- Department of Architecture, College of Architecture, Art and Design, Ajman University, Ajman, United Arab Emirates
Due to hot desert weather, residents of the United Arab Emirates (UAE) spend 90% of their time indoors, and the interior environment of the newly built apartments with inappropriate material and ventilation is causing sick building syndrome (SBS), faster than in any other country. NASA studies on indoor air pollutants indicate that the usage of 15–18 air-purifying plants in 18–24 cm diameter containers can clean the air in an average 167.2 m2 house (approximately one plant per 9.2 m2). This study investigates the effect of three different types of air-purifying plants, Pachira aquatica, Ficus benjamina, and Aglaonema commutatum, in reducing volatile organic compounds (VOCs) and formaldehyde (CH2O) in hot desert climate. An experiment is performed in which the CH2O and VOCs concentrations are measured in two laboratory spaces (Room 1 and Room 2). Different volumes (5 and 10% of the laboratory volume) of target plants are installed in Room 1, whereas Room 2 is measured under the same conditions without plants for comparison. The results show that the greater the planting volume (10%), the greater is the reduction effect of each VOCs. In summer in hot desert climate, the initial concentration (800 µg/cm3) of CH2O and VOCs is higher, and the reduction amount is higher (534.5 µg/cm3) as well. The reduction amount of CH2O and toluene (C7H8) is particularly high. In the case of C7H8, the reduction amount (45.9 µg/cm3) is higher in summer with Aglaonema commutatum and Ficus benjamina. It is statistically proven that Ficus benjamina is most effective in reducing CH2O and C7H8 in an indoor space in hot desert climate. The findings of this study can serve as basic data for further improving the indoor air quality using only air-purifying plants in hot desert climate of the United Arab Emirates.
1 Introduction
Residents of Dubai, United Arab Emirates (UAE), spend 90% of their time indoors because of the sweltering summers and lack of clear demarcation between different seasons (Khaleej Times, 2011; UAE, 2018; Hachicha et al., 2019; Jung and Awad, 2021a). The interior environment of the apartments in the UAE is causing sick building syndrome (SBS), faster than in any other country (Amoatey et al., 2020; Jung et al., 2021a; Awad and Jung, 2021). Moreover, due to unprecedented urbanization, many new residential projects have been constructed in the UAE, with inappropriate material and ventilation (Nassar et al., 2014; Jung et al., 2021b). This social phenomenon is accelerating the spread of SBS (Awbi, 2015). In particular, in an apartment structure in which natural ventilation is difficult to achieve and due to more hazardous the building and furnishing materials, a large quantity of volatile organic compounds (VOCs) are generated indoors (Zheng et al., 2011; Frey et al., 2014; Jung et al., 2021c). In addition, various indoor air pollutants, such as carbon dioxide (CO2), carbon monoxide (CO), formaldehyde (CH2O), radon, and microorganisms, are present in buildings (Peng et al., 2017; Carrer and Wolkoff, 2018). Dubai municipality has stipulated an indoor air quality (IAQ) of less than 0.08 ppm of formaldehyde (less than 300 µ/m3 of the total volatile organic compounds (TVOCs)) and less than 150 micrograms/m3 of suspended particulates (less than 10 microns) during 8 h of continuous monitoring prior to occupancy (Gulf News, 2020; DEWA, 2021). As general methods of reducing indoor air pollution, ventilation using various air conditioners or the usage of ecofriendly interior finishing material (Pamonpol et al., 2020; Settimo et al., 2020; Jung et al., 2021d) has been adopted. In general, although mechanical ventilation using an air-conditioning system is effective, it is expensive (Turner et al., 2013; Zhao et al., 2018). As an alternative to these methods, interest in air-purifying plants has increased in late, which can reduce costs and create a green environment (Martini et al., 2014; Liu et al., 2017; Gong et al., 2019).
Recently, in the UAE, research on IAQ improvement using indoor plants in the university classrooms in Ajman in order to enhance the learning efficiency has been conducted (Jung and Awad, 2021b). In areas such as architecture, landscaping, and environment, natural purification of indoor air using plants is being actively investigated (Armijos Moya et al., 2021). However, existing studies measure only the effect in unit space, at a basic research level, for determining the effect of plants in improving the environment, in the area of landscaping (Balocco and Leoncini, 2020; Mata et al., 2021).
This study aims to investigate the effect of three different types of air-purifying plants, Pachira aquatica, Ficus benjamina, and Aglaonema commutatum, on reducing the volatile organic compounds (VOCs) and formaldehyde (CH2O) in indoor spaces. For this purpose, an experiment was performed in which the effect of reducing pollutants in indoor space during each season was explored by varying the planting volume in an apartment. It is established that air purification using plants is possible by deriving practically usable results through this empirical experiment in a general apartment space (Gawrońska and Bakera, 2015). Moreover, it is possible to develop an environment-friendly system using air-purifying plants in the interior landscaping of apartments, which are multiunit dwellings.
2 Materials and Methods
NASA conducted an “air and water purification experiment” as part of a lunar base plan in the early 1980s and was the first to explore the improvement of the indoor air quality (IAQ) through air-purifying plants (Hort et al., 2012; Inbathamizh, 2020). NASA scientists were interested in the Earth’s self-cleaning ability, for which living plants were the solution (Berg et al., 2014; Wolverton and Nelson, 2020). Furthermore, they are exploring the possibility of creating ecological life support systems in enclosed spaces (Aydogan and Montoya, 2011).
Gas chromatography (GC) is the commonly used technique to separate smaller volatile and semi-volatile organic molecules such as hydrocarbons, alcohols, and aromatics, as well as pesticides, steroids, fatty acids, and hormones for environmental testing. Combined with the detection power of mass spectrometry (MS), gas chromatography–mass spectrometry (GC-MS) (Figure 1) can be used to separate complex mixtures, quantify analytes, identify unknown peaks, and determine trace levels of contamination. Based on GC-MS measurements, the air quality in the spacecraft raised the serious concern since more than 300 types of VOCs were detected from the indoor air of the spacecraft with the presence of the crew (Bandehali et al., 2021).
After extensive study, NASA established that plants were effective in removing formaldehyde (CH2O) in a closed laboratory (Choi, 2021). Subsequently, they developed a small, completely enclosed structure called “bio-home” designed to block heat and energy as much as possible. The interior included plastics and other synthetic materials to enable the discharge of VOCs for causing the appearance of typical SBS symptoms (Nelson and Wolverton, 2011). The indoor air samples collected and measured before and after the placement of indoor plants in a planter (a large container for planting plants) in the bio-home indicated that the VOCs were reduced (Kaur et al., 2014). In addition, NASA tested the air purification effect of plants that can grow indoors (Schlacht et al., 2016). The results revealed that indoor plants could absorb and purify aromatic organic compounds, such as toluene (C7H8), benzene (C6H6), and formaldehyde (CH2O), at 350–1,200 µg per 100 cm2 of the leaf area per day (Pegas et al., 2012; Rodgers et al., 2013). Moreover, the usage of plants can be the most representative method for biological decontamination (Su, 2014; Brilli et al., 2018). Although the results of such a system are scientifically insufficient to establish the degree of indoor air pollution reduction, it is certain that it can be applied sufficiently as a method for reducing indoor air pollutants (Dela Cruz et al., 2014). As such, the ability of plants to purify air has been studied for a long time.
In this study, the changes in the VOC and formaldehyde (CH2O) concentration in a room were identified by varying the volume of air-purifying plants, such as Pachira aquatica, Ficus benjamina, and Aglaonema commutatum (Park et al., 2010; Son et al., 2019) (Figure 2). The leaf area of the 3 target plants was 900 cm2/pot, calculated using an AutoCAD program after scanning the average leaf. 3 indoor air-purifying plants (pot size: 15 cm × 21 cm) are placed in line next to the windows in the laboratory. Plants were arranged in 10% (1.75 m2) and 5% (0.875 m2) of the laboratory space, and an experiment was conducted from March 4th, 2020, to February 24th, 2021 (Table 1).
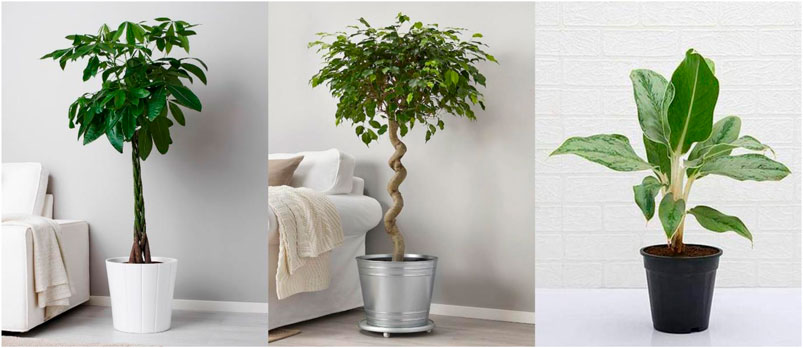
FIGURE 2. Target plants (left: Pachira aquatica, middle: Ficus benjamina, and right: Aglaonema commutatum).
Planting of Pachira aquatica, Ficus benjamina, and Aglaonema commutatum (Table 2) was performed in 10% (1.75 m2) and 5% (0.875 m2) of the laboratory space volume (Pennisi and van Iersel, 2012). An actual apartment setting was divided as used in a laboratory, which included a living room and balcony (3.5 m in width, 3.5 m in length, and 2.4 m in height) (Kim et al., 2020a).
The experimental space included two rooms, Room 1 and Room 2, with and without the target plants, respectively, in which the changes in the formaldehyde (CH2O) and VOC concentrations were measured under the same conditions for comparison (1.5 m high in the center of the room) (Kim et al., 2010a; Kim et al., 2010b; Kim et al., 2014). The target plants were 2 years old and had been propagated through bushes; there was a minor difference in the number of pots, but it was ensured that the volume of the plants was the same, in the experiment (Moya et al., 2019).
The plant volume was measured in terms of the width, length, and height (900 cm2/pot) (Lee et al., 2018). The concentrations of toluene (C7H8), ethylbenzene (C8H10), xylene (C8H10), styrene (C8H8), and formaldehyde (CH2O) among the individual VOCs were measured under the same conditions in Room 1 and Room 2. The interior material of these two experimental spaces was the same. A certain quantity of VOCs was artificially generated, and the indoor air was collected with a minipump without manual intervention. In Room 1, the initial concentration was measured 24 h after the target plant was installed; Room 2, without plants, was measured simultaneously (Kim and Kim, 2016). The second measurement was performed after 48 h in both rooms, and the final measurement was performed after 72 h. In another experiment, measurement was performed five times a day (9:00, 12:00, 15:00, 18:00, and 21:00) (Nitmetawong et al., 2020). As a reduction in the VOC concentration was observed between 12:00 PM and 15:00 PM, measurements were performed only once a day at 15:00 PM (Cao et al., 2019). In addition, as a process test method for apartment houses, after 30 min of ventilation and 5 h of sealing, the experiment was conducted between 13:00 PM and 17:00 PM. Figure 3 shows the laboratory plan and the measurement locations.
VOCs samples such as toluene (C7H8), ethylbenzene (C8H10), xylene (C8H10), and styrene (C8H8) were collected using a charcoal tube, and formaldehyde (CH2O) sample was collected using a DNPH cartridge (Brągoszewska et al., 2019), which were extracted with CS2 (carbon disulfide) and acetonitrile solutions, respectively. After extraction, VOCs were analyzed via gas chromatography with flame ionization detection (GC-FID), whereas formaldehyde (CH2O) was analyzed via high-performance liquid chromatography (HPLC) (Gunasinghe et al., 2021).
3 Results
3.1 Effect of 10% Planting on VOC Concentration Reduction
In order to investigate the effect of the volume of each plant in the reduction of the VOC and formaldehyde (CH2O) concentrations, an experiment was conducted by planting 10% of the laboratory space volume (Gawronski et al., 2017). As the reduction in indoor air pollutants by plants is affected by solar radiation, all the plants were placed near a window (Kim et al., 2020b; Zheng et al., 2020). In addition, as the sizes of the three types of plants used in the experiment differed slightly, the number of pots was varied to ensure that the total volume was the same (Zhou et al., 2011; Han and Ruan, 2020). Among the three plants, Aglaonema commutatum had the widest and longest leaves, but the number was rather less. The Pachira aquatica and Ficus benjamina trees were tall with small and numerous leaves. These three types of target plants were selected among seven types of air-purifying plants based on their excellent results in previous experiments (Kim et al., 2016; Fasihi et al., 2019) (Table 3).
3.1.1 Spring (March 2020–May 2020)
Pachira aquatica, Ficus benjamina, and Aglaonema commutatum trees were placed in 10% of the laboratory space during the spring season, from March to May 2020, and the change in the VOC concentration was measured. In the case of benzene (C6H6), there was a reduction in 22.7 µg/cm3 after the installation of Ficus benjamina, which was the maximum (Figure 4). For toluene (C7H8), the reduction effect of Ficus benjamina was the best. Toluene (C7H8) showed a reduction of approximately 19.2–35.4 µg/cm3 with each plant, which was relatively greater than that of benzene (C6H6) (Figure 5). The reduction of toluene (C7H8), whose concentration is generally high in apartments, is crucial; Ficus benjamina had the best effect in spring, among the three plants. The reduction amount of ethylbenzene (C8H10) when Pachira aquatica was installed was 11.9 µg/cm3, which was lesser than that of benzene (C6H6) or toluene (C7H8) (Figure 6). The effect of Ficus benjamina was excellent for benzene (C6H6), whereas, for toluene (C7H8), Pachira aquatica was the best. After Pachira aquatica, the ethylbenzene (C8H10) reduction effect of Ficus benjamina was excellent. In the case of xylene (C8H10) (Figure 7) and styrene (C8H8) (Figure 8), the effect was maximum when 10% Aglaonema commutatum was installed. The formaldehyde (CH2O) reduction effect was maximum with 10% Ficus benjamina (Figure 9).
Formaldehyde (CH2O) is generated by the building materials, preservatives, furniture, and adhesives used in interior finishing. In particular, its concentration is five times than those of the other VOCs; hence, reduction measures are urgently needed. Considering the concentration of the indoor air pollutants, the reduction of the other VOCs is also important; however, the placement of plants effective in reducing formaldehyde (CH2O) in spring will have a good effect. In addition, it was found Ficus benjamina was highly effective in reducing most VOCs, including formaldehyde (CH2O), benzene (C6H6), and toluene (C7H8).
3.1.2 Summer (June 2020–August 2020)
Another experiment was conducted from June–early September 2020, when the weather in Dubai was hot. Due to the characteristics of VOCs, their initial concentrations were mostly higher in summer when the temperature was high, compared to spring. When 10% Pachira aquatica was installed, the reduction in the concentration of benzene (C6H6) was maximum during each season (Figure 4). Among the VOCs, toluene (C7H8) was reduced by 45.9 µg/cm3 when 10% Ficus benjamina was installed; the reduction was maximum in summer, and the effect was excellent (Figure 5). In the case of ethylbenzene (C8H10) (Figure 6) and xylene (C8H10) (Figure 7), the concentration was mostly below 40 µg/cm3. Maximum reduction in the ethylbenzene (C8H10) and xylene (C8H10) concentrations was observed when Pachira aquatica and Aglaonema commutatum were installed, respectively. In particular, the reduction in xylene (C8H10) on planting Aglaonema commutatum was thrice, compared to Pachira aquatica or Ficus benjamina. In the case of formaldehyde (CH2O) (Figure 9), the reduction was 534.4 µg/cm3, 330.7 µg/cm3, and 321.9 µg/cm3, when Ficus benjamina, Aglaonema commutatum, and Pachira aquatica were installed, respectively.
The initial concentration was approximately 800 µg/cm3, and it decreased in all the cases. The reduction in the concentration of formaldehyde (CH2O) was almost the same during the 3 days of measurement, and the effect was most significant when Ficus benjamina was installed.
3.1.3 Autumn (September 2020–November 2020)
In autumn, the overall concentration was similar to that in spring because of the similarity in temperature, in the UAE. Benzene (C6H6) with similar initial concentrations of approximately 20 µg/cm3 in spring and autumn showed a reduction of 14.7–16.6 µg/cm3. Ficus benjamina achieved maximum benzene (C6H6) reduction (Figure 4). In the case of toluene (C7H8), the best effect was observed when Pachira aquatica was installed; Ficus benjamina was also effective (Figure 5). The reduction effect of ethylbenzene (C8H10) was the maximum with Pachira aquatica, whereas xylene (C8H10) and styrene (C8H8) showed the maximum reduction in concentration with Ficus benjamina (Figure 6). The initial concentration of formaldehyde (CH2O) was 800 µg/cm3 in autumn, similar to that in spring; however, the decrease in concentration was slightly greater than that in spring (Figure 9). Among the three plants, Aglaonema commutatum was the most in reducing formaldehyde (CH2O) to 361.6 µg/cm. The concentration was reduced from 812.2 µg/cm3 to 680.2 µg/cm3 after 48 h and 450.6 µg/cm3 after 72 h; a gradual reduction of 200 µg/cm3 was observed each day. Aglaonema commutatum exhibited the best effect for formaldehyde (CH2O) reduction, followed by Pachira aquatica and Ficus benjamina with similar reductions of 289.9 µg/cm3. When the planting volume was 10% in autumn, Ficus benjamina had the best effect for each VOC substance; however, in the case of formaldehyde (CH2O), which constituted a large portion of the pollutant concentration, Aglaonema commutatum had the best effect.
3.1.4 Winter (December 2020–February 2021)
Furthermore, the experiment was conducted in winter between December 2020 and February 2021, and the measurements were made at a slightly lower temperature. VOC release in the winter experiment was at a slightly lower concentration compared to the other seasons because of the low temperature. The reduction effect was not very good for most VOC substances. In the case of benzene (C6H6), the reduction was 5.5–9.3 µg/cm3, and the effect of Aglaonema commutatum was the best at 9.3 µg/cm3 (Figure 4). In the case of ethylbenzene (C8H10), xylene (C8H10), and styrene (C8H8), the reduction amount was approximately 10.0 µg/cm3 (Figures 6–8). Except for ethylbenzene (C8H10), the effect was excellent when Aglaonema commutatum was installed; for ethylbenzene (C8H10), Pachira aquatica was effective. The initial concentration of formaldehyde (CH2O) in winter was found to be approximately 200 µg/cm3 lower than those of the other seasons (Figure 9). Pachira aquatica was the most effective in reducing the concentration of formaldehyde (CH2O). With the other air-purifying plants, concentration reduction was observed, but it was less on the second day.
3.1.5 Reduction Rate According to the Season
With 10% planting, the reduction of VOCs and formaldehyde (CH2O) was expressed as a reduction ratio. In most cases, the best effect was observed in summer (Stevenson et al., 2020). In the case of benzene (C6H6) in Figure 4, the reduction effect was maximum in summer and autumn, similar to toluene (C7H8) in Figure 5. Ethylbenzene (C8H10) showed a reduction of 86.4% in Figure 6 when Pachira aquatica was planted in summer, and most of the benzene (C6H6) was removed. According to the season, the reduction ratio of benzene (C6H6) was 29.4–36.8% in spring, 47.4–86.4% in summer, 15.8–70.1% in autumn, and 34.4–53.4% in winter. In some cases, the reduction amount and reduction ratio were slightly different depending on the difference in the initial VOC and formaldehyde (CH2O) concentrations (Suárez-Cáceres and Pérez-Urrestarazu, 2021). However, most of the experimental results indicated that the concentration reduction effect was excellent in spring, summer, and autumn. In winter, when the temperature was low, the reduction amount and reduction ratio were poor compared to the other seasons (Weyens et al., 2015) (Table 2).
Figures 4–9 depict the changes in the concentration of benzene (C6H6), toluene (C7H8), ethylbenzene (C8H10), xylene (C8H10), styrene (C8H8), and formaldehyde (CH2O), respectively, when the three types of air-purifying plants occupy 10% of the laboratory space volume. The VOC and formaldehyde (CH2O) concentrations were measured thrice, 24 h, 48 h, and 72 h after planting.
3.2 Effect of 5% Planting on VOC Concentration Reduction
To investigate the effect of the planting volume of each plant on the reduction of the VOC and formaldehyde (CH2O) concentrations, plants were placed in 5% of the laboratory space, and the following experiment was conducted. The plants were placed near a window, considering the effect of solar radiation. The experimental method was the same as in that in the case of 10% planting, and the only change was in the number of plants.
3.2.1 Spring (March 2020–May 2020)
The changes in the concentration of formaldehyde (CH2O) and the VOCs were identified after setting the planting volume to 5%, in each season. In a previous experiment, the planting volume was set to 10, 5, and 3%, respectively, and the results were compared. It was found that the concentration reduction effect was effective when plants were installed in more than 5% of the laboratory space.
As shown in Figure 10, the reduction in benzene (C6H6) concentration was ranged from 14.9 to 34.9 µg/cm3. In spring, the reduction in benzene (C6H6) concentration was maximum when Ficus benjamina was installed; Pachira aquatica was effective as well. However, with Aglaonema commutatum, the reduction was lesser than twice that of Pachira aquatica and Ficus benjamina. The toluene (C7H8) concentration, as shown in Figure 11, was reduced in all the seasons, and it was found that the reduction effects of Aglaonema commutatum and Ficus benjamina were considerable. Among these two plants, the effect of Aglaonema commutatum was slightly better. The initial concentration value of toluene (C7H8) in spring was approximately 60.0 µg/cm3, similar to that in autumn; however, the decrease in concentration was slightly more than that in autumn. In addition, the initial concentration was lower than that in summer, and the amount of reduction was also less. However, all the results were better compared to winter.
In the case of ethylbenzene (C8H10), as shown in Figure 12, all the plants were effective in all the seasons. For reducing ethylbenzene (C8H10) in spring, 5% Aglaonema commutatum was the most effective, followed Pachira aquatica and Ficus benjamina; however, the reduction was greater on the third day than the second. The reduction of xylene (C8H10) (Figure 13) and styrene (C8H8) (Figure 14) was high in summer but low in spring. Both xylene (C8H10) and styrene (C8H8) were effectively reduced when Pachira aquatica was installed; Aglaonema commutatum was also effective in reducing xylene (C8H10). The concentration value of styrene (C8H8) ranged from 15.0 to 40.0 µg/cm3; in spring, the concentration gradually decreased from the initial concentration of 30.0 µg/cm3 and dropped significantly on the third day.
As depicted in Figure 15, the concentration of formaldehyde (CH2O) gradually decreased from approximately 800.0 µg/cm3 at the beginning of the measurement. Among the three plants, when Aglaonema commutatum was installed, the reduction amount was 200.0 µg/cm3, which was the maximum. In spring, the initial concentration was similar or slightly lower than that in summer, but the reduction amount was lesser.
3.2.2 Summer (June 2020–August 2020)
All the measurements were made from June to late August during summer, and the concentrations were higher than those in the other seasons. The effect of 5% planting was lesser than that of 10% planting. As shown in Figure 10, the reduction in benzene (C6H6) concentration in summer was maximum at 20.0 µg/cm3 when Pachira aquatica was planted. The initial concentration of benzene (C6H6) was approximately 20.0 µg/cm3 in the other seasons, but in summer, the initial concentration was 40.0–50.0 µg/cm3. In the case of toluene (C7H8), Aglaonema commutatum was most effective when planted in the summer, compared to other seasons (Figure 11). When 5% Aglaonema commutatum was installed, 60.0 µg/cm3 of toluene (C7H8) was reduced.
For ethylbenzene (C8H10), the concentration reduction effect of Pachira aquatica was the highest in summer, as shown in Figure 12. The reduction in ethylbenzene (C8H10) and styrene (C8H8) was maximum with Pachira aquatica, whereas Aglaonema commutatum was most effective in reducing xylene (C8H10). The effect of 10% as well as 5% Ficus benjamina planting was excellent in the case of formaldehyde (CH2O) (Figure 15). With 10% Ficus benjamina planting, the reduction amount was 534.4 µg/cm3, whereas 5% planting showed a reduction of 414.1 µg/cm3.
3.2.3 Autumn (September 2020–November 2020)
In autumn, the VOC and formaldehyde (CH2O) concentrations were measured in October and November. The effect of Aglaonema commutatum was excellent for almost all the VOCs, whereas the reduction effect of Pachira aquatica for benzene (C6H6) and formaldehyde (CH2O) was excellent. The initial concentration of benzene (C6H6) in autumn was lower than that in spring and summer (Figure 10). The reduction effect of Aglaonema commutatum was good in autumn, whereas Pachira aquatica and Ficus benjamina had excellent effect in spring. With Pachira aquatica, the concentration reduction of toluene (C7H8) was maximum at 25.0 µg/cm3 in autumn (Figure 11). In the case of toluene (C7H8), the concentration was reduced to 10.8 µg/cm3 and 22.0 µg/cm3 with Aglaonema commutatum and Ficus benjamina, respectively. Ethylbenzene (C8H10) (Figure 12), xylene (C8H10) (Figure 13), and styrene (C8H8) (Figure 14) showed the best reduction when Pachira aquatica was installed at 5%. In the case of formaldehyde (CH2O), the reduction was lower than that in summer, but when Aglaonema commutatum was installed, a large reduction of 268.8 µg/cm3 was observed (Figure 15). In autumn, 10% planting realized a greater reduction of approximately 92.7 µg/cm3. The greater the planting volume, the greater was the reduction effect.
3.2.4 Winter (December 2000–February 2021)
In winter, the concentration reduction effect was insignificant compared to the other seasons, and the reduction amount was less than 10.0 µg/cm3 in most cases. In the case of benzene (C6H6), the effect was greater when Pachira aquatica was planted (Figure 10). Aglaonema commutatum was most effective in reducing toluene (C7H8) (Figure 11), ethylbenzene (C8H10) (Figure 12), and styrene (C8H8) (Figure 14), whereas Ficus benjamina was the most effective for xylene (C8H10) (Figure 13). Formaldehyde (CH2O) exhibited a greater reduction in concentration than other VOCs, and the effect was excellent when Pachira aquatica was installed (Figure 15). The formaldehyde (CH2O) concentration changes in winter were 60.0 µg/cm3 or more, and the maximum reduction was 126.0 µg/cm3 when Pachira aquatica 5% was planted.
3.2.5 Reduction Rate as per Season
In the experiment with 5% planting volume, the reduction in the concentration of each VOC and formaldehyde (CH2O), depicted as a reduction ratio, was maximum in summer (Pichlhöfer et al., 2021). In the case of benzene (C6H6), when Ficus benjamina was planted in summer, the reduction effect was maximum at 61.1%, whereas it was 40–50% in spring and autumn (Figure 10). In the case of toluene (C7H8), when Aglaonema commutatum was planted in summer, the effect was excellent at 62.7%, and the reduction effect was close to 50%, except in winter (Figure 11). However, as the initial concentrations were slightly different, the same reduction amount and reduction ratio were not realized in all the seasons (Li et al., 2016). For example, the reduction amount as well as reduction ratio was maximum with the planting of Aglaonema commutatum in summer. However, in spring, when Pachira aquatica was planted, the reduction amount was 15.2 µg/cm3; Ficus benjamina was more effective, with a reduction of 28.6 µg/cm3. Due to the difference in the initial concentration, the reduction ratio with Pachira aquatica was 38.1%, which was better than the reduction ratio of 36.7% with Ficus benjamina.
The effect on the reduction ratio and reduction amount of formaldehyde (CH2O) was the same (Figure 15). When Ficus benjamina was planted in summer, the reduction amount was 414.1 µg/cm3, and the reduction ratio was 42.0%, which was the most effective. With Aglaonema commutatum, Pachira aquatica, and Ficus benjamina, the reduction ratios were 9.7–35.3%, 19.1–37.7%, and 14.6–42.1%, respectively. The experimental results with the three plants demonstrated that the reduction amount of formaldehyde (CH2O) was greater than those of the other individual VOCs. However, the reduction ratio was approximately 20–40%, which was lesser than those of the other VOCs. There was a difference in the initial concentration of each VOC. For the reduction ratio, it was found that a slight decrease in concentration at a low initial concentration was more effective than a decrease in concentration at a high concentration (Teiri et al., 2018).
Figures 10–15 depict the change in the concentration of benzene (C6H6), toluene (C7H8), ethylbenzene (C8H10), xylene (C8H10), styrene (C8H8), and formaldehyde (CH2O), respectively, when plants occupy 5% of the laboratory space volume. Table 4 lists the reduction amount of the VOCs and formaldehyde with respect to the planting volume.
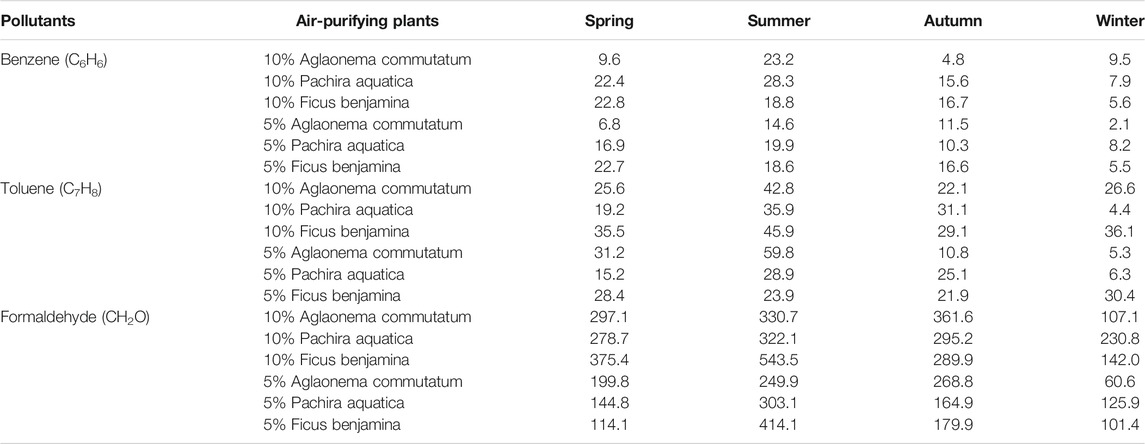
TABLE 4. VOC and formaldehyde (CH2O) concentration reduction with respect to the planting volume (10 and 5%).
4 Discussion
This study addresses the improvement of the indoor air quality using three types of indoor air-purifying plants alone. However, in future research, more diverse air-purifying plants will be integrated with building design and ventilation facilities as green walls or green ceilings to achieve better effects.
As the awareness of sustainability increases in hot desert climate, the use of indoor air-purifying plants will become more active due to over 41°C scorching summer. Especially in Dubai, UAE, without much green landscape in the urban context, residents will be eager to have indoor plants in their houses. Not only as the form of a potted plant but there are also many methods to implement indoor air-purifying plants seamlessly with building design such as green wall and green ceiling.
These indoor plant implementations could help to achieve better LEED (leadership in energy and environmental design) since LEED has indoor environmental quality (IEQ) category. As a biophilia design, a biofilter wall could be installed with three indoor air plants from this research such as Pachira aquatica, Ficus benjamina, and Aglaonema commutatum as hydroponic plants. Since these hydroponic green walls help conserve water in conditions where active humidification is required, they reduce HVAC loads by 15% and boost the relative humidity by up to 5–30% based on the 2008 study carried out by the Indian government.
5 Conclusion
In this study, the effect of the planting volume on the reduction of the formaldehyde (CH2O) and VOC concentrations was investigated for three types of air-purifying plants, namely, Pachira aquatica, Ficus benjamina, and Aglaonema commutatum.
The experimental results indicated that the greater the planting volume (10% of the laboratory space, 1.75 m2), the greater was the reduction effect for each VOC. Investigation of the change in the formaldehyde (CH2O) and VOC concentrations according to the season established that the initial concentration was high in summer, and the reduction effect was also excellent. In the case of benzene (C6H6), the reduction effect was maximum in summer and autumn, similar to toluene (C7H8). Ethylbenzene (C8H10) showed a reduction of 86.4% when Pachira aquatica was planted in summer. According to the season, the reduction ratio of benzene (C6H6) was 29.4–36.8% in spring, 47.4–86.4% in summer, 15.8–70.1% in autumn, and 34.4–53.4% in winter.
The reduction of the concentration of formaldehyde (CH2O) and toluene (C7H8) was particularly high, and in the case of toluene (C7H8), the concentration reduction effect was excellent with Aglaonema commutatum and Ficus benjamina in summer. It was determined that the concentration reduction effect was the best when the plants were installed in summer. Toluene (C7H8) was reduced by 45.9 µg/cm3 when 10% Ficus benjamina was installed; the reduction was maximum in summer, and the effect was excellent. In the case of ethylbenzene (C8H10) and xylene (C8H10), the concentration was mostly below 40 µg/cm3. Maximum reduction in the ethylbenzene (C8H10) and xylene (C8H10) concentrations was observed when Pachira aquatica and Aglaonema commutatum were installed, respectively. In particular, the reduction in xylene (C8H10) on planting Aglaonema commutatum was thrice, compared to Pachira aquatica or Ficus benjamina. In the case of formaldehyde (CH2O), the reduction was 534.4 µg/cm3, 330.7 µg/cm3, and 321.9 µg/cm3, when Ficus benjamina, Aglaonema commutatum, and Pachira aquatica were installed, respectively.
Although the reduction of all the VOC substances in an apartment is crucial, as formaldehyde (CH2O) and toluene (C7H8) are more hazardous, planting Ficus benjamina in indoor spaces could be more effective. The initial concentration was approximately 800 µg/cm3, and it decreased in all the cases. The reduction in the concentration of formaldehyde (CH2O) was almost the same during the 3 days of measurement, and the effect was most significant when Ficus benjamina was installed.
This study is meaningful in revealing the trend of the purification effect using the three plants for a long period of time, and the limitation of the study, however, is that it was not possible to do the experiment in apartment conditions where various conditions can occur because the actual place of the experiment is inhabited by people.
Data Availability Statement
The original contributions presented in the study are included in the article/Supplementary Material, and further inquiries can be directed to the corresponding author.
Author Contributions
All the authors contributed significantly to this study. CJ and NA identified and secured the example buildings used in the study. The data acquisition system was designed, and the sensors were installed by CJ and NA. NA was responsible for data collection. Data analysis was performed by CJ. The article was compiled by CJ and reviewed by NA. All the authors have read and agreed to the published version of the article.
Conflict of Interest
The authors declare that the research was conducted in the absence of any commercial or financial relationships that could be construed as a potential conflict of interest.
Publisher’s Note
All claims expressed in this article are solely those of the authors and do not necessarily represent those of their affiliated organizations, or those of the publisher, the editors, and the reviewers. Any product that may be evaluated in this article, or claim that may be made by its manufacturer, is not guaranteed or endorsed by the publisher.
Acknowledgments
The authors would like to express their gratitude to Ajman University for the generous support in publishing this paper.
References
Amoatey, P., Omidvarborna, H., Baawain, M. S., Al-Mamun, A., Bari, A., and Kindzierski, W. B. (2020). Association between Human Health and Indoor Air Pollution in the Gulf Cooperation Council (GCC) Countries: A Review. Rev. Environ. Health 35 (2), 157–171. doi:10.1515/reveh-2019-0065
Armijos Moya, T., Ottelé, M., van den Dobbelsteen, A., and Bluyssen, P. M. (2021). The Effect of an Active Plant-Based System on Perceived Air Pollution. Int. J. Environ. Res. Publ. Health 18 (15), 8233. doi:10.3390/ijerph18158233
Awad, J., and Jung, C. (2021). Evaluating the Indoor Air Quality after Renovation at the Greens in Dubai, United Arab Emirates. Buildings 11 (8), 353. doi:10.3390/buildings11080353
Awbi, H. B. (2015). Ventilation and Air Distribution Systems in Buildings. Front. Mech. Eng. 1, 4. doi:10.3389/fmech.2015.00004
Aydogan, A., and Montoya, L. D. (2011). Formaldehyde Removal by Common Indoor Plant Species and Various Growing media. Atmos. Environ. 45 (16), 2675–2682. doi:10.1016/j.atmosenv.2011.02.062
Balocco, C., and Leoncini, L. (2020). Energy Cost for Effective Ventilation and Air Quality for Healthy Buildings: Plant Proposals for a Historic Building School Reopening in the Covid-19 Era. Sustainability 12 (20), 8737. doi:10.3390/su12208737
Bandehali, S., Miri, T., Onyeaka, H., and Kumar, P. (2021). Current State of Indoor Air Phytoremediation Using Potted Plants and green walls. Atmosphere 12 (4), 473. doi:10.3390/atmos12040473
Berg, G., Mahnert, A., and Moissl-Eichinger, C. (2014). Beneficial Effects of Plant-Associated Microbes on Indoor Microbiomes and Human Health. Front. Microbiol. 5, 15. doi:10.3389/fmicb.2014.00015
Brągoszewska, E., Bogacka, M., and Pikoń, K. (2019). Efficiency and Eco-Costs of Air Purifiers in Terms of Improving Microbiological Indoor Air Quality in Dwellings-A Case Study. Atmosphere 10 (12), 742. doi:10.3390/atmos10120742
Brilli, F., Fares, S., Ghirardo, A., de Visser, P., Calatayud, V., Muñoz, A., et al. (2018). Plants for Sustainable Improvement of Indoor Air Quality. Trends Plant Sci. 23 (6), 507–512. doi:10.1016/j.tplants.2018.03.004
Cao, Y., Li, F., Wang, Y., Yu, Y., Wang, Z., Liu, X., et al. (2019). Assisted Deposition of PM2.5 from Indoor Air by Ornamental Potted Plants. Sustainability 11 (9), 2546. doi:10.3390/su11092546
Carrer, P., and Wolkoff, P. (2018). Assessment of Indoor Air Quality Problems in Office-like Environments: Role of Occupational Health Services. Int. J. Environ. Res. Publ. Health 15 (4), 741. doi:10.3390/ijerph15040741
Choi, J. H. (2021). The Selection of Plants for Indoor Garden and the Environmental Improvement Effects. Int. J. Adv. Cult. Technol. 9 (1), 129–135. doi:10.17703/IJACT.2021.9.1.129
Dela Cruz, M., Christensen, J. H., Thomsen, J. D., and Muller, R. (2014). Can Ornamental Potted Plants Remove Volatile Organic Compounds from Indoor Air? - a Review. Environ. Sci. Pollut. Res. 21 (24), 13909–13928. doi:10.1007/s11356-014-3240-x
DEWA (2021). Green Building Regulations & Specifications. Available at: https://www.dewa.gov.ae/∼/media/Files/Consultants%20and%20Contractors/Green%20Building/Greenbuilding_Eng.ashx (Accessed July 30, 2021)
Fasihi, M., Efimova, O., and Breyer, C. (2019). Techno-economic Assessment of CO2 Direct Air Capture Plants. J. Clean. Prod. 224, 957–980. doi:10.1016/j.jclepro.2019.03.086
Frey, S. E., Destaillats, H., Cohn, S., Ahrentzen, S., and Fraser, M. P. (2014). Characterization of Indoor Air Quality and Resident Health in an Arizona Senior Housing Apartment Building. J. Air Waste Manage. Assoc. 64 (11), 1251–1259. doi:10.1080/10962247.2014.937513
Gawrońska, H., and Bakera, B. (2015). Phytoremediation of Particulate Matter from Indoor Air by Chlorophytum Comosum L. Plants. Air Qual. Atmos. Health 8 (3), 265–272. doi:10.1007/s11869-014-0285-4
Gawronski, S. W., Gawronska, H., Lomnicki, S., Sæbo, A., and Vangronsveld, J. (2017). Plants in Air Phytoremediation. Adv. Bot. Res. 83, 319–346. doi:10.1016/bs.abr.2016.12.008
Gong, Y., Zhou, T., Wang, P., Lin, Y., Zheng, R., Zhao, Y., et al. (2019). Fundamentals of Ornamental Plants in Removing Benzene in Indoor Air. Atmosphere 10 (4), 221. doi:10.3390/atmos10040221
Gulf News (2020). Let’s Not Forget Indoor Air Quality as Well. Available at: https://gulfnews.com/business/analysis/lets-not-forget-indoor-air-quality-as-well-1.1589873286956 (Accessed August 10, 2021).
Gunasinghe, Y. H. K. I. S., Rathnayake, I. V. N., and Deeyamulla, M. P. (2021). Plant and Plant Associated Microflora: Potential Bioremediation Option of Indoor Air Pollutants. Nepal J. Biotechnol. 9 (1), 63–74. doi:10.3126/njb.v9i1.38669
Hachicha, A. A., Al-Sawafta, I., and Said, Z. (2019). Impact of Dust on the Performance of Solar Photovoltaic (PV) Systems under United Arab Emirates Weather Conditions. Renew. Energ. 141, 287–297. doi:10.1016/j.renene.2019.04.004
Han, K.-T., and Ruan, L.-W. (2020). Effects of Indoor Plants on Air Quality: A Systematic Review. Environ. Sci. Pollut. Res. 27 (14), 16019–16051. doi:10.1007/s11356-020-08174-9
Hort, D., Cantor, M., Buta, E., and Andriescu, I. (2012). Benefits and Positive Effects of Some Houseplants on Indoor Air Quality. Agricultura 81 (1-2). doi:10.15835/arspa.v81i1-2.8127
Inbathamizh, L. (2020). Indoor Medicinal Plants: Beneficial Biocatalysts for Air Filtration and Bioremediation–A Review. Int. J. Green Pharm. 14 (02), 130–137. doi:10.22377/ijgp.v14i02.2875
Jung, C., and Awad, J. (2021). The Improvement of Indoor Air Quality in Residential Buildings in Dubai, UAE. Buildings 11 (6), 250. doi:10.3390/buildings11060250
Jung, C., and Awad, J. (2021). Improving the IAQ for Learning Efficiency with Indoor Plants in university Classrooms in Ajman, United Arab Emirates. Buildings 11 (7), 289. doi:10.3390/buildings11070289
Jung, C., Al Qassimi, N., Arar, M., and Awad, J. (2021). The Analysis of Indoor Air Pollutants from Finishing Material of New Apartments at Business Bay, Dubai. Front. Built Environ. 7, 765689. doi:10.3389/fbuil.2021.765689
Jung, C., Awad, J., Sami Abdelaziz, N., and Salameh, M. (2021). An Analysis of Indoor Environment Evaluation for the Springs Development in Dubai. UAE: Open House International.
Jung, C., Awad, J., and Al Qassimi, N. (2021). Analyzing the Users’ Satisfaction Levels and Perceptions of the Dubai Water Canal for Future Waterfront Development in UAE. Future Cities Environ. 7 (1). doi:10.5334/fce.134
Jung, C., Awad, J., and Chohan, A. (2021). The Planning of Smart Elderly Housing in Dubai with IoT Technologies. UAE: Open House International.
Kaur, A., Misra, A., and Misra, A. (2014). Impact of Indoor Surface Materials and Environment on Perceived Air Quality. EH 2014 (1), 25–35. doi:10.15764/eh.2014.01004
Khaleej Times (2011). Sick Building Syndrome: The Killer within. Available at: https://www.khaleejtimes.com/nation/general/sick-building-syndrome-the-killer-within (Accessed July 24, 2021)
Kim, H., and Kim, H.-H. (2016). Assessment of Indoor Air Quality and the Eye Symptom of Occupants in Newly-Built Office Building after Planting Indoor Plants. J. Korean Ophthalmic Opt. Soc. 21 (3), 265–274. doi:10.14479/jkoos.2016.21.3.265
Kim, H.-H., Park, J.-W., Yang, J.-Y., Kim, K.-J., Lee, J.-Y., Shin, D.-C., et al. (2010). Evaluating the Relative Health of Residents in Newly Built Apartment Houses According to the Presence of Indoor Plants. J. Jpn. Soc. Hort. Sci. 79 (2), 200–206. doi:10.2503/jjshs1.79.200
Kim, K. J., Jeong, M. I., Lee, D. W., Song, J. S., Kim, H. D., Yoo, E. H., et al. (2010). Variation in Formaldehyde Removal Efficiency Among Indoor Plant Species. Horts 45 (10), 1489–1495. doi:10.21273/hortsci.45.10.1489
Kim, H.-H., Yang, J.-Y., Lee, J.-Y., Park, J.-W., Kim, K.-J., Lim, B.-S., et al. (2014). House-plant Placement for Indoor Air Purification and Health Benefits on Asthmatics. Environ. Health Toxicol. 29, e2014014. doi:10.5620/eht.e2014014
Kim, K. J., Kim, H. J., Khalekuzzaman, M., Yoo, E. H., Jung, H. H., and Jang, H. S. (2016). Removal Ratio of Gaseous Toluene and Xylene Transported from Air to Root Zone via the Stem by Indoor Plants. Environ. Sci. Pollut. Res. 23 (7), 6149–6158. doi:10.1007/s11356-016-6065-y
Kim, K. J., Shagol, C. C., Torpy, F. R., Pettit, T., and Irga, P. J. (2020). “Plant Physiological Mechanisms of Air Treatment,” in From Biofiltration to Promising Options in Gaseous Fluxes Biotreatment (Amsterdam: Elsevier), 219–244. doi:10.1016/b978-0-12-819064-7.00011-x
Kim, H.-H., Kwak, M.-J., Kim, K.-J., Gwak, Y.-K., Lee, J.-H., and Yang, H.-H. (2020). Evaluation of IAQ Management Using an IoT-Based Indoor Garden. Int. J. Environ. Res. Publ. Health 17 (6), 1867. doi:10.3390/ijerph17061867
Lee, J. H., An, S. M., Kwak, M. J., Kim, K. J., and Kim, H. H. (2018). Development of an IAQ index for Indoor Garden Based IoT Applications for Residents’ Health Management. J. Environ. Health Sci. 44 (5), 421–432. doi:10.5668/JEHS.2018.44.5.421
Li, J., Xie, C.-J., Cai, J., Yan, L.-S., and Lu, M.-M. (2016). Removal of Low-Molecular Weight Aldehydes by Selected Houseplants under Different Light Intensities and CO2 Concentrations. Atmosphere 7 (11), 144. doi:10.3390/atmos7110144
Liu, J., Zhu, J., Zhang, P., Han, L., Reynolds, O. L., Zeng, R., et al. (2017). Silicon Supplementation Alters the Composition of Herbivore Induced Plant Volatiles and Enhances Attraction of Parasitoids to Infested rice Plants. Front. Plant Sci. 8, 1265. doi:10.3389/fpls.2017.01265
Martini, X., Pelz-Stelinski, K. S., and Stelinski, L. L. (2014). Plant Pathogen-Induced Volatiles Attract Parasitoids to Increase Parasitism of an Insect Vector. Front. Ecol. Evol. 2, 8. doi:10.3389/fevo.2014.00008
Mata, T. M., Oliveira, G. M., Monteiro, H., Silva, G. V., Caetano, N. S., and Martins, A. A. (2021). Indoor Air Quality Improvement Using Nature-Based Solutions: Design Proposals to Greener Cities. Int. J. Environ. Res. Publ. Health 18 (16), 8472. doi:10.3390/ijerph18168472
Moya, T. A., van den Dobbelsteen, A., Ottelé, M., and Bluyssen, P. M. (2019). A Review of green Systems within the Indoor Environment. Indoor Built Environ. 28 (3), 298–309. doi:10.1177/1420326x18783042
Nassar, A. K., Alan Blackburn, G., and Duncan Whyatt, J. (2014). Developing the Desert: The Pace and Process of Urban Growth in Dubai. Comput. Environ. Urban Syst. 45, 50–62. doi:10.1016/j.compenvurbsys.2014.02.005
Nelson, M., and Wolverton, B. C. (2011). Plants+soil/wetland Microbes: Food Crop Systems that Also Clean Air and Water. Adv. Space Res. 47 (4), 582–590. doi:10.1016/j.asr.2010.10.007
Nitmetawong, T., Boonvisut, S., Kallawicha, K., and Chao, H. J. (2020). Effect of Indoor Environmental Quality on Building-Related Symptoms Among the Residents of Apartment-type Buildings in Bangkok Area. Hum. Ecol. Risk Assess. Int. J. 26 (10), 2663–2677. doi:10.1080/10807039.2019.1676636
Pamonpol, K., Areerob, T., and Prueksakorn, K. (2020). Indoor Air Quality Improvement by Simple Ventilated Practice and Sansevieria Trifasciata. Atmosphere 11 (3), 271. doi:10.3390/atmos11030271
Park, S., Kim, M. G., Yoo, M. H., Oh, M. M., and Son, K. C. (2010). Comparison of Indoor CO2 Removal Capability of Five Foliage Plants by Photosynthesis. Hortic. Sci. Technol. 28 (5), 864–870. Available at: https://www.koreascience.or.kr/article/JAKO201018651619348.page.
Pegas, P. N., Alves, C. A., Nunes, T., Bate-Epey, E. F., Evtyugina, M., and Pio, C. A. (2012). Could Houseplants Improve Indoor Air Quality in Schools. J. Toxicol. Environ. Health A. 75 (22-23), 1371–1380. doi:10.1080/15287394.2012.721169
Peng, Z., Deng, W., and Tenorio, R. (2017). Investigation of Indoor Air Quality and the Identification of Influential Factors at Primary Schools in the North of China. Sustainability 9 (7), 1180. doi:10.3390/su9071180
Pennisi, S. V., and van Iersel, M. W. (2012). Quantification of Carbon Assimilation of Plants in Simulated and In Situ Interiorscapes. Horts 47 (4), 468–476. doi:10.21273/hortsci.47.4.468
Pichlhofer, A., Sesto, E., Hollands, J., and Korjenic, A. (2021). Health-related Benefits of Different Indoor Plant Species in a School Setting. Sustainability 13 (17), 9566. doi:10.3390/su13179566
Rodgers, K., Handy, R., and Hutzel, W. (2013). Indoor Air Quality (IAQ) Improvements Using Biofiltration in a Highly Efficient Residential home. J. Green Build. 8 (1), 22–27. doi:10.3992/jgb.8.1.22
Schlacht, I. L., Foing, B., Bannova, O., Blok, F., Mangeot, A., Nebergall, K., et al. (2016). “Space Analog Survey: Review of Existing and New Proposal of Space Habitats with Earth Applications,” in 46th International Conference on Environmental Systems, Vienna, July 10–14, 2016 (ICES).
Settimo, G., Manigrasso, M., and Avino, P. (2020). Indoor Air Quality: A Focus on the European Legislation and State-Of-The-Art Research in Italy. Atmosphere 11 (4), 370. doi:10.3390/atmos11040370
Son, D., Kim, K. J., Jeong, N. R., Yun, H. G., Han, S. W., Kim, J., et al. (2019). The Impact of the Morphological Characteristics of Leaves on Particulate Matter Removal Efficiency of Plants. J. People Plants Environ. 22 (6), 551–561. doi:10.11628/ksppe.2019.22.6.551
Stevenson, P. C., Belmain, S. R., and Isman, M. B. (Editors) (2020). Pesticidal Plants: From Smallholder Use to Commercialisation (United Kingdom: MDPI).
Su, Y.-M. (2014). “CO2 Purify Effect on Improvement of Indoor Air Quality (IAQ) through Indoor Vertical Greening,” in Transactions on Engineering Technologies (Dordrecht: Springer), 569–580. doi:10.1007/978-94-017-8832-8_41
Suárez-Cáceres, G. P., and Pérez-Urrestarazu, L. (2021). Removal of Volatile Organic Compounds by Means of a Felt-Based Living wall Using Different Plant Species. Sustainability 13 (11), 6393. doi:10.3390/su13116393
Teiri, H., Pourzamani, H., and Hajizadeh, Y. (2018). Phytoremediation of VOCs from Indoor Air by Ornamental Potted Plants: A Pilot Study Using a palm Species under the Controlled Environment. Chemosphere 197, 375–381. doi:10.1016/j.chemosphere.2018.01.078
Turner, W. J. N., Logue, J. M., and Wray, C. P. (2013). A Combined Energy and IAQ Assessment of the Potential Value of Commissioning Residential Mechanical Ventilation Systems. Building Environ. 60, 194–201. doi:10.1016/j.buildenv.2012.10.016
UAE (2018). Air Quality index: Sustainable Environment and Infrastructure. Available at: https://u.ae/en/information-and-services/environment-and-energy/improving-air-quality (Accessed July 12, 2021).
Weyens, N., Thijs, S., Popek, R., Witters, N., Przybysz, A., Espenshade, J., et al. (2015). The Role of Plant-Microbe Interactions and Their Exploitation for Phytoremediation of Air Pollutants. Int. J. Mol. Sci. 16 (10), 25576–25604. doi:10.3390/ijms161025576
Wolverton, B. C., and Nelson, M. (2020). Using Plants and Soil Microbes to Purify Indoor Air: Lessons from NASA and Biosphere 2 Experiments. Field Actions Sci. Rep. 21 (1), 54–59. Available at: https://www.koreascience.or.kr/article/JAKO201018651619348.page.
Zhao, L., Liu, J., and Ren, J. (2018). Impact of Various Ventilation Modes on IAQ and Energy Consumption in Chinese Dwellings: First Long-Term Monitoring Study in Tianjin, China. Building Environ. 143, 99–106. doi:10.1016/j.buildenv.2018.06.057
Zheng, Q., Lee, D., Lee, S., Tai Kim, J., and Kim, S. (2011). A Health Performance Evaluation Model of Apartment Building Indoor Air Quality. Indoor Built Environ. 20 (1), 26–35. doi:10.1177/1420326x10393719
Zheng, X., Dai, T., and Tang, M. (2020). An Experimental Study of Vertical Greenery Systems for Window Shading for Energy Saving in Summer. J. Clean. Prod. 259, 120708. doi:10.1016/j.jclepro.2020.120708
Zhou, J., Qin, F., Su, J., Liao, J. W., and Xu, H. L. (2011). Purification of Formaldehyde-Polluted Air by Indoor Plants of Araceae, Agavaceae and Liliaceae. J. Food Agric. Environ. 9 (3&4), 1012–1018. Available at: https://www.koreascience.or.kr/article/JAKO201018651619348.page.
Keywords: air-purifying plant, Pachira aquatica, Ficus benjamina, Aglaonema commutatum, VOCs, formaldehyde (CH2O)
Citation: Al Qassimi N and Jung C (2022) Impact of Air-Purifying Plants on the Reduction of Volatile Organic Compounds in the Indoor Hot Desert Climate. Front. Built Environ. 7:803516. doi: 10.3389/fbuil.2021.803516
Received: 09 November 2021; Accepted: 20 December 2021;
Published: 13 January 2022.
Edited by:
Timothy O. Olawumi, Edinburgh Napier University, United KingdomReviewed by:
Mohamed F. Yassin, Kuwait Institute for Scientific Research, KuwaitGraziano Salvalai, Politecnico di Milano, Italy
Copyright © 2022 Al Qassimi and Jung. This is an open-access article distributed under the terms of the Creative Commons Attribution License (CC BY). The use, distribution or reproduction in other forums is permitted, provided the original author(s) and the copyright owner(s) are credited and that the original publication in this journal is cited, in accordance with accepted academic practice. No use, distribution or reproduction is permitted which does not comply with these terms.
*Correspondence: Chuloh Jung, Yy5qdW5nQGFqbWFuLmFjLmFl