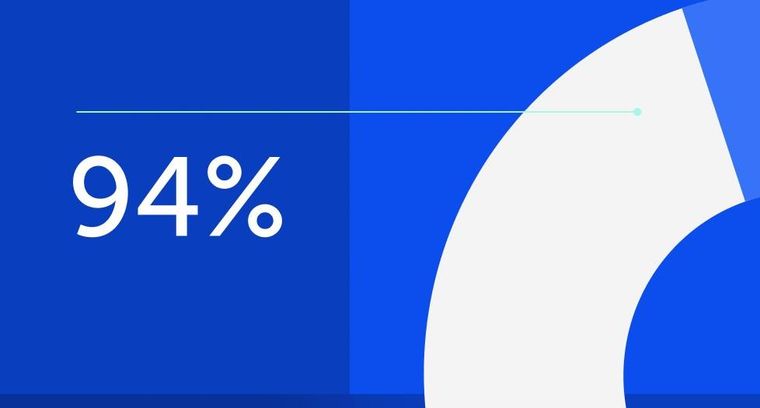
94% of researchers rate our articles as excellent or good
Learn more about the work of our research integrity team to safeguard the quality of each article we publish.
Find out more
ORIGINAL RESEARCH article
Front. Built Environ., 28 August 2019
Sec. Earthquake Engineering
Volume 5 - 2019 | https://doi.org/10.3389/fbuil.2019.00102
This article is part of the Research TopicSeismic Analysis and Retrofitting of Historical BuildingsView all 14 articles
In this paper, seismic vulnerability and risk assessment of two samples of churches, located in Teramo and Ischia island (Naples gulf), both affected by the most recent earthquakes that occurred in Italy, are presented. To this aim, we applied a simplified method particularly suitable for seismic evaluations at a territorial scale, providing a global resulting score to be compared among the cases analyzed. The data obtained allowed us to provide vulnerability maps and a seismic risk index for all the considered churches. In addition, the calculated indexes permit a preliminary health state evaluation of the inspected churches, for ranking the priorities and planning additional in-depth evaluations.
The structural analysis of monumental buildings belongs to a multidisciplinary study, where different types information are converging, such as construction history (year of erection, possible transformations, traumatic events), geometrical and structural critical survey, materials features and degradation, and the detection of crack patterns. In this way, it is possible to firstly diagnose the causes that produce instability and/or degradation of structural elements, which are very often multiple and generally act simultaneously. In general, these causes can be classified as intrinsic or extrinsic: the former refers to the origin and nature of the monumental buildings and, therefore, to their vulnerability; the latter is related to the site geographic conditions.
The Italian territory is characterized by a high seismicity level, demonstrated by the last earthquakes that occurred in the recent past such as in Irpinia (1980), San Giuliano of Puglia (2002), L'Aquila (2009), Emilia-Romagna (2012), Central Italy (2016), and Ischia (2017). These events unfortunately gave rise to serious consequences in terms of death and damage to historical buildings. Therefore, from an engineering point of view, appropriate numerical, experimental, and theoretical procedures are required in order to assess the seismic vulnerability of the structures and to design specific interventions useful for repairing the damages or avoiding future ones.
Recently, simplified models useful to preliminarily assess the seismic performance and the related risk at a territorial level have been proposed (Lourenc̣o and Roque, 2006; Directive of the Italian Prime Minister, 2011; Lourenc̣o et al., 2013). These methods are useful for ranking the priorities and for planning further analyses, to be conducted with more refined numerical models, which may regard some construction parts or the entire structure. To this aim, the second-level vulnerability forms developed by the Italian National Group for Earthquakes Defense (GNDT) (Benedetti and Petrini, 1984; GNDT, 1994), which are useful for screening the structures through systematic surveys, are worth noting. Among these simplified models, a new and simplified procedure, developed, and validated at the University of Basilicata, has been recently proposed. This procedure may also be applied to ancient masonry churches, and it is useful to evaluate the seismic risk at a territorial scale including natural threats due to the geographical surrounding context. This methodology, described and validated in Dı̀az Fuentes (2016), D'Amato et al. (2018), and D'Amato et al. (2019), and extended also to Chilean adobe churches in Fuentes et al. (2019a), is being applied in this paper to analyze the seismic vulnerability of two samples of Italian churches. In particular, the considered churches are located in two different geographical areas: in Teramo (Central Italy) and in Ischia island, located in the Naples gulf (Southern Italy). Both areas were affected by recent earthquakes and the considered churches suffered different structural damages. Initially, an international overview is shown on some researches devoted to the preservation of cultural heritage buildings through the proposal of manuals and principles for risk management, not limited to the seismic hazard. Later on, the simplified method considered in Dı̀az Fuentes (2016) and D'Amato et al. (2018) is described and applied to the two church samples, after collecting all information (structural and related to potential threats in the area) necessary for evaluating seismic vulnerability and the resulting risk indexes. Finally, the achieved results are compared and discussed. The obtained results show that the considered methodology is also useful for comparison of the seismic risk of different geographical areas. Moreover, a new territorial seismic risk score is also proposed.
Seismic vulnerability influences how damage caused by an earthquake in a given area is assessed from a construction point of view. The causes of high vulnerability even at very low levels of seismic forces may be different due, for instance, to structural irregularities, inadequate design, poor quality of materials, absence of constructive details, and scarce maintenance (Krstevska et al., 2010; Betti and Vignoli, 2011; Milani and Valente, 2015a; Clementi et al., 2017a,b; Fonti et al., 2017; Formisano et al., 2017, 2018; Milani et al., 2017a; Valente et al., 2017; Luchin et al., 2018; Valente and Milani, 2018a,b,c). After the recent seismic events, many efforts of the scientific community have been done in order to develop appropriate procedures for implementing seismic vulnerability analysis (Formisano, 2017; Formisano and Marzo, 2017; Laterza et al., 2017; Lopez et al., 2019) and specific retrofitting interventions (Faggiano et al., 2009; Terracciano et al., 2015; D'Amato et al., 2017; Milani et al., 2017b, 2018). In particular, as previously introduced, recurrent seismic damages were observed in historic masonry buildings characterized by local out-of-plane and in-plane response mechanisms regarding one or more isolated structure portions (Lagomarsino and Podestà, 2004; Formisano et al., 2010; Leite et al., 2013; Gattulli et al., 2014; Milani and Valente, 2015b; Stockdale, 2016; Valente et al., 2016; Betti et al., 2018; De Matteis et al., 2019; Fuentes et al., 2019b; Penna et al., 2019; Ramirez et al., 2019).
In order to assess the seismic performance of an existing structure, the current Italian Design Code (Ministry of Infrastructures and Transports, 2008a,b; Cecchi and Calvi, 2010; Directive of the Italian Prime Minister, 2011; Ministry of Infrastructures Transports, 2018) defines the design criteria and the performance targets to be satisfied under an earthquake action. These indications are useful for assessing vulnerability combined with the seismic hazard for evaluating the resulting seismic risk as well as all the possible effects in terms of expected damage that an earthquake can produce in a determined time and area.
With regard to cultural heritage conservation in the international scenario, various principles and manuals for risk management, such as those delivered by the United Nations Educational, Scientific and Cultural Organization (UNESCO), the International Council of Monuments and Sites (ICOMOS, 2008), the International Centre for the Study of Conservation and Restoration of Cultural Heritage (ICCROM), and the Getty Conservation Institute (UNESCO World Heritage Centre, 2002; ICOMOS, 2008), were developed. In addition, innovative prevention programs, such as the Risk Maps of different countries and the Disaster Prevention Program on Cultural Heritage (INAH, 2013), have been set up. However, the principles proposed found rare applications, because they did not take into account the different cultural, social, and economic values among countries. In this context, the ICCROM and the Getty Conservation Institute, with the publication “Between Two Earthquakes” in 1987 (Feilden, 1987), were the first to propose guidelines for prevention of disaster risks. These guidelines focused on two constructive vulnerability aspects, namely, the intrinsic structure, and vulnerability due to building location. In 1998, the first risk management manual for the world cultural heritage was developed (Stovel, 1998), whose most important proposals concerned both threats and the cultural heritage value for the community. This document was updated in 2009, when the United Nations Office for Disaster Reduction (UNISDR) published “Terminology on disaster risk reduction” (UNISDR, 2009). Subsequently, in 2010, UNESCO, ICCROM, ICOMOS, and the World Union for Conservation of Nature (IUCN) developed the Managing Disaster Risks for World Heritage (UNESCO/ICCROM/ICOMOS/IUCN, 2010), a document integrating Stovel's manual and introducing physical nature threats caused by climatic factors and chemical nature hazards.
Seismic risk is the measurement of the expected damage of buildings placed at a specified site in a given time interval. It is considered as the combination of three factors, namely, hazard, vulnerability, and exposure. Therefore, in order to assess the seismic risk of a built area, knowledge of only the hazard is not enough, since it is also necessary to carefully estimate the different construction vulnerabilities present during the seismic event and the related economic and social value of their content.
In this paper, the methodology applied refers to a recent work carried out by the University of Basilicata for providing territorial risk maps for planning useful intervention plans also addressed to increase cultural heritage resilience (D'Amato et al., 2018; Fuentes et al., 2019a). This simplified method may be applied, in general, in a multi-risk evaluation procedure, by considering both natural and anthropic threats. In this work, the method is applied only for a seismic risk analysis, involving the application of three distinct tools, each of which assigns a specific score:
1. Tool 1: Priority attention on actions related to the buildings according to their exposure value (E);
2. Tool 2: Description, classification, and mapping of seismic hazard (H);
3. Tool 3: Evaluation and quantification of the seismic vulnerability level (V).
The choice of this simplified method is due to the fact that it allows assessment of seismic risk at a territorial scale before a real seismic event, just supposing different earthquake magnitudes. On the contrary, other methods, such as the ones proposed by the GNDT or Italian civil protection, are based on a post-earthquake survey of damages with visual analyses. Moreover, the method permits a fast appraisal of a large number of ancient churches in a certain area requiring very simple information about dimensional features, environmental characteristics, and site morphology. Once the first seismic risk screening is conducted, it is possible to carry out more in-depth analyses in order to program retrofitting interventions. However, the present simplified model does not take into account the cumulative damage due to repeated shocks (for example, during a typical seismic sequence, or due to a series of events acting on unrepaired buildings) that influences the vulnerability of the buildings investigated and, therefore, may vary seismic risk ranking.
In this study, the simplified method considered is applied, as it will be discussed later on, to two different samples of churches located in two distinct geographical areas: it allows one to perform comparative seismic risk analyses at a territorial scale.
The simplified procedure allows one to separately score exposure value (application of Tool 1), seismic hazard (Tool 2), and seismic vulnerability (Tool 3). Then, the so-obtained scores are multiplied in accordance with the relationship (UNDRO, 1979; FEMA, 2004):
Tool 1 estimates the cultural values, divided into socio-cultural, and economic values. Socio-cultural values include antiquity, historical, symbolic, and aesthetic values. Economic values concern the value in use, as well as financial and scientific values. In this study, seismic risk assessment has been performed unless the score E is assigned by means of Tool 1.
Tool 2 provides the H score. It considers the risk from different points of view, with the aim of conducting a qualitative analysis that leads to the identification of threats conditioning the performances of buildings. Threats are divided into two categories, namely, sporadic events and continuous processes, depending on their occurrence probability. In particular, they can be grouped into three families:
- Natural threats, configured as sporadic events with catastrophic, or serious consequences;
- Physical threats, configured as continuous processes, whose consequences are generally low, even if they gradually increase;
- Anthropogenic, chemical, and electrochemical threats, generally corresponding to continuous processes with low or gradual consequences, except for the cases of sporadic events (i.e., fires caused by industrial activities and forest fires) with catastrophic consequences.
The risk scenarios are divided into the best, the most probable, and the worst. They are determined on the basis of the statistical principle that considers a higher probability of catastrophic events in areas already affected by earthquakes. The main natural threats are earthquakes and tsunami, landslides and floods, hydro-meteorological hazards, and volcanic phenomena. Physical threats are represented by water, terrestrial hazards, thermal risks, and dangers due to atmospheric environment. For these threats, the erosion index and the physical stress ones are defined.
The anthropogenic, chemical, and electrochemical threats are of the following different nature:
- Chemical: fires, explosions, radiation, toxic losses;
- Health-ecological: epidemics or parasites, air, soil, or water pollution;
- Socio-organizational: wars; social hardship manifestations; terrorism; vandalism; tourist pressure; population overload; relative humidity increase; air, marine, or terrestrial accidents; and forest fires;
- Severe demographic decline with consequent building abandonment and consequent lack of maintenance: material deterioration, loss of water (due to broken pipes, drainage problems, water protection, etc.).
Among these threats, those that influence cultural heritage the most are air and water pollution, which cause deterioration of materials and the environment.
All the above parameters are analyzed to determine the worst possible situation, based on historical information. They are subsequently classified according to the severity of the potential damage to monumental buildings. Damage can be absent, low or gradual, and catastrophic. These damage typologies are characterized by a given score assigned to each parameter on the basis of the threat influence on the building seismic behavior (Table 1). The resulting seismic hazard index (H), obtained by summing the singular threats scores, may assume a value ranging from 0 and 1. More details about the H scoring may be found in Dı̀az Fuentes (2016) and D'Amato et al. (2018).
The application of Tool 3 provides the vulnerability score V. This tool aims at evaluating the seismic vulnerability of the considered church, and it is based on the assessment of 13 vulnerability parameters related to various construction aspects. Specifically, 10 of these 13 parameters are derived from the Italian second-level GNDT vulnerability datasheet (GNDT, 1994). Each parameter has a different weight pi, and is characterized by four different scores vi associated to four possible classes (A, B, C, and D). The values of pi and vi are reported in Table 2.
Finally, according to the considered method, the vulnerability index V may be evaluated with the following relationship:
where, the sum is extended to all possible parameters considered. In particular, as it is worthy to note, the higher the V score, the higher the seismic vulnerability of a structure, which may fall within the following ranges:
- Low vulnerability: 0 < V ≤ 10.81;
- Medium vulnerability: 10.81 < V ≤ 55.52;
- High vulnerability: 55.52 < V ≤ 100.
In the following, each parameter considered is described in detail. More information may be found in Dı̀az Fuentes (2016) and D'Amato et al. (2018).
By indicating as Δh the foundation difference altitude, the four considered classes are as follows:
Class A:
- Buildings placed on rocky terrain with slopes equal to or lower than 10% and any Δh;
- Buildings placed on loose ground with slopes lower than or equal to 10% and Δh = 0.
Class B:
- Buildings placed on rocky terrain with a slope of 10–30% and any Δh;
- Buildings placed on loose ground with Δh ≤ 1 m and in the absence of unbalanced pressures due to embankments also verifying one of the following conditions:
1. A ground slope lower than 10% and 0 < Δh ≤ l;
2. A ground slope of 10–30% and Δh ≤ l;
3. Building without foundations, a ground slope of 10–30% and Δh ≤ l.
Class C:
- Buildings placed on rocky terrain with a slope of 30–50% and any Δh;
- Buildings on loose ground and Δh ≤ l, which verifies one of the following conditions:
1. Absence of unbalanced thrusts due to embankments, the building has foundations, a ground slope of 30–50%, and Δh ≤ l;
2. Absence of unbalanced thrusts due to embankments, the building has no foundations, the ground has a slope of 20–30%, and Δh ≤ l;
3. Presence of unbalanced thrusts due to embankments, the building has foundations, the ground has a slope <50%, and Δh ≤ l;
4. Presence of unbalanced thrusts due to embankments, the building has no foundations, the ground has a slope <30%, and Δh ≤ l.
Class D:
All the other cases that do not fall into the previous classes.
In the case of a rectangular building, a significant parameter is the ratio between the dimensions of minor and major sides β1 = a/l × 100 (Figure 1). In case of plan layouts with different shapes, in addition to the β1 parameter, it is necessary to take into account another parameter β2 = b/l × 100, which is the ratio between the deviation size and the larger dimension (Figure 1).
The assignment to a given class is made on the basis of the most unfavorable conditions set by the β1 and β2 parameters as follows:
Class A:
β1 ≥ 80 and β2 ≤ 10
Class B:
60 ≤ β1 <80 and 10 < β2 ≤ 20
Class C:
40 ≤ β1 <60 and 20 < β2 ≤ 30
Class D:
β1 <40 and β2> 30
It is necessary to take into account the presence of porticoes having a significant surface compared to that of the building, as well as towers of significant height and mass compared to the remaining part of the building. For the mass variation evaluation, the ± ΔM/M ratio is considered, where:
- ΔM is the mass variation between two successive levels;
- M is the mass of the lower floor.
Percentage variations <10% can be considered negligible. As a rule, the ΔM/M ratio can be replaced by the ±ΔA/A ratio, where A and ΔA are the plan covered surface and its variation, respectively. The four classes to be considered are as follows:
Class A:
- Buildings with uniform mass distribution over the whole height;
- Buildings with mass continually decreasing.
Class B:
- Buildings with porticoes and loggias of modest size;
- Buildings that present backwardness resulting in a decrease of the floor area >10 and <20%;
- Buildings with towers with a height <10% of the total building.
Class C:
- Buildings with porticoes or loggias having surface >10% and equal to or <20% of the total covering floor area;
- Buildings with retractions involving a reduction of the floor area more than 20%;
- Buildings with towers with a height more than 10 and <40% of the total building.
Class D:
All the other cases that do not fall into the previous classes.
The aim of this parameter is to evaluate the presence of walls (without considering partition walls) intersected by transverse ones that are able to constitute an efficient constraint to prevent the development of out-of-plane overturning mechanisms. The vulnerability classes are the following.
Class A:
Buildings having the following geometrical features:
- Slenderness (height/thickness) <8;
- The internal room width should not be more than 2.5 times the wall thickness;
- Door and window must be located at a distance from the nearest free edge almost three times the wall thickness;
- The distance between the wall bracing axes must be <6 times the wall thickness;
- The wall relative verticality must not be >10% of its height.
Class B:
- Buildings with only three of the Class A geometric features.
Class C:
- Buildings with only two of the Class A geometric features.
Class D:
- Buildings that do not have the geometrical features described in class A.
In this parameter, all the non-structural elements, such as fixtures, appendices, and projections that can cause damage to people or things, are considered. The classes are defined as follows:
Class A and Class B:
- Buildings without windows, appendices, overhangs, or false ceilings;
- Buildings with windows and fixtures well-connected to the walls;
- Buildings with balconies that are an integral part of the horizontal structures.
Class C:
- Buildings with external fixtures poorly bounded to the walls and with small false ceilings not well-connected.
Class D:
All the other cases that do not fall into the previous classes.
The organization of the vertical elements is evaluated regardless of the material characteristics of walls. The significant element is the presence and effectiveness of the connections among orthogonal walls in order to ensure box structure behavior efficiency. The four classes are as follows:
Class A:
- Existing buildings consolidated or repaired according to the actual seismic rules.
Class B:
- Buildings with good connections among orthogonal walls.
Class C:
- Buildings that do not have adequate connections between walls and upper floors;
- Buildings with orthogonal walls having a good connection at all levels and floors built up with materials different from the original ones;
- One-story buildings composed of orthogonal walls not adequately connected, which instead have a good connection between the walls and the roofing system thanks to continuous horizontal structures made of original materials or materials compatible to the existing ones in terms of strength and stiffness.
Class D:
All the other cases that do not fall into the previous classes.
It depends on the material and masonry type. The four classes are as follows:
Class A:
- Square stone masonry having a good-quality mortar;
- Tuff masonry with low porosity and a good-quality mortar;
- Masonry composed of solid bricks having a good-quality mortar;
- Retrofitted masonry according to the current seismic rules.
Class B:
- Stone masonry composed of non-homogeneous elements having a good-quality mortar;
- Masonry composed of solid bricks having a medium-quality mortar.
Class C:
- Squared masonry stones with irregularities having plaster and medium-quality mortar;
- Non-squared masonry stones having plaster and medium-quality mortar
- Sack masonry stones having plaster and medium-quality mortar;
- Squared masonry stones having plaster and medium-quality mortar;
- Masonry bricks having low-quality mortar.
Class D:
Masonry types that do not fall within the previous classes.
This parameter expresses the type and properties of horizontal structures. The four classes are defined as follows:
Class A:
Rigid slabs having:
a) Negligible in-plane deformability;
b) Effective floor–wall connections;
c) Absence of staggered floors.
Class B:
- Buildings that do not satisfy the third requirement of the previous class.
Class C:
- Deformable floor having good connections among walls.
Class D:
- Buildings that do not fall within the previous classes.
The roof elements influencing the building's seismic behavior are as follows: thrusts on the perimeter walls, connections between roof and walls, seismic mass, stiffness, and strength difference with respect to the masonry building. The four classes are as follows:
Class A:
- Buildings with non-thrusting roofs having edge beams and/or metal tie rods.
Class B:
- Buildings with non-thrusting roofs without edge beams and/or metal tie rods;
- Buildings with non-thrusting roofs having edge beams and/or metal tie rods with the absence of efficient connections between the roof and walls.
Class C:
- Buildings with thrusting roofs made of the original building materials or materials compatible to the original ones in terms of strength and stiffness and without edge beams and/or metal tie rods;
- Buildings with non-thrusting roofs made of the original building materials or materials compatible to the original ones in terms of strength and stiffness and without edge beams and/or metal tie rods.
Class D:
- Buildings that do not fall within the previous classes.
This parameter considers the actual building status. The four classes are as follows:
Class A:
- Walls in good condition without visible cracks.
Class B:
- Buildings with no diffused cracks, but with possible lesions generated by earthquakes.
Class C:
- Buildings with medium-size cracks (width of the lesion: 2–3 mm);
- Buildings without cracks, but with walls having a conservation status leading toward a significant resistance decrease.
Class D:
Buildings that do not fall within the previous classes.
Cracks and deformations can derive from different causes, such as construction defects, humidity presence, earthquakes, etc. The analyzed damage allows the interpretation of possible collapse mechanisms. In the vulnerability general form, it is necessary to identify in the appropriate section the type of existing damage (structural, non-structural, or humidity) and to express the percentage extension on the structural elements. The possible collapse mechanisms are illustrated in Figure 2.
The parameters useful for the vulnerability evaluation are as follows:
- Accessibility: in case of a catastrophic event, buildings have no accessibility to roads and/or infrastructures;
- Abandonment: the building is in an abandoned context;
- Population density: the building is located in a densely populated area;
- Isolation: the building is located at a considerable distance from the city center;
- Relationship with the geographical context: the building is in a situation of conflict with the site;
- Relationship with the built context: the building is in a situation of conflict with the adjacent constructions;
- Community relationship: the building is in a situation of conflict with the social context;
- Disinterest: the physical and social environments have no relationship with the building.
The classification is as follows:
Class A:
- Buildings that do not have any of the above conditions.
Class B:
- Buildings that have almost three of the above conditions.
Class C:
- Buildings that have almost six of the above conditions.
Class D:
- Buildings that have more than six of the above conditions.
Some interventions on buildings are useful for improving the response toward seismic events. However, following recent earthquakes, it was observed that invasive interventions with materials different from building original ones cause high vulnerabilities, leading to collapse in some cases. The classification is as follows:
Class A:
- Structures without interventions to the building system;
- Structures with modifications to the building system by reversible interventions made of materials compatible to the original ones in terms of strength and stiffness.
Class B:
- Structures with modifications to the building system by non-reversible interventions made of materials compatible to the original ones in terms of strength and stiffness.
Class C:
- Structures with interventions made of materials compatible to the existing ones that have modified the building mass.
Class D:
- Structures with interventions made of materials incompatible to the existing ones in terms of strength and stiffness.
The parameters affecting fire resistance are as follows:
- Presence of ornaments and flammable materials;
- Roofs or cellars dust accumulation;
- Walls, floors, and doors with low fire resistance;
- Lack of compartmentation;
- Inadequate exits through doors, corridors, or stairs;
- Faulty electrical systems;
- Faulty fireplaces with soot and grease accumulation;
- Low standard in organization of fire drills;
- Fire danger due to smoking or kitchen operations.
The resulting seismic risk score (R) may be calculated, in accordance with the simplified method considered, as follows (D'Amato et al., 2018):
where the H score is increased to unity for having a resulting score higher than 1. As it is easy to understand, the seismic risk score R increases as the vulnerability V and/or the hazard scoring H increases.
As previously introduced, in this paper, the vulnerability and the risk assessment of two samples of churches are presented. In particular, the chosen churches are located in two different geographical areas, both struck by recent Italian earthquakes. The first group of churches is located in Teramo, in central Italy, hit by the L'Aquila earthquake in 2009. The second group of churches falls within the Ischia island, in the province of Naples, which was hit by an earthquake in 2017.
Teramo is the provincial capital of the homonymous province, located in the northern area of Abruzzo. It is placed within the Tordino Valley, a hilly area near the Gran Sasso Mountain, which extends toward the coast with a rich vegetation of vineyards and olive groves. It is the third most populous town of the Abruzzo region and has an area of about 10 km2. The churches investigated, distributed within the Teramo area as reported in Figure 3, are 12 in total and listed as follows, indicating also the city hamlets where they are located:
1. Saint John church (Teramo);
2. Saint Anastasio church (Poggio Cono, hamlet of Teramo);
3. Holy Mary of Carmine church (Cavuccio, hamlet of Teramo);
4. Saint Nicola church (Cavuccio, hamlet of Teramo);
5. Saint Catherine of Alexandria church (Teramo);
6. Saint Luca church (Teramo);
7. Saint Mary de Praediis church (Pantaneto, hamlet of Teramo);
8. Saint Michael Archangel church (Magnanella, hamlet of Teramo);
9. Saint Francis of Assisi church (Villa Romano, hamlet of Teramo);
10. Saint John in Pergulis church (Valle San Giovanni, hamlet of Teramo);
11. Most Holy Salvatore church (Frondarola, hamlet of Teramo);
12. Saint Stephen church (Rapino, hamlet of Teramo).
Some images of the considered churches are reported in Table 3. For sake of completeness, the main geometric features of each church are reported in Table 4. In this table, the major and minor dimensions and the height of the hall and of the apse (if present) are reported. In addition, information about the presence of the bell tower and its estimated height are given as well.
Ischia is an Italian island belonging to the Flegree islands archipelago in the Naples province. The island, which is the largest of the Flegree islands, is located in the northern area of the Gulf of Naples and not far from the Procida island in the Tyrrhenian Sea. Ischia is about 18 nautical miles from Naples; it extends 10 and 7 km from east to west and from north to south, respectively, and has a coastline of 34 km and a surface area of about 46.3 km2. The island has a volcanic character, formed by several eruptions since about 150,000 years ago. The oldest parts of the island, which dates back to between 147,000 and 100,000 years ago, are recognizable along the southern coastlines. The following 10 churches are considered, located within the municipality indicated within parentheses, as illustrated in Figure 4:
1. Saint Francis of Paola church (Forio municipality);
2. Saint Vito church (Forio municipality);
3. Most Holy Annunciation church (Lacco Ameno municipality);
4. Saint Sebastiano church (Barano d'Ischia municipality);
5. Saint Michael Archangel church (Forio municipality);
6. Saint Mary of Loreto church (Forio municipality);
7. Saint Francis of Assisi church (Forio municipality);
8. Most Holy Annunciation coven (Forio municipality);
9. Saint Mary of Soccorso church (Forio municipality);
10. Saint Gaetano church (Forio municipality).
Table 5 depicts some images of the considered church samples, while the main geometric features of each church are reported in Table 6.
From the analysis of all the collected information related to sporadic and continuous events [described in the section Hazard Scoring (H)], it has been possible to qualitatively evaluate the potential damage severity that could affect the examined churches. The evaluations are numerically reported in Table 7, where the damage assigned for each event is in bold and underlined. One can easily note that the investigated area of Ischia has a hazard score H greater than that of Teramo. This is due to the fact that, in the island, the potential threats that could produce damages are greater than those in the Teramo area.
In order to apply the analysis with Tool 3, it has been necessary to carry out physical observations and detailed historical researches for each considered church, with the aim of acquiring as much information as possible. Table 8 and Figure 5 summarize the evaluation of the seismic risk score for the churches studied. In particular, in Table 8, the R score is evaluated in accordance with the proposed (Equation 3), while in the Figure 5, a comparison between vulnerability and seismic risk score for each church is represented in the form of a histogram.
Table 9 shows, for the Teramo and Ischia samples, the number of churches falling into each class (from A to D) for a given vulnerability parameter. In this way, the distribution of the classes may be observed.
In the Teramo churches, there is a prevailing class for some of the vulnerability parameters, such as position of the building and foundation (no. 1), non-structural elements (no. 5), resistant system type and organization (no. 6), floors (no. 8), and fire vulnerability (no. 12).
On the other hand, in the Ischia church samples, a prevailing class for each parameter is observed in almost all cases, with the exception of the parameter distance among walls (no. 4), where classes A and B have been assigned to the same number of churches.
Finally, by observing the obtained results, the following considerations can be remarked:
1. Class A of the parameter position of the building and foundations (no. 1) has been assigned to almost all the churches, since they are located on loose ground with a slope not higher than 10% and with discrete geotechnical properties. However, it has not been possible to detect the in-elevation differences of the foundations due to the absence of appropriate geological analyses;
2. Class D of the parameter floors (no. 8) has been attributed to all churches since rigid floors have never been observed;
3. It has not been possible to give objective judgments on the connection quality among orthogonal walls and between walls and horizontal structures because of the presence of frescos and decorations. However, the experience suggests neglecting a good level of connection among walls because, in the past, orthogonal walls were generally simply juxtaposed to each other, showing in most of the cases overturning mechanisms, especially in the façade elements;
4. Almost all roofs are made of timber elements that have, depending on the case, pushing or no-pushing structures. In general, it is rare to find edge beams with the exception of those where the roof was built in recent times. Even the presence of metal tie rods is quite rare: they are absent in the majority of cases;
5. The churches located in Teramo have a vulnerability index ranging from 21.22 to 66.32, with an average value of 45.68;
6. The churches located in Ischia have a vulnerability index ranging from 25.93 to 46.46, with an average value of 30.13;
7. The Ischia island hazard index (H = 1.31) is greater than that of Teramo (H = 0.78).
In order to determine the church sample and, consequently, the geographical area that are subjected to the highest seismic risk, in this paper, a new territorial seismic risk index ρ is proposed as follows:
where Sj is the jth church area, Rj is the jth church seismic risk index, and ΣSj is the total area of investigated churches. Precisely, in the two geographical areas analyzed, the ρ index is equal to 63.13 for Teramo churches and to 67.47 in the case of Ischia churches. The higher ρ value in the case of Ischia churches is probably due to their higher vulnerability with respect to Teramo churches.
In this paper, a simplified method recently developed and validated for ancient masonry churches has been applied to case studies. The proposed method separately evaluates hazard (H) and vulnerability (V) in order to assess the seismic risk at a territorial scale. The method is a very useful tool because it quickly provides a territorial preliminary ranking for screening the intervention priorities and for considering different earthquake scenarios as well. The method is also versatile for comparing seismic risk evaluations performed in different geographical areas.
The case studies examined involved two samples of churches located in Teramo and in the Ischia island in the gulf of Naples, both affected by recent seismic events. The territories of churches have many features in common, but there are some differences modifying the vulnerability index calculations. In particular, the analysis of results shows that all the churches of Teramo have a medium vulnerability index. The most vulnerable church is the Saint Catherine of Alexandria, which is, in fact, actually unusable. Even the Ischia churches have a medium vulnerability index, with the highest value found in the case of the Saint Michael Archangel church.
Finally, it has been possible to estimate the vulnerability of inspected church areas by means of a new territorial seismic risk index ρ, which depends on the territory area covered by the churches and on their seismic risk index R. This new introduced index, useful for territorial comparisons, may be applied for globally evaluating the seismic risk of a certain area, representing a unique parameter taking into account all the constructions built and the related seismic risk scores.
All datasets generated or analyzed for this study are included in the manuscript.
All authors listed have made a substantial, direct and intellectual contribution to the work, and approved it for publication.
The authors declare that the research was conducted in the absence of any commercial or financial relationships that could be construed as a potential conflict of interest.
The reviewer MV declared a past co-authorship with one of the authors AF to the handling editor.
Benedetti, D., and Petrini, V. (1984). On the seismic vulnerability of masonry buildings: an assessment method (in Italian). L'industria delle Costruzioni 149, 66–74.
Betti, M., Borghini, A., Boschi, S., Ciavattone, A., and Vignoli, A. (2018). Comparative seismic risk assessment of basilica-type churches. J. Earthq. Eng. 22, 62–95. doi: 10.1080/13632469.2017.1309602
Betti, M., and Vignoli, M. (2011). Numerical assessment of the static and seismic behaviour of the basilica of Santa Maria all'Impruneta (Italy). Constr. Build. Mater. 25, 4308–4324. doi: 10.1016/j.conbuildmat.2010.12.028
Cecchi, R., and Calvi, M. (2010). “Guidelines for evaluation and mitigation of seismic risk to cultural heritage with reference to technical standard for construction (In Italian),” in Ministry for Cultural Heritage and Activities, ed G. Gangemi (Rome: Department of Civil Protection Agency).
Clementi, F., Gazzani, V., Poiani, M., Mezzapelle, P. A., and Lenci, S. (2017a). Seismic assessment of a monumental building through nonlinear analyses of a 3D solid model. J. Earthq. Eng. 22, 35–61. doi: 10.1080/13632469.2017.1297268
Clementi, F., Pierdicca, A., Formisano, A., Catinari, F., and Lenci, S. (2017b). Numerical model upgrading of a historical masonry building damaged during the 2016 Italian earthquakes: the case study of the Podestà palace in Montelupone (Italy). J. Civil Struct. Health Monit. 7, 703–717. doi: 10.1007/s13349-017-0253-4
D'Amato, M., Gigliotti, R., and Laguardia, R. (2019). Comparative seismic assessment of ancient masonry churches. Front. Built Environ. 5:56. doi: 10.3389/fbuil.2019.00056
D'Amato, M., Laterza, M., and Casamassima, V. M. (2017). Seismic performance evaluation of a multi-span existing masonry arch bridge. Open Constr. Build. Technol. J. 11, 1191–1207. doi: 10.2174/1874149501711011191
D'Amato, M., Laterza, M., and Diaz Fuentes, D. (2018). Simplified seismic analyses of ancient churches in Matera's landscape. Int. J. Archit. Herit. doi: 10.1080/15583058.2018.1511000
De Matteis, G., Brando, G., and Corlito, V. (2019). Predictive model for seismic vulnerability assessment of churches based on the 2009 L'Aquila earthquake. Bull. Earthq. Eng. 17, 4909–3. doi: 10.1007/s10518-019-00656-7
Dìaz Fuentes, D. A. (2016). Diseño de herramientas de Evaluación del Riesgo Para la Conservación del Patrimonio Cultural Inmueble: Aplicación en dos Casos de Estudio del Norte Andino Chileno (in Spanish). Mexico: Publicaciones Digitales ENCRYM – INAH.
Directive of the Italian Prime Minister (2011). Directive of the Prime Minister Dated on 09/ 02/2011. Assessment and mitigation of seismic risk of cultural heritage with reference to the technical code for the design of construction, issued by D. M. 14/ 01/2008 (in Italian).
Faggiano, B., Marzo, A., Formisano, A., and Mazzolani, F. M. (2009). Innovative steel connections for the retrofit of timber floors in ancient buildings: a numerical investigation. Comput. Struct. 87, 1–13. doi: 10.1016/j.compstruc.2008.07.005
Feilden, B. (1987). Between Two Earthquakes: Cultural Property in Seismic Zones. Roma: ICCROM/Getty Conservation Institute.
FEMA (2004). Primer for design professionals (FEMA389). Washington, DC: Department of Homeland Security Emergency Preparedness and Response Directorate.
Fonti, R., Borri, A., Barthel, R., Candela, M., and Formisano, A. (2017). Rubble masonry response under cyclic actions: experimental tests and theoretical models. Int. J. Mason. Res. Innov. 2, 30–60. doi: 10.1504/IJMRI.2017.082391
Formisano, A. (2017). Local- and global-scale seismic analyses of historical masonry compounds in San Pio delle Camere (L'Aquila, Italy). Nat. Hazards 86, 465–487. doi: 10.1007/s11069-016-2694-1
Formisano, A., Ciccone, G., and Mele, A. (2017). Large scale seismic vulnerability and risk evaluation of a masonry churches sample in the historical centre of Naples. AIP Conf. Proc. 1906:090003. doi: 10.1063/1.5012360
Formisano, A., Di Feo, P., Grippa, M. R., and Florio, G. (2010). “L'Aquila earthquake: a survey in the historical centre of Castelvecchio Subequo,” in COST ACTION C26: Urban Habitat Constructions under Catastrophic Events - Proceedings of the Final Conference (Naples), 371–376.
Formisano, A., and Marzo, A. (2017). Simplified and refined methods for seismic vulnerability assessment and retrofitting of an Italian cultural heritage masonry building. Comput. Struct. 180, 13–26. doi: 10.1016/j.compstruc.2016.07.005
Formisano, A., Vaiano, G., Fabbrocino, F., and Milani, G. (2018). Seismic vulnerability of Italian masonry churches: the case of the Nativity of Blessed Virgin Mary in Stellata of Bondeno. J. Build. Eng. 20, 179–200. doi: 10.1016/j.jobe.2018.07.017
Fuentes, D. D., Baquedano, J. P. A., D'Amato, M., and Laterza, M. (2019b). Preliminary seismic damage assessment of Mexican churches after September 2017 earthquakes. Int. J. Archit. Herit. doi: 10.1080/15583058.2019.1628323
Fuentes, D. D., Laterza, M., and D'Amato, M. (2019a). “Seismic vulnerability and risk assessment of historic constructions: the case of masonry and adobe churches in Italy and Chile (2019) RILEM Bookseries, 18,” in 11th International Conference on Structural Analysis of Historical Constructions (Cusco), 1127–1137. doi: 10.1007/978-3-319-99441-3_122
Gattulli, V., Antonacci, E., and Vestroni, F. (2014). Field observations and failure analysis of the Basilica S. Maria di Collemaggio after the 2009 L'Aquila earthquake. Eng. Fail. Anal. 34, 715–734. doi: 10.1016/j.engfailanal.2013.01.020
GNDT (1994). (National Group for Earthquakes Defense) First and Second Level Form for Exposure, Vulnerability and Damage Survey (Masonry and Reinforced Concrete) (in Italian). Rome: GNDT.
Krstevska, L., Tashkov, L., Naumovski, N., Florio, G., Formisano, A., Fornaro, A., et al. (2010). “In-situ experimental testing of four historical buildings damaged during the 2009 L'Aquila earthquake,” in COST ACTION C26: Urban Habitat Constructions under Catastrophic Events - Proceedings of the Final Conference (Naples), 427–432.
Lagomarsino, S., and Podestà, S. (2004). Seismic vulnerability of ancient churches: II. Statistical analysis of surveyed data and methods for risk analysis. Earthq. Spectra 20, 395–412. doi: 10.1193/1.1737736
Laterza, M., D'Amato, M., and Casamassima, V. M. (2017). “Seismic performance evaluation of multi-span existing masonry arch bridge,” in Proceedings of the 14th International Conference of Numerical Analysis and Applied Mathematics - ICNAAM 2016 AIP Conference Proceedings (Rhodes), 1863:art. no. 450010. doi: 10.1063/1.4992619
Leite, J., Lourenco, P. B., and Ingham, J. M. (2013). Statistical assessment of damage to churches affected by the 2010–2011 Canterbury (New Zealand) earthquake sequence. J. Earthq. Eng. 17, 73–97. doi: 10.1080/13632469.2012.713562
Lopez, S., D'Amato, M., Ramos, L., Laterza, M., and Lourenço, P. B. (2019). Simplified formulation for estimating the main frequencies of ancient masonry churches. Front. Built Environ. 5:18. doi: 10.3389/fbuil.2019.00018
Lourenc̣o, P. B., Oliveira, D. V., Leite, J. C., Ingham, J. M., Modena, C., and Da Porto, F. (2013). Simplified indexes for the seismic assessment of masonry buildings: International database and validation. Eng. Fail. Anal. 34, 585–605. doi: 10.1016/j.engfailanal.2013.02.014
Lourenc̣o, P. B., and Roque, J. A. (2006). Simplified indexes for the seismic vulnerability of ancient masonry buildings. Constr. Build. Mater. 20, 200–208. doi: 10.1016/j.conbuildmat.2005.08.027
Luchin, G., Ramos, L. F., and D'Amato, M. (2018). Sonic tomography for masonry walls characterization. Int. J. Archit. Herit. doi: 10.1080/15583058.2018.1554723
Milani, G., Shehu, R., and Valente, M. (2017a). Role of inclination in the seismic vulnerability of bell towers: FE models and simplified approaches. Bull. Earthq. Eng. 15, 1707–1737. doi: 10.1007/s10518-016-0043-0
Milani, G., Shehu, R., and Valente, M. (2017b). Possibilities and limitations of innovative retrofitting for masonry churches: advanced computations on three case studies. Constr. Build. Mater. 147, 239–263. doi: 10.1016/j.conbuildmat.2017.04.075
Milani, G., Shehu, R., and Valente, M. (2018). A kinematic limit analysis approach for seismic retrofitting of masonry towers through steel tie-rods. Eng. Struct. 160, 212–228. doi: 10.1016/j.engstruct.2018.01.033
Milani, G., and Valente, M. (2015a). Failure analysis of seven masonry churches severely damaged during the 2012 Emilia-Romagna (Italy) earthquake: non-linear dynamic analyses vs conventional static approaches. Eng. Fail. Anal. 54, 13–56. doi: 10.1016/j.engfailanal.2015.03.016
Milani, G., and Valente, M. (2015b). Comparative pushover and limit analyses on seven masonry churches damaged by the 2012 Emilia-Romagna (Italy) seismic events: possibilities of non-linear finite elements compared with pre-assigned failure mechanisms. Eng. Fail. Anal. 47, 129–161. doi: 10.1016/j.engfailanal.2014.09.016
Ministry of Infrastructures and Transports (2008a). Ministerial Decree 14 January 2008 “Technical codes for constructions” (in Italian). Official Gazette n. 29.
Ministry of Infrastructures and Transports (2008b). Ministerial Circular 2 February 2008 n. 617 “Instructions for the Application of New Technical Codes for Constructions” (in Italian). Official Gazette n. 47.
Ministry of Infrastructures and Transports (2018). Ministerial Decree 17 January 2018, Updating of Technical Codes for Constructions (in Italian). Official Gazette n. 42 of 20/02/18, Ordinary Supplement n. 8.
Penna, A., Calderini, C., Sorrentino, L., Carocci, C. F., Cescatti, E., Sisti, R., et al. (2019). Damage to churches in the 2016 central Italy earthquakes. Bull. Earthq. Eng. 1–28. doi: 10.1007/s10518-019-00594-4
Ramirez, E., Lourenco, P. B., and D'Amato, M. (2019). “Seismic assessment of the Matera Cathedral. SAHC 2018,” in 11th International Conference on Structural Analysis of Historical Constructions (Cusco: RILEM Bookseries), 1346–1354. doi: 10.1007/978-3-319-99441-3_144
Stockdale, G. (2016). Reinforced stability-based design: a theoretical introduction through a mechanically reinforced masonry arch. Int. J. Mason. Res. Innov. 1, 101–141. doi: 10.1504/IJMRI.2016.077469
Stovel, H. (1998). Risk Preparedness: A Management Manual for World Cultural Heritage. Roma: ICCROM/ UNESCO, WHC/ICOMOS.
Terracciano, G., Di Lorenzo, G., Formisano, A., and Landolfo, R. (2015). Cold-formed thin-walled steel structures as vertical addition and energetic retrofitting systems of existing masonry buildings. Eur. J. Environ. Civ. Eng. 19, 850–866. doi: 10.1080/19648189.2014.974832
UNDRO (1979). Natural Disasters Vulnerability Analysis. United Nations Disaster Relief Organisation.
UNESCO World Heritage Centre (2002). Managing Tourism at World Heritage Sites: A Practical Manual for World Heritage Site Managers.
Valente, M., Barbieri, G., and Biolzi, L. (2016). Damage assessment of three medieval churches after the 2012 Emilia earthquake. Bull. Earthq. Eng. 15, 2939–2980. doi: 10.1007/s10518-016-0073-7
Valente, M., Barbieri, G., and Biolzi, L. (2017). Seismic assessment of two masonry Baroque churches damaged by the 2012 Emilia earthquake. Eng. Fail. Anal. 79, 773–802. doi: 10.1016/j.engfailanal.2017.05.026
Valente, M., and Milani, G. (2018a). Effects of geometrical features on the seismic response of historical masonry towers. J. Earthq. Eng. 22, 2–34. doi: 10.1080/13632469.2016.1277438
Valente, M., and Milani, G. (2018b). Damage assessment and partial failure mechanisms activation of historical masonry churches under seismic actions: three case studies in Mantua. Eng. Fail. Anal. 92, 495–519. doi: 10.1016/j.engfailanal.2018.06.017
Keywords: seismic vulnerability, seismic risk, masonry church, L'Aquila earthquake, Ischia earthquake, large-scale analysis method, damage index
Citation: Fabbrocino F, Vaiano G, Formisano A and D'Amato M (2019) Large-Scale Seismic Vulnerability and Risk of Masonry Churches in Seismic-Prone Areas: Two Territorial Case Studies. Front. Built Environ. 5:102. doi: 10.3389/fbuil.2019.00102
Received: 19 February 2019; Accepted: 09 August 2019;
Published: 28 August 2019.
Edited by:
Massimiliano Pittore, German Research Centre for Geosciences, Helmholtz Centre Potsdam, GermanyReviewed by:
Marco Valente, Politecnico di Milano, ItalyCopyright © 2019 Fabbrocino, Vaiano, Formisano and D'Amato. This is an open-access article distributed under the terms of the Creative Commons Attribution License (CC BY). The use, distribution or reproduction in other forums is permitted, provided the original author(s) and the copyright owner(s) are credited and that the original publication in this journal is cited, in accordance with accepted academic practice. No use, distribution or reproduction is permitted which does not comply with these terms.
*Correspondence: Antonio Formisano, YW50b2Zvcm1AdW5pbmEuaXQ=
Disclaimer: All claims expressed in this article are solely those of the authors and do not necessarily represent those of their affiliated organizations, or those of the publisher, the editors and the reviewers. Any product that may be evaluated in this article or claim that may be made by its manufacturer is not guaranteed or endorsed by the publisher.
Research integrity at Frontiers
Learn more about the work of our research integrity team to safeguard the quality of each article we publish.