- 1Preventive Medicine and Biostatistics, Uniformed Services University of the Health Sciences, Bethesda, MD, United States
- 2National Scientific Center, Institute of Experimental and Clinical Veterinary Medicine, Kharkiv, Ukraine
- 3Nonclinical Research, Appili Therapeutics USA Inc., Frederick, MD, United States
Tularemia is a highly dangerous, febrile zoonotic bacterial infection, endemic to many regions of the Northern hemisphere including Eastern Europe. The current war in Ukraine raises the risk for both natural outbreaks of tularemia resulting from destroyed infrastructure, and potential use as a weapon of war or bioterrorism. There has been a substantial history of outbreaks in prior wars in the region, and tularemia has been previously weaponized and stockpiled as an offensive agent. There are current reports of “mouse fever”, where Russian soldiers are affected with a high fever associated with mice in the battlefield. While antibiotic countermeasures to naturally occurring forms of tularemia are available, these may not be appropriate for long-term risks on the battlefield and could contribute to antibiotic resistance. At this time, there are no FDA or EMA approved tularemia vaccines, which are an ideal alternative to antibiotic prophylaxis. Vaccines have advantages in not requiring diagnosis, are generally safe and effective, leading to long-lasting prophylaxis with little impact to the patient and little need for future medical intervention. The potential for engineered forms of the disease for deliberate use may limit the effectiveness of existing countermeasures. The sporadic nature of outbreaks, and likely outcomes following deliberate use raise a number of complexities in a potential emergency response. Means to optimize potential countermeasure assessment, including responsible and effective vaccine candidate testing are discussed.
1 Introduction
Tularemia is an acute febrile zoonotic disease caused by infection with Francisella tularensis (Ft) (Snowden and Simonsen, 2023). Ft is a highly infectious gram negative, facultative intracellular bacteria capable of spread via multiple routes. Clinical tularemia manifestations depend on the site of infection, with ulceroglandular, glandular, oculoglandular, oropharyngeal, pneumonic, and typhoidal forms described (Saslaw et al., 1961a, Saslaw et al., 1961b; Samoilova et al., 1977; Adamovicz et al., 2006). Tularemia has long been associated with warfare, developed as a biological weapon by the Soviet Union and United States, among others (Alibek and Handleman, 2000). Factors favoring its use as a bioweapon include a very low infectious dose (Saslaw and Carhart, 1961), ease of aerosolization, multiple debilitating and potentially fatal clinical syndromes, suboptimal antibiotic countermeasures and lack of effective vaccines (Adamovicz et al., 2006).
Transmission of Ft can occur many ways, described in detail elsewhere (Hepburn et al., 2018): direct contact (e.g. dressing and eating wild rabbits and wild prairie dogs kept as pets), ingestion of food and water contaminated by infected animals, bites from infected arthropods or mammals during outdoor activities, and by aerosol transmission (e.g. aerolization of infected rabbits by lawn mowing equipment). Naturally occurring tularemia outbreaks have historically risen substantially in wartime conditions. A small number of well-described examples are found the English language scientific literature. A large outbreak of tularemia occurred during the 1942 Siege of Stalingrad (Croddy and Krcalova, 2001). Tularemia outbreaks were observed following the war in Kosovo from 1998-99. After the war, a surveillance system was implemented. Within 2 months, a cluster of tularemia was identified. Case control and environmental studies were published in 2001 (Reintjes et al., 2002). In a two-month period, over 247 tularemia cases were confirmed by serology. Ft was detected in rodents, rodent fecal specimens, and well water. Studies determined that households with rodent feces in food storage, large numbers of rodents near the house, and drinking water from piped water or personal wells were at higher risk for tularemia. Homes with water sources protected from rodents were at lower risk for tularemia. A second outbreak of tularemia was described from 2001 to 2002, with 327 tularemia cases confirmed by ELISA and Western blot (Grunow et al., 2012). Recently in the Russo-Ukraine war, news outlets are reporting on “mouse fever”, a disease associated with Russian soldiers and mice (Hart, 2023).
To understand the risk, considerably more information is in the eastern European and Russian literature. Available Russian language literature shows the Soviets were well-aware of conditions leading to tularemia outbreaks in eastern Europe. Olsuf’yev and Rudnev’s 1960 book on tularemia is available in English, covering history, animal experiments, epidemiology, immunology, vaccine prophylaxis, and environmental measurement of tularemia in the USSR (Olsuf’yev and Rudnev, 1962). Olsuf’yev and Rudnev describe how severe epizootic outbreaks of tularemia were observed when Germany occupied Soviet territories:
“The increase of recorded attacks was related not only to the improved diagnosis of tularemia1, but also to an intensification of the epizootics among the rodents due to their mass multiplication. During the war in particular massive tularemia epizootics were observed in the territories temporarily occupied by the German invaders, e.g., in the Ukraine, the Stalingrad and Rostov oblasts, etc. and also in the oblasts of the forest-steppe zone of the European part of the USSR, where the agriculture work was interrupted by the war operations (Tula, Voronezh, Riazan, Orlov and Kursk oblasts and others). Tularemia was (also) observed in the contending armies, the German doctors, not acquainted with the disease, first diagnosing it as Volhynian fever (Volkov, 1944 and others). Reports on a high incidence of tularemia made by Jusatz (1952), Trautmann (1953) and others”.
Olsuf’yev and Rudnev continue their epidemiology history, pointing out that the non-Soviet (particularly United States) experience was that individuals were infected in nature, particularly by hares or rabbits, from hunting. Modern literature agrees, as tularemia incidence continues to be associated with outdoor activities and tick bites (Juckett, 2015) and even theories of landscapers running over infected rabbits with lawn mowers to aerosolize Ft (Agger, 2005). In contrast, Olsuf’yev and Rudnev state:
“In the USSR the main sources of infection are water rats and small mouse-like rodents, from which people are infected under the most diverse conditions”.
Olsuf’yev and Rudnev describe numerous outbreaks of tularemia in the USSR under varying conditions. They describe water well, agricultural and domestic tularemia outbreaks associated with WWII with increases in rodent populations. Outbreaks were often linked to delays in harvesting crops, leading to rodent population explosions. Rodents would subsequently infect water supplies and homes, including food storage areas. The rodents would be caught up in delayed crop harvesting activities during the threshing of wheat, further disseminating tularemia. In one instance, Olsuf’yev and Rudnev describe:
“The mass multiplication of mouse-like rodents, which reached the scale of a ‘mouse invasion’, and the tremendous number of individuals among them infected with tularemia created an exceedingly high concentration of the tularemia microbe in the focus, which was spread out over a large territory. Food products – bread, flour, groats, grain, vegetables, fruit, nuts, etc. – as well as drinking water were contaminated by the excretions of the rodents and became routes of transmission of the tularemia infection”.
Olsuf’yev and Rudnev also describe links to wartime conditions:
“During the years of the Second World War, when in a number of oblasts, particularly those under temporary occupation, disorders in the management of agriculture led to the mass appearance of mouse-like rodents and the development of an active tularemia epizootic among them, agricultural, domestic, and water-borne outbreaks were predominant, distinguished by their mass nature and the variety of routes of transmission of this infectious disease. The characteristic features of wartime and the unique way of life of the population during this period led to the occurrence of a special type of outbreak which we called ‘trench’. These outbreaks were distinguished by certain rules and regulations in the spread of infection and required special prophylactic measures”.
“A particularly difficult test was given to Soviet epidemiologists during the years of the Second World War, when under conditions of the situation at the front as well as on territories liberated from enemy occupation, large outbreaks of tularemia of an unusual epidemiology were encountered and epidemiologists has to take extensive measures for eliminating them”.
Recent history from Kosovo combined with WWII-era Soviet Union history show a set of wartime events increase risk of tularemia outbreaks (Figure 1).
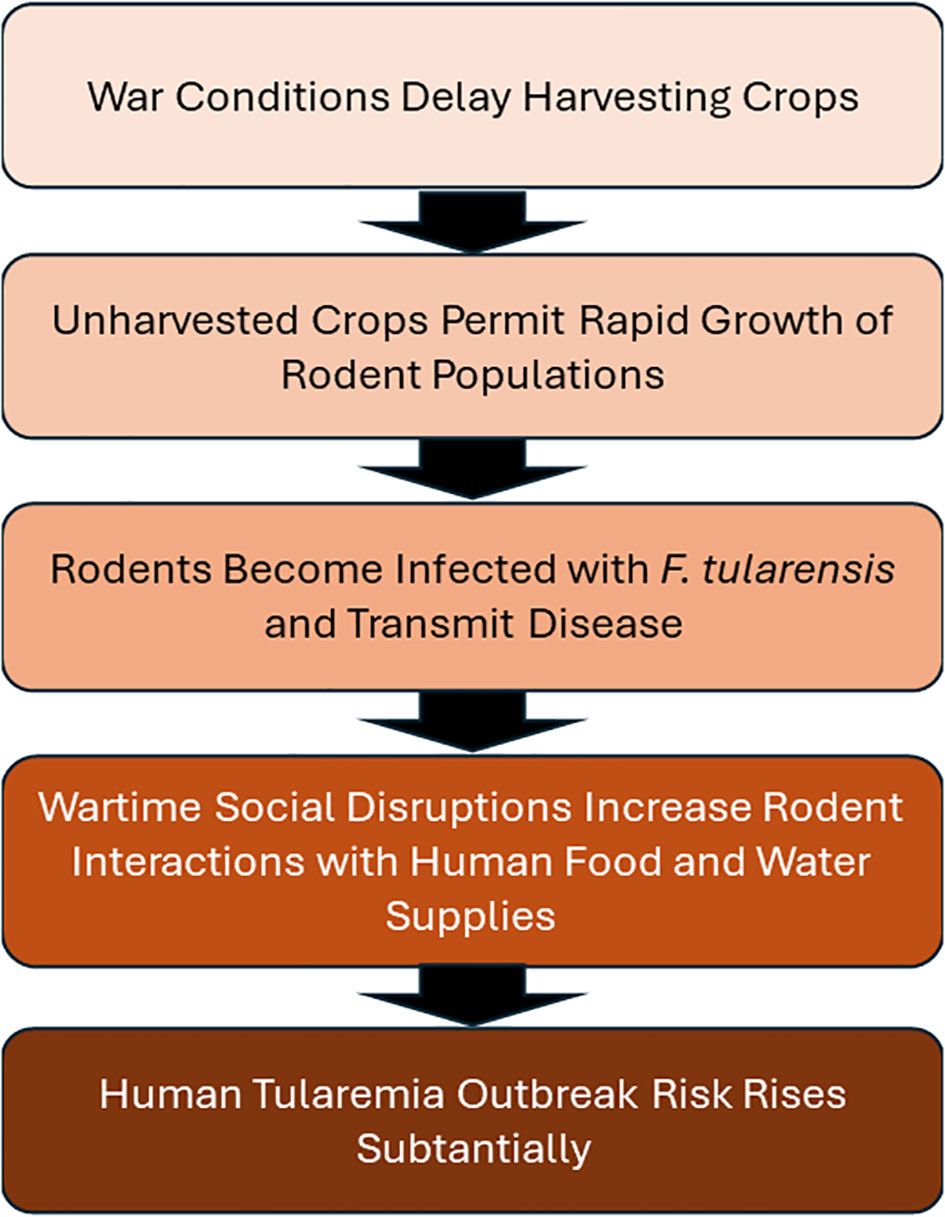
Figure 1 A set of sequential wartime events which lead to increased risk of tularemia outbreaks. Five events have been described which occur sequentially under wartime conditions in Eastern Europe, which have historically progressed to tularemia outbreaks.
2 The current situation in Ukraine
The Ukraine war has continued for nearly two years with more than 8,895 civilians officially reported killed, and vastly larger number of military deaths (USAID, 2023). Actual numbers of military and civilian deaths are likely far higher than reported. The over 600-mile current front line represents the largest land battle in Europe since WWII. The resulting destruction and infrastructure loss greatly increases tularemia outbreak risk. The current military stalemate also raises the possibility of deliberate use of bioweapons, including tularemia, perhaps under the guise of a naturally occurring outbreak.
The first risk factor for an outbreak is delayed crop harvesting. NASA satellite images show much of the Ukrainian winter 2022 harvest occurred, with a critically important exception: the band of harvest not collected along the battle front (Mitkish and Voiland, 2022), reminding us of conditions reported in the 1950’s Russian literature. Unharvested crops resulted in rodent population explosions with subsequent increase in tularemia infections.
Regarding the second criteria, an explosion in the rodent population, we have been in communication with the National Scientific Center Institute of Experimental and Clinical Veterinary Medicine in Ukraine. Conditions weren’t favorable for rodent populations in early 2023, as unstable weather resulted in flooding of rodent burrows (obtained from https://dpss.gov.ua/en). However, recent news articles from the end of 2023 indicate the battlefront is dealing with “exceptional levels of mice and rats” (Vincent, 2023).
Evidence for the third risk factor - Ft in the rodent population – is found in reports of Ft detected in arthropods, rodents, water, and agricultural products (Jurtubaeva et al., 2022). This included numerous regions of endemicity in Ukraine including Chernihiv, Odessa, and Sumy Oblasts. Hightower et al. described the distribution of tularemia across Ukraine in a temporo-spatial model, identifying historical foci of tularemia in Kharkiv, Kherson, and Zaporizhzhia Oblasts (Hightower et al., 2014). From 2015—2018 in Kharkiv Oblast, 78 of 380 tick and rodent samples were Ft positive by PCR (Zlenko et al., 2020b). The presence of Ft in Ukraine has long been observed and includes current conflict areas.
For the fourth criteria, social disruptions in eastern Ukraine are evident. Many buildings have been destroyed and a great many people have been displaced. Kharkiv Oblast has some particular characteristics, having been occupied for many months by Russians and then recaptured by Ukrainians. At this time, we do not have evidence that rodents have invaded food and water supplies in Ukraine, however it is easy to conclude that this is occurring.
Therefore, it appears that Ukraine is at high risk of tularemia outbreaks, similar to other eastern European conflicts. Before the war, a case of tularemia was recently reported in the English literature to have occurred in Mykolaiv Oblast in 2018 (Zlenko et al., 2020a). There were more than 50 cases reported from neighboring countries in 2019 including Hungary (22), Slovenia (7) and Poland (21). There were two confirmed cases of tularemia identified in Ukraine in 2022 (personal communication). The current numbers are unknown, as the challenges of disease surveillance in conflict areas are well described (Tong et al., 2011; Ismail et al., 2016).
3 Considerations for responses to tularemia outbreaks in Ukraine
Given clear risks for a tularemia outbreak in Ukraine, there are important considerations for a coordinated response. Figure 2 illustrates a stepwise approaches to prevention and mitigation of tularemia outbreaks.
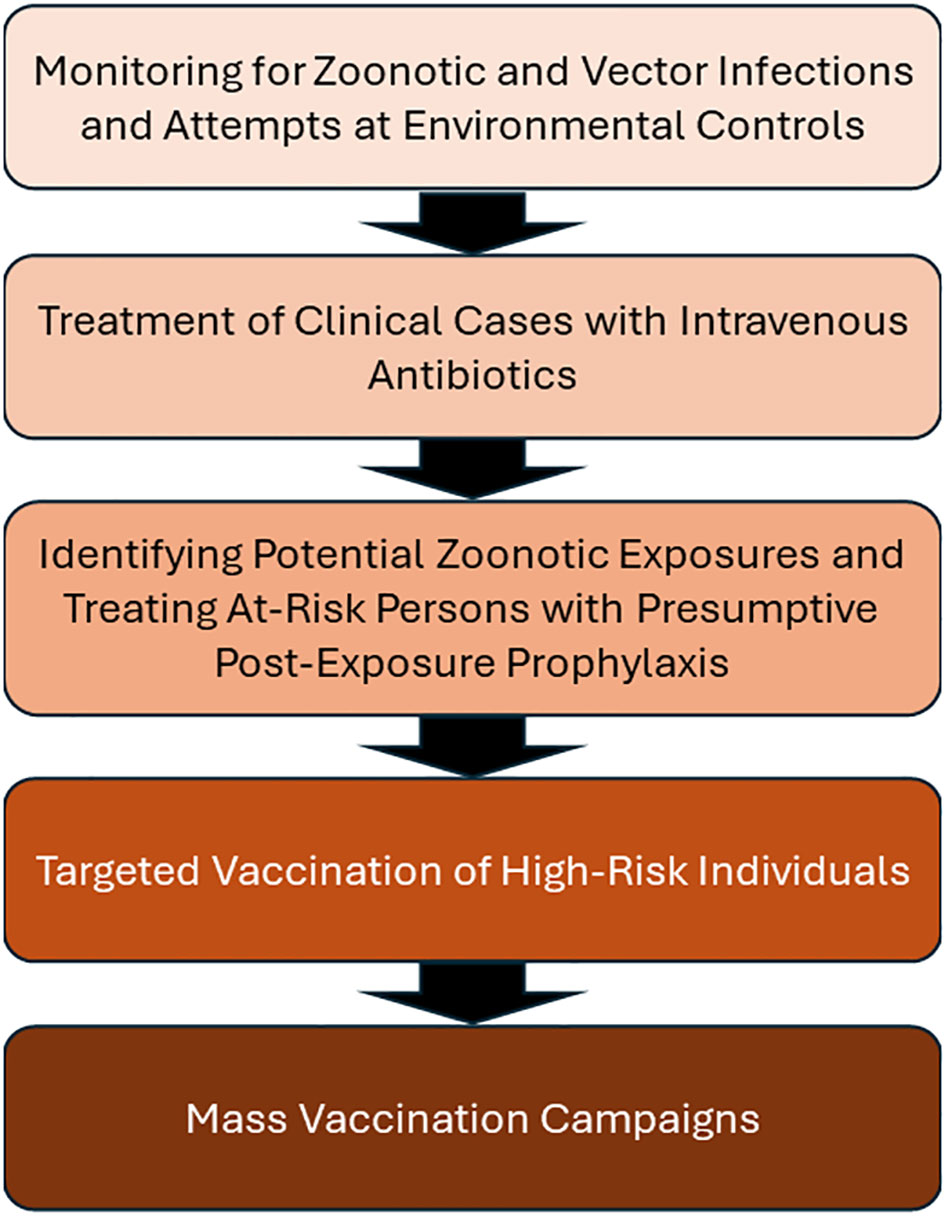
Figure 2 A stepwise approaches to prevention and mitigation of tularemia outbreaks. Here are five practices that may be used, in sequence from background surveillance to invasive mass vaccination. Note that wartime conditions, like those in Ukraine, may render earlier steps ineffective or impossible to implement. Under these conditions, implementation of vaccination may be needed.
The Kosovo outbreak response included improved sanitation and utilized animal control and surveillance (Grunow et al., 2012). This sound approach appears to be impractical under current Ukraine battlefield conditions. Vector monitoring, treatment, and post-exposure prophylaxis are also difficult under battlefield conditions.
The current Western approach to managing tularemia outbreaks focuses on approaches 1-3 through environmental monitoring, treatment and post-exposure prophylaxis. The Working Group on Civilian Biodefense published consensus-based recommendations (Dennis et al., 2001). The basis of the approach relied largely on the US and Swedish experience between 1966 and 2000 featuring sporadic outbreaks with small case numbers, primarily in hunters and workers in animal-based trades such as leather production.
The working group did not recommend vaccination outside of laboratory personnel. This may have been due to the lack of FDA approved vaccines. The working group did recommend post-exposure prophylaxis for at-risk patients using a two-week course of oral doxycycline or ciprofloxacin, and environmental decontamination of potentially exposed areas using 10% household bleach in water. While these recommendations are instructive, the Working Group envisioned a single biological weapon attack, rather than ongoing battlefield conditions favoring natural outbreaks. Descriptions of biological weapons scenarios have been described elsewhere (Wetter et al., 2002; Eliasson et al., 2006). A biological attack occurs in a defined time period, allowing antibiotic prophylaxis to also be for a definded time period, (e.g. two weeks). Extended antibiotic use is associated with antibiotic-resistance bacteria development (Endale et al., 2023). Therefore, individuals experiencing extended high infection risk (e.g. laboratorians) have been offered vaccines, but not antibiotic chemoprohylaxis. Antibiotics are reserved for treatment only. Furthermore, engineered antibiotic resistance is a concern for weaponized tularemia (Dennis et al., 2001). Chemoprophylaxis is not recommended for natural outbreaks (Stanek and Saunders, 2020), requiring alternatives like vaccines.
Vaccination with live, attenuated tularemia vaccine is known to be safe and effective. Perhaps the Soviet experience is most instructive. The Soviet Union produced a live attenuated vaccine for tularemia in use since 1942 known as the 15 NIIEG vaccine (aka, Gaiski, Gayskiy, Strain 15, or Gayskiy 15) (Timofeev et al., 2020) or Live Tularemia Vaccine (LTV). The LTV vaccine is reportedly effective 1-2 weeks after injection of 2x108 colony-forming units (cfu) by scarification or 1x107 cfu intradermally and requires a booster every 5 years (Feodorova et al., 2014). Olsuf’yev and Rudnev describe in detail how vaccinating people contained and eliminated tularemia outbreaks prior to 1960 in the USSR. These lessons could be applied in modern-day Ukraine.
Olsuf’yev and Rudnev report on the effectiveness of the LTV in preventing tularemia outbreaks in the post-WWII era as in this example:
“In one of the villages of Mikhaylovskiy Raion toward autumn a large number of mouse-like rodents appeared. In October a tularemia epizootic was found among them. Shortly after, cases of tularemia began to be recorded among people who had been in contact with agricultural products. During a period of five days the entire population of the village was inoculated with liquid yolk vaccine. During the first two weeks after the completion of vaccination only isolated cases of tularemia were found among the inoculees; after this time, not a single case of tularemia was detected. In the nearby inhabited places, where vaccination was not carried out in time tularemia was observed among people for a number of months afterwards”.
The Russian text describes over 80 cases in the first 5 days of vaccination as well as 20 cases in another place, rising to over 30 cases every 5 days for the next 25 days. Clearly, vaccination worked in this case. Olsuf’yev and Rudnev go on to describe measures to prevent, slow, or stop an outbreak. This begins with measuring rodent populations and identifying Ft in that population. Olsuf’yev and Rudnev indicate Ft in rodents is sufficient to vaccinate an entire rayon. Furthermore, any human case is indication for vaccination of the entire rayon. There are reports that the vaccination strategy using LTV was widely effective in the former Soviet Union, though documentation in the English literature remains scarce (Feodorova et al., 2014). Tularemia vaccinations in Ukraine, presumably with the LTV, stopped in 2000, suggesting most of the Ukrainian population are vulnerable to infection. Ukraine does not have access to the Soviet LTV, given the current military situation.
4 Discussion
Currently, the US does not have a tularemia vaccine in active production and no tularemia vaccine has been licensed by the US Food and Drug Administration. Historically, the Ft strains used to produce LTV were gifted to experts in the US during the mid-1950s, leading to development of an experimental vaccine known as the Live Vaccine Strain or LVS (Saunders et al., 2023). In repeated clinical trials, the vaccine was found to be safe and effective (Mulligan et al., 2017). Despite more than 70 years of clinical development and use protecting laboratory workers under an Investigational New Drug application, the vaccine remains unlicensed (Saunders et al., 2023). A replacement clinical candidate vaccine has been slow to develop, despite numerous preclinical candidates investigated (Sunagar et al., 2016). A search of clinicaltrials.gov reveals there are currently no registered tularemia vaccine candidates in trials at the time of writing.
There are currently a few active tularemia vaccine development efforts, many with live-attenuated vaccine candidates (Sunagar et al., 2016). Currently a live, attenuated tularemia vaccine (ATI-1701) appears to be the most advanced among these. ATI-1701 was created by a defined mutation in the clpB gene of the fully virulent Ft SCHU S4 strain, known as ΔclpB (Golovliov et al., 2013). It was shown to be less lethal in mice and guinea pigs than the legacy LVS vaccine (Conlan et al., 2021). The mutation was shown to be stable and growth methods for ΔclpB were scaled up to 25L. ΔclpB was lyophilized and was shown to be viable after 3 years of storage at -20°C and -80°C. Pilot cGMP manufacturing of ΔclpB is already underway, though the vaccine has yet to enter clinical trials. An effective vaccine would be of great value to Ukraine as well as the US and partner nations. Licensure of a tularemia vaccine will be time-consuming and require use of the FDA Animal Rule, given tularemia’s rarity in the west (Hickman et al., 2022).
One possibility to rapidly field and test candidate vaccines would be a monitored emergency use of unregistered and experimental interventions (MEURI) trial. The WHO has put forth a framework for MEURI trials (WHO, 2022). The Ukrainian tularemia outbreak risk of a public health emergency supports a MEURI approach to testing novel vaccine candidates. MEURI trials should have a strong and compelling justification, an ethical and regulatory framework, a consent process, and contribute to evidence supporting licensure and fielding of interventions. Justifications include risks for or an ongoing public health emergency, the absence of proven interventions, the impossibility of initiating research in a timely fashion under existing regulatory authority, scientific support based on a favorable risk-benefit analysis, and effective use of resources. Experience with Ebola demonstrated rapidly fielding and testing experimental drugs and vaccines in austere environments under the MUERI framework. We participated 2014 to 2016 Ebola outbreak reponse and the 2018 and 2019 Ebola outbreak response in the Democratic Republic of the Congo (DRC) (Iversen et al., 2020), including evaluation of mAb114, REGN-EB3, and remdesivir for the treatment of Ebola in DRC (Mulangu et al., 2019; Hickman et al., 2022). Our experience with Ebola outbreaks and MEURI trials leads us to believe that a MEURI trial of tularemia vaccines may be appropriate in Ukraine.
The tularemia countermeasures community should prepare to respond to a tularemia emergency in Ukraine with a plan to proactively study experimental vaccines. While tularemia does not have the high fatality rates or public health urgency associated with Ebola, the threat of both natural outbreaks and potential deliberate use by aerosolization should raise the level of urgency. The sporadic nature of natural tularemia transmission should not engender a sense of complacency. Credible vaccine candidates should be prepared urgently for testing in an outbreak setting, recalling the lessons of recent history.
Data availability statement
The original contributions presented in the study are included in the article/supplementary material. Further inquiries can be directed to the corresponding author.
Author’s note
The contents of this abstract are the sole responsibility of the authors and do not necessarily reflect the views, opinions, or policies of the Uniformed Services University of the Health Sciences; the Department of Defense.
Author contributions
DS: Conceptualization, Writing – original draft, Writing – review & editing. BP: Writing – original draft, Writing – review & editing. OZ: Investigation, Writing – original draft, Writing – review & editing. HG: Conceptualization, Funding acquisition, Writing – original draft, Writing – review & editing.
Funding
The author(s) declare financial support was received for the research, authorship, and/or publication of this article. This work was funded, in part, by United States Air Force Academy Cooperative Agreement number FA7000-23-2-0007.
Conflict of interest
HG is an employee of Appili Therapeutics USA Inc., which is developing a live attenuated tularemia vaccine, ATI-1701.
The remaining authors declare that the research was conducted in the absence of any commercial or financial relationships that could be construed as a potential conflict of interest.
Publisher’s note
All claims expressed in this article are solely those of the authors and do not necessarily represent those of their affiliated organizations, or those of the publisher, the editors and the reviewers. Any product that may be evaluated in this article, or claim that may be made by its manufacturer, is not guaranteed or endorsed by the publisher.
References
Adamovicz J. J., Wargo E. P., Waag D. M. (2006). “Tularemia,” in Biodefense Research Methodologies and Animal Models. Ed. Swearengen J. R. (Boca Raton, FL: CRC Press; Taylor & Francis Group), 137–162.
Agger W. A. (2005). Tularemia, lawn mowers, and rabbits’ nests. J. Clin. Microbiol. 43 (8), 4304; author reply 4304–4305. doi: 10.1128/JCM.43.8.4304-4305.2005
Alibek K., Handleman S. (2000). Biohazard: The Chilling True Story of the Largest Covert Biological Weapons Program in the World - Told from Inside by the Man Who Ran It (New York, NY: Delta).
Conlan J. W., Sjostedt A., Gelhaus H. C., Fleming P., McRae K., Cobb R. R., et al. (2021). Modern development and production of a new live attenuated bacterial vaccine, SCHU S4 deltaclpB, to prevent tularemia. Pathogens 10 (7). doi: 10.3390/pathogens10070795
Croddy E., Krcalova S. (2001). Tularemia, biological warfare, and the battle for Stalingrad, (1942-1943). Mil Med. 166 (10), 837–838. doi: 10.1093/milmed/166.10.837
Dennis D. T., Inglesby T. V., Henderson D. A., Bartlett J. G., Ascher M. S., Eitzen E., et al. (2001). Tularemia as a biological weapon: medical and public health management. JAMA 285 (21), 2763–2773. doi: 10.1001/jama.285.21.2763
Eliasson H., Broman T., Forsman M., Back E. (2006). Tularemia: current epidemiology and disease management. Infect. Dis. Clin. North Am. 20 (2), 289–311, ix. doi: 10.1016/j.idc.2006.03.002
Endale H., Mathewos M., Abdeta D. (2023). Potential causes of spread of antimicrobial resistance and preventive measures in one health perspective-A review. Infect. Drug Resist. 16, 7515–7545. doi: 10.2147/IDR.S428837
Feodorova V. A., Sayapina L. V., Corbel M. J., Motin V. L. (2014). Russian vaccines against especially dangerous bacterial pathogens. Emerg. Microbes Infect. 3 (12), e86. doi: 10.1038/emi.2014.82
Golovliov I., Twine S. M., Shen H., Sjostedt A., Conlan W. (2013). A DeltaclpB mutant of Francisella tularensis subspecies holarctica strain, FSC200, is a more effective live vaccine than F. tularensis LVS in a mouse respiratory challenge model of tularemia. PloS One 8 (11), e78671. doi: 10.1371/journal.pone.0078671
Grunow R., Kalaveshi A., Kuhn A., Mulliqi-Osmani G., Ramadani N. (2012). Surveillance of tularaemia in Kosovo 2001 to 2010. Euro Surveill 17 (28). doi: 10.2807/ese.17.28.20217-en
Hart R. (2023). Mouse Fever: What to Know About The Virus “Mowing Down” Putin’s Soldiers Fighting In Ukraine (Jersey City, NJ: Forbes). Available at: https://www.forbes.com/sites/roberthart/2023/12/21/mouse-fever-what-to-know-about-the-virus-mowing-down-putins-soldiers-fighting-in-Ukraine/?sh=14e755d03e5d.
Hepburn M. J., Kijek T. M., Sammons-Jackson W., Friedlander A. M. (2018). “Tularemia,” in Textbooks of Military Medicine: Medical Aspects of Biological Warfare. Eds. Bozue J., Cote C. K., Glass P. J. (Ft. Sam Houston, TX: Borden Institute).
Hickman M. R., Saunders D. L., Bigger C. A., Kane C. D., Iversen P. L. (2022). The development of broad-spectrum antiviral medical countermeasures to treat viral hemorrhagic fevers caused by natural or weaponized virus infections. PloS Negl. Trop. Dis. 16 (3), e0010220. doi: 10.1371/journal.pntd.0010220
Hightower J., Kracalik I. T., Vydayko N., Goodin D., Glass G., Blackburn J. K. (2014). Historical distribution and host-vector diversity of Francisella tularensis, the causative agent of tularemia, in Ukraine. Parasit Vectors 7, 453. doi: 10.1186/s13071-014-0453-2
Ismail S. A., Abbara A., Collin S. M., Orcutt M., Coutts A. P., Maziak W., et al. (2016). Communicable disease surveillance and control in the context of conflict and mass displacement in Syria. Int. J. Infect. Dis. 47, 15–22. doi: 10.1016/j.ijid.2016.05.011
Iversen P. L., Kane C. D., Zeng X., Panchal R. G., Warren T. K., Radoshitzky S. R., et al. (2020). Recent successes in therapeutics for Ebola virus disease: no time for complacency. Lancet Infect. Dis. 20 (9), e231–e237. doi: 10.1016/S1473-3099(20)30282-6
Juckett G. (2015). Arthropod-borne diseases: the Camper’s uninvited guests. Microbiol. Spectr. 3 (4). doi: 10.1128/microbiolspec.IOL5-0001-2014
Jurtubaeva G., Savchuk A., Kozishkurt O., Gerasimenko O., Gaidei V., Kostolonova L. (2022). Epidemic process of tularemia in the world and in the Ukraine. J. Education Health Sport 12 (1), 503–513. doi: 10.12775/JEHS.2022.12.01.042
Mitkish M., Voiland A. (2022). Larger Wheat Harvest in Ukraine Than Expected (Greenbelt, MD: N. E. Observatory). Available at: https://earthobservatory.nasa.gov/images/150590/larger-wheat-harvest-in-Ukraine-than-expected.
Mulangu S., Dodd L. E., Davey R. T. Jr., Tshiani Mbaya O., Proschan M., Mukadi D., et al. (2019). A randomized, controlled trial of Ebola virus disease therapeutics. N Engl. J. Med. 381 (24), 2293–2303. doi: 10.1056/NEJMoa1910993
Mulligan M. J., Stapleton J. T., Keitel W. A., Frey S. E., Chen W. H., Rouphael N., et al. (2017). Tularemia vaccine: Safety, reactogenicity, “Take” skin reactions, and antibody responses following vaccination with a new lot of the Francisella tularensis live vaccine strain - A phase 2 randomized clinical Trial. Vaccine 35 (36), 4730–4737. doi: 10.1016/j.vaccine.2017.07.024
Olsuf’yev N. G., Rudnev G. P. (1962). “Tulyaremia,” in Army Translation 662 (Fort Detrick, MD: Department of the Army).
Reintjes R., Dedushaj I., Gjini A., Jorgensen T. R., Cotter B., Lieftucht A., et al. (2002). Tularemia outbreak investigation in Kosovo: case control and environmental studies. Emerg. Infect. Dis. 8 (1), 69–73. doi: 10.3201/eid0801.010131
Samoilova L. V., Vasenin A. S., Piontkovskii S. A., Plotnikova E. A. (1977). [Experimental study of the pulmonary form of plague, tularemia and pseudotuberculosis]. Zh Mikrobiol Epidemiol. Immunobiol (3), 110–114.
Saslaw S., Carhart S. (1961). Studies with tularemia vaccines in volunteers. III. Serologic aspects following intracutaneous or respiratory challenge in both vaccinated and nonvaccinated volunteers. Am. J. Med. Sci. 241, 689–699. doi: 10.1097/00000441-196106000-00001
Saslaw S., Eigelsbach H. T., Prior J. A., Wilson H. E., Carhart S. (1961a). Tularemia vaccine study. II. Respiratory challenge. Arch. Intern. Med. 107, 702–714. doi: 10.1001/archinte.1961.03620050068007
Saslaw S., Eigelsbach H. T., Wilson H. E., Prior J. A., Carhart S. (1961b). Tularemia vaccine study. I. Intracutaneous challenge. Arch. Intern. Med. 107, 689–701. doi: 10.1001/archinte.1961.03620050055006
Saunders D. L., Pierson B. C., Haller J., Norris S. L., Cardile A. P., Reisler R. B., et al. (2023). Longitudinal phase 2 clinical trials of live, attenuated tularemia vaccine in otherwise healthy research laboratory workers operating in containment laboratories. medRxiv. doi: 10.1101/2023.01.09.23284371
Snowden J., Simonsen K. A. (2023). “Tularemia,” in StatPearls. (Treasure Island (FL): StatPearls Publishing). Available at: https://www.ncbi.nlm.nih.gov/books/NBK430905/.
Stanek S. M., Saunders D. L. (2020). “Tularemia,” in USAMRIID’s Medical Management of Biological Casualties Handbook. Eds. Stanek S. M., Saunders D. L. (Washington, DC: U.S. Government Printing Office).
Sunagar R., Kumar S., Franz B. J., Gosselin E. J. (2016). Tularemia vaccine development: paralysis or progress? Vaccine (Auckl) 6, 9–23. doi: 10.2147/VDT.S85545
Timofeev V., Titareva G., Bahtejeva I., Kombarova T., Kravchenko T., Mokrievich A., et al. (2020). The Comparative Virulence of Francisella tularensis Subsp. mediasiatica for Vaccinated Laboratory Animals. Microorganisms 8 (9). doi: 10.3390/microorganisms8091403
Tong J., Valverde O., Mahoudeau C., Yun O., Chappuis F. (2011). Challenges of controlling sleeping sickness in areas of violent conflict: experience in the Democratic Republic of Congo. Confl Health 5, 7. doi: 10.1186/1752-1505-5-7
USAID (2023). Fact Sheet 16. Ukraine - Complex Emergency, Vol. 16 (Washington, DC: United States Agency for International Development).
Vincent I. (2023). ‘Exceptional levels’ of rats and mice invade trenches on Ukraine’s front lines (New York: New York Post). Available at: https://nypost.com/2023/12/23/news/troops-in-Ukraine-hampered-by-exceptional-levels-of-rats/.
Wetter W. A., Silvers M. J., Humbaugh K. E. (2002). “Community response and testing of teh national pharmaceutical stockpile in a metropolitan area,” in 130th Annual Meeting of APHA, Philadelphia, PA.
WHO. (2022). Emergency use of unproven clinical interventions outside clinical trials: ethical considerations. Geneva: World Health Organization. Licence: CC BY-NC-SA 3.0 IGO.
Zlenko O. B., Ignatenkov O. S., Vinokurova K. V., Gerilovych A. P. (2020a). Case report on the human infection with tularemia in Mykolaiv region 2018. J. Vet Med Biotechnol. Biosafety 6 (1), 15–17. doi: 10.36016/JVMBBS-2020-6-1-3
Keywords: vaccine, MUERI, battlefield, mice, Europe
Citation: Saunders DL, Pierson BC, Zlenko O and Gelhaus HC (2024) Considerations for prevention of and emergency response to tularemia outbreaks in Ukraine: vaccine involvement. Front. Bacteriol. 3:1364708. doi: 10.3389/fbrio.2024.1364708
Received: 02 January 2024; Accepted: 17 January 2024;
Published: 02 February 2024.
Edited by:
Naim Deniz Ayaz, Kırıkkale University, TürkiyeReviewed by:
Max Maurin, Centre Hospitalier Universitaire de Grenoble, FranceThomas Robert Laws, Defence Science and Technology Laboratory, United Kingdom
Copyright © 2024 Saunders, Pierson, Zlenko and Gelhaus. This is an open-access article distributed under the terms of the Creative Commons Attribution License (CC BY). The use, distribution or reproduction in other forums is permitted, provided the original author(s) and the copyright owner(s) are credited and that the original publication in this journal is cited, in accordance with accepted academic practice. No use, distribution or reproduction is permitted which does not comply with these terms.
*Correspondence: H. Carl Gelhaus, cgelhaus@appilitherapeutics.com