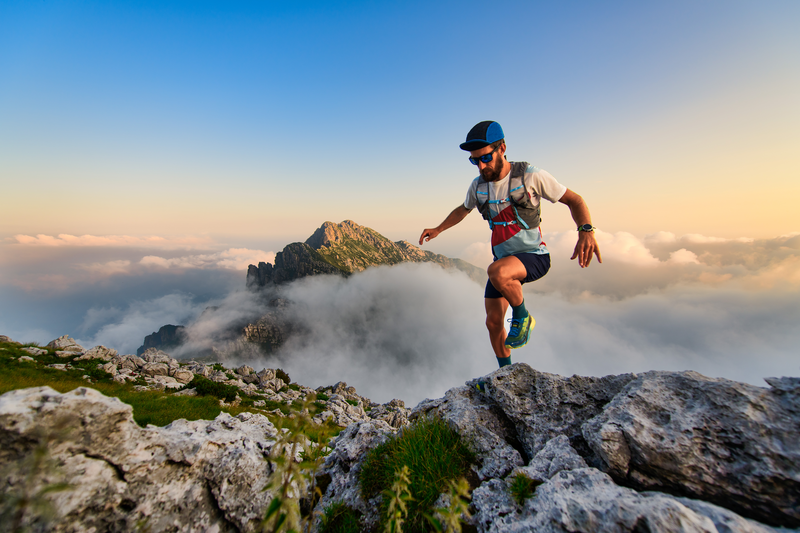
94% of researchers rate our articles as excellent or good
Learn more about the work of our research integrity team to safeguard the quality of each article we publish.
Find out more
ORIGINAL RESEARCH article
Front. Bacteriol. , 04 December 2023
Sec. Pathogenesis, Vaccines, and Immunity of Bacterial Infections
Volume 2 - 2023 | https://doi.org/10.3389/fbrio.2023.1287779
This article is part of the Research Topic Editors' Showcase: Pathogenesis, Vaccines, and Immunity of Bacterial Infections View all 8 articles
Children under one year of age are consistently found to be at the highest risk for shunt infection as a complication of hydrocephalus treatment, and repeated infections in the same patient are common. The mechanisms responsible for the increase in infant infection are not known. Our laboratory adapted our existing mouse model of Staphylococcus epidermidis central nervous system (CNS) catheter infection to characterize infection in infant mice as a foundation of on-going studies to determine the mechanisms of increased morbidity in infant shunt infections. The well-established model of S. epidermidis biofilm infection in the CNS was adapted to generate infection in 14-day old C57BL/6 mice. The catheter preparation was identical to the adult model, in which silicone catheters are pre-coated with a clinically derived biofilm-forming strain of S. epidermidis strain 1457) to introduce S. epidermidis into the brain. The catheters are stereotactically implanted into the lateral left ventricle. Bacterial titers as well as cytokine and chemokine levels were measured at days 1, 3, and 5 in mice implanted with sterile and S. epidermidis-coated catheters. Cultures demonstrated both catheter-associated and parenchymal infection through day 5 post-implantation. In contrast to prior adult models of CNS catheter infection, however, the catheter-associated bacterial burdens were less than or equal to parenchymal bacterial burdens. There was no significant difference between the chemokine and cytokine levels in the brain tissues of pups with sterile versus S. epidermidis infected catheters for any of the analytes tested. The infant model of S. epidermidis CNS catheter infection is characterized by both a greater degree of parenchymal spread of bacteria and the absence of the elevated pro-inflammatory cytokines previously observed in adult mice with this infection. The attenuated inflammatory response may contribute to the increased infection risk observed in this population.
Cerebrospinal fluid shunt placement for the treatment of hydrocephalus is the most common pediatric neurosurgery procedure performed in the United States (Hanak et al., 2017). Unfortunately, infants under the age of one year of age are at the highest risk for shunt infection following this life-saving procedure and may experience many shunt infections over the course of their childhood (Vinchon and Dhellemmes, 2006; Hommelstad et al., 2013; Simon et al., 2014). Shunt infections are most commonly caused by Staphylococcus epidermidis (S. epidermidis), which forms biofilms on the catheter that are recalcitrant to antibiotic therapy and immune-mediated clearance (Kockro et al., 2000; Fux et al., 2006; Vinchon and Dhellemmes, 2006; Gutierrez-Murgas and Snowden, 2014). Thus, infants with shunt infections undergo many surgical procedures to replace infected shunts (Kockro et al., 2000; Fux et al., 2006; Gutierrez-Murgas and Snowden, 2014). Additionally, shunt infections are associated with long-term increases in seizure risk, decreases in intellectual ability, and overall mortality (Fux et al., 2006; Vinchon et al., 2012). The mechanisms for the increased risk of shunt infections in infants are unknown. Studies designed to advance our understanding of the immune response to shunt infections in infants could significantly improve their life-long outcomes through development of diagnostic, treatment and prevention strategies for these serious infections.
Our laboratory previously developed adult murine models of S. epidermidis and Staphylococcus aureus (S. aureus) CNS catheter infection, mimicking what is seen in humans with ventricular shunt infections (Snowden et al., 2012; Snowden et al., 2013; Gutierrez-Murgas et al., 2016). Establishing a catheter model within the CNS was necessary because the immune response in this compartment often differs from that seen in the periphery due to the inherent anti-inflammatory environment of the normal CNS (Hampl et al., 2003). However, in response to injury or infection, the CNS is capable of mounting a robust immune response (Hampl et al., 2003; Lucas et al., 2006; Kielian et al., 2007). We have observed increases in pro-inflammatory cytokines IL6, IL1β, CXCL1, and CCL2 in this model, driven primarily by bacterial dissemination to surrounding parenchymal tissue, with less inflammation attributable to the catheter-associated infection (i.e. biofilm) (Snowden et al., 2013; Gutierrez-Murgas et al., 2016). This demonstrates that catheter-associated infections trigger an immune response distinct from parenchymal infections. It is important to consider the differences between biofilm and parenchymal inflammatory responses when attempting to understand the pathophysiology of device associated infections such as CNS catheter infections. In this report, we characterize our newly adapted infant mouse model of CNS catheter infection, which will serve as a powerful tool to identify key factors in the host immune response to staphylococcal infections in the CNS. Our results show that the inflammatory response to S. epidermidis is not markedly attenuated, which may have significant implications for diagnosis of shunt infection in this population and may contribute to their increased morbidity.
All in vivo experiments were performed using 14-day old C57BL/6 mice (The Jackson Laboratory, Bar Harbor, ME), hereafter referred to as infant mice. This age was selected as it is equivalent in maturity to late infancy in humans and, by extension, predicted to fall within the highest risk age group for CNS catheter infection (Clancy et al., 2001; Bilbo et al., 2006; Sengupta, 2013). Additionally, other mouse models have demonstrated that 14 days of age falls within the interval where the developing brain is at highest risk of damage from inflammatory stimuli (Bilbo et al., 2006; Spencer et al., 2011). The C57BL/6 mouse strain has been reported to be less susceptible to staphylococcal infection in other studies (Thanert et al., 2017); however, it was required for these experiments to allow comparison with the prior adult mouse model studies and future comparison to transgenic mouse strains which are largely on the C57BL/6 background. Each experiment was done independently at three different dates with 4 to 5 mice per experimental group (n=12-15 mice/group). The protocol for animal use was approved by both the University of Nebraska Medical Center Institutional Animal Care and Use Committee (Protocol #09-053-08-FC) and the University of Arkansas for Medical Sciences Institutional Animal Care and Use Committee (Protocol #3887) and is compliant with the National Institute of Health (NIH) guidelines for the use of rodents.
Staphylococcus epidermidis 1457 was graciously provided by Dr. Paul Fey laboratory (University of Nebraska Medical Center). This isolate was recovered from an infected central venous catheter and initially characterized by Mack et al. (1996).
To establish CNS catheter-associated infection, a hollow bore silicone catheter (2 mm in length, 1 mm in diameter) are individually incubated in a concentrated solution of S. epidermis for 24 hours, prior to implantation into the brain at the site of the lateral ventricle (relative to bregma: +0 mm rostral; +0.5 mm lateral) as previously described (Snowden, 2014; Gutierrez-Murgas et al., 2016). Twenty-four hour incubation in S. epidermidis 1457 resulted in reproducible catheter colonization of approximately 6x107 cfu/mL. This approach utilizes both the same surgical material and location of CNS catheter placement for the treatment of hydrocephalus in children (Cunningham et al., 2005). Young mice with infected catheters display signs of illness for the first 3 days following implantation and experience significantly greater weight loss than young mice receiving sterile catheters or anesthesia alone, with the former losing 8-10% of their initial body weight following the procedure. In these experiments, no seizure activity was noted and mortality outside the immediate operative period was less than 5%.
Mice were sacrificed at days 1, 3, and 5 post-surgery and the catheter and surrounding brain tissue were collected as previously described (Snowden et al., 2012). Briefly, catheters were rinsed in sterile phosphate-buffered saline (PBS) to decrease non-adherent bacteria and then sonicated to disrupt the adherent bacterial biofilm. Tissues within 1 mm of the catheter implantation location were homogenized in 250µl sterile PBS supplemented with a complete protease inhibitor cocktail tablet (Roche, Basel Switzerland) and RNase inhibitor (Promega, Madison WI) for quantitative culture of bacterial spread and to measure inflammatory mediators. A 100 µl aliquot of brain homogenates and sonicated catheter solution were used to quantitate bacterial titers via 10-fold serial dilutions in tryptic soy agar plates. The remaining brain homogenates were also centrifuged to collect supernatants for cytokine/chemokine analysis, as previously described (Snowden et al., 2012; Snowden et al., 2013).
Cytokines and chemokines in brain tissues surrounding sterile and infected catheters and a similar location in anesthesia control mice were measured with mouse microbead array system according to the manufacturer’s instruction (Milliiplex, Billerica, MA). This assay measured 12 different inflammatory molecules in a single 50µl supernatant sample including (IL1β, IL4, IL6, IL9, IL10, IL12p70, IL13, CXCL1, CXCL2, CXCL9, CXCL10, CCL2). Results were analyzed using Multiplex Assay Analysis Software.
We performed nest building evaluations on mice with sterile and infected catheters at 4 weeks post-catheter implantation. Nest building behavior reflects a wide range of factors, including thermoregulation, hormonal changes and behavioral dysfunction and has been to shown to be affected in other models of CNS infection (Cunningham et al., 2005). Briefly, mice were housed individually overnight with a single cotton nestlet that has been weighed prior to placement in the cage. After the 12 h dark cycle concludes, the mice and nests are photographed for scoring by an investigator blinded to treatment groups, and any intact nestlet material weighed. Nests are scored based on a standardized 1 (no nest) to 5 (near perfect nest) scale (Deacon, 2006).
Analysis of significant differences between experimental groups was determined using unpaired student t-test with GraphPad Prism (San Diego, CA) at the 95% confidence interval. A p-value of less than 0.05 was considered statistically significant.
To assess the bacteriologic kinetics of S. epidermidis catheter-associated infection in the CNS in infants, mice were implanted with either sterile silicone catheters or catheters pre-coated with S. epidermidis 1457 as described above. Importantly, the catheter preparation and bacterial strain are identical to the adult catheter implantation procedure. At days 1, 3, and 5 following implantation, the catheters were removed and both the catheter and surrounding parenchyma were cultured to compare the catheter-associated bacterial burden, which has been consistent with biofilm formation by electron microscopy in the adult mouse model of CNS catheter infection, with the bacteria isolated from the brain parenchyma surrounding the catheter (Snowden et al., 2012). All infant mice demonstrated catheter-associated bacterial growth, consistent with biofilm infection. As observed in Figure 1, significantly higher bacterial burdens were measured in the parenchyma at day 1 post-implantation, compared with the catheter associated bacterial growth. The predominance of parenchymal over catheter-associated bacterial growth is distinct from our prior findings in the adult model of CNS catheter infection, despite identical surgical approaches and initial inocula (Gutierrez-Murgas et al., 2016). On subsequent days post-implantation, the parenchymal and catheter-associated bacterial burdens were similar and both decreased over time. This time course of resolution is consistent with prior experiments utilizing S. aureus and S. epidermidis (Snowden et al., 2012; Gutierrez-Murgas et al., 2016). None of the mice with sterile catheters implanted had either catheter-associated or parenchymal bacterial growth at any time point. This model was capable of reproducing S. epidermidis catheter-related infection demonstrating its appropriateness to adequately evaluate host immune response to S. epidermidis infection in the CNS of infant mice.
Figure 1 Parenchymal bacterial growth predominates during the initial post-implantation period, suggesting dissemination from the biofilm. Catheters were removed from the brain tissue, rinsed in PBS and then sonicated in 500 ml of PBS. This solution was then titered and cultured on blood agar plates. Supernatants from homogenates of catheter associated tissue were also cultured on blood agar plates. The cultures from the catheters and parenchyma of the mice implanted with sterile catheter were negative and are not represented on this graph. *p<0.05.
Homogenates of catheter-associated tissue were analyzed for IL1β, IL4, IL6, IL9, IL10, IL12p70, IL13, CXCL1, CXCL2, CXCL9, CXCL10, and CCL2 levels by multianalyte microbead array to assess the inflammatory response to S. epidermidis catheter infection in the infant CNS as compared to the inflammatory response to a sterile catheter. As seen in Figures 2, 3, there is no significant difference at any time point between the levels of pro-inflammatory chemokines and cytokines in infant with sterile versus S. epidermidis infected catheters. Similarly, levels of anti-inflammatory cytokines IL10 and IL13 were similar between the two groups (Figure 4). IL4 was undetectable in all samples and is not shown. The induction of inflammatory mediators at early time points following implantation of sterile catheters likely results from tissue damage (Supplemental Figure, chemokine/cytokines in anesthesia controls). However, the lack of an initial increased pro- inflammatory response in mice with infected catheters is distinct from our prior observations in the adult model of S. epidermidis CNS catheter infection, in which CXCL1, CXCL2, IL1β, and IL6 were all significantly increased early in infection (Gutierrez-Murgas et al., 2016). Collectively, these findings demonstrate that the cytokine and chemokine response to S. epidermidis infected catheters in the infant CNS is attenuated.
Figure 2 No increase in pro-inflammatory mediators IL1β, CXCL1, IL6, or CCL2 in infant mice in response to S. epidermidis CNS catheter infection. Results from mice with infected (darker bar) versus sterile (lighter bar) at days 1, 3, and 5 post-implantation are shown here for IL1β (A), CXCL1 (B), IL6 (C), and CCL2 (D). Supernatants from homogenates of catheter-associated tissue were analyzed for by multiplex microbead array. There is no significant difference between infected or sterile results at any time point for any of the analytes.
Figure 3 No increase in inflammatory mediators IL12p70, CXCL9, IL9, or CXCL10 in infant mice in response to S. epidermidis CNS catheter infection. Results from mice with infected (darker bar) versus sterile (lighter bar) at days 1, 3, and 5 post-implantation are shown here for IL12p70 (A), CXCL9 (B), IL9 (C), and CXCL10 (D). Supernatants from homogenates of catheter-associated tissue were analyzed for by multiplex microbead array. There is no significant difference between infected or sterile results at any time point for any of the analytes.
Figure 4 No increase in anti-inflammatory mediators IL13 or IL10 in infant mice in response to S. epidermidis CNS catheter infection. Results from mice with infected (darker bar) versus sterile (lighter bar) at days 1, 3, and 5 post-implantation are shown here for IL13 (A) or IL10 (B). Supernatants from homogenates of catheter-associated tissue were analyzed for by multiplex microbead array. There is no significant difference between infected or sterile results at any time point for any of the analytes.
Human studies suggest that neurologic abnormalities can occur after CNS catheter infection (Fux et al., 2006; Vinchon et al., 2012), but this has not been previously evaluated in animal studies. As shown in Figure 5, a larger number of mice with a history of S. epidermidis-infected catheter placement (n=10; nestlet score range 1-5) displaced impaired nest building 4 weeks after catheter implantation than observed in infant mice with sterile catheter placement (n=8; nestlet scores all 5).
Figure 5 Infant mice with a history of infected CNS catheter placement may be more likely to have impaired nestlet building performance at 4 weeks post-implantation than infant mice with sterile CNS catheter placement. Results from mice with infected (left) versus sterile (right) catheter placement at 14 days of age, assessed at 28 days post-implantation. Nestlet scores assessed by two blinded assessors after mice were individually housed for 12 hours with the nestlet. Scores range from 1 (no nest) to 5 (near perfect nest). The difference in scores were not statistically significant. n= 10 infected; 8 sterile.
Infants are at higher risk of S. epidermidis infection following CNS catheter placement in the treatment of hydrocephalus, but the mechanisms of this increased morbidity are not well-defined. Our initial characterization of an infant model of S. epidermidis CNS catheter shows that the early immune response to CNS S. epidermidis catheter infection is notably attenuated in young mice, which is surprising given the significant bacterial burden in the brain tissue (Figure 1) and speaks to a distinct difference in the early immune responses of infants as opposed to adults. Specifically, we have observed that infant mice do not mount the early pro-inflammatory response observed in the previously reported adult model of this infection (Gutierrez-Murgas et al., 2016). This may have significant clinical implications, as diagnosis of shunt infections frequently relies on the detection of systemic and CNS markers of inflammation, which are variable in young infants with central nervous infections and are often absent until late in illness (Gutierrez-Murgas and Snowden, 2014). The relatively lower levels of CNS inflammation observed in our studies may explain the common lack of traditional measures of infection like fever in children with shunt infections and suggests that we may need an increased sensitivity for diagnosing infection in these children, as they may not mount the robust inflammatory response we expect in older children with meningitis or device associated infection.
Human studies have demonstrated a similar attenuation in inflammatory responses to S. epidermidis in infants, with age-dependent decreases in cytokineproduction following exposure of cord blood monocytes to S. epidermidis in vitro and decreased systemic levels of C-reactive protein in infants with S. epidermidis device-associated infections (Klingenberg et al., 2005; Strunk et al., 2012). However, an in vivo model of S. epidermidis bacteremia reported increased CXCL1, IL6, and TNFα in the bloodstream of neonatal mice, suggesting that the immune response to S. epidermidis may be tailored to the site of infection (Kronforst et al., 2012). Systemic measures of inflammation will be evaluated in future studies of infant CNS catheter-associated infection to assess parallels between human and murine illness, as well to identify potential novel biomarkers. Microglia and astrocytes also appear to have altered responses in infancy, which likely plays a key role in the phenotype observed in this infant model of infection and will be examined in future studies directly comparing adult and infant responses (Floden and Combs, 2006; Kurronen et al., 2012; Christensen et al., 2014). This highlights the importance of longitudinal mechanistic studies to increase our understanding of the immune response to S. epidermidis in the CNS, as this infection is clinically and immunologically distinct from peripheral bacterial infection.
Interestingly, a greater proportion of bacteria spread from the catheter to the parenchyma in young mouse brains (Figure 1). In contrast, in adult animals, significantly more bacteria are adherent to the catheter, with a lesser degree of parenchymal spread (Gutierrez-Murgas et al., 2016). Importantly, this increase in parenchymal bacterial spread in the presence of a hypo-inflammatory immune response in young hosts may play a role in the increased susceptibility of human infants to S. epidermidis disease. The mechanisms responsible for differential bacterial localization in young vs. adult brains will be pursued in other studies.
There is increasing evidence that inflammation in early development, from intrauterine, peripartum and early childhood insults, may have lifelong impacts on neurologic function. For example, elevated pro-inflammatory mediators in cord blood have been associated with an increased risk of cerebral palsy in several case series although it is difficult to determine how much of this effect is due to inflammation vs. other confounders (Armstrong-Wells et al., 2015; Bi et al., 2014; Varner et al., 2015). Animal studies attempting to better define the impact of specific inflammatory processes on neurologic function have demonstrated that cytokine exposure during infancy increases the risk for changes in memory or neuropsychiatric function later in life following a second immune challenge (Bilbo et al., 2005; Bilbo et al., 2006; Bilbo et al., 2007; Williamson et al., 2011). Additionally, following E. coli sepsis, rodents are less responsive to cognitive enrichment strategies than those without a history of infection (Bilbo et al., 2007). Interestingly, these effects are only observed in rodents less than 30 days of age, suggesting an early period of vulnerability in the developing brain (Bilbo et al., 2006; Williamson et al., 2011). Therefore, the attenuated inflammatory response observed in this infant model of S. epidermidis CNS catheter infection may be beneficial to the developing brain. The impact of CNS S. epidermidis infection on neurodevelopmental outcomes has not been previously explored, despite the frequency of this pathogen in human infants. The trend we observed in nest building studies (Figure 5) suggest that early CNS catheter infection may impact long-term neurologic outcomes; however, there was variability in the phenotypes observed. This variation parallels variations in human outcomes and suggests the need for more study of neurologic changes in this setting. Future studies will also need to include other biofilm-forming pathogens reported in CNS catheter infection, such as S. aureus, E. coli and other Gram-negative bacteria, and fungal pathogens such as Candida albicans (Gutierrez-Murgas and Snowden, 2014; Simon et al., 2014; Hanak et al., 2017).
The development of this infant murine model of central nervous system catheter infection is an important advancement as it now allows an investigation of the immunologic and neurologic responses to these infections in infants. With the identification of inflammatory responses that are skewed in young animals, we can design future studies to evaluate key signaling decision points, which will provide more specific targets for potential immunomodulatory therapy and screening for high-risk patients. New treatment and diagnostic modalities are imperative given the high costs, in healthcare expense and lifelong patient morbidity, associated with CNS catheter infections in infancy.
The raw data supporting the conclusions of this article will be made available by the authors, without undue reservation.
The animal study was approved by University of Nebraska Medical Center IACUC; University of Arkansas for Medical Sciences IACUC. The study was conducted in accordance with the local legislation and institutional requirements.
GS: investigation, methodology, writing – review & editing. KE: investigation, methodology, writing – review & editing. MB: investigation, methodology, writing – review & editing. BW: investigation, methodology, writing – review & editing. MH: investigation, methodology, writing – review & editing. JS: conceptualization, formal analysis, investigation, methodology, project administration, supervision, writing – original draft, writing – review & editing.
The author(s) declare financial support was received for the research, authorship, and/or publication of this article. NINDS R01NS092732 supported the experiments described herein.
The authors thank Dr. Paul Fey at the University of Nebraska Medical Center for providing the S. epidermidis strain used herein. We would like to thank Dr. Tammy Kielian for her mentorship and scientific guidance.
The authors declare that the research was conducted in the absence of any commercial or financial relationships that could be construed as a potential conflict of interest.
All claims expressed in this article are solely those of the authors and do not necessarily represent those of their affiliated organizations, or those of the publisher, the editors and the reviewers. Any product that may be evaluated in this article, or claim that may be made by its manufacturer, is not guaranteed or endorsed by the publisher.
The Supplementary Material for this article can be found online at: https://www.frontiersin.org/articles/10.3389/fbrio.2023.1287779/full#supplementary-material
CNS, Central nervous system; S. aureus, Staphylococcus aureus; S. epidermidis, Staphylococcus epidermidis; i.p., Intraperitoneal; PBS, Phosphate buffered saline; IL, Interleukin (IL1β, IL4, IL6, IL9, IL10, IL12p70, IL13).
Armstrong-Wells J., Donnelly M., Post M. D., Manco-Johnson M. J., Winn V. D., Sebire G. (2015). Inflammatory predictors of neurologic disability after preterm premature rupture of membranes. Am. J. Obstet Gynecol. 212 (2), 212.e1-9. doi: 10.1016/j.ajog.2014.09.016
Bi D., Chen M., Zhang X., Wang H., Xia L., Shang Q., et al. (2014). The association between sex-related interleukin-6 gene polymorphisms and the risk for cerebral palsy. J. Neuroinflammation 11, 100–2094-11-100. doi: 10.1186/1742-2094-11-100
Bilbo S. D., Levkoff L. H., Mahoney J. H., Watkins L. R., Rudy J. W., Maier S. F. (2005). Neonatal infection induces memory impairments following an immune challenge in adulthood. Behav. Neurosci. 119 (1), 293–301. doi: 10.1037/0735-7044.119.1.293
Bilbo S. D., Newsum N. J., Sprunger D. B., Watkins L. R., Rudy J. W., Maier S. F. (2007). Differential effects of neonatal handling on early life infection-induced alterations in cognition in adulthood. Brain Behav. Immun. 21 (3), 332–342. doi: 10.1016/j.bbi.2006.10.005
Bilbo S. D., Rudy J. W., Watkins L. R., Maier S. F. (2006). A behavioural characterization of neonatal infection-facilitated memory impairment in adult rats. Behav. Brain Res. 169 (1), 39–47. doi: 10.1016/j.bbr.2005.12.002
Christensen L. B., Woods T. A., Carmody A. B., Caughey B., Peterson K. E. (2014). Age-related differences in neuroinflammatory responses associated with a distinct profile of regulatory markers on neonatal microglia. J. Neuroinflammation 11, 70–2094-11-70. doi: 10.1186/1742-2094-11-70
Clancy B., Darlington R. B., Finlay B. L. (2001). Translating developmental time across mammalian species. Neuroscience 105 (1), 7–17. doi: 10.1016/S0306-4522(01)00171-3
Cunningham C., Deacon R. M., Chan K., Boche D., Rawlins J. N., Perry V. H. (2005). Neuropathologically distinct prion strains give rise to similar temporal profiles of behavioral deficits. Neurobiol. Dis. 18 (2), 258–269. doi: 10.1016/j.nbd.2004.08.015
Deacon R. M. (2006). Assessing nest building in mice. Nat. Protoc. 1 (3), 1117–1119. doi: 10.1038/nprot.2006.170
Floden A. M., Combs C. K. (2006). Beta-amyloid stimulates murine postnatal and adult microglia cultures in a unique manner. J. Neurosci. 26 (17), 4644–4648. doi: 10.1523/JNEUROSCI.4822-05.2006
Fux C. A., Quigley M., Worel A. M., Post C., Zimmerli S., Ehrlich G., et al. (2006). Biofilm- related infections of cerebrospinal fluid shunts. Clin. Microbiol. Infect. 12 (4), 331–337. doi: 10.1111/j.1469-0691.2006.01361.x
Gutierrez-Murgas Y. M., Skar G., Ramirez D., Beaver M., Snowden J. N. (2016). IL-10 plays an important role in the control of inflammation but not in the bacterial burden in S. epidermidis CNS catheter infection. J. Neuroinflammation 13 (1), 271. doi: 10.1016/j.jneuroim.2014.08.006
Gutierrez-Murgas Y., Snowden J. N. (2014). Ventricular shunt infections: Immunopathogenesis and clinical management. J. Neuroimmunol 276 (1-2), 1–8. doi: 10.1016/j.jneuroim.2014.08.006
Hampl J. A., Weitzel A., Bonk C., Kohnen W., Roesner D., Jansen B. (2003). Rifampin-impregnated silicone catheters: A potential tool for prevention and treatment of CSF shunt infections. Infection 31 (2), 109–111. doi: 10.1007/s15010-002-2113-2
Hanak B. W., Bonow R. H., Harris C. A., Browd S. R. (2017). Cerebrosponal fluid shunting complications in children. Pediatr. Neurosurg. 52 (6), 381–400. doi: 10.1159/000452840
Hommelstad J., Madso A., Eide P. K. (2013). Significant reduction of shunt infection rate in children below 1 year of age after implementation of a perioperative protocol. Acta Neurochir (Wien). 155 (3), 523–531. doi: 10.1007/s00701-012-1574-z
Kielian T., Phulwani N. K., Esen N., Syed M. M., Haney A. C., McCastlain K., et al. (2007). MyD88-dependent signals are essential for the host immune response in experimental brain abscess. J. Immunol. 178 (7), 4528–4537. doi: 10.4049/jimmunol.178.7.4528
Klingenberg C., Aarag E., Ronnestad A., Sollid J. E., Abrahamsen T. G., Kjeldsen G., et al. (2005). Coagulase-negative staphylococcal sepsis in neonates. association between antibiotic resistance, biofilm formation and the host inflammatory response. Pediatr. Infect. Dis. J. 24 (9), 817–822. doi: 10.1097/01.inf.0000176735.20008.cd
Kockro R. A., Hampl J. A., Jansen B., Peters G., Scheihing M., Giacomelli R., et al. (2000). Use of scanning electron microscopy to investigate the prophylactic efficacy of rifampin-impregnated CSF shunt catheters. J. Med. Microbiol. 49 (5), 441–450. doi: 10.1099/0022-1317-49-5-441
Kronforst K. D., Mancuso C. J., Pettengill M., Ninkovic J., Power Coombs M. R., Stevens C., et al. (2012). A neonatal model of intravenous staphylococcus epidermidis infection in mice <24 h old enables characterization of early innate immune responses. PloS One 7 (9), e43897. doi: 10.1371/journal.pone.0043897
Kurronen A., Pihlaja R., Pollari E., Kanninen K., Storvik M., Wong G., et al. (2012). Adult and neonatal astrocytes exhibit diverse gene expression profiles in response to beta amyloid ex vivo. World J. Neurosci. 2 (2), 57–67. doi: 10.4236/wjns.2012.22009
Lucas S. M., Rothwell N. J., Gibson R. M. (2006). The role of inflammation in CNS injury and disease. Br. J. Pharmacol. 147 Suppl 1, S232–S240. doi: 10.1038/sj.bjp.0706400
Mack D., Haeder M., Siemssen N., Laufs R. (1996). Association of biofilm production of coagulase-negative staphylococci with expression of a specific polysaccharide intercellular adhesin. J. Infect. Dis. 174, 881–884. doi: 10.1093/infdis/174.4.881
Sengupta P. (2013). The laboratory rat: Relating its age with human’s. Int. J. Prev. Med. 4 (6), 624–630.
Simon T. D., Pope C. E., Browd S. R., Ojemann J. G., Riva-Cambrin J., Mayer-Hamblett N., et al. (2014). Evaluation of microbial bacterial and fungal diversity in cerebrospinal fluid shunt infection. PloS One 9 (1), e83229. doi: 10.1371/journal.pone.0083229
Snowden J. N. (2014). Generation of a central nervous system catheter-associated infection in mice with Staphylococcus epidermidis. Methods Mol. Biol. 1106, 193–198. doi: 10.1007/978-1-62703-736-5_18
Snowden J. N., Beaver M., Beenken K., Smeltzer M., Horswill A. R., Kielian T. (2013). Staphylococcus aureus sarA regulates inflammation and colonization during central nervous system biofilm formation. PloS One 8, e84089. doi: 10.1371/journal.pone.0084089
Snowden J. N., Beaver M., Smeltzer M. S., Kielian T. (2012). Biofilm-infected intracerebroventricular shunts elicit inflammation within the central nervous system. Infect. Immun. 80, 3206–3214. doi: 10.1128/IAI.00645-12
Spencer S. J., Galic M. A., Pittman Q. J. (2011). Neonatal programming of innate immune function. Am. J. Physiol. Endocrinol. Metab. 300 (1), E11–E18. doi: 10.1152/ajpendo.00516.2010
Strunk T., Prosser A., Levy O., Philbin V., Simmer K., Doherty D., et al. (2012). Responsiveness of human monocytes to the commensal bacterium staphylococcus epidermidis develops late in gestation. Pediatr. Res. 72 (1), 10–18. doi: 10.1038/pr.2012.48
Thanert R., Goldmann O., Beineke A., Medina E. (2017). Host-inherent variability influences the transcriptional response of Staphylococcus aureus during in vivo infection. Nat. Commun. 8, 14268. doi: 10.1038/ncomms14268
Varner M. W., Marshall N. E., Rouse D. J., Jablonski K., Leveno K., Reddy U., et al. (2015). The association of cord serum cytokines with neurodevelopmental outcomes. Am. J. Perinatol. 30 (2), 115–22. doi: 10.1055/s-0034-1376185
Vinchon M., Baroncini M., Delestret I. (2012). Adult outcome of pediatric hydrocephalus. Childs Nerv Syst. 28 (6), 847–854. doi: 10.1007/s00381-012-1723-y
Vinchon M., Dhellemmes P. (2006). Cerebrospinal fluid shunt infection: Risk factors and long-term follow-up. Childs Nerv Syst. 22 (7), 692–697. doi: 10.1007/s00381-005-0037-8
Keywords: Staphylococcus epidermidis, infant, central nervous system, cytokines, chemokines, biofilm, catheter, shunt
Citation: Skar GL, Eaton K, Beaver M, White B, Harris M and Snowden JN (2023) Attenuated inflammatory response in infant mice with Staphylococcus epidermidis CNS catheter infection. Front. Bacteriol. 2:1287779. doi: 10.3389/fbrio.2023.1287779
Received: 02 September 2023; Accepted: 07 November 2023;
Published: 04 December 2023.
Edited by:
Myron Christodoulides, University of Southampton, United KingdomReviewed by:
Keer Sun, University of Texas Medical Branch at Galvesto, United StatesCopyright © 2023 Skar, Eaton, Beaver, White, Harris and Snowden. This is an open-access article distributed under the terms of the Creative Commons Attribution License (CC BY). The use, distribution or reproduction in other forums is permitted, provided the original author(s) and the copyright owner(s) are credited and that the original publication in this journal is cited, in accordance with accepted academic practice. No use, distribution or reproduction is permitted which does not comply with these terms.
*Correspondence: Jessica N. Snowden, anNub3dkZW5AdWFtcy5lZHU=
Disclaimer: All claims expressed in this article are solely those of the authors and do not necessarily represent those of their affiliated organizations, or those of the publisher, the editors and the reviewers. Any product that may be evaluated in this article or claim that may be made by its manufacturer is not guaranteed or endorsed by the publisher.
Research integrity at Frontiers
Learn more about the work of our research integrity team to safeguard the quality of each article we publish.