- Zero Carbon Lab, School of Creative Arts, University of Hertfordshire, Hatfield, United Kingdom
Numerous local authorities are committed to constructing buildings to net-zero carbon emissions performance, and have declared carbon emergency, striving to reach carbon neutrality well before 2050. However, buildings in the UK are currently being designed and constructed to current building regulations which do not require net-zero performance, and these buildings will last well beyond 2050. This paper presents a case study of a housing development in Hertfordshire, UK, where a structured approach for achieving net-zero carbon performance homes was developed. The methodology was based on dynamic simulation modelling to design buildings which achieve net-zero operational emissions, and an industry standard inventory of carbon and energy database was used to evaluate embodied emissions in building materials. The approach comprised of developing dynamic simulation models to investigate the improvement in energy performance of the development through fabric-first approach, focusing on building envelope design prior to introducing renewable energy systems, in order to achieve operational net-zero carbon performance. Carbon emissions (operational and embodied) were investigated to assess the appropriateness of the deployed strategies. Dynamic simulation results combined with embodied emissions analysis illustrated that, by combining embodied and operational emissions, a net-zero carbon performance would be achievable by the 2050 target only if alternative building materials based on photosynthetic bio-composites are used. This analysis also highlighted the limitations of conventional retrofit interventions carried out 10 years after the construction as they resulted in increased embodied carbon emissions, thus lengthening the time period well beyond the 2050 target for achieving net-zero carbon performance. As the use of conventional materials appeared to delay the achievement of net-zero emissions by several decades, the only way to achieve net-zero targets before 2050 is to design new buildings to be carbon negative from the operational point of view and to use photosynthetic materials for their construction.
Introduction
Background
The current regulations for UK housing construction do not require the new buildings to be designed and built to net-zero emissions.
Therefore, we are in a challenging situation where new buildings, which will last well beyond 2050, are being designed and built to far lower standards than net-zero. As 80% of the 2050 building stock already exists (UKGBC, 2018), we believe that retrofit to net-zero is also urgently required. The £5k government grant (BEIS, 2021) for thermal insulation upgrades of existing buildings falls well below the insulation requirements for net-zero.
Thus, an urgent matter for policy change at local and central government planning guidance levels is needed to introduce a requirement for net-zero design of new buildings and for net-zero retrofit of existing buildings in order to help the UK achieve zero emissions targets by 2050. Some initiatives are starting to emerge, including the RIBA’s climate change plan (RIBA, 2019) or the UK Architects Declare Climate and Biodiversity Emergency (Architects Declare, 2019) to encourage the profession and wider construction industry to achieve net zero whole life carbon for all new and retrofitted buildings by 2030. However, as these plans are in initial stages and will need time to be implemented across the building industry, we are still far from achieving the expected results as set out through fourth and fifth carbon budgets (EAC, 2021) using conventional aproaches. Therefore, a structured and science-led approach is required to take the carbon emissions under control in the built environment sector.
The UK building stock is one of the oldest in Europe and accounts for nearly 40% of the nation’s total carbon emissions (UKGBC, 2018). To limit rising carbon emissions, the UK parliament, enshrined in law is a revised carbon reduction target to achieve “at least” 100% reduction emissions by 2050, in comparison to pre-1990 levels (BEIS, 2019a). This legislation was supported with the introduction of various schemes, such as the Green Homes Grants (GHGs), Home Upgrade Grants (HUGs) etc. to promote the uptake of energy efficiency solutions. Additionally, amendments were also introduced to the building regulations requiring all new developments to be constructed at minimum to the specified standards such as those in building regulation Part L and “Passivhaus Standards.” However, as we will see from the research introduced in this paper, these measures are not sufficient to achieve net zero performance when both embodied and operational carbon emissions are combined.
Thus, a specific objective of this work is to investigate the requirements for achieving net zero emissions from new buildings by 2050. As carbon emissions from newly constructed buildings occur as result of emissions embodied in building materials and emissions arising from the operation of the building, the main research question is:
how to bring UK housing projects in line with net zero emissions targets, taking into account the combined embodied and operational emissions?
The Need for a Structured Approach
There have been many barriers to achieving zero carbon buildings, net zero energy buildings, or nearly zero-energy buildings. Most of the previous work has focused on case studies of specific projects or specific aspects of building performance, rather than on fundamental principles and integration of these principles into holistic designs. Thus, a study on “Energy Performance Assessment of a 2nd-Generation Vacuum Double Glazing” (Kim and Ru-Da Lee, 2017) found that careful considerations are needed when implementing such glazing in climates with distinct summer and winter seasons. A case study on “Optimizing Energy Efficiency in Operating Built Environment Assets through Building Information Modeling” (Petri et al., 2017) was conducted in order to establish if BIM can be utilised to address operational building energy efficiency. The study found that specialised training is required to use BIM for ongoing applications in operation building energy efficiency. A study on “Delivering Zero Carbon Buildings: The Role of Innovative Business Models” (Zhao and Pan, 2015) addressed the financial barrier in achieving zero carbon buildings, as they are more expensive to construct. The study found that a structured business model, combined with integrated design and performance based design-build was able to address the financial, technological and social barriers on achieving zero carbon buildings. A study entitled “Opportunities for financing sustainable development using complementary local currencies” (Jankovic, 2019a). The study found that sustainable development could be achieved through a circular economy driven by the value of solar radiation falling on roofs of a housing association. A study on “From solar building design to Net Zero Energy Buildings: performance insights of an office building” (Aelenei and Gonçalves, 2014) looks into a sustainable framework for sharing methodology for net zero energy buildings applied to a Portuguese office building. The study found that the application of passive solar principles in an office building considerably outperforms standard office buildings in Portugal and leads to achievement of easily achieved zero energy performance. A study on “Design strategies and energy performance of a net-zero energy house based on natural philosophy” (Shi et al., 2020). It focuses on a design of a net-zero energy house designed and built for the 2018 Solar Decathlon Competition in Dezhou, China. The study combined natural living spaces, natural materials, and natural family relationships in the design concept and demonstrated the achievement of net zero design with a significant renewable energy surplus during the competition in Dezhou. A study “Towards zero-energy buildings and neighbourhoods—A combination of energy-efficiency and local renewable energy production” (Wall, 2017). The study raises the issue of the role or urban planning in achieving active and passive solar strategies in buildings and urban structures. It raises the importance of joint work between urban planners, researchers, designers, citizens and energy companies in achieving net zero energy neighbourhoods.
Despite the body of previous work, there appears to be a gap in the holistic “how to” studies in the field of zero carbon buildings, net zero energy buildings, or nearly zero-energy buildings. That gap was overcome by the structured approach introduced in “Designing Zero Carbon Buildings Using Dynamic Simulation Methods” (Jankovic, 2017), giving a wider context to this study.
Research undertaken by the Environment Audit Committee (EAC) (EAC, 2021) indicates that the schemes and amendments introduced have fallen short to deliver low/net-zero carbon performance solutions—either through financial aid or meeting set targets—this could impact significantly on the achievement of the successive carbon budgets and in turn the net-zero carbon emissions target.
Research by BEIS suggests (BEIS, 2020) that sixteen million homes, two-thirds of the total English homes, fall below the Energy Performance Rating (EPC) of “D” or worse (BEIS, 2019b). In other words, these homes consume more than two times the energy and result in twice as much emission as homes of EPC rating of “A” or above. Furthermore, research also indicates (EAC, 2021) that the cost of retrofitting homes to EPC ratings of “C” to low “B” is estimated between £17,000 to £24,000. These costs are significantly higher than those estimated by the Climate Change Committee (CCC) and Department of Business, Energy and Industrial Strategy (BEIS). Additionally, financial aid available through home improvement grants (GHGs and HUGs) fall significantly short of the estimated costs. Thus, the retrofit process (Jankovic, 2019b) appears to be cost intensive, deterring homeowners and tenants from undergoing the retrofit process.
This indicates the need for a structured approach towards developing and delivering net-zero carbon performance solution, to meet the 2050 target specified by the UK government.
Case Study
The focus of this research is to investigates how UK housing projects can be brought in line with net-zero targets. The choice of the Case Study was based on the specific grant funding received for this project and on the availability of a scheme approved for construction by the Planning Department of the collaborating local authority in Hertfordshire. The scope was limited to one scheme only based on available funding. Thus, a housing development with complete planning permissions was identified in England and selected as a candidate for investigation of requirements for requirements of bringing UK housing projects in line with net-zero targets.
The development is comprised of six homes of which two homes are semi-terrace and four homes are mid-terrace. It has a total footprint of 330 m2 and each home has an area of 94 m2. The typology of the development is six three-bedroom homes spread over two storeys (ground and first floor), with North–South orientation. The construction technology of the building was assumed to be of conventional concrete blockwork and brick masonry, with adequate amount of insulation in the cavity and air-tightness levels to meet minimum current UK building regulations Part L specifications, Table 1. These assumptions were made by the research team to provide a generic starting point for the investigation and analysis representing the vast number of homes in the UK designed and built to meet the specified Part L Building Regulations.
New houses, or new dwellings as they are called in the UK Building Regulations, need to comply with Part L of the Regulations specified in Approved Document L1A (HM Government, 2016). The compliance is achieved by comparing the new dwelling with a “Notional Building” that has the same shape and size as the actual dwelling, and with specified heat loss coefficients through external walls, floors, roof, glazing and doors, with specified airtightness, with specified linear thermal transmittance for thermal bridges, and by using natural ventilation. This Notional Building specification is used to determine Target Emissions Rate (TER) and Target Fabric Energy Efficiency (TFEE). Subsequently, Dwelling Emissions Rate (DER) and Dwelling Fabric Energy Efficiency (DFEE) are calculated using accredited software programs. Compliance with the Regulations is achieved by demonstrating that the DER and DFEE rate are not greater than the TER and TFEE rate. Buildings can achieve net zero operational performance only if, in addition to being energy efficient, they are fitted with renewable energy systems. As no renewable energy systems are specified for the Notional Building, compliance with Building Regulations does not require renewable energy in new buildings, and therefore Building Regulations compliance does not lead to net zero operational performance.
This study uses building performance simulation, which is the only way to explain and evaluate the complex ways of interaction between the building, its occupants and the climate. The simulation is conducted over every hour of the simulation year, namely 8760 hours, using weather data for the particular location, and slicing the simulation timestep to as low as 10-min intervals whilst dealing with significant complexity of heat and energy transfer in and around the building. As building performance simulation is a universal method based on the first principles, there is an implicit information regarding the generalizability, reliability, and versatility of the study to be applicable to the other context and/or countries.
The solar geometry used in the simulation was for the location of London Luton, Latitude 51.88°N, Longitude 0.37°W. The weather data was for London Kew example weather year (Kew.fwt), sourced within the IESVE simulation software (IES, 2021) weather data library as a combination of individual years from the UK MET Office.
Originality of the Present Study
Climate Change Committee (CCC) defines net-zero as the 100% reduction in operational emissions (CCC, 2019). However, this research characterizes net-zero carbon as the 100% reduction in combined embodied and operational emissions of a building, thus bringing total cumulative emissions to zero. The majority of studies into net zero buildings and net zero houses only consider operational emissions as a criterion for achieving net zero. None of the current studies combine emissions embodied from building materials and emissions from building use as criteria for achieving net zero emissions. The findings of this study show that taking embodied emissions into account delays reaching net zero by several decades.
Methods
Dynamic Simulation Modelling
To investigate the energy performance of the housing development used as a case study, dynamic simulation models were developed, using an industry standard tool from Integrated Environment Solutions called Virtual Environment IES-VE (IES, 2021).
Model geometry of the building was developed in IES-VE 2021 and building geometry and construction details were specified in the model, Figures 1A,B. Building heating systems details, such as the use of air source heat-pumps, was also specified in the model, to replicate the intent of the housing development stakeholders.
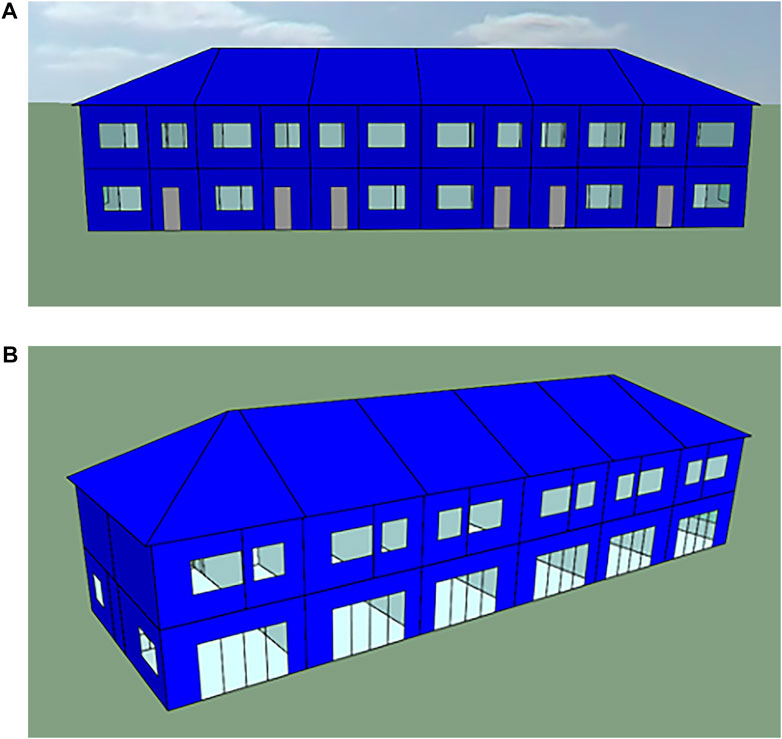
FIGURE 1. IES–VE model of the housing development (A) North façade (front) of the building (B) South façade (rear) of the building.
Once the model was developed to satisfactory detail, analysis of the building was undertaken to assess the baseline performance. This was the starting point for the development, termed as Case A—Built to planning approval. Several iterations of the model were developed, investigating the energy performance in response to strategic treatment of the building. This was achieved by following a fabric-first approach, to improve the performance of the building using passive measures and then employing use of active low-carbon measures such as heat-pumps, PV modules etc.
The energy efficiency improvement process of the building was split into four stages; 1) Building fabric improvement, 2) Building systems improvement, 3) Renewable energy strategy, and 4) Internal thermal comfort analysis.
To maximise energy efficiency through passive measures, building fabric was selected as the first point of focus. Through iterations of the Case A model, energy efficiency of the building fabric was improved to meet the zero-carbon performance U-values, Table 1.
This model was further utilised to improve the building systems of the housing development. Building systems such as lighting and domestic hot water (DHW) were specified as systems defaults using fluorescent lights and gas heated water with system default sizing. Space heating systems were changed from system default gas boilers to represent air-source heat pumps to match closely with the aim of the developers. Lighting systems were improved to represent LED lighting and DHW consumption was adjusted to reflect a rate of 5L/person/hr therefore 120 L/person/day, apportioned across wet rooms only (bathroom/kitchen/toilet).
Once satisfactory reductions in carbon emissions and energy demand were obtained from improving building systems, it was necessary to improve the energy strategy for the housing development so that its energy demand would be met using renewable sources. This was accomplished using Photovoltaic (PV) modules, which were added to meet the energy demand of the building. As shown in Figure 2, a total of 88 panels were added utilising an area of 132 m2. Furthermore, energy demand for supplying DHW was met by adding vertical solar thermal panels on the south façade of the building, Figure 2.
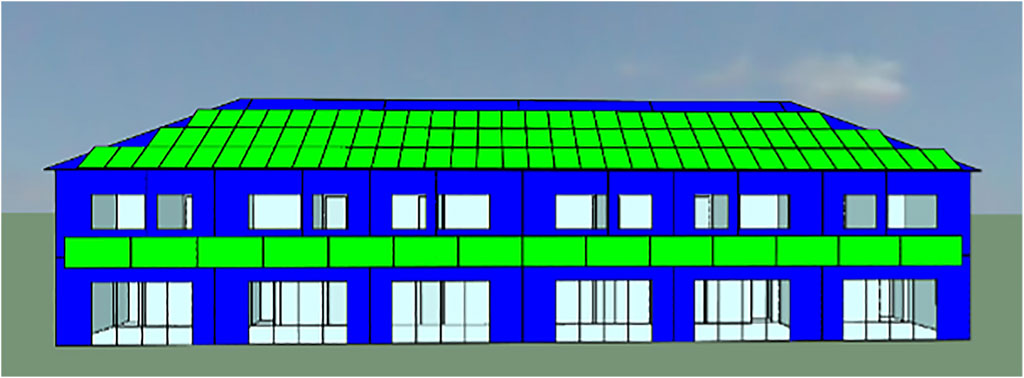
FIGURE 2. Renewable energy sources: photovoltaic solar panels placed on the roof and solar thermal panels placed vertically on the south facing wall.
Once an operational zero carbon performance model was achieved, it was termed as Case B—Zero carbon performance model. This served as the second performance reference point for the housing development. This was later used for developing a cumulative carbon analysis for the housing development, as discussed in the next section.
Cumulative Carbon Analysis
In order to assess the environmental impact of the building, a Lifecycle Carbon Assessment (LCA) approach was utilised, to quantify the carbon footprint of the building until 2050, in line with the UK government target of achieving net-zero performance.
This was done through the use of embodied carbon emissions values from Inventory of Carbon Emissions (Hammond and Jones, 2011) for the various materials utilised in the construction process of the building.
To investigate the environmental impact and carbon footprint of the building, four key scenarios were investigated; Case A—Built to planning approval, Case B—Zero carbon performance, Case C—Zero carbon retrofit of Case A in 10 years, and Case B.1—Net-zero carbon design using hemp-lime construction. This was done in order to draw parallels between the different approaches for the construction of the building.
For each scenario, total embodied carbon emissions of the building were calculated, and a cumulative total was established by adding annual operational emissions, which were in fact slightly negative, to the embodied emissions. This was done in order to identify the time period required to achieve net-zero carbon performance in each case. Observations and results from this step are discussed in the main results section of this paper.
It is worth noting that the simulation models in this analysis could not be calibrated and validated, as they were based on non-existing buildings with no opportunity to get actual performance data for calibration. As previous research shows (Jankovic, 2019c), the calibration of simulation models is possible in the case of existing buildings where data from energy performance or instrumental monitoring is available. In the absence of that possibility, comparison between uncalibrated models gives accurate relative comparison, even though the assessment of absolute performance could lack accuracy.
Main Results
Preliminary analysis of the building (Case A—As built to planning approval) revealed significant areas for improvement, in order to achieve a zero carbon performance as in Case B—Zero carbon performance, as shown in Table 2.
Results from the dynamic simulation illustrate significant improvement in the energy efficiency of the building as it was able to achieve zero carbon emissions in its operations as in Case B, shown Table 3. This was achieved through a careful combination of the fabric first approach along with renewable energy strategies as mentioned in Case B, Table 2.

TABLE 3. Energy performance in Case A—designed to planning approval and Case B—designed to zero carbon performance.
Cumulative carbon emissions analysis of the building revealed key insights into the ability of the building to achieve a true net-zero carbon performance status. The research found that, of the four scenarios investigated, only two scenarios, Case B and Case B.1, were able to achieve a true net-zero carbon performance, before the end of this century. Out of these two cases, only one scenario, Case B.1, was able to achieve net-zero carbon performance before the target date of 2050, Figure 3. In comparison, Case A could not to achieve a net-zero carbon performance, and Case C achieved net-zero emissions well beyond the 2050 target.
This analysis provides evidence to support that embodied emissions can delay the achievement of net-zero emissions by 3 decades.
Discussion
This paper has presented a case study where net-zero carbon performance was achieved through 100% reduction of operational carbon emissions, and gradual reduction of embodied emissions based on negative operational emissions over several decades. To achieve this, the research utilised a three-step approach; 1) Be Lean: Use of less Energy—achieved through careful implementation of passive design strategies, 2) Be Clean: Supply energy efficiently—achieved through careful selection and implementation of active measures such as lighting, HVAC systems etc., and 3) Be Green: Use of renewable energy systems—achieved through careful use of appropriate renewable energy technologies such as PV panels, solar hot water systems etc.
To achieve a net-zero carbon performance target, it was crucial for a building to first achieve operational zero carbon performance. After numerous simulation runs, with net-zero operational carbon emissions set as a target and improving the thermal insulation envelope between each simulation run, this was achieved by the set of parameters shown in Table 2, combined with renewable energy systems as shown in the same table. The U-value targets in Tables 1, 2 were chosen to surpass standards introduced by Passivhaus Institute (Passivhaus Institut, 2015).
Research suggests (CCC, 2019) that the present definition of net-zero carbon performance does not include embodied carbon emissions and focuses solely on reduction of operational carbon emissions. This is not adequate for achieving zero emissions target by 2050, as significant emissions would not be accounted for.
The impact of embodied carbon emissions on cumulative carbon emissions, operational and embodied, is plotted for the four scenarios: Case A, Case B, Case B.1 and Case C in Figure 3. These scenarios are discussed in more detail in the remainder of this paper.
Of the four scenarios explored in this paper, only one scenario—Case B.1—Net-zero carbon design using hemp-lime construction—presented promising results for achieving net-zero carbon before the target date of 2050 set by the Climate Change Act. This was achieved through the use of natural materials—in this case hemp-lime bio-composite. This material is able to provide significantly low embodied carbon values through carbon sequestration in the plant material during its growth, with negative emissions of −108 kgCO2/m3 (Bevan and Woolley, 2008). Our research found that the use of hemp-lime bio-composite resulted in much lower initial embodied carbon emissions starting at 82.64 kgCO2/m2 and was hence able to achieve net-zero carbon performance by 2043 with negative emissions of −1.49 kgCO2/m2, 7 years before 2050.
In comparison, Case B—Zero carbon performance model built from conventional materials, was also able to achieve negative emissions of −3.33 kgCO2/m2 in 2065, 15 years past the 2050 target. In Case A, we observed that due to the energy inefficiency nature of the building fabric leading to high energy consumption and therefore high operational carbon emissions compounding each year, it required significant improvements to the building fabric to achieve a net-zero carbon performance.
We wanted to investigate what would have happened happen if Case A had been retrofitted to a zero-carbon performance level. This scenario is explored in Case C—Case A retrofit in 10 years i.e., 2031, where we observe a spike in the cumulative carbon emissions of the building–from 535.85 to 587.8 kgCO2/m2, arising from embodied emissions in retrofitted materials.
After the retrofit of the building to operational zero carbon performance, cumulative carbon emission value starts reducing each year. However, despite achieving operational zero carbon performance in 2031, achieving net-zero carbon performance was not possible by 2050. This was because the cumulative carbon emissions compounded up to the year 2031, set a higher starting point for achieving a net-zero carbon performance. Hence, operational reductions in carbon emissions were not adequate to offset the high starting point. Thereby the building was on track to achieving net-zero carbon performance in the next century, which is significantly later than the target year.
In parallel with cumulative carbon emissions, we wanted to explore the cost implications of the net-zero carbon retrofit process. We used a Lifecycle Cost Analysis approach for the building to investigate the trade-offs between building to net-zero carbon emissions and retrofitting at a later stage. To develop this, the costs of building materials utilised were obtained through the open market for each material and inflation was assumed to be at 3%. We found that the most cost-effective approach towards developing net-zero carbon buildings was through Case B and Case B.1 as illustrated in Figure 4. Furthermore, the results also illustrated that if conventional retrofit approach is followed, such as that in Case C, the total project cost would be very high, as shown in Table 4. Why is cost-analysis of multiple cases of the building development explored in this research? A comparative cost analysis for the housing development was carried out to identify an optimum cost-effective approach for construction of net-zero carbon performance buildings, thereby making them a more affordable solution for developers and homeowners. Thus, enabling the built-environment sector to meet the goal of becoming completely net-zero carbon by the target date of 2050. What targets can be promoted by a fabric first approach and how might these differ at various scale of construction projects? Through the analysis of the housing development, this research believes that some targets, such as achieving target zero carbon performance building fabric U-values and air-tightness levels are key and feasible to achieve under the fabric-first approach. This research believes that the resulting figures for cumulative carbon emissions per sq. meter of floor area can be utilised as indicative targets to achieve for domestic buildings at various scales within the United Kingdom.
How do these results compare between the four different cases and with other performance criteria? This is summarised in Table 5. Also, as it can be seen from Figure 3, embodied emissions can easily ad over 3 decades worth of operational emission reductions in net zero buildings. This means that organisations declaring carbon emergency and aspiring to reach net zero by 2030 could already be about 2 decades late.
As it can be seen from this table, in terms of operational energy, UK Building Regulations (UK Part L 2021) require energy performance of 120 kWh/m2.yr or less. The Pssivhaus standard requires less than 60 kWh/m2.yr and RIBA 2030 requires less than 30 kWh/m2.yr. Case A, is close to Part L 2021, and cases B and B1 exceed this performance by significant amount. Case C starts the same as Case A and after the retrofit in 10 years it is aligned with Cases B and B1.
In terms of operational emissions, RIBA 2030 requires net zero operational emissions, whilst Case A is high above that. Cases B and B.1 exceed RIBA 2030 requirements, performing at negative emissions. Case C starts with the same emissions as Case A in 20201 and it aligns with Cases B and B.1 after the retrofit in 10 years.
The starting embodied emissions in all cases are lower than the RIBA 2030 requirement of less than 625 kgCO2e/m2.yr. However, in Case C after the retrofit in 2031 the embodied emissions increase to 899.79 kgCO2e/m2.yr, well above the RIBA 2030 threshold. Due to positive operational emissions in Case A, net zero is never reached. In cases B and B.1 net zero is reached in 2065 and 2043, respectively. In Case C, net zero is not reached even well beyond 2100.
At the 60 years mark defined by the RICS as the extent of the building lifetime, Case A will have accumulated nearly 4000 kgCO2e/m2.yr and Case C nearly 600 kgCO2e/m2.yr, whereas Cases B and B.1 will have accumulated negative emissions of −108 kgCO2e/m2.yr and −246 kgCO2e/m2.yr, respectively.
As these results show, the design that fulfils the objective of reaching net zero by 2050 is Case B.1, designed for negative operational emissions and constructed with hemp-lime bio-composite material.
As it can be seen from Table 5, the UK Building Regulations are totally inadequate for achieving carbon neutrality and an urgent action is required.
It is worth pointing out why the embodied emissions depend so much on the choice of materials used in construction. For instance, 1 m3 of brick will have embodied emissions of around 357 kgCO2/m3 depending on the brick type and concrete will have embodied emissions of around 3507 kgCO2/m3 depending on the concrete type (Jones and Hammond, 2019). However, hemp-lime bio-composite, also called hempcrete, will have negative embodied emissions of −108 kgCO2/m3 due to the sequestration of carbon in the plant material from which it originates during the plant growth. When using hemp-lime for construction, not all building materials will come with negative embodied carbon. Such as roof tiles, internal finishes etc., to mention just a few. Nevertheless, this reduces the starting embodied emissions on day one, and it is easier to get to net zero sooner by negative operational emissions.
What other barriers could impact the achievement and development of net-zero carbon performance buildings? This research recognises that factors such as user-behaviour, building systems design and performance, skills-gap and the design of the building impact highly on the performance of the building. To alleviate such issues, the research team believes that it is crucial that there is an early collaboration between the design team and building physics analysis team and with other suppliers and manufacturers, thereby empowering built-environment professionals with the knowledge to achieve net-zero carbon performance buildings. The research team has undertaken post-occupancy monitoring and analysis of several homes across the UK such as the Zero Carbon House in Birmingham and believes that building performance can be optimised by developing a strong understanding of the user-behaviour through post-occupancy monitoring and providing the user a strong understanding of how to optimally utilise the building to meet their operational and thermal comfort needs.
Another barrier to better carbon emissions performance is the increased cost of low carbon solutions. As it can be seen from the last row of Table 4, net zero design with conventional materials increases the construction cost by 35%. Retrofit in 10 years to zero carbon is 65% more expensive, and construction with hemp-lime material is 41% more expensive. Figure 4 shows that between 2030 and 235 the total construction and operation costs in Cases B and B1 are lower than the total costs in Cases A and C. This creates a number of opportunities for experimentation. For instance, new ownership models could be developed that align the interests of building developers and building owners through self-build initiatives. Research into new photosynthetic materials, such as naturally grown materials like straw, natural cellulose, and various recycled materials, could lead to nuanced differences and alternatives to conventional construction materials.
What can different stakeholders, including Quantity Surveyors and other professionals, take from this research? In order to bring climate change under control, it is a responsibility of all professions involved in building design and construction to become familiar with the consequences of embodied emissions arising from construction materials. Ignoring these consequences can lead to a point of no return as far as climate change is concerned. Various professional bodies need to include these findings into their continuing professional development courses and make such courses mandatory for a license to practice their respective professions.
What are the sectoral and policy challenges associated with delivering building of this nature in practice, including design team perceptions? Delivering hemp-lime buildings is not new and useful practical lessons have been learnt, as reported by Jankovic (2016). That research demonstrates a significant performance gap between design simulations and instrumental monitoring results of hemp-lime buildings. This is because hemp-lime material contributes to significant moisture buffering through its primary plant-based capillary pores, and its secondary capillary structures created by lime-based bonding of shredded hemp plant. This is not readily available in design simulation tools, but can be overcome by post-processing of simulation results using a Fourier filtering method (Jankovic, 2016). The main lessons for the construction sector and policy makers are to become more reliant on science, and that hemp-lime buildings cannot only be designed by architects, but involvement of building physicists is absolutely essential in the design team. Other matters that need careful consideration are the upskilling of construction workers as found by Eberlin and Jankovic (2014) on the basis of post occupancy evaluation of 40 houses in the UK built from this material.
What might be the recommendations around energy strategy and operations? What are the implications and opportunities of scale of construction? The work by Jankovic (2016) identified a potential for significant investment savings on the HVAC (heating, ventilating, an air-conditioning) plant. This was tested in a Hemcrete Museum Store constructed for the British Science Museum, with a two-thirds reduction on the investment cost on the HVAC plant (Leskard, 2020). Whilst the increased scale of construction offers opportunities for prefabricated cassettes of this material to be delivered and installed on site, in-situ casting of hemp-lime leads to prolonged drying times of the building immediately after the construction. An implication for the design team is to implement a mechanical ventilation system that can operate with full fresh air intake during cold nights when moisture content is lower than that in the building, and thus achieve rapid drying of the building prior to the occupation.
What policies and incentives could be suggested to local and central planning guidance levels? The research recognises that some local councils in the UK have issued mandate for all developments, to improve their performance beyond Part L standards, in form of a supplementary planning guidance document (SPG) such as those issued by the Greater London Authority (GLA). This research shows that a similar type of policy change, outlining the requirements to meet net-zero carbon levels through use of low-embodied materials such as hemp-lime bio composite as well as use of the three step hierarchy strategy discussed earlier in this paper, would empower the industry professionals with holistic guidance for design and development of new net-zero carbon performance buildings and develop net-zero carbon retrofit solutions.
Are there any wider benefits to society? As hemp-lime material is not yet widely used, opportunities exist developing new skilled construction personnel. As the material is fire resistant, its application to high-rise apartment blocks could lead to safer buildings. In this particular context, there are gaps, as well as opportunities. There is an opportunity for new research and development into application of this material as retrofitted insulation on tall facades, with 3D printers redeveloped for vertical 2D printing along the facade. As hemp-lime is not a load-bearing material, there are opportunities for further research into the application of natural fibres to improve its structural properties. However, the most significant societal benefit is the ability of this material to help with bringing climate change under control if used more widely.
Whilst the strength of this study is in highlighting the importance of embodied emissions as a contributor to the overall emissions in newly constructed buildings, a weakness of this study is that it is based on a single case of a cluster of six family homes in Hertfordshire, United Kingdom. However, in order to find this case, the research team had to secure funding for this work, establish a working relationship with a local borough council, and then to find a suitable project that had received a planning approval to be constructed. With more funding and time, more suitable cases could have been found and analysed. However, on balance, we believe that this weakness has been outweighed by the strength of highlighting the scale of efforts needed in the UK and beyond to achieve net zero by 2050.
Conclusion
This study used an example of a housing development in Hertfordshire, which was approved for construction by the Planning Department of the collaborating local authority. As the current Building Regulations in the UK do not require new houses to be built to net-zero standards, and as the local authority in question declared carbon emergency and their aim to become carbon neutral by 2030, the study investigated how this could be achieved by alternative designs in comparison with the design that was approved for planning.
Building performance simulation was used as the basis for designing zero carbon operational performance based on the methods developed by Jankovic (2017), and analysis of embodied emissions was based on the Inventory of Carbon and Energy database (Jones and Hammond, 2019).
The study demonstrates that achieving net-zero carbon design by 2050 is possible, but only through design for operational negative emissions and the use of natural photosynthetic materials such as hemp-lime bio-composite. Through cost analysis, this work also illustrates that using natural materials with very low or negative embodied carbon emissions results in lower cumulative carbon emissions and therefore can provide an easier pathway towards achieving net-zero carbon performance buildings to be achieved sooner despite higher initial costs in comparison to conventional solutions, which have shown to prolong the achievement of net-zero carbon performance.
The use of conventional materials appeared to delay the achievement of net-zero emissions by several decades, either in construction of new or in retrofit of existing buildings. One of the most important conclusions is therefore that while the UK Building Regulations are not yet adequate for achieving carbon neutrality and do not completely restrict the use of fossil fuels, the only way to achieve net-zero targets before 2050 in new buildings is to design them to be carbon negative from the operational point of view and to use photosynthetic materials with negative embodied emissions for their construction.
Future studies will focus on salability of the presented solution, and on accelerating the adoption of net zero designs by developing preset designs for specific building archetypes.
Data Availability Statement
The original contributions presented in the study are included in the article/Supplementary Material, further inquiries can be directed to the corresponding author.
Author Contributions
Conceptualization, LJ and SC; methodology, LJ and PB; investigation, LJ, PB, and SC; writing—original draft preparation, PB, LJ and SC; writing—review and editing, LJ and SC.
Funding
This study was funded by a grant from Research England via QR Strategic Priorities Fund administered by the University of Hertfordshire.
Conflict of Interest
The authors declare that the research was conducted in the absence of any commercial or financial relationships that could be construed as a potential conflict of interest.
Publisher’s Note
All claims expressed in this article are solely those of the authors and do not necessarily represent those of their affiliated organizations, or those of the publisher, the editors and the reviewers. Any product that may be evaluated in this article, or claim that may be made by its manufacturer, is not guaranteed or endorsed by the publisher.
Acknowledgments
The authors gratefully acknowledge the collaboration with, and the help and support from the Welwyn Hatfield Borough Council through the provision of information relevant for this project.
References
Aelenei, L., and Gonçalves, H. (2014). From Solar Building Design to Net Zero Energy Buildings: Performance Insights of an Office Building. Energ. Proced. 48, 1236–1243. doi:10.1016/j.egypro.2014.02.140
Architects Declare (2019). UK Architects Declare Climate and Biodiversity Emergency [WWW Document]. UK Archit. Declare Clim. Biodivers. Emerg. URL Available at: https://www.architectsdeclare.com/ (accessed 5.6.21).
BEIS (2019b). Energy Efficiency: Building towards Net Zero - Business, Energy and Industrial Strategy Committee - House of Commons [WWW Document]. URL Available at: https://publications.parliament.uk/pa/cm201719/cmselect/cmbeis/1730/173004.htm (accessed 5.6.21).
BEIS (2020). Httpswwwgovukgovernmentpublicationsenergy-White-Pap.-Powering-Our-Net-Zero-Future. URL Available at: https://www.gov.uk/government/publications/energy-white-paper-powering-our-net-zero-future (accessed 5.6.21). Energy white paper: Powering our net zero future [WWW Document]
BEIS (2019a). The Climate Change Act 2008 (2050 Target Amendment) Order 2019 [WWW Document]. URL Available at: https://www.legislation.gov.uk/ukdsi/2019/9780111187654 (accessed 5.6.21).
Bevan, R., and Woolley, T. (2008). Hemp Lime Construction: A Guide to Building with Hemp Lime Composites. 1st ed. Watford, United Kingdom: BRE Press.
Eberlin, C., and Jankovic, L. (2014). “Exploring the Energy Performance of Hemcrete in Affordable Housing and Future Implications for Carbon Reduction in the Housing Sector,” in Proceedings of Zero Carbon Buildings Today and in the Future.
Jankovic, L. (2019c). Improving Building Energy Efficiency through Measurement of Building Physics Properties Using Dynamic Heating Tests. Energies 12, 1450. doi:10.3390/en12081450
Jankovic, L. (2017). Designing Zero Carbon Buildings Using Dynamic Simulation Methods. 2nd ed. London and New York: Routledge.
Jankovic, L. (2019b). Lessons Learnt from Design, Off-Site Construction and Performance Analysis of Deep Energy Retrofit of Residential Buildings. Energy and Buildings 186, 319–338. doi:10.1016/j.enbuild.2019.01.011
Jankovic, L. (2019a). Opportunities for Financing Sustainable Development Using Complementary Local Currencies. IOP Conf. Ser. Earth Environ. Sci. 297, 012023. doi:10.1088/1755-1315/297/1/012023
Jankovic, L. (2016). Reducing Simulation Performance Gap in Hemp-Lime Buildings Using Fourier Filtering †. Sustainability 8, 864. doi:10.3390/su8090864
Jones, C., and Hammond, G. P. (2019). Embodied Carbon Footprint Database [WWW Document]. Embodied Carbon Database. URL Available at: https://circularecology.com/embodied-carbon-footprint-database.html (accessed 10.1.21).
Kim, S.-C., Yoon, J.-H., and Lee, R.-D. (2017). Energy Performance Assessment of a 2nd-Generation Vacuum Double Glazing Depending on Vacuum Layer Position and Building Type in South Korea. Energies 10, 1240. doi:10.3390/en10081240
Leskard, M. (2020). A Sustainable Storage Solution for the Science Museum Group. Sci. Mus. Group J. 4. doi:10.15180/150405
Passivhaus Institut, 2015. Passivhaus Institut [WWW Document]. URL Available at: https://passivehouse.com/02_informations/02_passive-house-requirements/02_passive-house-requirements.htm (accessed 5.7.21).
Petri, I., Kubicki, S., Rezgui, Y., Guerriero, A., and Li, H. (2017). Optimizing Energy Efficiency in Operating Built Environment Assets through Building Information Modeling: A Case Study. Energies 10, 1167. doi:10.3390/en10081167
RIBA (2019). RIBA 2030 Climate Challenge [WWW Document]. 2030 Clim. Chall. URL Available at: https://www.architecture.com/about/policy/climate-action/2030-climate-challenge (accessed 5.6.21).
Shi, F., Wang, S., Huang, J., and Hong, X. (2020). Design Strategies and Energy Performance of a Net-Zero Energy House Based on Natural Philosophy. J. Asian Architecture Building Eng. 19, 1–15. doi:10.1080/13467581.2019.1696206
UKGBC (2018). Climate Change UKGBC’s Vision for a Sustainable Built Environment Is One that Mitigates and Adapts to Climate Change. [WWW Document]. UKGBC - UK Green Build. Counc. URL Available at: https://www.ukgbc.org/climate-change/(accessed 5.6.21).
Wall, M. (2017). Towards Zero-Energy Buildings and Neighbourhoods - A Combination of Energy-Efficiency and Local Renewable Energy Production. Indoor Built Environ. 26, 1313–1318. doi:10.1177/1420326X17739713
Keywords: embodied emissions, operational emissions, cumulative emissions, dynamic simulation models, net zero
Citation: Jankovic L, Bharadwaj P and Carta S (2021) How can UK Housing Projects be Brought in Line With Net-Zero Carbon Emission Targets?. Front. Built Environ. 7:754733. doi: 10.3389/fbuil.2021.754733
Received: 06 August 2021; Accepted: 03 November 2021;
Published: 29 November 2021.
Edited by:
Tao Liu, Peking University, ChinaReviewed by:
Timothy O. Adekunle, University of Hartford, United StatesDonagh Horgan, University of Strathclyde, United Kingdom
Copyright © 2021 Jankovic, Bharadwaj and Carta. This is an open-access article distributed under the terms of the Creative Commons Attribution License (CC BY). The use, distribution or reproduction in other forums is permitted, provided the original author(s) and the copyright owner(s) are credited and that the original publication in this journal is cited, in accordance with accepted academic practice. No use, distribution or reproduction is permitted which does not comply with these terms.
*Correspondence: Ljubomir Jankovic, TC5KYW5rb3ZpY0BoZXJ0cy5hYy51aw==