- 1Division of Maternal Fetal Medicine, UConn Health, Farmington, CT, United States
- 2Division of Maternal Fetal Medicine, Hartford Hospital, Hartford, CT, United States
- 3Department of Periodontology, University of Connecticut School of Dental Medicine, UConn Health, Farmington, CT, United States
- 4Connecticut Convergence Institute for Translation in Regenerative Engineering, Department of Public Health Sciences, UConn Health, Farmington, CT, United States
- 5Department of Medicine, UConn Health, Farmington, CT, United States
- 6Microbial Analysis Research Services, University of Connecticut, Storrs, CT, United States
Preeclampsia (PE) is a leading cause of morbidity and mortality in pregnancy with elusive etiology. Patients with PE are thought to be associated with a higher rate of periodontal diseases (PDs) and changes of oral bacteria with targeted PCR techniques. However, few studies have investigated the associations between oral microbiome dysbiosis and secondarily disseminated microbes in the placenta simultaneously in patients with PE. The association between detected microbiome in placenta and systemic inflammation in PE is also unclear. We enrolled 54 pregnant patients with and without PE and PD, and profiled the subgingival and placenta microbiome by the V4 region of 16S rRNA gene sequencing. Systemic inflammatory markers tumor necrosis factor-alpha (TNF-α), C-reactive protein (CRP), lipopolysaccharide binding protein (LBP), and interleukins 6 and 8 (IL-6 and IL-8) in blood were measured by ELISA. We found that PD significantly increased the risk of PE after adjusting for age and smoking status (OR = 2.26, 95% CI = 1.14–4.48, p = 0.024). A combined group of oral-associated bacteria Veillonella, Fusobacterium, Haemophilus, Granulicatella, Streptococcus, Gemella, and Neisseria in placenta had a significantly higher prevalence in women with PE compared to women without PE (53.8% vs. 19.0%, p = 0.018), with the highest prevalence in patients with both PE and PD (58.8%). The relative abundance of Haemophilus, Veillonella, and Fusobacterium in subgingival samples was significantly higher in patients with PE than those without PE. The relative abundance of Haemophilus in subgingival samples was associated with increased risk of PE (OR = 2.11, 95% CI = 1.11–4.52, p = 0.032). Proinflammatory cytokine analysis showed that PE patients with PD had higher blood IL-8 levels than PE patients without PD (p = 0.026). CRP, LBP, and TNF-α showed no statistical difference in patients with and without PE or PD. Blood IL-6 levels were significantly higher in patients with detectable placenta microbiome compared to those without placenta microbiome (p = 0.028). Together, our data suggest a potential oral origin of the placental microbiota present in patients with PE, and the microbiota detected in placenta is associated with increased IL-6 level in the blood.
Introduction
Preeclampsia (PE) is a leading cause of morbidity and mortality in pregnancy, complicating approximately 2%–5% of pregnancies (Gestational hypertension and preeclampsia, 2020; Mustafa et al., 2012). The etiology of PE remains unclear. The placenta has been considered as a central organ in the pathogenesis of PE.
Periodontal disease (PD) has been shown to be positively correlated with PE. The prevalence of PD among pregnant women is approximately 40% with a higher prevalence of 60%–70% among racial and ethnic minorities (Lieff et al., 2004; Eke et al., 2012; Azofeifa et al., 2016). PD-associated bacteria can potentiate an aggressive inflammatory response, even in extra-oral sites (Krohn et al., 1993; Offenbacher et al., 2008). Hematogenous spread of the oral bacteria to the placenta was theorized as an important source of amniotic fluid and placenta microbiome. It is possible that oral pathogens can transmit to the blood and disseminate to the placenta where the blood flow is slow. Indeed, oral bacteria Porphyromonas gingivalis and Actinobacillus actinomycetemcomitans have been detected in amniotic fluid of pregnant women with periodontitis (Esra et al., 2013), and reported to be associated with placental infections in hypertensive women (Swati et al., 2012). A study showed patients with PE had higher levels of A. actinomycetemcomitans, Fusobacterium nucleatum spp., P. gingivalis, Prevotella intermedia, Tannerella forsythensis, and Treponema denticola in the placenta (Barak et al., 2007; Amarasekara et al., 2015). However, the study had a small sample size and detected a limited number of PD-associated bacteria in placenta using a targeted PCR approach. To date, there is a lack of non-targeted global characterization of placenta microbiome and oral microbiome simultaneously in the same patients to track the origin of microbiome detected in the placenta of patients with PE. Furthermore, the relationship between placenta microbiome and systemic inflammation in PE is unclear.
To answer such unresolved questions, we prospectively enrolled patients according to their maternal diagnosis at the time of admission: preeclampsia/periodontal disease (PE+/PD+), preeclampsia/no periodontal disease (PE+PD−), no preeclampsia/periodontal disease (PE−/PD+), and no preeclampsia/no periodontal disease (PE−/PD−). We determined that few bacteria on the placenta with a potential oral origin are associated with PE and heightened systemic inflammatory responses. We also identified specific oral microbiota that were associated with an increased risk of PE. Outcomes from this study will help strengthen our understanding of the pathogenesis of PE and offer a novel preventive or treatment strategy for hypertensive disorders in pregnancy.
Materials and methods
Study participants
We conducted a prospective, cross-sectional cohort study (Supplementary Figure 1). The study was conducted at two academic centers from October 2019 to December 2020. Both hospitals are considered high-volume maternal referral centers. The protocol was approved by the institutional review boards at both sites (IRB #: 19-140H-1). Pregnant women admitted to the maternal unit for delivery were approached regarding participation in the study. Women were eligible if they were 18–50 years old, they carried a singleton gestation, and gestational age was greater than 28 weeks. Exclusion criteria were pregestational or gestational diabetes, pre-existing chronic hypertension, known or diagnosed HIV infection in pregnancy, hepatitis B or C positive status, inflammatory bowel disease, major fetal anomalies, renal disease, chronic antibiotic use in pregnancy, chronic steroid use (≥2 months use), multiple gestation, and inability to collect specimens within 72 h after birth. Owing to the possibility that labor may influence risk of infection and concern for bacterial contamination of the placenta, all cesarean deliveries for this study were confined to women who underwent elective cesarean delivery prior to undergoing spontaneous labor.
PE was defined as new-onset hypertension: BP ≥140 mmHg systolic or ≥90 mmHg diastolic (sustained on two occasions at least 6 h apart) in the presence or absence of proteinuria (at least 300 mg per 24 h, a score of 0.3 on protein/creatinine ratio during the study period (Gestational hypertension and preeclampsia, 2020).
Serum blood collection: Blood was collected on admission. The samples were centrifuged and serum was stored at −80°C for cytokine analysis (Supplementary Figure 2).
Placenta specimen collection: At the time of delivery, the placenta was placed in a sterile container and immediately handed off to trained study personnel who wore facial masks and used sterile gloves, scalpel, and tissue forceps (Supplementary Figure 2). Two 1-cm × 1-cm × 1-cm cuboidal sections were circumferentially excised under the surface area of the placenta from specified areas (chorionic plate to basal plate); one area was located 4 cm proximal to cord insertion and one area was located 4 cm from the placental edge site (Faul et al., 2007; Aagaard et al., 2014). The specimens were stored at −80°C for microbiome assessment.
Oral cavity specimen collection. All patients were evaluated for the presence or absence of PD by a trained periodontist. The dental exam was a full mouth periodontal examination, with positioning of a periodontal probe parallel to the long axis of the tooth at each site (Chapple et al., 2018). The exam was performed on postpartum day 1 (Supplementary Figure 2). Measurements were taken at six sites around each tooth, namely, mesiobuccal, midbuccal, distobuccal, mesiolingual, midlingual, and distolingual on postpartum day 1 (Eke et al., 2018). The dental provider was blinded to all sample groups to decrease the risk of selection bias. The following parameters were evaluated: periodontal probing depth (PPD), bleeding on probing (Bop), Recession—measured (when present) from the cement–enamel junction (CEJ) to free gingival margin, and clinical attachment loss (CAL) (Lang et al., 1990).
PD was defined as the dental exam finding ≥2 non-adjacent interproximal sites with CAL ≥3 mm, and ≥2 non-adjacent interproximal sites with PPD ≥ 4 mm, or ≥1 site with PPD ≥5 mm (Eke et al., 2018). For the purpose of this study, severe periodontitis was defined as ≥2 interproximal sites with CAL ≥6 mm and ≥1 interproximal site with PPD ≥5 mm. Non-severe periodontitis was categorized into moderate (≥2 interproximal sites with CAL ≥4 mm or ≥2 sites with PPD ≥5 mm) and mild ≥2 interproximal sites with CAL ≥3 mm and ≥2 interproximal sites PPD ≥4 mm or ≥1 site with PPD ≥5 mm. Subgingival dental plaque samples were collected by swiping the tooth surface with a dental explorer, and were placed in DNA Genotek media and stored at −80°C until sequencing.
DNA extraction, PCR amplification, and 16S rRNA gene sequencing
To determine the microbiome profile in placenta and subgingival plaque, we performed V4 regions of 16S rRNA gene sequencing (Maas and GitHub: Bioinformatics, [[NoYear]]). In brief, a maximum of 0.25 g of placenta and 200 μL of subgingival samples were used for DNA extraction using a Qiagen DNA mini kit for tissue and blood, according to the manufacturer’s protocol (The Human Microbiome Project Consortium, 2012). Genomic DNAs were amplified using primers targeting the V4 region of the 16S rRNA gene, followed by 2× 250-bp paired-end sequencing using the Illumina MiSeq sequencing platform. To control potential microbiome contaminations, negative controls including extraction controls and operation room controls were included for all sample extractions. The 16S rRNA gene sequences were processed with the DADA2 pipeline to generate amplicon sequence variants (ASVs) that represent the lowest taxonomy unit in 16S rRNA gene sequencing. Data processing included paired-end reads merging, denoising, identification and removal of chimeric sequences, and assigned sequences to bacterial taxonomies. Sequences detected in any negative control were removed from all samples. Bacterial taxonomic identification of ASVs was done using the RDP Bayesian classifier against the Silva nr_v119 taxonomy database (Wang et al., 2007; Quast et al., 2012). Sequences that were unidentified at the domain level and those identified as mitochondria were also removed. We employed rigorous procedures to remove potential placenta microbiome contamination from various sources as illustrated in Supplementary Figure 3.
Analysis of demographic and clinical data
Descriptive statistics were used for demographic and clinical information. We first summarized women by PD and PE status for continuous variables using mean and standard deviation; categorical variables were analyzed using frequencies and percentages (Table 1). The differences between the groups were tested using two-sample t-tests for continuous variables after proper transformation and Fisher’s exact tests for categorical variables. The prevalence of PD between women with and without preeclampsia was then determined by Chi-square testing. A logistic regression model was fitted to evaluate the associations between PE and PD, adjusting for age and smoking variables (Table 2).
Statistical analysis of placenta and oral microbiome
Descriptive analysis of the placenta microbiome includes prevalence and relative abundance of the placenta microbiome in each group. Prevalence refers to the proportion of participants who have a given taxon. Data in two placenta sites were combined to represent placenta microbiome for a given patient. Kruskal–Wallis test or Fisher’s exact test and Wilcox on sum rank test were used to test differences of the prevalence and relative abundance of the placenta microbiome across groups, respectively. Permutational multivariate analysis of variance (PERMANOVA) was performed to evaluate the global microbiome difference between two groups after accounting for potential confounding variables including age, ethnicity, smoking status, and history of PE (Kelly et al., 2015). Wilcox on sum rank testing and DESeq2 were performed to identify differential bacteria between groups (Corcoll et al., 2017). A logistic regression model was used to determine the risk of oral microbiome in the development of PE. p-values from multiple comparisons were adjusted using the false discovery approach. Adjusted p-value <0.05 was considered as statistically significant. All above statistical analyses of the microbiome were performed with R 3.3.2 (Vienna, Austria) software.
Cytokine analysis
Cytokine serum levels were determined using commercially available kits according to the manufacturer’s instructions. The cytokine serum levels, expressed as medium fluorescence intensity (MFI), were log transformed and compared among groups by using Kruskal–Wallis, followed by Dunn’s post-test where the p-value was adjusted for multiple testing using the Benjamini–Hochberg method.
Results
Study population
A total of 126 women were screened from October 2019 to December 2020. Seventy-two patients did not meet inclusion criteria, leaving 54 women eligible for the study (Supplementary Figure 1). Patient characteristics and demographics (PE and periodontal status) are presented in Tables 1, 2. A subgroup analysis was performed to evaluate the prevalence of PD among patients with and without PE (Supplementary Table 1). All clinical variables were comparable across four groups (PE+/PD+, PE+/PD−, PE−/PD+, and PE−/PD−) except that birth weight (p = 0.001) and gestational age (p = 0.008) were significantly lower in patients with PE. Interestingly, PD was confirmed in 19 (65.5%) women with PE compared to 7 (28%) women without PE (p = 0.007). Further logistic regression analysis showed that PD increased the risk of PE after adjustment for age, preterm delivery, and smoking status (OR = 2.26, 95% CI = 1.14–4.48, p = 0.024).
Track the oral origin of the placenta microbiome
Ninety-six out of 99 placental samples from 54 patients were successfully sequenced, resulting in 2,241 ASVs. DNA extraction and procedure room control accounted for a large proportion of sequenced reads of placenta (81.7%). After contamination removals from DNA extraction controls and procedure room controls, 2,140 ASVs were left (Supplementary Figure 3).
To address the potential microbial contamination during delivery, difference in placenta microbiome between cesarean and vaginal delivery were evaluated. The microbiome in placenta after removing extraction and procedure room controls was significantly different between cesarean and vaginal delivery by PERMANOVA (p < 0.05) (Supplementary Figure 4) suggesting that delivery mode contributes to the microbiota detected in placenta. Wilcox on sum rank test showed that vagina-related microbiome represented by Lactobacillus, Prevotella, Gardnerella, and Megasphaera and common stool microbiota represented by Bacteroides and Faecalibacterium were significantly higher among vaginal deliveries. Skin-related bacteria Bacilli were significantly higher in women who had cesarean deliveries. In total, ASVs from 67 genera noted to be different between delivery modes were removed from placenta microbiome, resulting in 1,482 ASVs in 96 placental samples.
Next, we filtered the placental microbiota by keeping ASVs identified in oral samples and with >1% of averaged abundance in oral samples (Supplementary Figure 4). The relative abundance of 1% was considered a biologically reliable or relevant cutoff for microbiome research in low biomass samples and was used for previous placenta microbiome studies (Eke et al., 2012; de Goffau et al., 2019). This yielded a final set of 23 ASVs from 10 genera including Streptococcus, Haemophilus, Veillonella, Fusobacterium, Neisseria, Granulicatella, Gemella, Actinomyces, Campylobacter, and Leptotrichia in placenta. The majority of these microbiota are well-known PD-associated bacteria. Streptococcus was the most prevalent bacteria in placenta, accounting for 27.7% of patients in our cohort (13/47 = 27.7%), with the rest of nine genera each accounting for 2%–8% of total patients (Figure 1A).
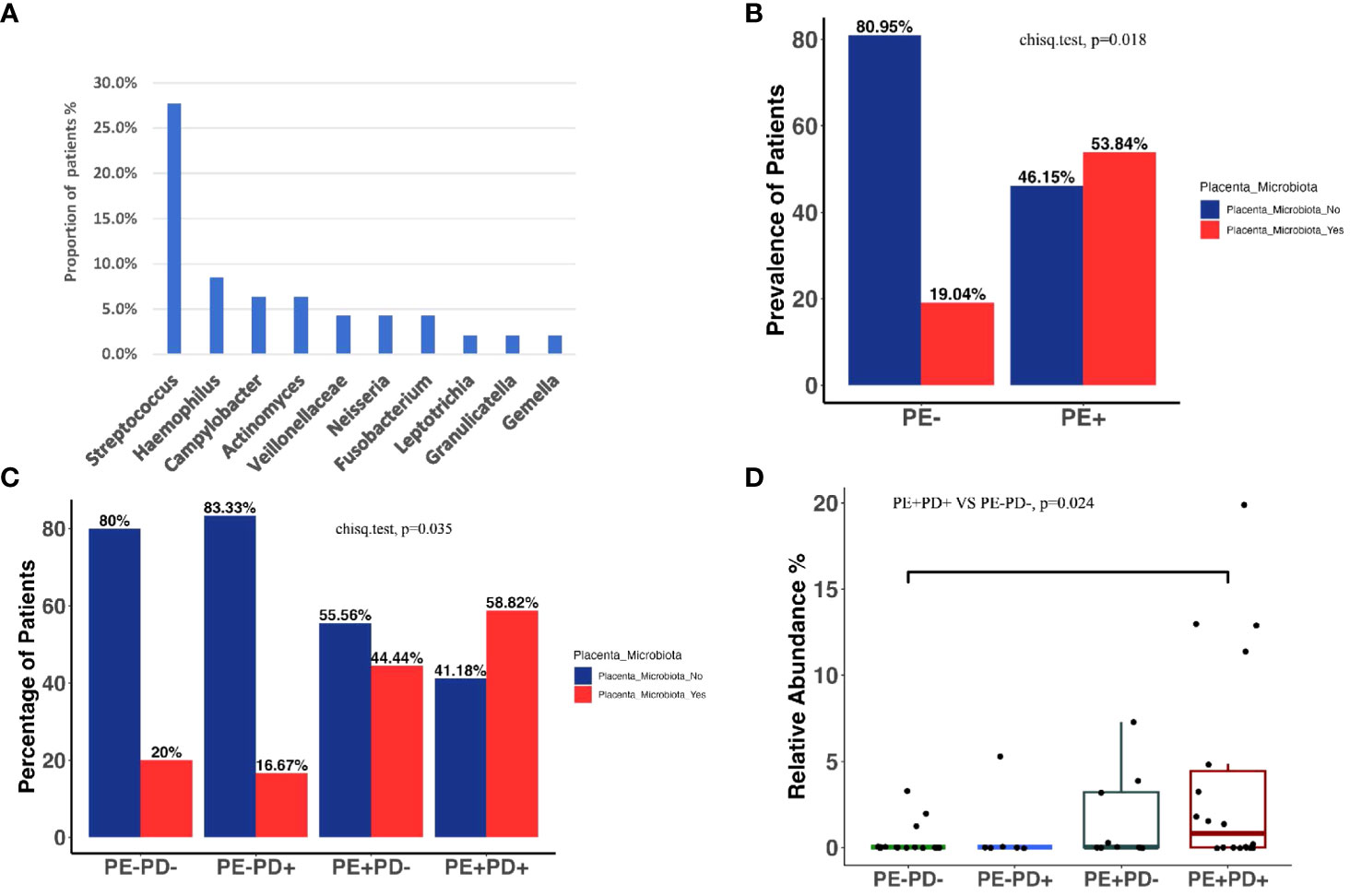
Figure 1 Prevalence of the placenta microbiome in different groups. Ten bacterial genera are identified in placenta after rigorous bioinformatic processing. Proportions of women who have a given detectable placental microbiota are illustrated by barplots (A). Prevalence of patients with and without placental microbiota among PE+ vs. PE− women (p = 0.018, Fisher exact test) (B). Prevalence of patients with and without placenta microbiota among four subgroups (p = 0.035, Fisher exact test) (C). The total relative abundance of placenta microbiome across four groups, with PE−PD− being significantly lower than PE+PD+ (p = 0.024, Wilcox on test) (D).
The placenta microbiome differs by PD and/or PE status
We analyzed the placenta microbiome in patients with and without PE (PE+ and PE−) and four subgroups: PE+/PD+, PE+PD−, PE−/PD+, and PE−/PD−. We did not find overall difference in alpha and beta diversity of the microbiome detected in the placenta based on PE and PD status (Supplementary Figure 5). Among 10 genera identified in placenta, 7 genera, namely, Veillonella, Fusobacterium, Haemophilus, Granulicatella, Streptococcus, Gemella, and Neisseria, were present in higher proportion in the PE+ group compared to the PE− group. However, none of the individual genus showed statistical difference in prevalence or abundance among PE+ and PE− groups or the four subgroups. This is likely due to different patients having different bacterial composition in their placenta microbiome. However, because different bacteria may confer similar function and the identified placenta microbiome were all associated with PD, we combined the prevalence or abundance of the seven bacteria genera and tested the differences of combined microbiome among groups. Interestingly, the prevalence of combined placenta microbiome in PE+ patients (14/26 = 53.84%) was significantly higher than that of PE− patients (4/21 = 19.04%) (p = 0.018) (Figure 1B). The combined placenta microbiome showed the highest prevalence in the PE+/PD+ group (10/17 = 58.82%), and it was significantly higher than the PE−/PD− group (3/15 = 20%) (p = 0.035). (Figure 1C). The abundance of combined placenta microbiome showed a significant difference in the PE−/PD− and PE+/PD+ groups (p = 0.024) (Figure 1D). Together, these data support our hypothesis that PD-related bacteria are high in prevalence and abundance in PE patients.
The subgingival microbiome differs by PE status
Thirty-five out of 48 oral samples were successfully sequenced and included in the analysis. After data processing, we totally yielded 2,087,144 reads, with a median of 51,382 and an IQR of 64,570. As expected, Streptococcus was the most abundant genus in subgingival samples (Supplementary Figure 6). Alpha diversity analysis did not find differences in richness and Shannon diversity between the PE+ and PE− groups and subgroups (Supplementary Figures 7A–D). PERMANOVA analysis showed that the global subgingival microbiome profile had a marginally significant difference between the PE+ and PE− groups (p = 0.09) and between the PE+PD+ and PE−PD− groups (p = 0.07) (Supplementary Figures 7E–G). Differential taxa analysis by DESeq2 showed that Haemophilus (p = 0.015), Veillonella (p = 0.020), and Fusobacterium (p = 0.046) had higher relative abundance in the PE+ than in the PE− groups (Figure 2A). The increase of Haemophilus in PE+ was confirmed by LefSe analysis (Supplementary Figure 7H). Subgroup analysis showed that the relative abundance of Haemophilus and Fusobacterium was significantly higher in PE+PD+ than in PE−PD+ patients (Figure 2B), indicating that these two bacteria may be specific to PE. Importantly, logistic regression analysis showed that Haemophilus but not Veillonella and Fusobacterium in subgingivae was associated with increased risk of PE (OR = 2.11, 95% CI = 1.11–4.52, p = 0.032). This raises the possibility that the oral microbiome may potentially serve as a marker for PE.
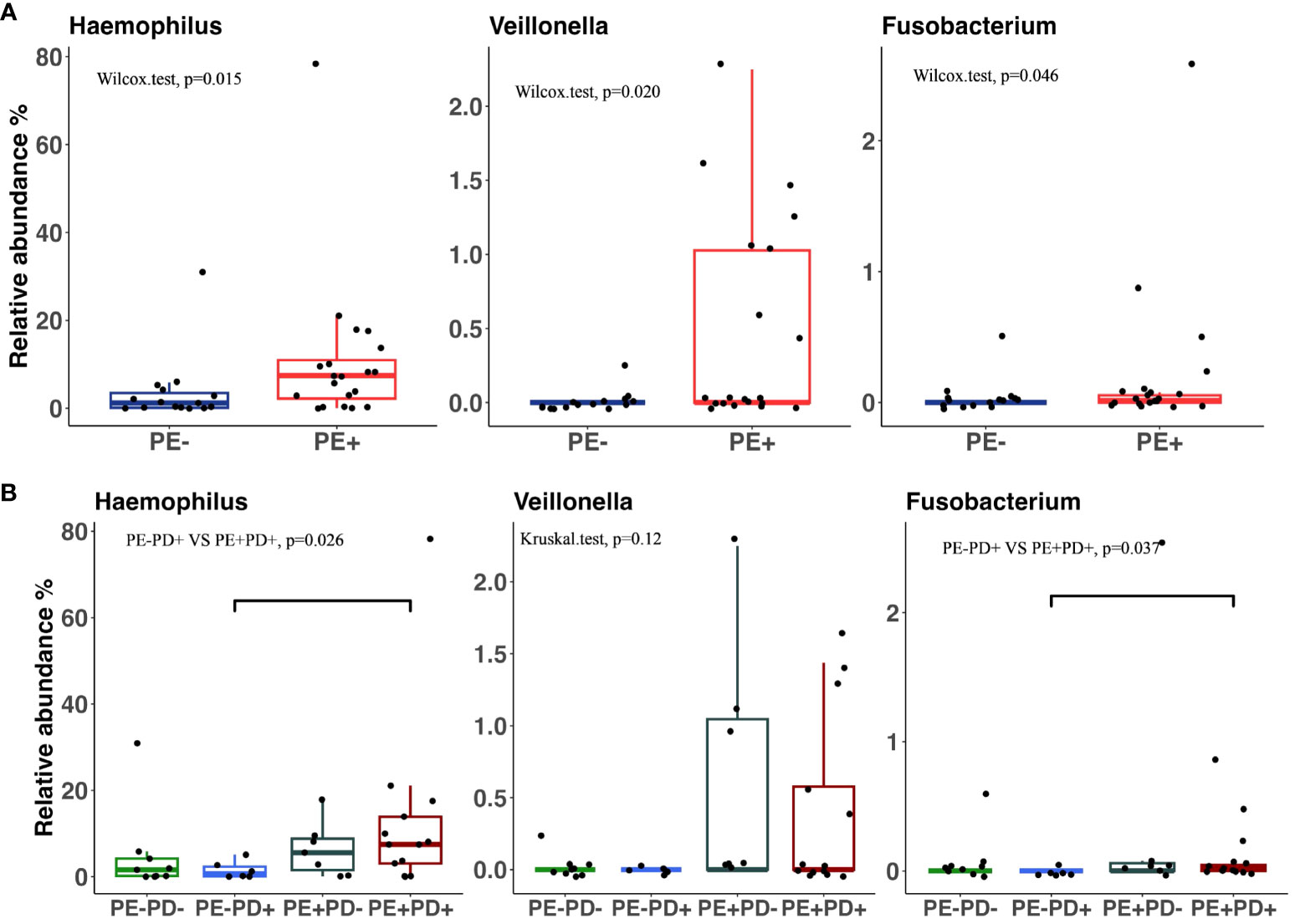
Figure 2 Relative abundance of three microbiota in subgingival samples that are significantly different between PE+ and PE− patients (A) and among four subgroups (B).
Associations between systemic inflammation and placenta microbiome
The blood levels of CRP, LBP, TNF-α, and IL-6 showed no statistical difference in patients with or without PE or PD (Supplementary Tables 2, 3) even after stratification for term deliveries with Kruskal–Wallis. However, IL-8 levels were significantly higher in the PE+/PD+ group compared to the PE+/PD− group (p = 0.26, Figure 3A). To examine the relationship between proinflammatory cytokine and placenta microbiome, we compared cytokine levels in patients who had a detected and non-detected placenta microbiome. Interestingly, we found that there were elevated levels of blood IL-6 in placentas with detected microbiome than placentas without detected microbiome (p = 0.028, Figure 3B), indicating that the presence of placenta microbiome is associated with elevated systemic inflammation.
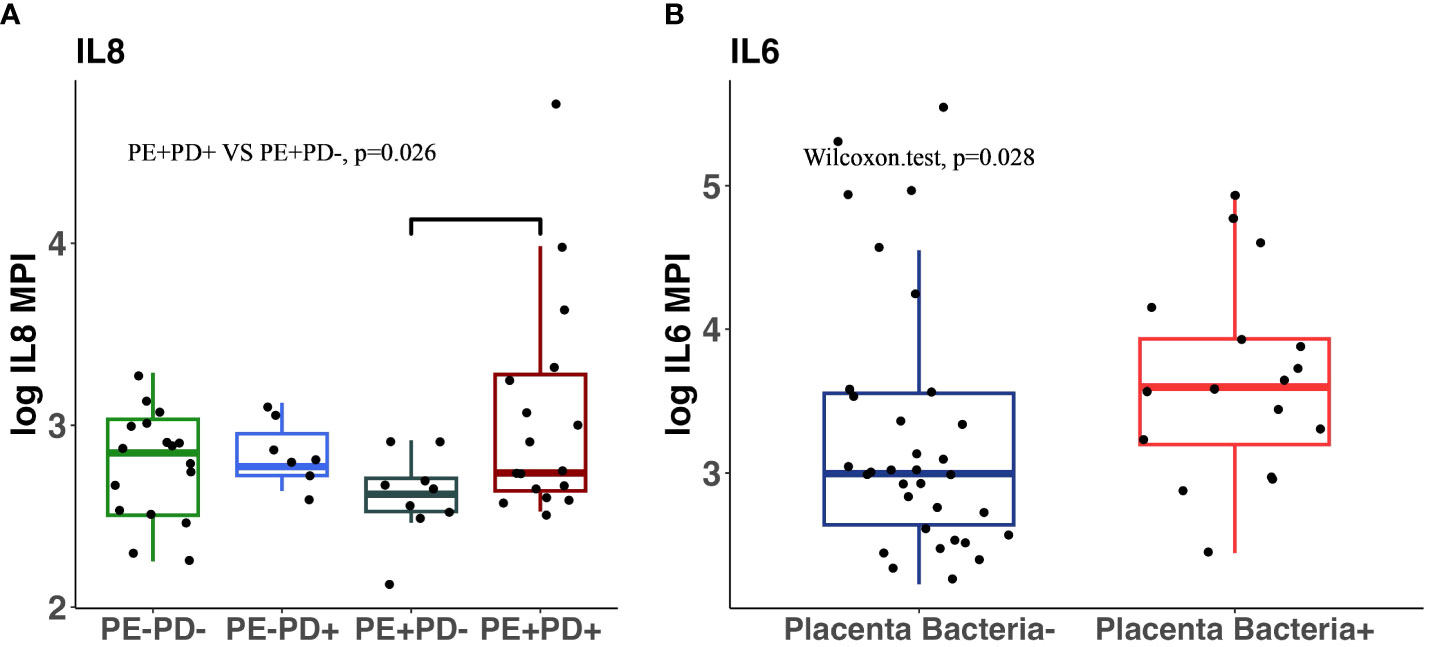
Figure 3 Comparison of inflammatory cytokines in four subgroups and in patients with and without detectable placental microbiota. IL-8 levels in blood are significantly higher in PE+/PD+ patients than in PE+/PD− patients (p = 0.028, Wilcox on sum rank) (A). IL-6 levels in blood are significantly higher in patients with detectable placenta microbiota than those without (p = 0.028, Wilcox on sum rank test) (B).
Discussion
Overall, the placenta microbiome continues to present challenges in characterization due to the low microbial biomass and the potential for DNA contamination through sampling techniques, reagents, and mode of delivery. Although recent studies with rigorous decontamination approaches do not support the presence of placental microbiota, transient bacteria in circulation in pregnancy may lead to the detection of a small number of oral bacteria (de Goffau et al., 2019). By simultaneously profiling the oral and placenta microbiome in the same pregnant women, we identified oral-origin bacteria in placenta from more than half of the patients with PE, compared to less than 20% of patients without PE. The presence of placenta microbiome was associated with increased systemic inflammation in PE. High abundance of Haemophilus in the subgingivae was associated with increased risk of PE.
PE has long been considered as a placental disorder. Despite the lack of cohesive evidence of healthy placenta microbiome (Thies et al., 2019; Sterpu et al., 2021), there is a consensus that the microbiome may be present in placenta in maternal or fetal diseases. After rigorous potential contamination removal, we found that the placenta microbiome was associated with PE. Sequencing the oral and placenta microbiome in parallel in our study is advantageous compared to any previous studies that only focused on either placenta or the oral microbiome. In addition, among the seven placenta-associated microbiota, the relative abundance of Haemophilus, Veillonella, and Fusobacterium was significantly higher in the oral samples of PE+ patients than PE− patients, further supporting an oral origin of these placenta-associated bacteria. Whole genome shotgun sequencing with higher-resolution taxonomic classification is needed to confirm the exact origin of the bacteria. Despite identification of the potential oral origin of placenta microbiome, our study does not exclude the possibility of other sources of the placenta microbiome such as the vagina and the gut. In fact, we found a common gut and vaginal microbiome in the placenta of patients who had a vaginal delivery, as compared to those who underwent a cesarean delivery, suggesting that the microbiome from multiple origins may contribute to the placenta microbiome in PE.
Fusobacterium was previously found to be prevalent in the placenta of PE patients by targeted PCR amplification (Barak et al., 2007; Chen et al., 2020). Our results confirmed this finding and additionally revealed other PE-associated placenta microbiome through 16S sequencing. The underlying reasons why these specific taxa can disseminate to placenta merit future investigation. It is possible that both host factors and microbiota can contribute to microbiome seeding in placenta since hormonal changes during pregnancy in women and the invading capacity of FadA adhesin from F. nucleatum (Richardson et al., 2020) can increase endothelial permeability.
Although the relative abundance of Haemophilus, Veillonella, and Fusobacterium in the oral samples were significantly higher in PE+ patients than in PE− patients, they were similar between PD+ and PD− patients. This suggests that these oral bacteria are potential markers for PE independent of PD status. Interestingly, when analyzing the oral microbiome, we found that Haemophilus but not Veillonella and Fusobacterium in subgingivae was a risk factor for PE. A prior study showed that Haemophilus was important in early biofilm formation but was not necessarily more prominent in caries (Schoilew et al., 2019). This suggests that, in addition to bacteria associated with caries, non-caries-associated microbiome may also be important for PE. Since oral samples were collected when PE was diagnosed, we could not determine whether the increased abundance of these oral bacteria is a consequence or a driver of PE.
Previous studies have shown that proinflammatory cytokines produced in pregnancy may be responsible for the exacerbated endothelial damage seen in women who develop PE in pregnancy (Delassus et al., 1994; Chaouat et al., 1995). However, our study did not observe any statistical difference in circulating cytokines in either of the two groups (PE and PD) even after removing the potential confounder, preterm delivery, and evaluating only the term deliveries. However, subgroup analysis found that within the PE+ group, PE+/PD+ patients had higher IL-8 levels than PE+/PD− patients. We showed that detectable microbiota in placenta was associated with increased circulating IL-6 levels, suggesting that the presence of placenta microbiome may increase proinflammatory response.
This study has several strengths. Special attention was given to the protocol development for collection of placenta specimens, and a rigorous bioinformatic pipeline was employed to decrease the risk of contamination. This study is also one of the first studies to investigate the origins of the placenta microbiome in women with PE and PD by comparing the bacterial taxonomic levels for multiple body sites. Prior to our study, this has not been investigated in detail.
One major limitation of this study is the absence of vagina and stool sampling collection, which restricts the analysis of the placental microbial origins to only oral microbiota. Second, because the placenta in the study was not subjected to removal of maternal blood, it is possible that the microbiota we identified in the placenta represents the disseminating maternal microbiota. Rinsing maternal blood from placenta and inclusion of additional sample types such as maternal blood and cord blood will provide more information on the placenta microbiota during PE. Third, prophylactic antibiotic use during cesarean section and group B Streptococcus infection in the study may alter the oral or placental microbiome, which may complicate the interpretation of the results. However, we found no statistical difference in PE+ and PE− participants in antibiotics usage. Thus, results from group comparisons remain meaningful. In addition, our study is an association study, and we are not able to show a causative link between the microbiome in women with PD and/or PE. Nevertheless, our findings provided important evidence that there exists higher prevalence and abundance of bacteria in the placenta of patients with PE compared to controls, and there is an oral microbiota dysbiosis in PE. Future studies should include early and multiple sampling time points through the whole pregnancy, which allows one to determine whether there is a causal role of the oral microbiome in PE. If this is confirmed, oral microbiome, with easy sampling in clinic, may be used as an alternative strategy to predict or prevent PE.
Data availability statement
The datasets presented in this study can be found on the NCBI database with accession number: PRJNA1077338.
Ethics statement
The studies involving humans were approved by University of Connecticut Health. The studies were conducted in accordance with the local legislation and institutional requirements. Written informed consent for participation in this study was provided by the participants’ legal guardians/next of kin.
Author contributions
SC: Conceptualization, Data curation, Investigation, Methodology, Resources, Visualization, Writing – original draft, Writing – review & editing. AB: Conceptualization, Investigation, Supervision, Validation, Writing – review & editing. ST: Data curation, Investigation, Methodology, Supervision, Writing – review & editing. EH: Supervision, Writing – review & editing. AH: Data curation, Investigation, Methodology, Writing – review & editing. CK: Data curation, Formal analysis, Methodology, Software, Writing – review & editing. NB: Data curation, Investigation, Methodology, Writing – review & editing. HY: Data curation, Formal analysis, Investigation, Methodology, Software, Writing – review & editing. HP: Data curation, Investigation, Methodology, Project administration, Supervision, Writing – review & editing. QL: Data curation, Formal analysis, Investigation, Methodology, Writing – review & editing. KM: Data curation, Formal analysis, Investigation, Methodology, Software, Validation, Writing – original draft. WC: Conceptualization, Investigation, Methodology, Supervision, Writing – original draft. YZ: Conceptualization, Data curation, Formal analysis, Investigation, Methodology, Project administration, Resources, Supervision, Validation, Writing – original draft, Writing – review & editing.
Funding
The author(s) declare that no financial support was received for the research, authorship, and/or publication of this article.
Conflict of interest
The authors declare that the research was conducted in the absence of any commercial or financial relationships that could be construed as a potential conflict of interest.
Publisher’s note
All claims expressed in this article are solely those of the authors and do not necessarily represent those of their affiliated organizations, or those of the publisher, the editors and the reviewers. Any product that may be evaluated in this article, or claim that may be made by its manufacturer, is not guaranteed or endorsed by the publisher.
Supplementary material
The Supplementary Material for this article can be found online at: https://www.frontiersin.org/articles/10.3389/fbrio.2023.1322165/full#supplementary-material
References
Aagaard K., Ma J., Antony K. M., Ganu R., Petrosino J., Versalovic J. (2014). The placenta harbors a unique microbiome. SciTransl. Med. 6 (237), 237ra65. doi: 10.1126/scitranslmed.3008599
Amarasekara R., Jayasekara R. W., Senanayake H., Dissanayake V. H. (2015). Microbiome of the placenta in pre-eclampsia supports the role of bacteria in the multifactorial cause of pre-eclampsia. J. Obstet Gynaecol. Res. 41 (5), 662–669. doi: 10.1111/jog.12619
Azofeifa A., Yeunf L., Alverson C. (2016). Dental caries and periodontal disease among U.S. pregnant women and nonpregnant women of reproductive age, National Health and Nutrition Examination Survey, 1999–2004. J. Public Health Dent. 76 (4), 320–329. doi: 10.1111/jphd.12159
Barak S., Oettinger-Barak O., Machtei E., Sprecher H., Ohel G. (2007). Evidence of periopathogenic microorganisms in placentas of women with preeclampsia. J. Periodontol. 78, 670. doi: 10.1902/jop.2007.060362
Chaouat G., Assal Meliani A., Martal J., Raghupathy R., Elliott J. F., Mosmann T., et al. (1995). IL-10 prevents naturally occurring fetal loss in the CBA x DBA/2 mating combination, and local defect in IL-10 production in this abortion-prone combination is corrected by in vivo injection of IFN-tau. J. Immunol. 154 (9), 4261–4268. doi: 10.4049/jimmunol.154.9.4261
Chapple I. L. C., Mealey B. L., Van Dyke T. E., Bartold P. M., Dommisch H., Eickholz P., et al. (2018). Periodontal health and gingival diseases and conditions on an intact and a reduced periodontium: Consensus report of workgroup 1 of the 2017 World Workshop on the Classification of Periodontal and Peri-Implant Diseases and Conditions. J. Clin. Periodontol.
Chen X., Li P., Liu M., Zheng H., He Y., Chen M. X., et al. (2020). Gut dysbiosis induces the development of pre-eclampsia through bacterial translocation. Gut 69 (3), 513–522. doi: 10.1136/gutjnl-2019-319101
Corcoll N., Osterlund T., Sinclair L., Eiler A., Kristiansson E., Backhaus T., et al. (2017). Comparison of four DNA extraction methods for comprehensive assessment of 16S rRNA bacterial diversity in marine biofilms using high-throughput sequencying. FEMS Microbiol. Lett. 364 (14), fnx139. doi: 10.1093/femsle/fnx139
de Goffau M. C., Lager S., Sovio U., Gaccioli F., Cook E., Peacock S. J., et al. (2019). Human placenta has no microbiome but can contain potential pathogens. Nature 572, 329–334. doi: 10.1038/s41586-019-1451-5
Delassus S., Coutinho G. C., Saucier C., Darche S., Kourilsky P. (1994). Differential cytokine expression in maternal blood and placenta during murine gestation. J. Immunol. 152 (5), 2411–2420. doi: 10.4049/jimmunol.152.5.2411
Eke P. I., Dye B. A., Wei L., Thornton-Evans G. O., Genco R. J., CDC Periodontal Disease Surveillance workgroup, et al. (2012). Prevalence of periodontitis in adults in the United States: 2009–2010. J. Dent. Res. 91 (10), 914–920. doi: 10.1177/0022034512457373
Eke P. I., Thornton-Evans G. O., Wei L., Borgnakke W. S., Dye B. A., Genco R. J. (2018). Periodontitis in US adults: national health and nutrition examination survey 2009-2014. J. Am. Dental Assoc.
Esra E., Kenan E., Ozrur D., Deniz G., Ozgur O., Belgin A., et al. (2013). Evaluation of periodontal pathogens in amniotic fluid and the role of periodontal disease in preterm birth and low birth weight. Acta Odontol. Scand. 71 (3–4), 553–559.
Faul F., Erdfelder E., Lang A.-G., Buchner A. (2007). G*Power 3: a flexible statistical power analysis program for the social, behavioral, and biomedical sciences. Behav. Res. Methods 39, 175–191. doi: 10.3758/BF03193146
Gestational hypertension and preeclampsia. (2020). ACOG Practice Bulletin No. 222. American College of Obstetricians and Gynecologists. Obstet Gynecol. 135, e237–e260. doi: 10.1097/AOG.0000000000003891
Kelly B., Gross R., Bittinger K., Sherrill-Mix S., Lewis J. D., Collman R. G., et al. (2015). Power and sample-size estimation for microbiome studies using pair wise distances and PERMANOVA. Bioinformatics 31 (15), 2461–2468. doi: 10.1093/bioinformatics/btv183
Krohn M., Rabe L., Klebanoff S., Eschenbach D. (1993). The normal vaginal flora, H2O2-producing lactobacilli, and bacterial vaginosis in pregnant women. Clin. Infect. Dis. 16, S273–8110.
Lang N. P., Adler R., Joss A., Nyman S. (1990). Absence of bleeding on probing An indicator of periodontal stability. J. Clin. Periodontol. doi: 10.1111/j.1600-051X.1990.tb01059.x
Lieff S., Boggess K. A., Murtha A. P., Jared H., Madianos P. N., Moss K., et al. (2004). The oral conditions and pregnancy study: periodontal status of a cohort of pregnant women. J. Periodontol. 75, 116–126. doi: 10.1902/jop.2004.75.1.116
Maas K.. GitHub: Bioinformatics. https://github.com/krmaas/bioinformatics/blob/master/mothur.batch.
Mustafa R., Ahmed S., Gupta A., Venuto R. C. (2012). A comprehensive review of hypertension in pregnancy. J. Pregnancy. 2012, 105918. doi: 10.1155/2012/105918
Offenbacher S., Barros S. P., Beck J. D. (2008). Rethinking periodontal inflammation. J. Periodontol. 79, 1577–1584. doi: 10.1902/jop.2008.080220
Quast C., Pruesse E., Yilmaz P., Gerken J., Schweer T., Yarza P., et al. (2012). The SILVA ribosomal RNA gene database project: improved data processing and web-based tools. Nucleic Acids Res. 41 (D1), D590–D596. doi: 10.1093/nar/gks1219
Richardson M., Ren J., Rubinstein M. R., Taylor J. A., Friedman R. A., Han B. S. Y. (2020). Analysis of 16S rRNA genes reveals reduced Fusobacterial community diversity when translocating from saliva to GI sites. Gut Microbes 12, 1. doi: 10.1080/19490976.2020.1814120
Schoilew K., Ueffing H., Dalpke A., Wolff B., Frese C., Wolff D., et al. (2019). Bacterial biofilm composition in healthy subjects with and without caries experience. J. Oral. Microbiol. 11, 1. doi: 10.1080/20002297.2019.1633194
Sterpu I., Fransson E., Hugerth L. W., Du J., Pereira M., Cheng L., et al. (2021). No evidence for a placenta microbiome in human pregnancies at term. Am. J. Obstet Gynecol. 224 (3), 296.e1–296.e23.
Swati P., Thomas B., Vahab S. A., Kapaettu S., Kushtagi P. (2012). Simultaneous detection of periodontal pathogens in subgingival plaque and placenta of women with hypertension in pregnancy. Arch. Gynecol. Obstet 285 (3), 613–619. doi: 10.1007/s00404-011-2012-9
The Human Microbiome Project Consortium (2012). Structure, function and diversity of the healthy human microbiome. Nature 486, 207–214. doi: 10.1038/nature11234
Thies K. R., Romero R., Winters A. D., Greenberg J. M., Gomez-Lopez N., Alhousseini A., et al. (2019). Does the human placenta delivered at term have a microbiota? Results of cultivation, quantitative real-time PCR, 16S rRNA gene sequencing, and metagenomics. Am. J. Obstet Gynecol. 220, 267.e1–267.e39. doi: 10.1016/j.ajog.2018.10.018
Keywords: preeclampsia, placenta, microbiome, gut, pregnancy, oral, periodontal disease, periodontitis
Citation: Cooper SM, Borgida A, Thacker S, Hammer E, Hariharan A, Kuo C, Blanck N, Yuan H, Panier H, Lin Q, Maas K, Campbell W and Zhou Y (2024) Oral origin of the placenta microbiome in pregnant women with preeclampsia. Front. Bacteriol. 2:1322165. doi: 10.3389/fbrio.2023.1322165
Received: 15 October 2023; Accepted: 08 December 2023;
Published: 14 March 2024.
Edited by:
Rajan P. Adhikari, Integrated BioTherapeutics, Inc., United StatesReviewed by:
Roger M. Arce, University of Texas Health Science Center at Houston, United StatesChristina Megli, University of Pittsburgh, United States
Copyright © 2024 Cooper, Borgida, Thacker, Hammer, Hariharan, Kuo, Blanck, Yuan, Panier, Lin, Maas, Campbell and Zhou. This is an open-access article distributed under the terms of the Creative Commons Attribution License (CC BY). The use, distribution or reproduction in other forums is permitted, provided the original author(s) and the copyright owner(s) are credited and that the original publication in this journal is cited, in accordance with accepted academic practice. No use, distribution or reproduction is permitted which does not comply with these terms.
*Correspondence: Shontreal M. Cooper, schooper@uchc.edu