: A Distributed Operating System for Permissioned Blockchains,” in Proceedings of the 13th EuroSys Conference (Porto, Portugal: ACM). doi:10.1145/3190508.3190538
Bach, L. M., Mihaljevic, B., and Zagar, M. (20182). “Comparative Analysis of Blockchain Consensus Algorithms,” in 41st International Convention on Information and Communication Technology, Electronics and Microelectronics (MIPRO)21-25 May 2018, 1545–1550. doi:10.23919/MIPRO.2018.8400278
Berglund, E. Z., Monroe, J. G., Ahmed, I., Noghabaei, M., Do, J., Pesantez, J. E., et al. (2020). Smart Infrastructure: A Vision for the Role of the Civil Engineering Profession in Smart Cities. J. Infrastruct. Syst. 26 (2), 03120001. doi:10.1061/(asce)is.1943-555x.0000549
Bindra, L., Eng, K., Ardakanian, O., and Stroulia, E. (2021). Flexible, Decentralised Access Control for Smart Buildings with Smart Contracts. Cyber-Physical Syst., 1–35. doi:10.1080/23335777.2021.1922502
Bresnahan, T. F., and Trajtenberg, M. (1995). General Purpose Technologies 'Engines of Growth'? J. Econom. 65 (1), 83–108. doi:10.1016/0304-4076(94)01598-T
Cheng, M., Liu, G., Xu, Y., and Chi, M. (2021). When Blockchain Meets the AEC Industry: Present Status, Benefits, Challenges, and Future Research Opportunities. Buildings 11 (8), 340. doi:10.3390/buildings11080340
Das, M., Tao, X., and Cheng, J. C. P. (2021). BIM Security: A Critical Review and Recommendations Using Encryption Strategy and Blockchain. Automation in Construction 126, 103682. doi:10.1016/j.autcon.2021.103682
Dounas, T., Lombardi, D., and Jabi, W. (2021). Framework for Decentralised Architectural Design BIM and Blockchain Integration. Int. J. Architectural Comput. 19 (2), 157–173. doi:10.1177/1478077120963376
Estébanez, C., Saez, Y., Recio, G., and Isasi, P. (2014). Performance of the Most Common Non-cryptographic Hash Functions. Softw. Pract. Exper. 44 (6), 681–698. doi:10.1002/spe.2179
Federal Deposit Insurance Corporation. (2020). "How America Banks: Household Use of Banking and Financial Services, 2019 FDIC Survey."
Hamledari, H., and Fischer, M. (2021b). Role of Blockchain-Enabled Smart Contracts in Automating Construction Progress Payments. J. Leg. Aff. Dispute Resolut. Eng. Constr. 13 (1), 04520038. doi:10.1061/(ASCE)LA.1943-4170.0000442
Hamledari, H., and Fischer, M. (2021a). The Application of Blockchain-Based Crypto Assets for Integrating the Physical and Financial Supply Chains in the Construction & Engineering Industry. Automation in Construction 127, 103711. doi:10.1016/j.autcon.2021.103711
Hargaden, V., Papakostas, N., Newell, A., Khavia, A., and Scanlon, A. (2019). “The Role of Blockchain Technologies in Construction Engineering Project Management,” in IEEE International Conference on Engineering, Technology and Innovation (ICE/ITMC), Valbonne Sophia-Antipolis, France, June 17-19, 2019 (IEEE), 1–6. doi:10.1109/ICE.2019.8792582
Hewavitharana, T., Nanayakkara, S., Nanayakkara, S., and Perera, S. (2019). Blockchain as a Project Management Platform. World Construction Symp., 137–146. doi:10.31705/WCS.2019.14
Kroll, J. H. (2012). “The Monetary Background of Early Coinage,” in The Oxford Handbook of Greek and Roman Coinage. Editor W. E. Metcalf (Oxford University Press). doi:10.1093/oxfordhb/9780195305746.013.0003
Lam, R. C. Y., Junus, A., Mak, J. Y. W., Lam, L. C. H., and Lee, P. K. K. (2018). “Blockchain for Civil Engineering Practices in Smart Cities,” in 2018 IEEE International Conference on Internet of Things (iThings) and IEEE Green Computing and Communications (GreenCom) and IEEE Cyber, Physical and Social Computing (CPSCom) and IEEE Smart Data (SmartData), 1294–1300. doi:10.1109/Cybermatics_2018.2018.00225
Lee, D., Lee, S. H., Masoud, N., Krishnan, M. S., and Li, V. C. (2021). Integrated Digital Twin and Blockchain Framework to Support Accountable Information Sharing in Construction Projects. Automation in Construction 127, 103688. doi:10.1016/j.autcon.2021.103688
Lemeš, S., and Lemeš, L. (2020). Blockchain in Distributed CAD Environments. Lecture Notes Networks Syst. 76, 25–32. doi:10.1007/978-3-030-18072-0_3
Li, J., Greenwood, D., and Kassem, M. (2019). Blockchain in the Built Environment and Construction Industry: A Systematic Review, Conceptual Models and Practical Use Cases. Automation in Construction 102, 288–307. doi:10.1016/j.autcon.2019.02.005
Li, X., Wu, L., Zhao, R., Lu, W., and Xue, F. (2021). Two-layer Adaptive Blockchain-Based Supervision Model for Off-Site Modular Housing Production. Comput. Industry 128, 103437. doi:10.1016/j.compind.2021.103437
Liu, Z., Chi, Z., Osmani, M., and Demian, P. (2021). Blockchain and Building Information Management (BIM) for Sustainable Building Development within the Context of Smart Cities. Sustainability 13 (4), 2090. doi:10.3390/su13042090
McNamara, A. J., and Sepasgozar, S. M. E. (2021). Intelligent Contract Adoption in the Construction Industry: Concept Development. Automation in Construction 122, 103452. doi:10.1016/j.autcon.2020.103452
Mehendale, D. K., Masurekar, R. S., and Patil, H. V. (2019). Implications of Block Chain in Real Estate Industry. Int. J. Recent Techn. Eng. 8 (1), 500–503. https://www.ijrte.org/wp-content/uploads/papers/v8i1/A5388058119.pdf.
Mishra, I., SupriyaSahoo, A., Sahoo, A., and Vivek Anand, M. (2021). “Digitalization of Land Records Using Blockchain Technology,” in International Conference on Advance Computing and Innovative Technologies in Engineering, Greater Noida, India, March 4-5, 2021 (IEEE), 2021, 769–772. doi:10.1109/ICACITE51222.2021.9404678
Nanayakkara, S., Perera, S., Senaratne, S., Weerasuriya, G. T., and Bandara, H. M. N. D. (2021). Blockchain and Smart Contracts: A Solution for Payment Issues in Construction Supply Chains. Informatics 8 (2), 36. doi:10.3390/informatics8020036
Nasarre-Aznar, S. (2018). Collaborative Housing and Blockchain. Administration 66 (2), 59–82. doi:10.2478/admin-2018-0018
Nawari, N. O., and Ravindran, S. (2019b). Blockchain and the Built Environment: Potentials and Limitations. J. Building Eng. 25, 100832. doi:10.1016/j.jobe.2019.100832
Nawari, N. O., and Ravindran, S. (2019c). Blockchain Technologies in BIM Workflow Environment. Comput. Civil Eng. 2019, 343–352. doi:10.1061/9780784482421.044
Nawari, N., and Ravindran, S. (2019a). Blockchain and Building Information Modeling (BIM): Review and Applications in Post-Disaster Recovery. Buildings 9 (6), 149. doi:10.3390/buildings9060149
Penard, W., and van Werkhoven, T. (2008). “On the Secure Hash Algorithm Family,” in Cryptography in Context. Editor G. Tel (Utrecht, 1–18.
Perera, S., Hijazi, A. A., Weerasuriya, G. T., Nanayakkara, S., and Rodrigo, M. N. N. (2021). Blockchain-Based Trusted Property Transactions in the Built Environment: Development of an Incubation-Ready Prototype. Buildings 11 (11), 560. doi:10.3390/buildings11110560
Perera, S., Nanayakkara, S., Rodrigo, M. N. N., Senaratne, S., and Weinand, R. (2020). Blockchain Technology: Is it Hype or Real in the Construction Industry? J. Ind. Inf. Integration 17, 100125. doi:10.1016/j.jii.2020.100125
Plevris, V., and Tsiatas, G. C. (2018). Computational Structural Engineering: Past Achievements and Future Challenges. Front. Built Environ. 4 (21), 1–5. doi:10.3389/fbuil.2018.00021
Pradeep, A. S. E., Amor, R., and Yiu, T. W. (2020). "Blockchain Improving Trust in BIM Data Exchange: A Case Study on BIMCHAIN. Construction Res. Congress 2020, 1174–1183. doi:10.1061/9780784482865.124
Qian, X., and Papadonikolaki, E. (2020). Shifting Trust in Construction Supply Chains through Blockchain Technology. Ecam 28 (2), 584–602. doi:10.1108/ECAM-12-2019-0676
Sandner, P., and Schulden, P. M. (2019). Speciality Grand Challenges: Blockchain. Front. Blockchain 2 (1). doi:10.3389/fbloc.2019.00001
Shojaei, A. (2019). “Exploring Applications of Blockchain Technology in the Construction Industry,” in ISEC 2019 - 10th International Structural Engineering and Construction Conference. doi:10.14455/isec.res.2019.786
Shojaei, A., Ketabi, R., Razkenari, M., Hakim, H., and Wang, J. (2021). Enabling a Circular Economy in the Built Environment Sector through Blockchain Technology. J. Clean. Prod. 294, 126352. doi:10.1016/j.jclepro.2021.126352
Tezel, A., Febrero, P., Papadonikolaki, E., and Yitmen, I. (2021). Insights into Blockchain Implementation in Construction: Models for Supply Chain Management. J. Manag. Eng. 37 (4), 04021038. doi:10.1061/(ASCE)ME.1943-5479.0000939
Tezel, A., Papadonikolaki, E., Yitmen, I., and Bolpagni, M. (2022). “Blockchain Opportunities and Issues in the Built Environment: Perspectives on Trust, Transparency and Cybersecurity,” in Industry 4.0 for the Built Environment: Methodologies, Technologies and Skills. Editors M. Bolpagni, R. Gavina, and D. Ribeiro (Cham: Springer International Publishing), 569–588. doi:10.1007/978-3-030-82430-3_24
Tezel, A., Papadonikolaki, E., Yitmen, I., and Hilletofth, P. (2020). Preparing Construction Supply Chains for Blockchain Technology: An Investigation of its Potential and Future Directions. Front. Eng. Manag. 7 (4), 547–563. doi:10.1007/s42524-020-0110-8
Tiwari, A., and Batra, U. (2021). Blockchain Enabled Reparations in Smart Buildings Cyber Physical System. Def. Sc. J. 71 (4), 491–498. doi:10.14429/DSJ.71.16454
Turk, Ž., and Klinc, R. (2017). Potentials of Blockchain Technology for Construction Management. Proced. Eng. 196, 638–645. doi:10.1016/j.proeng.2017.08.052
Udokwu, C., Norta, A., and Wenna, C. (2021). “Designing a Collaborative Construction-Project Platform on Blockchain Technology for Transparency, Traceability, and Information Symmetry,” in ASSE '21: 2021 2nd Asia Service Sciences and Software Engineering Conference, Macau, February 24 - 26, 2021 (ACM), 1–9. doi:10.1145/3456126.3456134
Valtanen, K. (2021). “Design Challenges of Developing a Blockchain-Enabled Smart home,” in 2021 Conference on Information Communications Technology and Society, Durban, South Africa, March 10-11, 2021 (IEEE), 34–39. doi:10.1109/ICTAS50802.2021.9395016
van Eck, N. J., and Waltman, L. (2007). VOS: A New Method for Visualizing Similarities between Objects. Berlin, Heidelberg, 299–306. doi:10.1007/978-3-540-70981-7_34
Vigliotti, M. G. (2021). What Do We Mean by Smart Contracts? Open Challenges in Smart Contracts. Front. Blockchain 3 (45), 55371. doi:10.3389/fbloc.2020.553671
Wang, M., Wang, C. C., Sepasgozar, S., and Zlatanova, S. (2020). A Systematic Review of Digital Technology Adoption in Off-Site Construction: Current Status and Future Direction towards Industry 4.0. Buildings 10 (11), 204. doi:10.3390/buildings10110204
Wouda, H. P., and Opdenakker, R. (2019). Blockchain Technology in Commercial Real Estate Transactions. Jpif 37 (6), 570–579. doi:10.1108/JPIF-06-2019-0085
Xu, J., Liu, H., and Han, Q. (2021). Blockchain Technology and Smart Contract for Civil Structural Health Monitoring System. Computer‐Aided Civil Infrastructure Eng. 36 (10), 1288–1305. doi:10.1111/mice.12666
Yoon, J. H., and Pishdad-Bozorgi, P. (2022). State-of-the-Art Review of Blockchain-Enabled Construction Supply Chain. J. Construction Eng. Manag. 148 (2), 03121008. doi:10.1061/(asce)co.1943-7862.0002235
Keywords: blockchain, general purpose technology (GPT), distributed ledger, civil engineering, architecture, construction, engineering
Citation: Plevris V, Lagaros ND and Zeytinci A (2022) Blockchain in Civil Engineering, Architecture and Construction Industry: State of the Art, Evolution, Challenges and Opportunities. Front. Built Environ. 8:840303. doi: 10.3389/fbuil.2022.840303
Received: 21 December 2021; Accepted: 10 March 2022;
Published: 29 March 2022.
Edited by:
Georgios Eleftherios Stavroulakis, Technical University of Crete, GreeceReviewed by:
Ibrahim Yitmen, Jönköping University, SwedenSameh Samir F Mehanny, Cairo University, Egypt
Copyright © 2022 Plevris, Lagaros and Zeytinci. This is an open-access article distributed under the terms of the Creative Commons Attribution License (CC BY). The use, distribution or reproduction in other forums is permitted, provided the original author(s) and the copyright owner(s) are credited and that the original publication in this journal is cited, in accordance with accepted academic practice. No use, distribution or reproduction is permitted which does not comply with these terms.
*Correspondence: Vagelis Plevris, dnBsZXZyaXNAcXUuZWR1LnFh