- 1Faculty of Architecture Building and Planning, University of Melbourne, Melbourne, VIC, Australia
- 2School of Biosciences, Faculty of Science, University of Melbourne, Melbourne, VIC, Australia
- 3Research Manager, Arup University, Docklands, VIC, Australia
Architects, artists and engineers around the world have been experimenting with the potential of mycelium, the vegetative body of a fungus, as a future building material for the past 15 years. It shares many of the positive material attributes of polystyrene but unlike the synthetic material it is fully sustainable and completely biodegradable. Mycelium has also proved to be simple to grow at scale. Its capacity to rapidly grow its tangled hyphae in a multiplicity of directions, digesting nothing more than organic waste, has shown promise for the production of a variety of materials for the building industry. But despite this, mycelium has struggled to find a market within the building industry. Drawing on the literature, this article argues that the challenges have been psychological, aesthetic and economic, rather than technical. Western industrial systems have conditioned us to expect material cultures to be clean, precise and durable. Mycelium is messy and some fungi are known pathogens. Like any living creature it can be unpredictable. Further, while the materials for growing mycelium are cheap, initial production costs for mass production and distribution typical of industrial fabrication are high. The risk for investors in the absence of an assured market stymied early forays into production. But as the environmental crisis becomes more urgent, there is evidence of a growing interest in finding new avenues for production. Centralised large-scale production is only one way forward. Another, which learns from early failures, is mass production through a multiplicity of micro-scale, do-it-yourself systems.
Introduction
The Intergovernmental Panel on Climate Change warns that there is unequivocal evidence of escalating global climate change and advises that developing better building systems is critical for a more resilient future (IPCC 1988; IPCC 2022; Sachs et al., 2021, World Resources Institute 2022). Intensifying concerns about environmental performance have led the building industry to broaden its approach beyond decreasing energy use through solar orientation, insulation, and low energy electrical fittings, to systemic concerns that take into account the materials from which buildings are constructed (Stephan, Crawford and De Myttenaere 2011; Mirabella et al., 2018; Moncaster et al., 2018). Research into novel bio-materials has grown in response (Jones and Brischke 2017; Pacheco-Torgal et al., 2020). While some research has translated into products for manufacture, there have been many failures along the way.
This article considers the successes and challenges for mycelium, a bio-polymer that has received significant attention globally. Although the innovations in the field have developed through trans-disciplinary collaborations, most of the publications are narrowly focused along disciplinary silos—architectural design, biology, mechanical engineering, or agricultural engineering. Other literature reviews focus more comprehensively but narrowly on experimental scientific research and patents rather than those that have emerged in creative research or product development. This article, on the other hand, brings together varied interests. It draws selectively on scholarly literature from a range of pure and applied scientific disciplines—microbiology, engineering, bio-technology and agriculture—as well as grey literature, design industry websites, and the websites of material developers and producers.
Polymers in the Built Environment: Synthetic and Natural
Polymers are used in all facets of the building industry: paints, fillers, adhesives, flooring, cabinetry, electrical wiring, insulation and roofing (Harrison & Hester 2018). They are lightweight, versatile, impermeable and durable. While polymers are one of the most multiplicitous organic material types on earth (Seymour 1988; Rasmussen 2018), most of the polymers now in use in the built environment are synthetic. First developed in the early 19th century, they have multiplied and proliferated exponentially since the 1930s (Rasmussen 2018). Petroleum-based synthetic polymers present two significant problems: Firstly they perpetuate fossil fuel extraction, and secondly their durability renders them an enduring blight in landscapes long after their useful life in buildings is over. In an era facing twin challenges of global warming and plastic pollution, lessening the dependency on synthetics in the building industry is both a necessity and a challenge.
One of the most ancient and prolific natural polymers is found in fungi. Carbon-based polymers in the form of chitin and glucans constitute the cell walls of fungi, while their mycelium are akin to fibres. Fungi generally grow in two vegetative forms; single-celled yeast and multicellular hyphae. The budding yeast Saccharomyces cerevisiae is one of the most familiar domesticated species, used for bread, beer and wine making. But it is the multi-cellular filamentous fungi, which grow as long, tube-like cells joined end-to-end and form highly branched networks, that shares many characteristics with synthetic polymers and have recently been identified as a possible substitute in the building industry. Like synthetic polymers, mycelium can be lightweight, strong, and a good insulator. It is also similarly versatile. Through careful selection of appropriate species, adjusting the feeding stock and molds, mycelium can be made into a material as thin and flexible as leather, aggregated into blockwork, providing a substitute for thermal and acoustic insulation and even simulate mass concrete Figure 1.
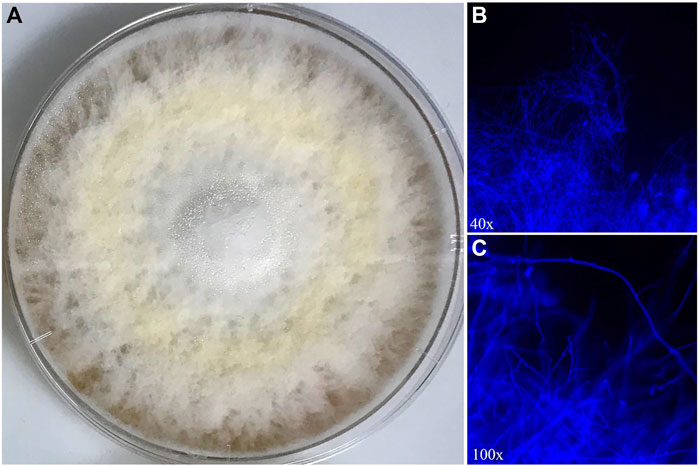
FIGURE 1. Pleurotus ostreatus, commonly known as the oyster mushroom. P. ostreatus mycelial growth on a malt extract agar plate after 7 days incubation at 22°C (A). The plate was inoculated in the centre and the fungus has grown to the edges and filled the entire plate. Microscopic images of mycelium from the plate on the left after staining cell walls with calcofluor white (fluorescent brightener 28). Images taken under fluorescent microscopy at 40 × (B) and 100 × (C) magnification. Image supplied by Alex Andrianopoulos.
Fungi are also key players in most ecosystems. They are major recyclers of organic material, transforming often inaccessible matter into available essential nutrients, as well as playing more direct and critical roles in the growth of numerous plant species. Across the fungal kingdom, mycelium displays many unique biological properties including a generic capacity to grow without light, to specialised activities such as the production of oxalic acids that have the strength to decompose rock, and peroxidase enzymes that break hydrocarbon bonds in complex carbohydrates such as lignin and turn them into simple sugars. These properties mean they can grow rapidly on carbon-based products we typically discard as waste materials such as husks, sawdust, woodchip, and even coffee grounds. So, unlike artificial polymers which perpetuate the use of fossil fuels and, when discarded, endure for centuries as waste, fungi can grow on agricultural waste and are completely biodegradable. Despite these advantages, until a decade ago, fungi were a largely untapped resource as a building material.
Different Approaches to Experimentation With Mycelium
Experimental research in mycelium for the built environment has branched and proliferated, much like mycelia itself. After a decade of largely invisible activity deep in the homes and minds of early developers in the 1990s and early 2000s, a first flush of experimental prototypes emerged between 2007 and 2014 in New York and California in the United States, and a few years later in Europe (focused in the Netherlands). Since then, a global network of researchers across creative and scientific disciplines, with and without manufacturers, has flowered. Most are working collaboratively in interdisciplinary teams: With artists, architects, interior designers, industrial designers and furniture makers engaging in creative research to explore the poetic and aesthetic possibilities of the material; scientific researchers, including engineers, microbiologists, bio-technologists and agricultural scientists testing mechanical, thermal and acoustic performance, morphology, optimal species and feeding regimes; and industry partners focussing on trials around repeatability, reproducibility and scalability. Outcomes have been disseminated through academic journals and conferences and exhibited in galleries and public and professional orientated design websites. Around 50 patents have been filed for numerous processes using fungal mycelium as a replacement for synthetic polymers since 2006 (Cerimi et al., 2019, Hüttner et al., 2020). The US-based companies Ecovative and MycoWorks were early leaders. Ecovative now dominates the US patents landscape (Cerimi et al., 2019). However, China dominates globally. Some products that hit the market early have disappeared. Others have found a niche and continued to grow. The field has developed as much by these failures as it has by the successes. Success in material performance is not the only requirement for viability for production.
Mycelium-based experimentation and prototyping has pursued four lines: Flat hardboard products for building panels; flexible leather substitutes; disposable packaging; architectural fittings and homewares. Architects, engineers, and building contractors, are increasingly aligning their strategies to sustainable development goals and net-zero targets which is translating into awareness of how design choices impact the carbon footprint of the built environment, and ultimately supply chain choices. The biggest advantage of mycelium is its biodegradability; where supply materials are sourced from waste material and renewable energy is used for production, bio-based materials promise considerable environmental performance in comparison to traditional counterparts. While the technology shows maturity in both low-risk disposable products and in industries with high turnovers, mycelium has struggled to find a niche within the construction industry. Markets need customers. Customers’ desires and interests are driven to varying degrees by aesthetics, cost, and ethical concerns (Gagnier 2000). These in turn are shaped by bigger, often global, social, environmental and economic forces (Kompatsiaris and Chrysagis, 2020). As the climate crisis accelerates, economies are shifting (Miles 2014). So too is the willingness to embrace different aesthetics and pay higher premiums to satisfy ethical concerns. Consequently, there is an increasing appetite and interest in continuing the experimentation.
Panel Products
Mycelium’s similarity to polystyrene and particle board led two groups to test and prototype biodegradable material substitutes for building products currently made from synthetic material from 2006 to 2009. In New York, engineering students Eben Bayer and Gavin McIntyre at Rensselaer Polytechnic developed a mycelium-based insulation material they thought could replace synthetic counterparts (Rensselaer 2007). They filed for a patent on the method they developed to grow the material in 2007 (US Patent no. 9485917) and set up a small company, Ecovative around the same time (Ecovative 2021a). Ecovative applied this technique to make an insulation board trademarked as ‘Greensulate’ the same year (Material District, 2022). Californian, artist and herbalist, Phil Ross, meanwhile, had spent the 1990s and early 2000s experimenting with cultivating mushrooms and testing similar material ideas. His small catenary shaped pavilion, assembled from blocks cast from the mycelium of Ganoderma lucidum (Reishi mushrooms), Alpha Mycotectural teahouse, was installed in an art gallery in Dusseldörf Germany in 2009 (Debatty et al., 2011). Ross filed for his first patent describing a method for producing fungus structures for construction and manufacturing in 2011 (US Patent no. 9410116). The patent describes methods for increasing the strength of the material through compression during the growth phase and methods for integrating structural support members. After teaming up with collaborator Sophie Wang to form the start-up MycoWorks, Ross and Wang spent 3 years applying these techniques into prototypes for the building industry. The suite of materials included panels and other flat moulded forms for interior linings, cabinets, furniture and structural systems. Both companies engaged in further exhibitions of their work: MycoWorks’ collection was exhibited in the Design and Violence interactive at MoMA (Superflux 2014) and Daring Growth display at the 2016 Venice Biennale (MycoWorks 2021a); while Ecovative supplied mycelium blocks for a tower installation designed by architect David Benjamin from The Living, and Arups Engineering, at MoMA PSI Hy-Fi (Stott 2014).
Both MycoWorks and Ecovative developed long-term experimental research collaborations with engineers to test the mechanical, thermal and biological properties of mycelium. Ross worked with Travaglini et al (2013, 2014, 2015, 2016) while McIntyre collaborated with teams led by agricultural engineers Matthew Pelletier et al. (2013) and Alexander Zeigler et al. (2016), mechanical engineers Lai Jiang and Daniel Walczyk (Jiang et al., 2014a, 2014b, 2015, 2016, 2017a, 2017b, 2018) and Mohammed Islam et al. (2017). They found mycelium boards performed well enough on stability, strength and thermal insulation to compete with other comparable products on the market, which seemed to indicate that the products would be viable for use in the building industry. But from a business perspective, bio-based building materials could not compete with traditional materials based on cost-effectiveness Figure 2.
In theory mycelium panel products should be inexpensive to produce once systems are established. Growing media, like sawdust from softwood mills, organic waste from agribusiness or textiles from consumer goods, could be sourced from waste diverted from existing industries. Spores, meanwhile, are tiny and light to distribute. They could be engineered quickly at a relatively low cost en masse at research centres and distributed to cultivation and drying facilities. But there were a number of systemic challenges that needed to be addressed first to reduce risk for investors. Scaling up needed a degree of surety around demand. Production needed consistent and reliable supply chains for growing media, which in turn needed systems change within agriculture and industry. Demonstrating compliance to national building codes was costly. Fire testing, for example, costs tens of thousands of dollars. Large scale dedicated controlled spaces for growing were expensive but necessary and so to was large scale equipment for heat-treating. Consequently none of these panel products were developed into a viable product for the market.
Flexible Fabrics
Mycelium material manufacturers instead, responded to the failures in one market by tweaking their products for another. MycoWorks responded to their economic challenges by switching focus to a product line with a high turnover, but one for which consumers are willing to pay significant sums of money: Fashion. Partnering with Hermès, MycoWorks developed flexible mycelium fabrics with similar qualities to leather that are being used in their handbags (Hahn 2021). “Sylvania,” also known as “Reishi Fine Mycelium,” performs equally well or better than leather on measures of tensile strength, abrasion resistance and colourfastness (MycoWorks 2021b). Aligning with a luxury fashion house willing to pay for hand crafted materials allowed them to set a price that would turn a profit. US Biotech company Bolt Threads, launched an equivalent product, Mylo in 2017 (Hahn 2020). It is now being used by global sportswear manufacturers, Adidas and Lululemon, and the fashion house Stella McCartney and Kering, for “vegan” footwear and clothing lines (Hahn 2020). Ecovative has a similar product, marketed by the sheet, ready for “tanning” as well as a flexible, insulating foam for garments (Ecovative 2021b).
In developing a product that addressed economic imperatives, product developers discovered a new challenge. A number of familiar fungi are pathogens of animals, plants and other fungi which engenders a perception of threat in customers. Would mycelium fabrics bring molds and dry rots into wardrobes? Would people inhale dangerous spores? Some strains of fungi are known to cause significant yield losses in many crops such as wheat and rice and others of fruit. MycoWorks responded to such perceived fears by rehabilitating the reputation of fungi through sensorial design measures and customer education. These have included imprinting the material with a texture that mirrors leather, suffusing it with the fragrance of tanned leather, and visual marketing that creates an association of indulgence (MycoWorks 2021c). The process of both growing, but more importantly, killing mycelium through a drying process has also been communicated to customers.
Packaging
Ecovative, by contrast, responded to the failures in producing their panel insulation product for the market by exploiting mycelium’s other great advantage—its capacity to grow to fit any container in which it is cultivated. They applied their know-how to disposable packaging. Packaging has an even higher turnover than the fashion industry. Indeed perishability is desirable. It also has a higher tolerance for irregularity and lower performance requirements than the building industry. Using a process that involves making a 3D virtual model of the form to be cradled, and producing custom designed thermoform growth trays, Ecovative has been able to grow packaging to suit any product. Their “mycelium foundry” in Green Island New York—a term that connotes fine craftsmanship and industry in one –is now capable of producing packaging at volume. Ecovative now has a contract to supply the multinational furniture giant, IKEA, with a bio-substitute for polystyrene packaging (Steffen 2019). IKEA, in turn, is leveraging the biodegradable packaging to appeal to a customer base increasingly concerned with the impacts of plastic waste on oceans, waterways and the ecosystems they support (Gosden 2016).
Other industries that tolerate, indeed embrace, materials that are part of a sustainable, circular economy include landscape design and gardening products. Grown.bio has licenced Ecovative’s technology to market a line of planter boxes and other small pots (Grown.bio. 2022). An Australian collaboration between Swinburne University, Arup, the Royal Botanic Gardens and Studio Edward produced a floating sacrificial planter, “Mushi,” that exploits mycelium’s capacity to float and then biodegrade after extended contact with water (Melbourne Design Week 2021). The waffle form is planted with wetland grasses that promote biodiversity. It builds on earlier work by one of the most influential mycologists, Paul Stamets, who explored interventions that exploit mycelium’s dynamic capacities while it is alive to remediate contaminated soils and waterways (Miller 2013).
Architectural Fittings and Homewares
Mycelium’s capacity to be cast into complex forms inspired much of the early creative experimentation by designers in Europe and subsequent application in furniture and other interior products and homewares. Maurizio Montalti of Officina Corpuscoli, in collaboration with Utrecht University, curated a series of exhibitions, including “The Future of Plastic” (2009), which focused on bowls and other vessels cast from mycelium, “Mycelium Design” (2014) and “Fungal Futures” (2016), which showcased a number of other European designers exploring the many shapes and textures that mycelium can morph into. Designers included Aniela Hoitink, who was an early developer of fine flexible mycelium for fashion; Jonas Edvard Neilson who was prototyping furniture; Kristel Peters, who exhibited concepts for footwear; and Phil Ross who displayed his interlocking blockwork. Many have continued to apply their knowledge in industrial design. Like fashion, these are building items that can attract a higher price than hidden lining boards and insulation. Edvard Neilson’s MYX Lamps from 2014 (Chin 2014) and Danielle Trofe’s Mushlume (Ecovative supply the mycelium) from 2011 are lighting products of note (Danielle Trofe Design 2020).
Montalti has also launched a company, Mogu, based in Italy. Mogu and Arup are working on a suite of mycelium-based products. One of them is “Foresta,” an acoustic panel. This product has now been tested and certified for use and is now entering the European market (Arup 2021). It combines good technical properties (acoustic properties comparable to traditional panels; fire-resistance compliance; dimensional stability; safety; ease of assembly, disassembly and prospects for reconfiguring) with environmental performance and circular principles (the panel can be bio-degraded, unlike traditional composite panels that often end up in landfill). Like Ecovative’s earlier Greensulate, Foresta is a sustainable alternative to similar synthetic products. But rather than a hidden filler, like the early thermal insulation, its selling point is that it is an office feature panel. Mogu and Arup are also developing further products for premium interior fit-outs. Compliances in the building industry are often less strict for temporary or non-structural elements, such as interior fitouts, so this market currently has the best applicability potential for bio-based material.
Interface Between Science and Manufacturing: Prospects for the Future
A range of biological scientists have been working closely with these product designers to develop the best strains, growing media, and processes of cultivation to shape the performance of the product. Agricultural engineer, Alexander Zeigler (Ziegler et al., 2016) considered specific gravity, surface hardness, water absorption, coefficient of linear thermal expansion, and resistance to tension with different fungal strains and feed—hemp and cotton waste. Microbiologist Freek Appels, working with architect Maurizio Montalti and others from Utrecht and Maastricht Universities, has explored the relationship between moisture, feed, and heat on mechanical performance. They found that glycerol can change mycelium’s density, hydrophilicity and mechanical properties from paper-like, to leather-like, to rubber-like, (Appels et al., 2019, 2020). Like plants, fungi have a wall that surrounds cells. The cell wall is composed of various carbohydrate types such as glucans and chitin and decorated by proteins such as mannans. The composition of the cell wall, that is the relative proportion of each of these components, varies between fungi and composition can also change depending on the growth conditions. Similarly, the architecture of cell walls—how each of these core components is layered relative to the underlying cell membrane and the way the components are chemically linked—also varies between fungi and growth conditions. Moreover, cell walls are continually remodelled by fungi to deal with changing environments and to allow for growth.
Mechanical engineers, on the other hand, have focused on aspects of structural performance. Since the pioneering work led by Travaligni for MycoWorks and Jiang and Islam (2017) for Ecovative, other collaborations have tested different mechanical limits. The recent wave of experimentation has tested more ambitious structural systems. Advancing on the earlier block systems which were modelled on masonry units, a research team led by Heisel has tested mycelium’s capacity to replace cast concrete. The team, assembled from academics based at KIT Karlsruhe Germany, ETH Zürich, ETH Singapore and the Mycotech Lab Indonesia, has engineered a load-bearing branching structural system, dubbed the Mycotree (Heisel et al., 2017). Recognising mycelium’s relative strength in compression but relative weakness in tension, the design uses funicular geometry much like those used in medieval stone vaults. The team has also developed neat timber brackets and connectors, supplemented with small sections of steel. Architecture students from Georgia Tech (Dessi-Olive 2019) have also tested casting monolithic mycelium in situ. Outcomes included arches and vaults, culminating with a 2.5 × 2.5 × 2.5 m cubic pavilion with a pointed Gothic style vaulted interior, grown from an inoculated base material supplied by Ecovative, within a removable plywood formwork. Finally, Eugene Soh’s team, based in Singapore, is developing a technique for extruding mycelium paste that has applications for digital printing systems (Soh et al., 2020). This would enable an additive approach to making mycelium elements, eliminating the need for a mold, which the casting method requires.
There is also experimental work underway that capitalises on other material attributes shared by mycelium with synthetic polymers. Some polymers are particularly good at flowing into tiny voids in substrates while in their liquid form, interlocking with other particles to create a bond. They are the basis of many adhesives. A collaboration between plant biotechnology and environmental science research units in China, Malaysia and Denmark, led by Nyuk Ling Ma and Christian Sonne, has tested mycelium as a possible “green” adhesive substitute for the toxic urea-formaldehyde commonly used in particle board (Khoo et al., 2020). Rather than focusing on feeding regimes for living mycelium, as other researchers and manufacturers have done, this team has explored the potential of dead mushrooms, a growing waste product from the food industry. Experiments show that heat compressing spent mushroom waste, at 160°C causes it to melt. Placing it under pressure (10 mPa for 20 min) with woody waste, like sawdust, forces it to penetrate and cohere to the particulate matter producing a particle board with higher internal bonding strength than building standards require, and high water and fire resistance too.
Finally, partnerships between computer scientists and mycologists are exploring the prospect of working collaboratively with mycelium whilst it is still alive. Unconventional computer scientists and mycologists are focussing on the patterns of electrical signals that mycelium produces (Adamatzky and Gandia 2021). Fungal Architectures consortium (Fungal Architectures 2020) is exploring how to enrich the functionality of mycelium to make it responsive to external stimuli (Beasley et al., 2020a; Adamatzky et al., 2020). These studies suggest that embedding nanoparticles and polymers into the fungal substrate would enable mycelium objects to become intelligent sensors that could respond to external environments (Olsson and Hansson, 1995; Adamatzky & Gandia 2021). This is opening further research into self-growing and self-repairing materials for the built environment. Metabolically inactive spores of certain fungi that biomineralize calcium carbonate when exposed to water and oxygen have been shown to be a successful concrete additive that can delay concrete degradation. Alkaliphilic fungi will remain viable for long periods of time despite the environmental conditions in concrete. When the concrete begins to crack, allowing air and water to penetrate, the spores germinate and the fungus precipitates calcium carbonate, repairing cracks (Van Wylick et al., 2021).
Conclusion
This article has offered a focused review of literature related to creative and scientific collaborations that have developed mycelium-based materials as an alternative to synthetic polymers. It shows that considerable advances have been made over the past 15 years. Early research revealed that the material held much promise. But creating a viable product for the market has met unexpected challenges. Interestingly creative responses to failures in one market have led to viable products for another. For mycelium to be used more broadly in the building industry, however, there remain three key challenges: Balancing biodegradability with an acceptable level of durability; demonstrating compliance with building standards through testing; and developing systems of production that are cost-effective. As research has both diversified and specialised, a range of composite materials that are fit-for-purpose are addressing the first challenge. The cost of testing for compliance with building codes remains high but the investment by some manufacturers, like Mogu, has paid off. Approvals have followed and markets are growing for their products. This is lowering the risk for others to do the same. Centralised large-scale production has been shown to work for packaging and textiles, but not yet for large-scaled building materials. Policy settings that support the status quo remain an impediment to investment. With an ever-broadening range of novel systems under development, these costs will continue to reduce. Micro-scale, do-it-yourself systems are another alternative. There are numerous products on the market and instructionals on the internet for mycelium-based products for gardening and food growing at home. These include Stamets’ “Life Box,” a corrugated cardboard impregnated with fungal spores and seeds, as well as mushroom growing kits. There are also some resources available for DIY builders, but these are still in their infancy. MycoWorks provides some resources (MycoWorks 2021d), as does Critical Concrete, a social initiative to educate on sustainable building generally and mycelium production for the building industry specifically (Criti.co 2022). Finally, novel research that is exploring the capabilities of fungus across its whole life cycle—as spores, while growing, and after it is dead—is revealing that there are many underexplored avenues for working with fungus in the built environment. As policies continue to evolve in light of the urgency of the climate crisis, zero-waste products such as these are likely to become increasingly viable.
Author Contributions
JM, AA, and AL contributed to the conception and intellectual framing of the review. JM prepared the literature review and the first draft of the manuscript. AA and AL wrote sections of the manuscript. All authors contributed to the manuscript revision. All authors read and approved the submitted version.
Conflict of Interest
AL is Research Manager, Arup University. Global parent company, Arup Group, was engaged as engineering consultants for the HyFi Tower, MoMA PS1, developed with architecture practice, The Living, and the Foresta acoustic panels, developed in collaboration with Mogu. Both are mentioned in the article.
The remaining authors declare that the research was conducted in the absence of any commercial or financial relationships that could be construed as a potential conflict of interest.
Publisher’s Note
All claims expressed in this article are solely those of the authors and do not necessarily represent those of their affiliated organizations, or those of the publisher, the editors and the reviewers. Any product that may be evaluated in this article, or claim that may be made by its manufacturer, is not guaranteed or endorsed by the publisher.
Acknowledgments
The authors would like to thank the University of Melbourne’s Bio-Inspiration Hallmark Research Initiative for seed funding support: https://research.unimelb.edu.au/research-at-melbourne/multidisciplinary-research/hallmark-research-initiatives/bioinspiration.
References
Adamatzky, A., and Gandia, A. (2021). On Electrical Spiking of Ganoderma Resinaceum. Biophys. Rev. Lett. 16, 133–141. doi:10.1142/S1793048021500089
Adamatzky, A., Tegelaar, M., Wosten, H. A. B., Powell, A. L., Beasley, A. E., and Mayne, R. (2020). On Boolean Gates in Fungal Colony. Biosystems 193-194, 104138. doi:10.1016/j.biosystems.2020.104138
Appels, F. V. W., van den Brandhof, J. G., Dijksterhuis, J., de Kort, G. W., and Wösten, H. A. B. (2020). Fungal Mycelium Classified in Different Material Families Based on Glycerol Treatment. Commun. Biol. 3 (1), 334–335. doi:10.1038/s42003-020-1064-4
Appels, F. V. W., Camere, S., Montalti, M., Karana, E., Jansen, K. M. B., Dijksterhuis, J., et al. (2019). Fabrication Factors Influencing Mechanical, Moisture- and Water-Related Properties of Mycelium-Based Composites. Mater. Des. 161, 64–71. doi:10.1016/j.matdes.2018.11.027
Arup (2021). Acoustic Panel System Made from Fungi Offers Innovative Circular Design Solution for Workplaces. Available at: https://www.arup.com/projects/foresta-acoustic-panel-system (Accessed October 26, 2021).
Attias, N., Danai, O., Abitbol, T., Tarazi, E., Ezov, N., Pereman, I., et al. (2020). Mycelium Bio-Composites in Industrial Design and Architecture: Comparative Review and Experimental Analysis. J. Clean. Prod. 246, 119037. doi:10.1016/j.jclepro.2019.119037
Beasley, A. E., Powell, A. L., and Adamatzky, A. (2020a). Fungal Photosensors. arXiv 2003.07825. doi:10.48550/arXiv.2003.07825
Cerimi, K., Akkaya, K. C., Pohl, C., Schmidt, B., and Neubauer, P. (2019). Fungi as Source for New Bio-Based Materials: a Patent Review. Fungal Biol. Biotechnol. 6 (1), 1–10. doi:10.1186/s40694-019-0080-y
Chin, A. (2014). Jonas Edvard Nielsen Grows MYX Lamps from Mushroom-Mycelium, Designboom. Available at: https://www.designboom.com/design/jonas-edvard-myx-lamps-mushroom-mycelium-09-02-2014 (Accessed October 26, 2021).
Criti, co. (2022). Organic Materials in Architecture: Inside Mycelium, Porto: Critical Concrete. Available at: https://criti.co/course/view.php?id=61 (Accessed April 12, 2022).
Danielle Trofe Design (2020). A New Paradigm of Sustainability. Available at: https://danielletrofe.com (Accessed October 26, 2021).
Debatty, R., Evans, C., Garcia, P., and Grover, A. (2011). New Art/Science Affinities. Carnegie Mellon University, 84–87.
Ecovative (2021a). Ecovative. Available at: https://ecovative.com/our-story/>(Accessed October 16, 2021).
Ecovative (2021b). Ecovative. Available at: https://ecovative.com/foams/>(Accessed October 16, 2021).
Feldman, D. (2008). Polymer History. Des. monomers Polym. 11 (1), 1–15. doi:10.1163/156855508x292383
Fungal Architectures (2020). Fungal Architectures. Available at: https://www.fungar.eu (Accessed March 13, 2022).
Gagnier, R. (2000). The Insatiability of Human Wants: Economics and Aesthetics in Market Society. University of Chicago Press.
Girometta, C., Picco, A. M., Baiguera, R. M., Dondi, D., Babbini, S., Cartabia, M., et al. (2019). Physico-mechanical and Thermodynamic Properties of Mycelium-Based Biocomposites: a Review. Sustainability 11 (1), 281. doi:10.3390/su11010281
Gosden, E. (2016). Ikea Plans Mushroom-Based Packaging as Eco-Friendly Replacement for Polystyrene, the Sydney Morning Herald, 25 February. Available at: https://www.smh.com.au/business/companies/ikea-plans-mushroombased-packaging-as-ecofriendly-replacement-for-polystyrene-20160225-gn331e.html (Accessed October 16, 2017).
Grown.bio (2022). Grown.bio. Available at: https://www.grown.bio (AccessedApril 12, 2022).
Hahn, J. (2021). Hermès Creates Mycelium Version of its Classic Leather Victoria Handbag. Dezeen. Available at: https://www.dezeen.com/2021/03/18/hermes-mycelium-leather-victoria-bag-mycoworks (Accessed October 16, 2021).
Hahn, J. (2020). Major Fashion Houses Will Sell Products Made from Mushroom Leather by Next Year. Dezeen. Available at: https://www.dezeen.com/2020/10/08/mylo-consortium-adidas-stella-mccartney-lululemon-kering-mycelium (Accessed October 16, 2021).
Harrison, R. M., and Hester, R. E. (Editors) (2018). Plastics and the Environment (Cambridge: RSC Publishing).
Heisel, F., Schlesier, K., Lee, J., Rippmann, M., Saeidi, N., Javadian, A., et al. ((2017). “Design of a Load-Bearing Mycelium Structure through Informed Structural Engineering,” in World Congress on Sustainable Technologies–49. WCST-2017)ss. 45 Available at: https://www.block.arch.ethz.ch/brg/files/HEISEL_2017_WCST_design-loadbearing-mycelium-structure_1546891598.pdf (Accessed March 13, 2022).
Hüttner, S., Johansson, A., Teixeira, P. G., Achterberg, P., and Nair, R. B. (2020). Recent Advances in the Intellectual Property Landscape of Filamentous Fungi. Fungal Biol. Biotechnol. 7 (16). doi:10.1186/s40694-020-00106-z
Islam, M. R., Tudryn, G., Bucinell, R., Schadler, L., and Picu, R. C. (2017). Morphology and Mechanics of Fungal Mycelium. Sci. Rep. 7, 13070. doi:10.1038/s41598-017-13295-2
Jiang, L. (2015). A New Manufacturing Process for Biocomposite Sandwich Parts Using a Myceliated Core, Natural Reinforcement and Infused Bioresin. USA: Rensselaer Polytechnic Institute. doi:10.13140/RG.2.1.4166.5528
Jiang, L., Bucinell, R., Li, B., Walczyk, D., and McIntyre, G. (2018). Bioresin Infused Then Cured Mycelium-Based Sandwich-Structure Biocomposites: Resin Transfer Molding (RTM) Process, Flexural Properties, and Simulation. J. Clean. Prod. 207, 123e135. doi:10.1016/j.jclepro.2018.09.255
Jiang, L., Walczyk, D. F., and McIntyre, G. (2014b). Vacuum Infusion of Mycelium-Bound Biocomposite Preforms with Natural Resins. Adv. Compos. Mat. Expo. Comb. Strength Unsurpassed Innov. Available at: https://www.researchgate.net/profile/Lai-Jiang-2/publication/274139042_Vaccum_Infusion_of_Mycelium-bound_Biocomposite_Preforms_with_Natural_Resins/links/55176c1e0cf2d70ee277bd5c/Vaccum-Infusion-of-Mycelium-bound-Biocomposite-Preforms-with-Natural-Resins.pdf (Accessed March 13, 2022).
Jiang, L., Walczyk, D., and McIntyre, G. (2017a). A New Approach to Manufacturing Biocomposite Sandwich Structures: Investigation of Preform Shell Behavior. J. Manuf. Sci. Eng. Trans. ASME 139, 021014. doi:10.1115/1.4034278
Jiang, L., Walczyk, D., and Mcintyre, G. (2014a). “A New Process for Manufacturing Biocomposite Laminate and Sandwich Parts Using Mycelium as a Binder,” in American Society for Composites 29th Technical Conference. Available at: https://www.researchgate.net/profile/Lai-Jiang-2/publication/274138941_A_New_Process_for_Manufacturing_Biocomposite_Laminate_and_Sandwich_Parts_using_Mycelium_as_a_Binder/links/55176cb90cf2f7d80a3a7c32/A-New-Process-for-Manufacturing-Biocomposite-Laminate-and-Sandwich-Parts-using-Mycelium-as-a-Binder.pdf (Accessed March 13, 2022).
Jiang, L., Walczyk, D., McIntyre, G., and Bucinell, R. (2016). “A New Approach to Manufacturing Biocomposite Sandwich Structures: Mycelium-Based Cores,” in ASME 2016 11th International Manufacturing Science and Engineering Conference (American Society of Mechanical Engineers). MSEC 2016. doi:10.1115/msec2016-8864
Jiang, L., Walczyk, D., McIntyre, G., Bucinell, R., and Tudryn, G. (2017b). Manufacturing of Biocomposite Sandwich Structures Using Mycelium-Bound Cores and Preforms. J. Manuf. Process. 28, 50–59. doi:10.1016/j.jmapro.2017.04.029
Jones, D., and Brischke, C. (2017). Performance of Bio-Based Building Materials. Sawston: Woodhead Publishing.
Khoo, S. C., Peng, W. X., Yang, Y., Ge, S. B., Soon, C. F., Ma, N. L., et al. (2020). Development of Formaldehyde-Free Bio-Board Produced From Mushroom Mycelium and Substrate Waste. J. Hazard. Mater. 400, 123296. doi:10.1016/j.jhazmat.2020.123296
Kompatsiaris, P., and Chrysagis, E. (2020). Crafting Values: Economies, Ethics and Aesthetics of Artistic Valuation. J. Cult. Econ. 13 (6), 663–671. doi:10.1080/17530350.2020.1798803
Material District (2022). Greensulate. Available at: https://materialdistrict.com/material/greensulate (Accessed March 13, 2022).
Melbourne Design Week (2021). Waterfront: ‘Mushi’ – Concept to Prototype. Available at: https://2021.designweek.melbourne/events/mycelium-wetland-an-organic-solution (Accessed October 18, 2021).
Miles, M. (2014). Eco-aesthetics: Art, Literature and Architecture in a Period of Climate Change. A&C Black, London: Bloomsbury Publishing.
Miller, K. (2013). How Mushrooms Can Save the World, Discover, Milwaukee: Kalmbach Media Co. Available at: https://www.discovermagazine.com/environment/how-mushrooms-can-save-the-world (Accessed October 27, 2021).
Mirabella, N., Röck, M., Ruschi Mendes Saade, M., Spirinckx, C., Bosmans, M., Allacker, K., et al. (2018). Strategies to Improve the Energy Performance of Buildings: A Review of Their Life Cycle Impact. Buildings 8 (8), 105. doi:10.3390/buildings8080105
Moncaster, A. M., Pomponi, F., Symons, K. E., and Guthrie, P. M. (2018). Why Method Matters: Temporal, Spatial and Physical Variations in LCA and Their Impact on Choice of Structural System. Energy Build. 173, 389–398. doi:10.1016/j.enbuild.2018.05.039
MycoWorks (2021c). An Advanced Materials Platform. Available at: https://www.mycoworks.com/our-products (Accessed February 24, 2022).
MycoWorks (2021a). Our Heritage: 2014-2016. Available at: https://www.mycoworks.com/ourOur Heritage: 2014-2016: Acceleration-heritage#acceleration (Accessed October 18, 2021).
MycoWorks, (2021b). Reishi Is Fine Mycelium. Available at: https://www.madewithreishi.com/products (Accessed October 18, 2021).
MycoWorks (2021d). Resources. Available at: https://www.mycoworks.com/resources (Accessed October 18, 2021).
Olsson, S., and Hansson, B. S. (1995). Action Potential-like Activity Found in Fungal Mycelia Is Sensitive to Stimulation. Naturwissenschaften 82, 30–31. doi:10.1007/bf01167867
F. Pacheco-Torgal, V. Ivanov, and D. C. Tsang (Editors) (2020). Bio-based Materials and Biotechnologies for Eco-Efficient Construction (Sawston: Woodhead Publishing).
Pelletier, M. G., Holt, G. A., Wanjura, J. D., Bayer, E., and McIntyre, G. (2013). An Evaluation Study of Mycelium Based Acoustic Absorbers Grown on Agricultural By-Product Substrates. Industrial Crops Prod. 51, 480–485. doi:10.1016/j.indcrop.2013.09.008
Rasmussen, S. C. (2018). Revisiting the Early History of Synthetic Polymers: Critiques and New Insights. Ambix 65 (4), 356–372. doi:10.1080/00026980.2018.1512775
Rensselaer (2007). Designs on the Future, Rensselaer Winter 2007-08. Available at: https://www.rpi.edu/magazine/winter2007-08/pdi-4.html (Accessed October 16, 2017).
Ross, P. (2017). “Your Rotten Future Will Be Great,” in Routledge Companion to Biology in Art and Architecture. Editors C. N. Terranova, and M. Tromble (Abingdon: Routledge), 370–403.
Sachs, J., Kroll, C., Lafortune, G., Fuller, G., and Woelm, F. (2021). Sustainable Development Report: A Decade of Action of the Sustainable Development Goals. Cambridge: Cambridge University Press. Available at: https://s3.amazonaws.com/sustainabledevelopment.report/2021/2021-sustainable-development-report.pdf (Accessed March 12, 2022).
Soh, E., Chew, Z. Y., Saeidi, N., Javadian, A., Hebel, D., and Le Ferrand, H. (2020). Development of an Extrudable Paste to Build Mycelium-Bound Composites. Mater. Des. 195, 109058. doi:10.1016/j.matdes.2020.109058
Steffen, A. (2019). IKEA Starts Using Compostable Mushroom-Based Packaging for its Products. Intelligent Living. Available at: https://www.intelligentliving.co/ikea-mushroom-based-packaging (Accessed October 16, 2021).
Stephan, A., Crawford, R. H., and De Myttenaere, K. (2011). Towards a More Holistic Approach to Reducing the Energy Demand of Dwellings. Procedia Eng. 21, 1033–1041. doi:10.1016/j.proeng.2011.11.2109
Stott, R. (2014). Hy-fio, the Organic Mushroom Brick Tower Opens at MoMA’s PS1courtyard. ArchDaily. Available at: https://www.archdaily.com/521266/hy-fi-the-organic-mushroom-brick-tower-opens-at-moma-s-ps1-courtyard (Accessed October 16, 2017).
Superflux, 2014. Mycotecture (Phil Ross) Design and Violence. New York: MoMA Available at: https://www.moma.org/interactives/exhibitions/2013/designandviolence/mycotecture-phil-ross (Accessed October 18, 2021).
Travaglini, S., Dharan, C. K. H., and Ross, P. G. (2016). Manufacturing of Mycology Composites. Proc. Am. Soc. Compos. - 31st Tech. Conf. ASC.
Travaglini, S., Dharan, C. K. H., and Ross, P. G. (2015). “Thermal Properties of Mycology Materials,” in Proceedings of the American Society for Composites - 30th Technical Conference.ACS.
Travaglini, S., Dharan, C., and Ross, P. G. (2014). “Mycology Matrix Sandwich Composites Flexural Characterization,” in Proceedings of the American Society for Composites - 29th Technical Conference (ASTM-D30 Meeting), 16th., ASC US-Japan Conference on Composite Materials, La Jolla, CA.
Travaglini, S., Noble, J., Ross, P. P. G. P., and Dharan, C. C. K. H. C. (2013). Mycology Matrix Composites. Annu. Tech. Conf. Am. Soc. Compos.ASC 1, 517e535. doi:10.1017/CBO9781107415324.004
Van Wylick, A., Monclaro, A. V., Elsacker, E., Vandelook, S., Rahier, H., De Laet, L., et al. (2021). A Review on the Potential of Filamentous Fungi for Microbial Self-Healing of Concrete, Fungal Biol. Biotechnol. 8, 16. doi:10.1186/s40694-021-00122-7
Keywords: mycelium, architecture, fungus, bio-materials, building, sustainable construction
Citation: McGaw J, Andrianopoulos A and Liuti A (2022) Tangled Tales of Mycelium and Architecture: Learning From Failure. Front. Built Environ. 8:805292. doi: 10.3389/fbuil.2022.805292
Received: 30 October 2021; Accepted: 25 April 2022;
Published: 27 May 2022.
Edited by:
Cecilia Laschi, National University of Singapore, SingaporeReviewed by:
Asya Ilgün, University of Graz, AustriaAndré Stephan, Catholic University of Louvain, Belgium
Copyright © 2022 McGaw, Andrianopoulos and Liuti. This is an open-access article distributed under the terms of the Creative Commons Attribution License (CC BY). The use, distribution or reproduction in other forums is permitted, provided the original author(s) and the copyright owner(s) are credited and that the original publication in this journal is cited, in accordance with accepted academic practice. No use, distribution or reproduction is permitted which does not comply with these terms.
*Correspondence: Janet McGaw, bWNnYXdqa0B1bmltZWxiLmVkdS5hdQ==