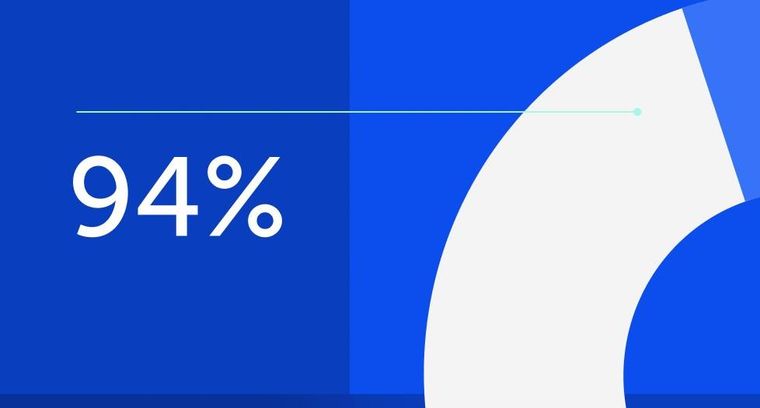
94% of researchers rate our articles as excellent or good
Learn more about the work of our research integrity team to safeguard the quality of each article we publish.
Find out more
REVIEW article
Front. Built Environ., 16 November 2021
Sec. Construction Management
Volume 7 - 2021 | https://doi.org/10.3389/fbuil.2021.671408
This article is part of the Research TopicReviews for Advanced Construction ManagementView all 5 articles
This research explores the current state of Construction 4.0 and discusses a four-layer implementation of Construction 4.0 in the industry. The research methodology consists of an extensive literature review to gain insights about Construction 4.0 and frame the four-layer implementation plan. A case study is also presented to showcase the proposed implementation plan. Nine Construction 4.0 technologies were discussed, their integration throughout the project lifecycle was presented in a roadmap, their integration and connectivity with one another were outlined in an interaction roadmap, and the requirements necessary for achieving the 4.0 transformation were articulated. However, the proposed implementation plan is focused on nine Construction 4.0 technologies. The research presents a comprehensive plan for integrating Construction 4.0 technologies into the industry and serves as a guideline to help construction companies better understand the implications of Construction 4.0.
The construction industry sits at a crossroads. It is economically vital to the prosperity of nations, and a key player that affects our everyday lives. Yet, the construction industry lags behind other major industries in its adoption of technological advances (Osunsanmi et al., 2018). Nassereddine et al. (2020a) noted that the complex nature of the construction industry and its heavy reliance of information require the adoption of new and emerging technologies. Challenges facing the construction industry span a multitude of reasons including the daunting decline in productivity, shortage in the workforce, low levels of research and development (R&D), and the inefficient and insufficient transfer of knowledge from project to project (Sawhney et al., 2020; El Jazzar and Hamzeh, 2015; Eljazzar et al., 2013). These challenges add more pressure on construction to move from an industry that has resisted emerging technology to one that is embracing it. One industry that has been a source of innovation in construction is manufacturing (Koskela, 1992). From the many practices that construction has adopted from manufacturing, this research focuses on the concepts of Industry 4.0.
The term Industry 4.0 was first coined by the German Federal Government in 2011 to highlight the fourth industrial revolution (Osunsanmi et al., 2018) as part of the High-Tech Strategy 2020 outlined by the German government to promote the digitization of manufacturing in response to the Asian industrial competition (BMBF-Internetredaktion, 2016; Klinc and Turk, 2019). Since the emergence of Industry 4.0, researchers and practitioners have tended to issue their own definitions (I-SCOOP EU, 2021). For instance, according to MacDougall (2014), Industry 4.0 can be defined as “a new technological age for manufacturing that uses cyber-physical systems and Internet of Things, Data and Services to connect production technologies with smart production processes.” Hermann et al. (2016) defined Industry 4.0 as “a collective term for technologies and concepts of value chain organization.” Schuh et al. (2016) pointed out that Industry 4.0 is the “real-time, intelligent, horizontal, and vertical networking of people, machines, objects and ICT systems for a dynamic management of complex systems.” Hofmann and Rüsch (2017) noted that Industry 4.0 is the “shift in the manufacturing logic towards an increasingly decentralized, self-regulating approach of value creation, enabled by concepts and technologies such as cyber physical systems (CPS), Internet of things (IoT), Internet of Services (IoS), cloud computing, or additive manufacturing and smart factories.” Others such as BCG (2018) and Klinc and Turk (2019) reflected on the historical evolution from Industry 1.0 to Industry 3.0 and defined Industry 4.0 as “the fourth industrial revolution which affects every manufacturing domain and comprises advanced manufacturing technologies that capture, optimize, and deploy data.” More recently, Derigent et al. (2020) stated that Industry 4.0 is the “real-time, high data volume, multilateral communication and interconnectedness between cyber-physical systems and people.” IBM (2021) argued that Industry 4.0 is “the realization of the digital transformation of the field, delivering real-time decision making, enhanced productivity, flexibility and agility.”
The implementation of Industry 4.0 is said to revolutionize manufacturing. Montgomery and Norman (Construction Products Association, 2016) noted that manufacturing has passed through three different revolutions before reaching Industry 4.0, namely, mechanization, electrification, and digitalization. Schwab (2017) explained that Industry 4.0 is the stage that enables the full integration between people and digitally controlled machines with the help of internet and information technology (IT). Lu (2017) added that industry 4.0 supports the growth and evolution of various fields and industries. The automotive industry has greatly benefited from Industry 4.0, where manufactured cars are being 40% controlled by electronics (Manohar, 2016). The health sector has also taken full advantage of Industry 4.0 in creating new diagnostic methods and technologies to sequence genes (Thuemmler and Bai, 2017).
The development of Industry 4.0 has been enabled by various key components. First, Internet of Things (IoT) is considered the backbone or the core of Industry 4.0. IoT connects different physical objects together and enables them to communicate. Next, there is the Cyber-Physical System (CPS) which is a mechanism that is controlled by computer algorithms to integrate the cyber world and the physical dynamic world. The concept of Digital Twin is associated with Cyber-Physical integration. In addition to CPS, Cyber-Physical Production System (CPPS) is another component of Industry 4.0 that provides data anywhere and anytime, enabling a strong connection between the cyber world and the physical world and its ongoing processes (Vogel-Heuser and Hess, 2016). Another component of Industry 4.0 is Internet of Service (IoS) that establishes networks to integrate all of the manufacturing stages together (Kagermann et al., 2013). Researchers have also discussed Internet of People (IoP) as a new paradigm that perceives people as an active component of the internet rather than being considered as end-users only (Conti et al., 2017).
The industry is said to transform the lifecycle process of products and production systems by increasing the connectivity and interaction among parts, machines, and humans (Manohar, 2016). This transformation is enabled and driven by nine fundamental technological advances, also referred to as pillars: autonomous robots, Augmented Reality, Simulations, the cloud, Big Data and analytics, the Industrial Internet of Things, cybersecurity, additive manufacturing, and horizontal and vertical system integration (Klinc and Turk, 2019). It should be noted that the technology itself is not powering Industry 4.0: while most of the nine pillars are not entirely new to manufacturing, it is the full integration of these building blocks and their connectivity across the borders that define Industry 4.0 (Sawhney et al., 2020).
The construction industry has also experienced a radical transformation and made great strides in changing its status quo and embrace technological advancements (Bou Hatoum and Nassereddine, 2020; Nassereddine et al., 2019; El Jazzar et al., 2020). Influenced by the gains that resulted from the fourth industrial revolution, researchers in construction began investigating the potential of integrating Industry 4.0 into construction. While construction is often compared to manufacturing, the former is approaching and embracing Industry 4.0 from a different direction (Klinc and Turk, 2019). Recently, the term “Construction 4.0” emerged in the 21st century construction research corpus (Rastogi, 2017). The interest in Construction 4.0 is fueled by the development of various technologies, the drastic change in the needs of owners, the shift toward mass customization, and the need for green construction and sustainability (Rastogi, 2017).
Researchers noted that there are several elements necessary to implementing Construction 4.0 in the construction industry (Sawhney et al., 2020). Building on existing work in the area of Construction 4.0 and interactions the authors have had with construction organizations in a previous effort that used questionnaires and workshops to solicit input of construction practitioners (Goger et al., 2017), four main questions emerged: What technologies are included in Construction 4.0? Where are these technologies used? Do the technologies integrate with one another? What requirements should construction organizations consider when implementing Construction 4.0? To answer the above questions, this article proposes a four-layer implementation plan (Figure 1). The first layer introduces Construction 4.0 and nine of its most commonly cited technologies, namely: integrated Building Information Modeling (iBIM), augmented realty (AR), virtual reality (VR), robotics, 3D printing, artificial intelligence (AI), drones, Internet of Things (IoT), and Big Data. The second and third layers highlight the two-level integration effort of the Construction 4.0 approach. The second layer outlines the integration of a Construction 4.0 technology throughout the construction project lifecycle. A roadmap for the integration of Construction 4.0 technologies across the project lifecycle is created. The third layer explores the integration and connectivity of Construction 4.0 technologies. An interaction map of Construction 4.0 is developed. The fourth layer of the implementation plan discusses the requirements needed for achieving Construction 4.0. Each layer is discussed using information gathered from the literature review and the consulting work of the authors. In addition to introducing and discussing the four-layer implementation plan, a case study is presented to highlight how Construction 4.0 can be realized by showcasing the four layers put forth in the article.
The research methodology employed to achieve the research objective consists of questionnaires, a literature review, and a case study.
In a first step, the authors conducted questionnaires and workshops to gain the view of the AEC industry about adopting to Construction 4.0 (Goger et al., 2017). In the questionnaires a total of 144 stakeholder of the AEC industry answered questions about the opportunities and risks of employing Construction 4.0 in the individual phases of building projects. These questions also covered the necessary changes in processes and responsibilities, as well as the required framework conditions. In a second step, 32 AEC companies participated in two workshops to refine the results of the questionnaires. The output of the workshop led to the formulation of the four questions mentioned earlier. Companies were also asked to identify the technologies they perceive to have an impact on their operations. Nine technologies were captured: iBIM, AR, VR, robotics, 3D printing, AI, drones, IoT, and Big Data. Additionally, companies provided a discussion of how these technologies can be integrated into their processes. To provide more context to the four questions articulated by the companies, and to verify the results of the questionnaires and workshops, a literature review was performed next.
Among the various technologies discussed under the umbrella of Construction 4.0, the following nine technologies are presented in this article: iBIM, AR, VR, robotics, 3D printing, AI, drones, IoT, and Big Data. The identification of the nine technologies was based on a bibliometric analysis of articles between 2010 and 2021 that the authors performed in a separate study to identify the most cited Construction 4.0 technologies. The nine technologies identified from the literature review aligned with the technologies outlined in the workshops. This first step covers the first layer of the proposed Construction 4.0 implementation plan. Once the nine technologies were selected and defined, a thorough literature review was conducted to extract relevant articles that fit the second and third layers of the implementation plan (lifecycle and interaction). Google Scholar was used to identify articles. The search filters included various keywords to tackle the two layers. Keywords such as “(Construction 4.0 AND 3D printing)” were used to find articles that can be used to discuss the use of a certain technology in different phases of the construction lifecycle. Keywords that include two technologies at a time such as “(AI AND Drones)” were used to identify articles the touched on the interactions between Construction 4.0 technologies. The search results were restricted to English and included articles, journal papers, dissertations, and conference proceedings published between 2017 and 2021. The total number of papers first identified was 240 papers, and after manual filtering, 100 articles were retained, 47 of which contributed to the interaction matrix. The manual selection focused on papers that served the research objectives. The authors chose to select papers that discussed both the application of a technology throughout the construction project and its interaction with other technologies.
To illustrate the use of the four-layer implementation plan, a case study is discussed. In this real case study, the authors worked together with FCP, one of the major consulting engineering offices in Austria, to review the applicability of the proposed implementation plan. The on-site inspection of the Heating, Ventilation, and Air Conditioning (HVAC) system was used to showcase the use of Construction 4.0 technologies that acted as a cross-linked system.
Given the novelty of Construction 4.0, and prior to discussing the four-layer implementation plan, it is important to present an overview of the evolution of this concept in the construction industry. Forcael et al. (2020) provided a historical overview of the development of the concept of Construction 4.0 which can be traced back to 2014. Lasi et al. (2014) were the first to discuss the concepts of Industry 4.0 and construction together. A year later, Li and Shi (2015) introduced the term “Dam construction 4.0” that aims to leverage the concept of Industry 4.0 to “digitize and intellectualize” dam construction. The authors limited their Construction 4.0 discussion to dams and did not provide a broader view of the concept. Finally, the term “Construction 4.0” was first coined in a Roland Berger’s report and was defined as the “digitization of the construction industry” (Schober, 2016).
Similar to Industry 4.0, Boton et al. (2020) and Forcael et al. (2020) performed a comprehensive literature overview of Construction 4.0 in the body of knowledge and noted that there is no consensus on a single definition of Construction 4.0. For instance, Construction 4.0 was referred to as a “way of finding a coherent complementarity between the main technological approaches to best meet the current major challenges of the industry” (Boton and Forgues, 2017). Rastogi (2017) stated that the main goal of construction 4.0 is to create a digital construction site that monitors progress throughout the lifecycle of a project by using different technologies. The European Industry Construction Federation (FIEC) explained that Construction 4.0 is the counterpart of Industry 4.0 in the Architecture, Engineering and Construction (AEC) industry and it refers to the digitalization of the construction industry (Sommer, 2018; García de Soto et al., 2019). Others defined Construction 4.0 as a simple instantiation of Industry 4.0 in construction characterized by “the use of ubiquitous technologies for real-time decision making” (Schober, 2016; Craveiro et al., 2019; Forgues, 2019). Construction 4.0 was also thought of as an “encompassing variable approaches and a set enabling technologies” such as the Internet of Things (IoT), Big Data, and artificial intelligence (Oesterreich and Teuteberg, 2016; Klinc and Turk, 2019). While the definition of Construction 4.0 varies, there is agreement that the adoption of Construction 4.0 will not only change the construction process, but also change the organization and project structures, shifting the fragmented construction industry into an integrated industry (García de Soto et al., 2019).
The authors of this article define Construction 4.0 as the digitization and industrialization of the industry that 1) enable real-time, horizontal, and vertical integration and connectivity of stakeholders across the construction project lifecycle, 2) promote the advancement of construction processes by employing mechanization and automation, and 3) bridge the gap between the physical and cyber environments.
To understand the various layers of Construction 4.0, it is important to first understand the technologies that are enabling this transformation. While the existing research corpus discusses various Construction 4.0 technologies, this article focuses on nine Construction 4.0 technologies that have been frequently cited. A brief introduction to each of these technologies is provided below.
Integrated Building Information Modeling (iBIM) is considered the higher level of traditional BIM and consists of three elements: 1) the integration architecture that defines major layers of iBIM and how they are interconnected, 2) the product model that defines the content and function of the object’s behavior, and 3) the process model that identifies the interaction scheme and mechanism between model objects (Abdalla and Eltayeb, 2018).
Augmented reality (AR) is both an information aggregator and a data publishing platform that allows the user to 1) passively view displayed information, 2) actively engage and interact with published content, and 3) collaborate with others in real time from remote locations (Nassereddine et al., 2019; Schranz et al., 2020).
Virtual reality (VR) is a step further than AR on the spectrum of virtuality. VR creates a virtual and immersive experience for the user through headsets with 360-degree visions, allowing the user to experience a completely different environment.
Robotics uses machines that can perform or replicate human actions. While robotics has been widely used in manufacturing and aerospace, construction is following suit and is using robotics, mainly in the vertical construction sector (Liu, 2017).
3D printing, also known as additive manufacturing, is the process of creating a complex, physical 3D object from a CAD model. 3D printing has undergone 25 years of research and development, and as a result, the technology is currently used in different industries such as aerospace, automobile, and medical (Chua and Leong, 2014). The construction industry is also exploring the use of 3D printing, mainly for small and medium-sized applications at the time being (Rouhana et al., 2014).
Artificial intelligence (AI) is a term used to describe a machine that replicates the human cognitive functions (Rao, 2019). One of the main components of AI is machine learning, where a machine learns from a set of data using statistical methods.
Drones, also known as Unmanned Aerial Vehicles (UAVs), are unpiloted small-sized aircrafts that are remotely controlled. In early 2006, drones were mainly used for military applications (Nisser and Westin, 2006). In recent years, their use in construction and other industries has been on the rise (Irizarry and Costa, 2016).
According to the International Telecommunication Union (ITU, 2012), IoT is “a global infrastructure for the information society enabling advanced services by interconnecting (physical and virtual) things based on, existing and evolving, interoperable information and communication technologies.” The European Research Cluster on the Internet of Things (IERC, 2012) stated that IoT is “a dynamic global network infrastructure with self-configuring capabilities based on standard and interoperable communication protocols where physical and virtual things have identities, physical attributes, and virtual personality and use intelligent interfaces, and are seamlessly integrated into the information.” Ibrahim et al. (2021) highlighted the importance of IoT in driving the adoption of construction 4.0. Their work outlined how IoT can be leveraged to enhance site monitoring, machine control, construction safety, fleet management, and project management.
Finally, Big Data can be defined as the ability to process large amounts of data and to extract useful insights from data (Bilal et al., 2016). Five attributes are used to describe Big Data, namely: volume, variety velocity, value, and veracity. Bou Hatoum et al. (2020) argued that Big Data is integral to the construction industry and showcased that the proper adoption of Big Data is possible throughout the entire lifecycle of a construction project. The authors also highlighted the perceived benefits such as enabling stakeholder-driven analysis, enhancing decision-making, increasing transparency and information exchange, and enhancing project performance (Bou Hatoum et al., 2020).
The first level of the Construction 4.0 integration efforts takes a lifecycle view for the use of Construction 4.0 technologies. A project moved from its early planning phase, to design, construction, and then facility management. A technology is used to its full potential when it is integrated throughout the construction project lifecycle, where applicable (Eadie et al., 2013).
The use of iBIM throughout the construction project lifecycle has been discussed by various researchers. For instance, Remmen et al. (2015) introduced and tested an iBIM framework driven my simulations to efficiency design building energy. Jrade and Lessard (2015) proposed an iBIM time and cost management system (ITCMS) that acts as an Earned Value Management platform used during preconstruction planning and construction. Alizadehsalehi et al. (2018) highlighted the synergistic use of iBIM and drones to manage construction site safety. Kazado et al. (2019) presented a software tool to integrate building sensor data with iBIM to enable the visualization and analysis of historical and real-time facility management data.
AR is gaining increased momentum in the construction industry, and various use-cases are being explored and tested. Nassereddine (2019), Schranz et al. (2020) and Urban et al. (2019) listed different use-cases of AR throughout the construction project lifecycle. Several authors (Arashpour and Aranda-Mena, 2017; Calderon-Hernandez and Brioso, 2018; Schranz et al. (2020)) discussed the applications of AR during design and planning. Nassereddine, et al. (2020a) mapped 23 use-cases of AR during the construction phase of the project. Moreover, Lin et al. (2019) developed a real-time 4D AR system to monitor the progress of modular construction. Palmarini et al. (2018) reviewed current use-cases of AR during operation and maintenance of industrial projects. Additionally, Chen et al. (2020) showcased a BIM-AR application for the inspection and maintenance of fire equipment in buildings.
Similar to AR, the use of VR during planning, design, and construction has been also highlighted (Haggard, 2017). Li et al. (2018) categorized the use of VR in construction into the hazard identification, which allows construction teams to sense, analyze, and extract potential dangers. Moreover, it can be used for safety training and education, in which construction workers will train in a safe environment in comparison with on-site training, which might be expensive and hazardous.
Robotics has gained increased momentum in the construction industry, especially during the construction phase. This technology is heavily used in construction assembly work, especially for high rise buildings. SMART system developed by SHIMIZU in Japan was used to construct more than 30 stories of an office building (Amediya, 2016). In addition, robotics can be used for different construction tasks such as painting, brick overlaying, and earthwork can be performed by robots (Bogue, 2018). In addition, Elhouar et al. (2019) discussed the use of robotics during construction and highlighted the potential use of robotics in later phases of the lifecycle.
3D printing is another technology that has been showing great potential. Rael and San Fratello (2018) discussed the importance of 3D printing in advancing the construction industry. The authors listed a series of 3D printing techniques and materials used during planning, design, and construction. While this technology continues to gain momentum in the construction industry, a number of challenges such as layering effect that results in uneven surfaces with voids, tensile strength, and issues associated with the lack of steel reinforcement need to be overcome before the industry embraces this technology (Tay et al., 2017).
Most recently, AI has been a hot topic for construction academicians and practitioners for its diverse use in the industry. According to a study by McKinsey and Company, AI is starting to gain momentum in the construction industry (Blanco et al., 2020). The study highlighted three main current AI applications: 1) project scheduling optimization that is achieved by continuously testing a large number of plan alternatives and selecting the better option, 2) image recognition and classification that can be used to identify issues related to safety on site and to collect the information for future learning, 3) enhanced analytic platforms that collect and analyze building machine data and building sensor data to predict any issues related to maintenance. Węglarz (2018) showcased the use of AI during design to enhance energy efficiency and improve sustainability. Several authors (Bigham et al., 2018; Mohan and Varghese, 2019) presented AI applications to improve construction site risk assessment and workers’ safety during the construction phase. Moreover, Chen et al. (2018) presented a framework to the automatic scheduling of maintenance work orders to improve facility management. The framework was established based on BIM, facility management software, and AI to analyze data and predict areas of concern.
The use of drones during design, construction, and facility management was explored by Li and Liu (2019). Fleming et al. (2016) showcased the use of drones for surveying at the Transbay Transit Centre project. Akinade (2020) also highlighted the use of drone aerial surveying in the mining industry. Other researchers investigated use-cases of drones during construction (Goessens et al., 2018). Moreover, Irizarry and Costa (2016) presented various applications of using drones for project monitoring during construction on four different projects. Additional work also explored the use of drones for inspection and monitoring during surveying, construction, and facility management (Zaychenko et al., 2018). Furthermore, Fernandez Galarreta et al. (2015) highlighted the use of drones for facility management, specifically damage assessment.
Finally, IoT and Big Data play a central role in driving Construction 4.0. Forcael et al. (2020) showcased these two technologies are strongly correlated and drive the other Construction 4.0 technologies and are, thus, essential to ensure information flows between the different technologies. Sawhney et al. (2020) highlighted how integrating IoT and Big Data connects the digital virtual to the physical world, enabling better service and enhancing decision-making and predictions. Stone (2021) also elaborated on how IoT and Big Data can improve construction productivity by automating time-consuming manual processes, enhancing the monitoring of machinery and equipment, and ultimately, reducing maintenance costs by enabling preventive maintenance.
An overview of the current and expected use of these nine Construction 4.0 technologies is presented in Figure 2. The green circles reflect that a technology’s use-cases have been tested and used on construction projects and the yellow circles represent the potential benefits of additional use-cases of a technology that are being explored.
The second level of the integration efforts demands an increased connectivity and interaction of Construction 4.0 technologies (Rüßmann et al., 2015). Aleksandrova et al. (2019) noted that the full integration of digital technologies is a radical transformation in the construction that creates a united digital ecosystem.
A scan of the literature on Construction 4.0 and its associated technologies was performed to identify current and future potential interactions. A map was then developed (Figure 3) to outline those interactions and illustrate potential synergistic efforts.
iBIM and AI can be thought of as core technologies of Construction 4.0 and the impetus to connectivity (Aleksandrova et al., 2019). Copper (2018) added that BIM and cloud-based common data environment (CDE) are central to Construction 4.0 framework: BIM carries the simulation feature that is a core component for Industry 4.0, and CDE acts as the data warehouse for all information related to construction project over its lifecycle. BIM and CDE establish a single platform that helps integrate all of the construction project phases and link the physical and cyberspace (Sawhney et al., 2020), thus allowing the implementation of Construction 4.0. Additionally, Banihashemi et al. (2015) studied the possible interaction between BIM and AI to improve the energy performance of buildings. The research introduced a conceptual framework to transform passive BIM into an active one by utilizing the capabilities of AI. The framework focused on the design phase that is considered critical for ensuring building sustainability as decisions related to construction materials and methods are made during this phase.
Researchers have also discussed the various ways in which BIM and 3D printing are changing the landscape of the construction industry (Johnson and K. Collins, 2017). The authors examined how BIM and 3D printing can be used simultaneously to enhance the visualization of important aspects of the project, assisting the construction team and the various stakeholders in making better informed decisions. The study also noted that printed BIM models can be used to showcase construction methods and highlight areas of concerns for maintenance and demolition.
Moreover, the potential synergies between BIM and robotics have been gaining increased interest in construction. Park et al. (2016), for instance, developed a self-governing mobile robot system for construction applications. The system included a robot navigation system, control units, motion sensors, and an Ultra-Wideband platform (UWB) integrated with a BIM path planner. The system utilized a BIM model as a navigation site map, resulting in the effective selection of the best path for robots. This selection is executed in real time and is especially effective in a complex indoor construction site. The positioning capabilities of the system were improved through testing various navigation algorithms and corrections methods. The findings of this research can be also applied to other applications such as material delivery, inspection, and site security.
The synergies between 3D printing and robotics in construction were discussed by Elhouar et al. (2019) who listed the benefits of using 3D printing and robotics to automate various construction tasks, resulting in lowered safety risks, better control of schedule, and reduced cost of construction.
Some of the interactions outlined in Figure 3 are extracted from the existing construction research corpus, while others are extracted from adjacent industries such as manufacturing and are interactions yet to come to construction. For example, interactions between drones and AR/VR were documented by Kim et al. (2018) who analyzed 28 use-cases of drones and AR/VR from literature within the entertainment industry. The study highlighted multiple applications such as integrating AR objects with live feed from drone, employing AR for safety training and inspection, combining aerial drone surveying with AR/MR simulations, and providing real-time virtual tour using a camera mounted on drones. Thermistocleous et al. (2015) noted many of the applications of AR and drones in other industries can be applied to the construction industry, especially applications related to surveying and inspection.
Embracing Construction 4.0 entails several challenges that have been discussed by construction researchers. Industries are normally averse to change, and, therefore, it is initially resisted until it can be perceived as a guaranteed opportunity. Several authors (Oesterreich and Teuteberg, 2016; Cooper, 2018; Dallasega et al., 2018) noted that the construction industry is no stranger to the resistance of change.
Researchers pointed out that the multidisciplinary nature and uniqueness of the construction industry results in an unclear understanding of the value proposition of Construction 4.0 technologies (Sawhney et al., 2020). Building on the work of Goger et al. (2017) which one of the authors was involved in, the discussion presented in this section is based on conversations the authors had with construction organizations on the requirements needed to shift toward Construction 4.0 (Goger et al., 2017).
In the past construction companies often relied on a project-thinking approach when managing construction projects. The shift to Construction 4.0 requires a change of the thinking-approach and the processes. The development of digital technologies and their adaption to the construction company’s needs is highly dynamic, tailorable, and expensive. Additionally, when new technologies are introduced, employees and workers will need extra training. Therefore, construction companies will generate a surplus value only if the new technologies are integrated within the company’s processes and can be used across projects. The switch to Construction 4.0 requires a process-oriented mindset, as opposed to the traditional project-oriented approach. However, this mindset shift pushes construction companies to digitize their existing processes, posing an additional challenge for two reasons: 1) the existing process has mostly been designed before current digital tools were available, and 2) not every process can be directly digitized. Therefore, all the existing processes have to be reengineered to accommodate the shift in the mindset and support the 4.0 transformation of construction companies.
Figure 4 shows a general process to implement new digital technologies in a construction company, as required for the transition to Construction 4.0. Prior to rethinking a process, the actual process must be first recorded and analyzed to explore how and where the technology(ies) could be used. A thorough analysis and documentation of the “status quo” of the existing process is crucial to successfully manage the technological changes. Assessing current processes helps in identifying the relevant decision-making structures and cost-relevant process steps. The process-oriented approach also forms the basis for the identification of waste and inefficiencies in a company, and thus, becomes a prerequisite for the application of Lean management in a company.
FIGURE 4. Process to implement Construction 4.0 (based on Goger et al. (2017)).
Once the existing process has been investigated, the potential for innovation and integration of technologies, along with efficiency enhancement analysis, is assessed next. This includes an application analysis of the different technologies of interest, as well as the digital tools that are already in use. The structural needs as well as the existing barriers to innovation have to be identified. The structural needs include the aforementioned aspects of implementing the various Construction 4.0 technologies in construction companies. Other considerations for companies to think of are the high investments associated with adopting technologies, the need to additional human resources, and R&D investments, all of which poses a major financial barrier.
For a successful transition to Construction 4.0, a construction company needs a vision of the digitization change process that fits the company’s needs. This is especially important since Construction 4.0 goes beyond technology alone. It includes a change in the mindset of all people involved (from field personnel to management) as well as of the processes in consideration. Hence, a clear vision, which is supported by these people, is important. Only when these requirements are met, companies can make the transition from the traditional project-thinking to process-thinking.
This shift also changes the organization and infrastructure of many companies, i.e., their partners. Another consideration to address is the long-standing problem of longitudinal fragmentation that renders stakeholders nearly powerless to pilot their companies through this change (Fergusson and Teicholz, 1996). Over the past decade, the construction industry has made significant changes to its structure as organizational changes have already been applied to companies that implement Lean management. The importance of some traditional departments decreases significantly, whereas some new departments emerge.
In addition to the challenges associated with moving construction toward a process-thinking industry, the lack of global standards and framework for implementation is another roadblock. Furthermore, Construction 4.0 heavily relies on various Information Technology (IT) systems, and, therefore, concerns related to data and cybersecurity must be addressed and stringent security standards must be put forth. Legal and contractual issues need to be also discussed to allocate risks among stakeholders.
To illustrate the four-layer construction 4.0 implementation plan, a case study where Construction 4.0 technologies act as a cross-linked system is presented. For the case study, the authors worked together with FCP, one of the major consulting engineering offices in Austria. The team defined the on-site inspection of the Heating, Ventilation, and Air Conditioning (HVAC) system as the use-case of interest. According to Figure 4, the process of the current HVAC inspection was first documented, as well as the HVAC defects occurring during the HVAC installation on the construction site. Then, the process was analyzed and the potential for efficiency enhancement using Construction 4.0 technologies was assessed. Among the technologies discussed in this article, iBIM and AR were selected (showcasing the use of the first layer of the Construction 4.0 implementation plan) and the existing HVAC inspection process was adapted for integrating these two technologies. The integration of Construction 4.0 in this use-case can bring a benefit throughout the lifecycle of the building—not only in the construction phase, but also in the facility management phase (second layer). A classification system for the most common HVAC defects was established next (Haponiuk, 2020) based on the Industry Foundation Classes (IFC). IFC is a standard for the data exchange in openBIM that enables the interaction between AR and iBIM (third and fourth layers).
A big advantage of cross-linked Construction 4.0 technologies is the closed-loop data transfer. Figure 5 shows a generalized data path for a closed-loop data transfer between iBIM and AR. All begins with a BIM model, which is created in a native modeling environment, like Revit or ArchiCAD. For a general use, the model is exported as an Industry Foundation Classes (IFC) file to a collaborative data environment on a BIM server. This export allows the model to be used in several of the previously discussed Construction 4.0 technologies. After exporting the model to a smartphone or tablet, the site supervisor and/or inspector (referred to hereinafter as a user) can benefit from the visualization and interaction with the model on the smart device when, for example, inspecting a Heating, Ventilation, and Air Conditioning (HVAC) system. QR codes or RFID chips attached to a building component connect the smart device with the manufacturer’s database of the building component. This enables the user to access specific product data, which can be also transferred via BIM Collaboration Format (BCF) to the BIM server and, hence, back into the native BIM model. This two-way communication leads to a closed-loop data transfer. Moreover, the manufacturer data, also referred to as semantic data, can be transferred to an .xml database that is then connected with the component’s Globally Unique Identifier (GUID) in the BIM model. This information can be then retrieved by facility managers. This is especially valuable for maintenance and safety-technical relevant data and leads to component-referenced manufacturer data. An example application of the closed-loop data transfer system is presented next.
FIGURE 5. On-site inspection: closed-loop data transfer between BIM and AR (adapted from Schranz et al. (2020)).
The BIM model normally contains the general specifications for a required fire protection flap. After the installation of the fire protection flap, the specific product data can be transferred into the BIM model. Then, the correct maintenance requirements for this product are available for the facility management. Another option consists of using an IFC transfer with an appropriate Model View Definition (MVD) directly from the BIM model.
This closed-loop data transfer works not only during the construction phase, but also in the later operation and maintenance phase. Each time one or more building components are replaced; the new product data are transferred into the central BIM model. Hence, this closed-loop data transfer will be used throughout the whole lifecycle of a facility.
The other used technology is AR. The authors worked on different use-cases for AR in Construction 4.0 (Gerger et al., 2020; Schranz et al., 2020; Schranz et al., 2021). AR is one of the emerging technologies which have a great potential to transform the construction industry. The aforementioned closed-loop data transfer can be used with several AR use-cases, e.g., for monitoring the progress of the construction site, visualizing augmented drawings or construction systems in the field, as well as conducting on-site inspection. In one of the research projects of the authors, AR-AQ-Bau (Urban and Schranz, 2018), specific AR use-cases for on-site inspection have been developed. In this case, the BIM model was also exported to a BIM server and then an AR model was created using the game-engine Unity. This AR model is then transferred to an AR device (tablet or head-mounted displays). On construction sites head-mounted displays (HMD), like Daqri Smart Glasses or Microsoft Hololens 2, can improve the safety of the users of AR devices compared to the use of handheld devices such as tablets or smartphones. In the latter case the focus of the users would be on the handheld devices. Additionally, HMDs have the advantage that they are used hands-free.
The site supervisor can use the AR model to visualize drawings or construction systems in the field before they are built. This helps to review installation and check, for example, whether there is enough space for the construction or for the construction workers to build these systems. In case of an HVAC system, the site supervisor checks, for instance, if the space for movement in front of the inspection hatch is sufficient. After the HVAC system is installed, the site inspection can monitor and check if everything was built correctly. This process or application works not only for HVAC but also for the whole construction. In the AR HMD device, the site inspection marks every defect or deficiency observed in the executed work. Hence, the position of the defect in the building is stored in the AR model.
In the research project AR-AQ-Bau (Urban and Schranz, 2018) a new AR user interface for HVAC site inspection is developed. This development follows the aforementioned description of Construction 4.0 requirements. The authors and Haponiuk (2020) worked closely with the HVAC department of FCP. The required features and the aforementioned IFC-based defect classification will be implemented into a new AR user interface. The site supervisor can then easily select an existing HVAC defect (which is then connected with the right IFC class) as well as additional information (like inspection time etc.). This information is directly connected with the right part of the BIM model. Additionally, a description (text or audio) of the defect as well as photos can be added.
All this information is then transferred back into the BIM model via BCF. On one hand, this helps to keep a record of all the defects in a building and, on the other hand, improves the repair of the existing defects. Additionally, the AR tool for site inspection is also very invaluable for the facility management during the operation phase. Hence, also this combination of AR and BIM can be used during the whole lifecycle of a building.
Construction 4.0 technologies need several functionalities to be used on the construction site. Therefore, in the aforementioned research project, the authors added another function to AR and BIM which can be used for the site inspection, showcasing various possibilities to leverage the use and integration of the same Construction 4.0 technologies throughout the lifecycle of the project (stressing the practicability of the first and second layers of the Construction 4.0 implementation plan). The AR remote expert system connects remote experts to the field personnel using HMD glasses. Figure 6 shows how the remote expert system works. On the upper left corner, the AR view of the HMD on the construction site is shown. The live video stream from the HMD is transferred to the program running on the computer of the remote expert in the office (green arrow). The remote expert can watch the video stream and place markings, instructions, and pictures (red marking) into the program. These markings, instructions, and pictures are then transferred to the HMD on the construction site (red arrows). Hence, the HMD user can view this additional information. This functionality can be used for all forms of site inspection.
FIGURE 6. Remote expert system: BIM and AR (Reprinted from Schranz et al., 2020).
Another AR use-case connected with BIM through the closed-loop data transfer is the monitoring of the progress of the construction site. In this use-case the site supervisor uses the AR device to mark all building parts that are finished. This information is again transferred back into the BIM model using BCF. This helps to monitor and check the time schedule of the construction phase and recalculate and adjust it if necessary. Additionally, the accountant can use this information in the BIM model to semi-automatically generate the invoices. Therefore, also the accounting process is supported.
The AR device also assists the collection of maintenance and safety-technical relevant data. The scan of QR-codes or RFID becomes easier with AR devices. After scanning the tag, the AR devices can be connected with the manufacturer’s database and can retrieve the specific product data of the built-in component. These data are then transferred to the BIM model or another specific database (as described before).
The fourth wave of technological advancement (Industry 4.0) and the digital transformation at its helm are pushing industries worldwide to embrace newer technologies to continue to remain competitive. Although the construction industry is not leading this change, it is not an exception to the digital revolution. The unique nature of the construction industry provides fertile ground for research on digitizing the industry and the term Construction 4.0. This research builds on the existing construction research corpus and discusses four layers of Construction 4.0 implementation. The first layer consists of the understanding of the technologies associated with Construction 4.0. This research discussed nine frequently cited technologies, namely, iBIM, AR, VR, robotics, 3D printing, AI, drones, IoT, and Big Data. The second and third layers defined the two-level of integration needed to implement Construction 4.0. The second layer presents a roadmap that outlines the integration of each of the nine technologies across the construction project lifecycle. The roadmap is a depiction of the current state-of-practice of the technologies and an outlook into future developments. The third layer offers insights into how the nine technologies can be connected and integrated together. An interaction matrix is developed to outline the relationships between the different technologies. It should be noted that the two-level integration cannot happen across the board, but rather only when applicable. The implementation of these three layers is only enabled when the fourth layer is realized. The fourth layer encompasses a set of requirements that construction companies must consider. At the core of these requirements is the necessary shift to process-thinking. Construction stakeholders must have the right mindset to pilot their companies through the disruptive Construction 4.0 storm. Finally, a case-study was presented to discuss the four-layer implementation plan outlined in the article. The case-study highlighted different Construction 4.0 technologies which are integrated into the construction process, namely, iBIM and AR. It demonstrated how a closed-loop data transfer can connect the different technologies and enable their use over the whole lifecycle of a building. The user collects information, e.g., about work progress or existing defects, on the AR device and transfers this new information to the iBIM model. The iBIM model acts as a central data model. The implementation of these Construction 4.0 technologies needs a change of the existing processes from a project-oriented to a process-oriented approach. Therefore, in the case-study the existing processes were documented, analyzed, and adopted to integrate one or several of the Construction 4.0 technologies.
This article contributes to the Construction 4.0 technologies implementation roadmap by providing industry practitioner an understanding of nine of its technologies, their use throughout the construction project lifecycle, their integration with one another, and a list of requirements construction companies need to consider when going through the Construction 4.0 transformation. As interest in Construction 4.0 continues to grow, more use-cases will be piloted and verified on construction projects to further showcase the implementation of Construction 4.0. Future research efforts can extend on the nine technologies discussed in the article and introduce additional elements of Construction 4.0. Additional research is also needed to verify the interactions between all the Construction 4.0 technologies. While technology is a central aspect of Construction 4.0, it is important to investigate how integrating technology into the industry will change the discipline and skills needed to design, execute, manage, lead, and maintain construction projects.
All authors listed have made a substantial, direct, and intellectual contribution to the work and approved it for publication.
The authors acknowledge the TU Wien Bibliothek for financial support through its Open Access Funding Program.
The authors declare that the research was conducted in the absence of any commercial or financial relationships that could be construed as a potential conflict of interest.
All claims expressed in this article are solely those of the authors and do not necessarily represent those of their affiliated organizations, or those of the publisher, the editors and the reviewers. Any product that may be evaluated in this article, or claim that may be made by its manufacturer, is not guaranteed or endorsed by the publisher.
The Supplementary Material for this article can be found online at: https://www.frontiersin.org/articles/10.3389/fbuil.2021.671408/full#supplementary-material
Abdalla, J. A., and Eltayeb, T. M. (2018). “An Integrated Building Information Model (IBIM) for Design of Reinforced Concrete Structures,” in Proceedings of 17th International Conference on Computing in Civil and Building Engineering (Tampere, Finland). Available at: http://hdl.handle.net/11073/16204.
Akinade, O. O. (2020). Bim-Based Software for Construction Waste Analytics Using Artificial Intelligence Hybrid Models. Bristol, United Kingdom: University of the West England. Dissertation.
Aleksandrova, E., Vinogradova, V., and Tokunova, G. (2019). Integration of Digital Technologies in the Field of Construction in the Russian Federation. Eng. Manag. Prod. Serv. 11 (3), 38–47. doi:10.2478/emj-2019-0019
Alizadehsalehi, S., Yitmen, I., Celik, T., and Arditi, D. (2018). The Effectiveness of an Integrated BIM/UAV Model in Managing Safety on Construction Sites. Int. J. Occup. Saf. Ergon. 26 (4), 829–844. doi:10.1080/10803548.2018.1504487
Arashpour, M., and Aranda-Mena, G. (2017). “Curriculum Renewal in Architecture, Engineering, and Construction Education: Visualizing Building Information Modeling via Augmented Reality,” in Proceedings of 9th International Structural Engineering and Construction Conference, ISEC 2017 (Valencia, Spain). doi:10.14455/isec.res.2017.54
Banihashemi, S., Ding, G., and Wang, J. (2015). “Developing a Framework of Artificial Intelligence Application for Delivering Energy Efficient Buildings through Active BIM,” in Proceedings of COBRA AUBEA 2015 (Sydney, Australia).
BCG (2018). Industry 4.0. BCG. Available at: https://www.bcg.com/capabilities/manufacturing/industry-4.0 (Accessed May 30, 2021).
Bigham, G. F., Adamtey, S., Onsarigo, L., and Jha, N. (2018). “Artificial Intelligence for Construction Safety: Mitigation of the Risk of Fall,” in Intelligent Systems and Applications. Editors K. Arai, S. Kapoor, and R. Bhatia (Springer International Publishing), 1024–1037. doi:10.1007/978-3-030-01057-7_76
Bilal, M., Oyedele, L. O., Qadir, J., Munir, K., Ajayi, S. O., Akinade, O. O., et al. (2016). Big Data in the Construction Industry: A Review of Present Status, Opportunities, and Future Trends. Adv. Eng. Inform. 30, 500–521. doi:10.1016/j.aei.2016.07.001
Blanco, J. L., Fuchs, S., Parsons, M., and Ribeirinho, M. J. (2020). Artificial Intelligence: Construction Technology’s Next Frontier McKinsey & Company. Available at: https://www.mckinsey.com/industries/capital-projects-and-infrastructure/our-insights/artificial-intelligence-construction-technologys-next-frontier.
BMBF-Internetredaktion (2016). Industrie 4.0 - BMBF. Bundesminist. für Bild. Forsch. - BMBF. Available at: https://www.bmbf.de/de/zukunftsprojekt-industrie-4-0-848.html (Accessed May 28, 2021).
Bogue, R. (2018). What Are the Prospects for Robots in the Construction Industry. Ir 45 (1), 1–6. doi:10.1108/IR-11-2017-0194
Boton, C., and Forgues, D. (2017). Construction Industrialization and it Integration: How Tall Wood Buildings Can Show the Right Path towards Construction 4.0. mocs. doi:10.29173/mocs64
Boton, C., Rivest, L., Ghnaya, O., and Chouchen, M. (2020). What Is at the Root of Construction 4.0: A Systematic Review of the Recent Research Effort. Arch. Computat Methods Eng. 28, 2331–2350. doi:10.1007/s11831-020-09457-7
Bou Hatoum, M., and Nassereddine, H. (2020). “Developing a Framework for the Implementation of Robotics in Construction Enterprises,” in Proceedings of the 27th International Workshop on Intelligent Computing in Engineering (Berlin, Germany), 453–462.
Bou Hatoum, M., Piskernik, M., and Nassereddine, H. (2020). A Holistic Framework for the Implementation of Big Data throughout a Construction Project Lifecycle. Kitakyushu, Japan. doi:10.22260/ISARC2020/0178
Calderon-Hernandez, C., and Brioso, X. (2018). Lean, BIM and Augmented Reality Applied in the Design and Construction Phase: A Literature Review. Ijimt 9 (1), 60–63. doi:10.18178/ijimt.2018.9.1.788
Chen, W., Chen, K., Cheng, J. C. P., Wang, Q., and Gan, V. J. L. (2018). BIM-based Framework for Automatic Scheduling of Facility Maintenance Work Orders. Automation in Construction 91, 15–30. doi:10.1016/j.autcon.2018.03.007
Chen, Y.-J., Lai, Y.-S., and Lin, Y.-H. (2020). BIM-based Augmented Reality Inspection and Maintenance of Fire Safety Equipment. Automation in Construction 110, 103041. doi:10.1016/j.autcon.2019.103041
Chua, C. K., and Leong, K. F. (2014). 3D Printing and Additive Manufacturing. 4th ed. World Scientific. doi:10.1142/9008
Construction Products Association (2016). The Future for Construction Product Manufacturing Digitalisation, Industry 4.0 and the Circular Economy. London, UK: Construction Products Association. Available at: http://thenorrisgroup.com/learning/files/media_manager/original/106.pdf.
Conti, M., Passarella, A., and Das, S. K. (2017). The Internet of People (IoP): A New Wave in Pervasive Mobile Computing. Pervasive Mobile Comput. 41, 1–27. doi:10.1016/j.pmcj.2017.07.009
Cooper, S. (2018). Civil Engineering Collaborative Digital Platforms Underpin the Creation of 'digital Ecosystems'. Proc. Inst. Civil Eng. - Civil Eng. 171, 14. doi:10.1680/jcien.2018.171.1.14
Craveiro, F., Duarte, J. P., Bartolo, H., and Bartolo, P. J. (2019). Additive Manufacturing as an Enabling Technology for Digital Construction: A Perspective on Construction 4.0. Automation in Construction 103, 251–267. doi:10.1016/j.autcon.2019.03.011
Dallasega, P., Rauch, E., and Linder, C. (2018). Industry 4.0 as an Enabler of Proximity for Construction Supply Chains: A Systematic Literature Review. Comput. Industry 99, 205–225. doi:10.1016/j.compind.2018.03.039
Derigent, W., Cardin, O., and Trentesaux, D. (2020). Industry 4.0: Contributions of Holonic Manufacturing Control Architectures and Future Challenges. J. Intell. Manuf. 32, 1797–1818. doi:10.1007/s10845-020-01532-x
Eadie, R., Browne, M., Odeyinka, H., McKeown, C., and McNiff, S. (2013). BIM Implementation throughout the UK Construction Project Lifecycle: An Analysis. Automation in Construction 36, 145–151. doi:10.1016/j.autcon.2013.09.001
El Jazzar, M., Piskernik, M., and Nassereddine, H. (2020). “Digital Twin in Construction: An Empirical Analysis,” in Proceedings of the EG-ICE 2020 Workshop on Intelligent Computing in Engineering. (Berlin, Germany).
El Jazzar, M. S., and Hamzeh, F. (2015). “Post Measuring the Last Planner Metrics in Shelter Rehabilitation Projects,” in Proceedings of the 23rd Annual Conference of the International Group for Lean Construction, IGLC 23. (Perth, Australia), 783–792.
Elhouar, S., Alzarrad, M. A., and Elhouar, S. (2019). “A Synopsis of 3D Printing and Robotics Applications in Construction,” in Proceedings of the Creative Construction Conference 2019 (Budapest, Hungary: Budapest University of Technology and Economics), 769–775. doi:10.3311/CCC2019-105
Eljazzar, M., Beydoun, A., and Hamzeh, F. (2013). “Optimizing Workflow for Shelter Rehabilitation Projects in Refugee Camps,” in Proc. 21st Ann. Conf. of the Int’l. Group for Lean Construction, 31–32.
Fergusson, K. J., and Teicholz, P. M. (1996). Achieving Industrial Facility Quality: Integration Is Key. J. Manag. Eng. 12 (1), 49–56. doi:10.1061/(ASCE)0742-597X10.1061/(asce)0742-597x(1996)12:1(49)
Fernandez Galarreta, J., Kerle, N., and Gerke, M. (2015). UAV-based Urban Structural Damage Assessment Using Object-Based Image Analysis and Semantic Reasoning. Nat. Hazards Earth Syst. Sci. 15 (6), 1087–1101. doi:10.5194/nhess-15-1087-2015
Fleming, K. L., Hashash, Y. M. A., McLandrich, S., O’Riordan, N., and Riemer, M. (2016). Novel Technologies for Deep-Excavation Digital Construction Records. Pract. Period. Struct. Des. Constr. 21 (4), 05016002. doi:10.1061/(asce)sc.1943-5576.0000295
Forcael, E., Ferrari, I., Opazo-Vega, A., and Pulido-Arcas, J. A. (2020). Construction 4.0: A Literature Review. Sustainability 12, 9755. doi:10.3390/su12229755
Forgues, D. (2019). De l’Industrie 4.0 à la Construction 4.0 : Des exemples concrets! Congrès 2019: Influencez l´avenir.
García de Soto, B., Agustí-Juan, I., Joss, S., and Hunhevicz, J. (2019). Implications of Construction 4.0 to the Workforce and Organizational Structures. Int. J. Construction Manag., 1–13. doi:10.1080/15623599.2019.1616414
Gerger, A., Schranz, Ch., and Urban, H. (2020). Neue Möglichkeiten durch den Einsatz von Augmented Reality im Kontext digitaler Bauvorhaben. (German) Scientific report. Vienna, Austria: BRISE-Vienna.
Goessens, S., Mueller, C., and Latteur, P. (2018). Feasibility Study for Drone-Based Masonry Construction of Real-Scale Structures. Automation in Construction 94, 458–480. doi:10.1016/j.autcon.2018.06.015
Goger, G., Piskernik, M., and Urban, H. (2017). Studie: Potenziale der Digitalisierung im Bauwesen. (German). Vienna, Austria: Scientific report. WKO.
Haggard, K. E. (2017). Case Study on Virtual Reality in Construction. San Lois Obispo, USA: California Polytechnic State University.
Haponiuk, B. (2020). Entwicklung einer Augmented Realilty Interface für TGA-Abnahme (Development of an Augmented Reality Interface for Technical Building Equipment Inspection). Vienna, Austria: TU Wien. Master thesis.
Hermann, M., Pentek, T., and Otto, B. (2016). “Design Principles for Industrie 4.0 Scenarios,” in 2016 49th Hawaii International Conference on System Sciences (HICSS), 3928–3937. doi:10.1109/HICSS.2016.488
Hofmann, E., and Rüsch, M. (2017). Industry 4.0 and the Current Status as Well as Future Prospects on Logistics. Comput. Industry 89, 23–34. doi:10.1016/j.compind.2017.04.002
I-SCOOP EU (2021). Industry 4.0: Fourth Industrial Revolution Guide to Industrie 4.0. -SCOOP. Available at: https://www.i-scoop.eu/industry-4-0/(Accessed May 30, 2021).
IBM (2021). What Is Industry 4.0. Available at: https://www.ibm.com/topics/industry-4-0 (Accessed May 28, 2021).
Ibrahim, F. S., Esa, M., Esa, M. R., and A. Rahman, R. (2021). The Adoption of IOT in the Malaysian Construction Industry: Towards Construction 4.0. Ijscet 12. doi:10.30880/ijscet.2021.12.01.006
IERC (2012). The Internet of Things 2012 –New Horizons. Available at: http://www.internet-of-things-research.eu/pdf/IERC_Cluster_Book_2012_WEB.pdf (Accessed May 31, 2021).
Irizarry, J., and Costa, D. B. (2106). Exploratory Study of Potential Applications of Unmanned Aerial Systems for Construction Management Tasks. J. Manag. Eng. 32 (3), 05016001. doi:10.1061/(ASCE)ME.1943-5479.0000422
ITU (2012). Series Y: Global Information Infrastructure, Internet Protocol Aspects and Next-Generation Networks, Overview of the Internet of Things. Available at: https://www.itu.int/rec/T-REC-Y.2060-201206-I/en (Accessed May 31, 2021).
Johnson, M. D., and K. Collins, P. (2017). Destech Conference Paper Outline. Keg 2 (1), 249–257. doi:10.18502/keg.v2i2.623
Jrade, A., and Lessard, J. (2015). An Integrated BIM System to Track the Time and Cost of Construction Projects: A Case Study. J. Construction Eng. 2015, 1–10. doi:10.1155/2015/579486
Kagermann, H., Wahlster, W., and Helbig, J. (2013). Recommendations for Implementing the Strategic Initiative Industrie 4.0: Final Report of the Industrie 4.0 Working Group. Berlin, Germany: Forschungsunion.
Kazado, D., Kavgic, M., and Eskicioglu, R. (2019). Integrating Building Information Modeling (BIM) and Sensor Technology Ror Facility Management. J. Inf. Techn. Construction (ITcon) 24, 440–458.
Kim, S. J., Jeong, Y., Park, S., Ryu, K., and Oh, G. (2018). “A Survey of Drone Use for Entertainment and AVR (Augmented and Virtual Reality),” in Augmented Reality and Virtual Reality. Editors T. Jung, and M. C. tom Dieck (Springer International Publishing), 339–352. doi:10.1007/978-3-319-64027-3_23
Klinc, R., and Turk, Ž. (2019). Construction 4.0 - Digital Transformation of One of the Oldest Industries. Econ. Business Rev. 21, 393–410. doi:10.15458/EBR.92
Koskela, L. (1992). Application of the New Production Philosophy to Construction. CIFE Technical Report #72, 72. USA: Stanford University. Available at: https://purl.stanford.edu/kh328xt3298.
Lasi, H., Fettke, P., Kemper, H.-G., Feld, T., and Hoffmann, M. (2014). Industry 4.0. Bus. Inf. Syst. Eng. 6, 239–242. doi:10.1007/s12599-014-0334-4
Li, Q., and Shi, J. (2015). Dam Construction 4.0. Shuili Fadian Xuebaojournal Hydroelectr. Eng. 34, 1–6. doi:10.11660/slfdxb.20150801
Li, X., Yi, W., Chi, H.-L., Wang, X., and Chan, A. P. C. (2018). A Critical Review of Virtual and Augmented Reality (VR/AR) Applications in Construction Safety. Automation in Construction 86, 150–162. doi:10.1016/j.autcon.2017.11.003
Li, Y., and Liu, C. (2019). Applications of Multirotor Drone Technologies in Construction Management. Int. J. Construction Manag. 19 (5), 401–412. doi:10.1080/15623599.2018.1452101
Lin, Z., Petzold, F., and Ma, Z. (2019). “A Real-Time 4D Augmented Reality System for Modular Construction Progress Monitoring,” in Proceedings of the 36th International Symposium on Automation and Robotics in Construction, 743–748 (Banff, AB, Canada. doi:10.22260/ISARC2019/0100
Liu, B. (2017). Construction Robotics Technologies 2030. Delft, Netherlands: Delft University of Technology. Available at: http://resolver.tudelft.nl/uuid:fcacf6fb-112c-453f-9903-8ec53274153f.
Lu, Y. (2017). Industry 4.0: A Survey on Technologies, Applications and Open Research Issues. J. Ind. Inf. Integration 6, 1–10. doi:10.1016/j.jii.2017.04.005
MacDougall, W. (2014). Industrie 4.0-Smart Manufacturing for the Future. Berlin: Germany Trade & Invest.
Manohar, N. (2016). Industry 4.0 and the Digital Transformation of the Automotive Industry. Available at: https://www.automotiveworld.com/articles/industry-4-0-digital-transformation-automotive-industry/.
Mohan, M., and Varghese, S. (2019). Artificial Intelligence Enabled Safety for Construction Sites. Int. Res. J. Eng. Techn. 06 (06), 681–685.
Nassereddine, H., Schranz, Ch., Bou Hatoum, M., and Urban, H. (2020a). “A Comprehensive Map for Integrating Augmented Reality during the Construction Phase,” in Proceedings of the Creative Construction E-Conference 202 (Opatija, Croatia: Budapest University of Technology and Economics), 13–18. doi:10.3311/ccc2020-069
Nassereddine, H., El Jazzar, M., and Piskernik, M. (2020b). “Transforming the AEC Industry: A Model-Centric Approach,” in Proceedings of the Creative Construction E-Conference 202 (Opatija, Croatia: Budapest University of Technology and Economics), 56–64. doi:10.3311/ccc2020-076
Nassereddine, H. (2019). Design, Development and Validation of an Augmented Reality-Enabled Production Strategy Process for the Construction Industry. USA: The University of Wisconsin-Madison. Dissertation.
Nassereddine, H., Veeramani, D., and Hanna, A. (2019). “Augmented Reality-Enabled Production Strategy Process,” in Proceedings of the 36th ISARC (Banff, AB, Canada), 297–305. doi:10.22260/ISARC2019/0040
Nisser, T., and Westin, C. (2006). Human Factors Challenges in Unmanned Aerial Vehicles (UAVs): A Literature Review. Scientific Report. Sweden: Lund University.
Oesterreich, T. D., and Teuteberg, F. (2016). Understanding the Implications of Digitisation and Automation in the Context of Industry 4.0: A Triangulation Approach and Elements of a Research Agenda for the Construction Industry. Comput. Industry 83, 121–139. doi:10.1016/j.compind.2016.09.006
Osunsanmi, O., Aigbavboa, C., Oke, A., and Ohiomah, I. (2018). “Construction 4.0: Towards Delivering of Sustainable Houses in South Africa,” in Proceedings of the Creative Construction Conference 2018 (Ljubljana, Slovenia).
Palmarini, R., Erkoyuncu, J. A., Roy, R., and Torabmostaedi, H. (2018). A Systematic Review of Augmented Reality Applications in Maintenance. Robotics and Computer-Integrated Manufacturing 49, 215–228. doi:10.1016/j.rcim.2017.06.002
Park, J., Cho, Y. K., and Martinez, D. (2016). A BIM and UWB Integrated Mobile Robot Navigation System for Indoor Position Tracking Applications. J. Construction Eng. Project Manag. 6 (2), 30–39. doi:10.6106/JCEPM.2016.6.2.030
Rael, R., and San Fratello, V. (2018). Printing Architecture: Innovative Recipes for 3D Printing. New York, USA: HudsonPrinceton Architectural Press.
Rao, S. (2019). The Benefits of AI in Construction. Available at: https://constructible.trimble.com/construction-industry/the-benefits-of-ai-in-construction.
Rastogi, S. (2017). “Construction 4.0: The 4th Generation Revolution,” in Proceedings of the ILCC 2017 Indian Lean Construction Conference, C288–98 (Madras, India).
Remmen, P., Cao, J., Ebertshäuser, S., Frisch, J., Lauster, M., Maile, T., et al. (2015). “An Open Framework for Integrated BIM-Based Building Performance Simulation Using Modelica,” in Proceedings of 14th IBPSA Conf. Building Simulation (India: Hyderabad), 379–386.
Rouhana, C., Aoun, M., Faek, F., El Jazzar, M., and Hamzeh, R. (2014). “The Reduction of Construction Duration by Implementing Contour Crafting (3D Printing),” in Proceedings of the 22nd Annual Conference of the Int. Group of Lean Construction, 1031–1042. (Olso, Norway. doi:10.13140/RG.2.1.1718.4646/1
Rüßmann, M., Lorenz, M., Gerbert, P., Waldner, M., Justus, J., Engel, P., et al. (2015). Industry 4.0: The Future of Productivity and Growth in Manufacturing Industries. Boston Consulting Group 9 (1), 54–89.
Sawhney, A., Riley, M., and Irizarry, J. (Editors) (2020). Construction 4.0: An Innovation Platform for the Built Environment (New York, NY: Routledge).
Schober, K.-S. (2016). Digitization in the Construction Industry: Building Europe’s Road to “Construction 4.0”. Germany: Roland Berger GMBH.
Schranz, Ch., Gerger, A., and Urban, H. (2020). Augmented Reality im Bauwesen: Teil 1 - Anwendungs - und Anforderungsanalyse (Aumgnted reality in Civil Engineering: Part 1 - Use-Case and Requirement Analysis). Bauingenieur 95 (10), 379–388. doi:10.37544/0005-6650-2020-10-49
Schranz, Ch., Urban, H., and Gerger, A. (2021). Potentials of Augmented Reality in a BIM Based Building Submission Process. J. Inf. Techn. Construction (ITcon) 26, 441–457. doi:10.36680/j.itcon.2021.024
Schuh, G., Jordan, F., Maasem, C., and Zeller, V. (2016). Industrie 4.0: Implikationen für produzierende Unternehmen. München, Germany: Geschäftsmodelle - Erfolgsfaktoren - Fallstudien, 39–58. doi:10.3139/9783446451148.004
Sommer, A. (2018). What Is Construction 4.0 and Is the Industry Ready. Available at: https://blogs.oracle.com/construction-engineering/post/what-is-construction-40-and-is-the-industry-ready (Accessed May 29, 2021).
Stone, M. (2021). How IoT & Big Data Are Transforming Construction. Verizon Enterp. Available at: https://enterprise.verizon.com/resources/articles/s/how-iot-and-big-data-are-transforming-the-construction-industry/(Accessed May 31, 2021).
Tay, Y. W. D., Panda, B., Paul, S. C., Noor Mohamed, N. A., Tan, M. J., and Leong, K. F. (2017). 3D Printing Trends in Building and Construction Industry: A Review. Virtual Phys. Prototyping 12 (3), 261–276. doi:10.1080/17452759.2017.1326724
Themistocleous, K., Ioannides, M., Agapiou, A., and Hadjimitsis, D. G. (2015). “The Methodology of Documenting Cultural Heritage Sites Using Photogrammetry, UAV, and 3D Printing Techniques: The Case Study of Asinou Church in Cyprus,” in Proceedings of the 3rd Int. Conference on Remote Sensing and Geoinformation of the Environment RSCy2015 (Cyprus: Paphos). doi:10.1117/12.2195626
Thuemmler, C., and Bai, C. (2017). “Health 4.0: Application of Industry 4.0 Design Principles in Future Asthma Management,” in Health 4.0: How Virtualization and Big Data Are Revolutionizing Healthcare. Editors C. Thuemmler, and C. Bai (Springer), 23–37. doi:10.1007/978-3-319-47617-9_2
Urban, H., and Schranz, Ch. (2018). AR-AQ-Bau – Use of Augmented Reality for Acceptance and Quality Assurance on Construction Sites. Available at: https://nachhaltigwirtschaften.at/en/sdz/projects/ar-aq-bau.php (Accessed May 31, 2021).
Urban, H., Schranz, Ch., and Gerger, A. (2019). BIM Auf Baustellen mit Augmented Reality (BIM on the Construction Site with Augmented Reality). Bauaktuell 10 (5), 192–196.
Vogel-Heuser, B., and Hess, D. (2016). Guest Editorial Industry 4.0-Prerequisites and Visions. IEEE Trans. Automat. Sci. Eng. 13 (2), 411–413. doi:10.1109/TASE.2016.2523639
Węglarz, A. (2018). Using Artificial Intelligence in Energy Efficient Construction. E3s Web Conf. 49, 00125. doi:10.1051/e3sconf/20184900125
Keywords: industry 4.0, technology interactions, construction lifecycle, construction 4.0, BIM, construction 4.0 implementation, technology integration
Citation: El Jazzar M, Schranz C, Urban H and Nassereddine H (2021) Integrating Construction 4.0 Technologies: A Four-Layer Implementation Plan. Front. Built Environ. 7:671408. doi: 10.3389/fbuil.2021.671408
Received: 23 February 2021; Accepted: 19 October 2021;
Published: 16 November 2021.
Edited by:
Olli Seppänen, Aalto University, FinlandReviewed by:
Eric Forcael, University of the Bío Bío, ChileCopyright © 2021 El Jazzar, Schranz, Urban and Nassereddine. This is an open-access article distributed under the terms of the Creative Commons Attribution License (CC BY). The use, distribution or reproduction in other forums is permitted, provided the original author(s) and the copyright owner(s) are credited and that the original publication in this journal is cited, in accordance with accepted academic practice. No use, distribution or reproduction is permitted which does not comply with these terms.
*Correspondence: Mahmoud El Jazzar, bWVsamF6emFyQHVreS5lZHU=; Christian Schranz, Y2hyaXN0aWFuLnNjaHJhbnpAdHV3aWVuLmFjLmF0; Hala Nassereddine, aGFsYS5uYXNzZXJlZGRpbmVAdWt5LmVkdQ==
Disclaimer: All claims expressed in this article are solely those of the authors and do not necessarily represent those of their affiliated organizations, or those of the publisher, the editors and the reviewers. Any product that may be evaluated in this article or claim that may be made by its manufacturer is not guaranteed or endorsed by the publisher.
Research integrity at Frontiers
Learn more about the work of our research integrity team to safeguard the quality of each article we publish.