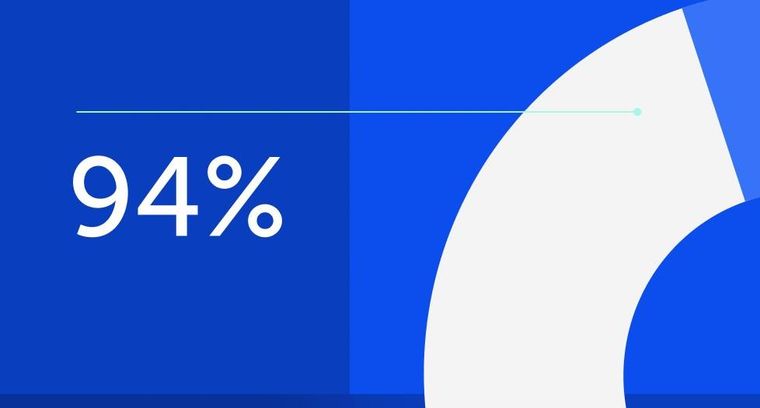
94% of researchers rate our articles as excellent or good
Learn more about the work of our research integrity team to safeguard the quality of each article we publish.
Find out more
ORIGINAL RESEARCH article
Front. Bird Sci., 02 February 2024
Sec. Bird Ecology and Behavior
Volume 3 - 2024 | https://doi.org/10.3389/fbirs.2024.1307628
This article is part of the Research TopicSeasonal, Cross-Seasonal, and Carry-Over Effects of Global Change on Migratory BirdsView all 3 articles
The reproductive phenology of many long-distance migrants breeding at high latitudes remains unresponsive or responds only slightly to climate warming. In order to adjust migration speed and arrival time on the breeding grounds, environmental cues encountered during the migration must be related to conditions prevailing at the breeding site. However, heterogeneity in rate of climate warming across latitudes may hamper the ability of birds to adjust their migration speed adequately. We examined to what extent temperatures encountered at stopovers were a good predictor of temperatures at subsequent stopovers and at the breeding site in greater snow geese (Anser caerulescens atlanticus), an Arctic species that showed little change in its breeding phenology over the past 3 decades. We also examined temperature trends at various stopovers used by geese and at the breeding site over a 40-year period. We found that warming was stronger at the Arctic stopovers and the breeding site than at southern staging areas. The mean temperature and rate of temperature change encountered at the southern stopover, where this species accumulates most of its body reserves, was weakly correlated to temperatures prevailing at more northern stopovers and the breeding site located 3,000 km further north. Correlations in temperatures between stopovers and the breeding site increased with decreasing distance between sites and as geese moved further north. Lastly, laying date was strongly related to temperature during the arrival and pre-laying period on the breeding grounds but not to temperatures encountered at stopovers during migration. Temperatures encountered along the migratory route are thus a poor predictor of temperatures prevailing at the breeding site in any given year except when geese are getting close to their breeding grounds. This may be a major constraint preventing geese from adjusting their migratory schedule to conditions on their breeding grounds and may explain why their reproductive phenology did not change despite a warming trend at the breeding site.
Migrating animals often travel over long distances to exploit seasonal resource pulses occurring at high latitudes during the summer (Alerstam et al., 2003; Shaffer et al., 2006). In birds, environmental conditions encountered during spring migration can influence the timing of breeding and reproductive success due to carry-over effects (Studds and Marra, 2005; Legagneux et al., 2012; Harrison et al., 2013). Bird migration requires a considerable amount of energy and most long-distance migrants have to make multiple stops along the route in order to rest and replenish energy stores (Shaffer et al., 2006). Staging areas typically provide birds with abundant and high-quality food resources, but their timing and availability depend on inter-annual fluctuation in environmental conditions (Tøttrup et al., 2008).
Departure time of long-distance migrants from wintering grounds is mainly triggered by the photoperiod, a fixed cue independent from environmental conditions (Gwinner, 1996; Bauer et al., 2008). As migrating birds approach their breeding grounds, they should fine-tune their movements using environmental cues such as temperature and food availability to cope with inter-annual variation in conditions (Marra et al., 2005; Tøttrup et al., 2008). Although many weather-related factors can influence migration speed, temperature remains one of the most important (Bauer et al., 2008; Tøttrup et al., 2010; Haest et al., 2018; Burnside et al., 2021). Temperature is a key determinant of biological processes such as the onset of plant growth or insect emergence (Ovaskainen et al., 2013) and thus influences refueling opportunities at stopovers. It was proposed that herbivorous birds moving northwards in spring surf on a “green wave” as they continuously encounter nutritious young plants at successive stopovers due to the staggered onset of vegetation growth with latitude (Shariatinajafabadi et al., 2014; Kölzsch et al., 2015; Lameris et al., 2018; but see Wang et al., 2019). Birds should time their migratory movements in order to benefit from optimal feeding conditions because individuals arriving too early or too late at staging areas may face reduced feeding opportunities.
Arrival time on the breeding grounds has a strong influence on timing of breeding and reproductive success of migratory birds (Saino et al., 2004). This is especially true in Arctic ecosystems due to the short breeding season and narrow peak of food availability in summer. Arriving at the optimal time depends on the capacity of birds to anticipate environmental conditions on the breeding grounds using available information along the flyway (Marra et al., 2005). Because seasonality increases with latitude, environmental cues such as temperature and food availability are likely to become more reliable to anticipate conditions further north, when birds approach their breeding grounds (Bauer et al., 2008). As birds advance in their migration, potential adjustments in migration speed will be increasingly constrained by staging duration and departure time from previous stopovers (Nilsson et al., 2013; Schmaljohann and Both, 2017). Therefore, temperature and feeding conditions encountered at successive staging areas and upon arrival in the Arctic may both influence the timing of breeding.
Migrating birds have experienced climate warming across much of the northern hemisphere in recent decades, but the rate of warming has been faster at higher than lower latitudes (Francis et al., 2017; Rantanen et al., 2022). Uneven rates of climate warming along the flyway may create a mismatch between conditions encountered at successive stopovers (Both and Marvelde, 2007). For herbivores, this means that their migration schedule may no longer be in phase with the ‘green wave’, leading to a mismatch between feeding requirements of migrants and vegetation availability at staging areas. This may have important consequences on their body condition and future reproduction.
The greater snow goose is a long-distance migrant that must accumulate large fat stores at spring stopovers for migration and subsequent reproduction. Despite a warming trend on the breeding grounds, its mean laying date has remained constant over the last three decades (Gauthier et al., 2013; Reséndiz-Infante et al., 2020), leading to potential mismatch between hatching of goslings and the timing of peak food availability (Doiron et al., 2015). We examined factors that could explain the lack of response in breeding phenology of this goose population to warming temperatures. First, we determined to what extent warming trends differ among spring staging areas and the breeding grounds, which could limit the ability of migrating geese to adjust their breeding phenology. Secondly, we hypothesized that conditions encountered at their temperate staging areas could be a poor predictor of conditions prevailing at their Arctic staging and breeding sites, which would prevent birds from adjusting their migration schedule and arrival time to conditions on the breeding grounds. To test this idea, we examined if mean temperature or its rate of change encountered at any given stopover during the migration was correlated with temperatures at subsequent stopovers and ultimately during the arrival and pre-laying period on the breeding grounds in the same year. We expected these correlations to be weak, especially between temperate and Arctic staging areas, because birds move across different climate zones. Lastly, previous studies had shown that laying date in this population was related to temperature prevailing during the pre-laying and laying period on the breeding grounds (Dickey et al., 2008). We tested if laying date could also be affected by temperatures encountered at various stopovers during spring migration.
The greater snow goose winters along the Atlantic coast of the United States (Gauthier et al., 2005). At the end of the winter, in late March, they migrate to the St. Lawrence Valley in southern Quebec where they stage for 6 to 8 weeks (Figure 1; Béchet et al., 2003). During that period, they gradually move downriver and northeastward. Geese accumulate most of their body reserves for the subsequent migration to the Arctic and reproduction while staging along the St. Lawrence River (Gauthier et al., 1992). In these areas, geese feed on waste corn, new growth in hayfields and on coastal marsh plants (bulrush, Schoenoplectus americanus and cordgrass, Spartina alterniflora; Gauthier et al., 2005).
Greater snow geese breed across the eastern Canadian Arctic Archipelago, north of ca. 68°N (Gauthier et al., 2005). The largest colony is located on Bylot Island (73°N, 80°W), where the breeding ecology of the population has been studied for the past 30 years (Gauthier et al., 2013; Reséndiz-Infante et al., 2020). Geese depart from the St. Lawrence Valley for the Arctic in mid-May (see below) and migrate to the Bylot Island colony, a distance of ca. 3,000 km covered in 1 to 2 weeks (Gauthier et al., 1992; Bêty et al., 2003). Geese are known to stop in two regions in between. A first area is along river valleys throughout a large portion of Nunavik above the tree line (LeHenaff et al., 1995), a low Arctic region dominated by low shrubs, mainly dwarf birch (Betula nana) and willows (Salix spp). A second area is located further north in low-lying coastal areas of north Foxe Basin and further inland on Baffin Island (Matt Evans, personal communication, 2007), an area where some birds also breed. Duration of staging at these two Arctic sites is likely variable among individuals and years (see below) and it is unknown if all birds stop at both sites or if one may be overflown. Average distances traveled by geese between these stopovers are 1,470 km from the St. Lawrence Valley to Nunavik, 1,300 km from Nunavik to Baffin Island and 400 km from Baffin Island to the breeding colony of Bylot Island (distance based on the centroid of area used at each site).
When geese arrive in these northern areas in late May, most of the ground is still covered by snow and feeding opportunities may be limited. Upon arrival on Bylot Island, geese concentrate on the few snow-free patches located on south-facing slopes where they can feed prior to laying (Gauthier, 1993). Egg production relies on a combination of nutrient stores accumulated by females at southern staging areas and food acquired locally in the Arctic during the pre-laying period (Gauthier et al., 2003). Reproductive success is strongly related to the timing of breeding, being highest in early breeders (Lepage et al., 2000).
Areas used by geese during their stopover in the St. Lawrence Valley are well known. We delimited this stopover based on the citizen science database eBird (Sullivan et al., 2009) and on scientific studies (Reed et al., 1998; Béchet et al., 2003; Gauthier et al., 2005). Although geese gradually move downriver along the St. Lawrence Valley as spring progresses, the area was considered as a single stopover because the whole area is used by geese during spring and temperatures are highly correlated among different sites within this area. Stopover areas in Nunavik and on Baffin Island were delimited using: (1) locations of birds (n = 54) marked on Bylot Island with satellite transmitters and tracked from 2008 to 2011 (Joël Bêty, unpubl. data), (2) data from aerial surveys conducted in 2006 during environmental assessment studies for industrial projects in Nunavut (Matt Evans, personal communication, 2007) and (3) the literature (LeHenaff et al., 1995). In these two regions, stopovers were defined as the area where groups of radio tracked geese (n > 2 birds) were observed to stay >2 days. Although not all marked birds migrated to the breeding colony of Bylot Island, data was still adequate to coarsely delimit staging areas used by snow geese during spring migration. Polygons for the three stopover areas were refined according to known goose habitat preferences using vegetation and elevation digital layers in shapefile format obtained from Natural Resources Canada (2018). Finally, the breeding area for this study was defined as the south plain of Bylot Island where a large snow goose colony is located (Gauthier et al., 2013). See Figure 1 for the delimitation of the stopovers and breeding area used in this study.
To extract temperature data, we had to define periods when geese were most likely to be present at each stopover and during the period of arrival and pre-laying on the breeding grounds. Geese gradually arrive in the St. Lawrence Valley during the last two weeks of March and are present until mid-May (Béchet et al., 2003; Gauthier et al., 2005). Departure of geese from the St. Lawrence occurs rather synchronously in mid-May (Supplementary Material 1, Supplementary Table S1). We thus defined the period of goose presence in the St. Lawrence estuary from 1 April to 15 May. Ground observations at the Bylot Island colony over a 30-year period as well as radio-tracking of geese in some years show that peak arrival of geese at the site occurs in early June although some geese are already present in late May (Supplementary Material 1, Supplementary Table S2; Choinière and Gauthier, 1995). We thus defined the arrival and pre-laying period of geese at the colony from 30 May to 15 June.
Information regarding the timing of goose presence in Nunavik and on Baffin Island is more fragmentary. During caribou surveys conducted in spring in Nunavik, 75 snow goose flocks were observed between 29 May-8 June 1975, 20 flocks between 29 May-3 June 1977 and 28 flocks between 31 May-4 June 1983 (LeHenaff et al., 1995). All goose flocks were observed in tundra habitat, which corresponds to the southern limit of the goose staging area delimited on Figure 1. In the north Baffin region, surveys conducted for an industrial project detected geese in the area during the first two weeks of June in the early 2000s (Matt Evans, personal communication, 2007). Finally, some geese were individually tracked with satellite transmitters in the northern areas in 2008-2010 (Supplementary Material 1, Supplementary Tables S3, S4). Based on that, and the known departure date of geese from the St. Lawrence estuary and arrival date at Bylot Island, we defined the period of presence of geese in Nunavik as 10 to 31 May and on Baffin Island as 20 May to 5 June. More recent radio-tracking studies of snow geese (2019-2022) provided additional information on their migration phenology at the individual level. Information extracted from these data largely confirmed the dates used to determine the period of goose presence in these two regions (Supplementary Material 1, Supplementary Tables S3, S4).
We obtained daily temperature data from 1979 to 2018 for each of the delimited stopover areas and the breeding site on Bylot Island from the high-resolution North American Regional Reanalysis (NARR, pixel = 32 km) produced by the National Centers for Environmental Prediction (https://psl.noaa.gov/data/gridded/data.narr.html; Mesinger et al., 2006). Temperature data from weather stations are very scarce for the Arctic region. Reanalysis methods integrate remote sensing data with observational ground data into multidimensional datasets that provide a continuous spatial and temporal time series of temperature. We nonetheless validated temperature data from the reanalysis with observational data from weather stations at a few sites (see Supplementary Material 2, Supplementary Table S5).
We extracted temperature data for the time periods defined above at each stopover and the breeding site. Because of the lengthy period that geese stage in the St. Lawrence Valley, we also used a shorter sub-period similar in length to other sites, from 1-15 May, which corresponds to the end of the staging there. There was some overlap between successive periods due to inter-individual differences in the time that geese arrive and depart at each site. Daily mean temperatures were extracted from reanalysis data to create subsets for each of the areas and time periods. Datasets and digital layers were processed in ArcGIS 10.4 and R 3.2.3 (R Core Team, 2015). Data on laying dates of the population breeding on Bylot Island were collected from 1991 to 2018 (for details, see Reséndiz-Infante et al., 2020; data source: Gauthier and Cadieux, 2020).
We calculated average annual temperature for each area defined above and time period based on daily values. A preliminary analysis revealed the presence of autocorrelation in most time series. Because of that, we first fitted auto-regressive integrated moving average (ARIMA) models for each period and study site (methodological details are provided in Supplementary Material 3). We calculated temperature trends for each area and time period when geese are present during spring migration (mean annual values) for the years 1979–2018 using ARIMA models.
We measured the degree of correlation in temperatures between areas and periods for the same year from 1979 to 2018. We used the residuals from the models selected in the ARIMA analyses to conduct the correlation analyses. We first correlated mean temperatures in the St. Lawrence Valley (both periods, i.e. 1 April - 15 May or 1 - 15 May) to mean temperatures in Nunavik, Baffin Island and Bylot Island for the same time periods and for later periods (10 - 31 May, 20 May - 5 June, and 30 May - 15 June). In the next analysis, we correlated Nunavik mean temperatures for the period 10 - 31 May to Baffin Island and Bylot Island mean temperatures during the same period and during 20 May - 5 June and 30 May - 15 June. Finally, we correlated Baffin Island mean temperature for the period 20 May - 5 June to those on Bylot Island during the same period and during 30 May - 15 June. We also calculated the annual rate of change in temperatures at each site. Using daily temperature data, we conducted linear regression analyses for each year, site and time period. The slope of these annual relationships was correlated to the slope from another area for the same or the subsequent period following the same logic as for mean temperatures (see above).
When examining correlations, we must consider not only statistical significance but also the strength of the correlation, which provides an index of effect size. Here, we considered correlation coefficients (r) weak when <0.4, moderate when between 0.4 and 0.7 and strong when >0.7.
Finally, we examined the influence of temperatures encountered by geese at various stopovers and during the arrival and pre-laying period at the breeding site on laying dates with regression analysis for the period 1991–2018. To do so, we built a set of models using different combinations of stopover areas along with the breeding site. Model selection and model averaging was based on AIC using the R package AICmodavg (Mazerolle, 2019).
Over the period 1979–2018, temperatures between 1 April and 15 May increased by 0.5°C in the St. Lawrence Valley (slope = 0.013°C/yr, 95% CI = -0.022, 0.470; Figure 2; Supplementary Table S6), 2.1°C in Nunavik (slope = 0.053°C/yr, 95% CI = 0.001, 0.106), 3.4°C on Baffin Island (slope = 0.085°C/yr, 95% CI = 0.049, 0.120), and 2.6°C at the breeding site (slope = 0.065°C/yr, 95% CI = 0.030, 0.099). During the staging of geese in the Arctic, temperatures warmed by 1.6°C in Nunavik between 10 and 31 May (slope = 0.039°C/yr, 95% CI = -0.044, 0.122) and 1.1°C on Baffin Island between 20 May and 5 June (slope = 0.028°C/yr, 95% CI = 0.007, 0.050). Finally, at Bylot Island, temperatures warmed by 0.85°C between 30 May and 15 June (slope = 0.021°C/yr, 95% CI = -0.002, 0.044).
Figure 2 Warming trends in mean temperatures over 40 years (1979–2018) at stopovers and the breeding site of the greater snow goose.
We first examined if temperatures at the various stopovers and during the arrival and pre-laying period on the breeding grounds were correlated for the same time periods (Table 1; Supplementary Figure S1). Mean temperature during the whole staging period along the St. Lawrence Valley was significantly correlated to mean temperatures in Nunavik and Baffin Island at the same time, although in the latter case the correlation coefficient was weak (r = 0.31). However, when restricting the analysis to the last two weeks of staging in the St. Lawrence (1-15 May), the correlation with Baffin Island was no longer significant and became weak with Nunavik (r = 0.33). Mean temperatures between Nunavik, Baffin Island and Bylot Island were all significantly correlated (Table 1; Supplementary Figure S1), but the correlation coefficient was strong (r = 0.80) only between the last two sites, which were the closest ones.
Table 1 Pairwise correlations in temperature between stopovers or the breeding site (Bylot Island) of greater snow geese for the same time periods over a 40-year time span (1979–2018).
Next, we examined how temperatures encountered at each stopover were a good predictor of temperatures encountered by geese at the next time period in areas further north. In all cases, correlations were weaker than in the previous analysis (Table 2; Supplementary Figure S2). Mean temperature along the St. Lawrence was significantly correlated with mean temperature at the next time period in Nunavik, although the correlation coefficient was relatively weak (r = 0.37), and not correlated with temperatures in areas further north (Supplementary Figure S2A). All correlation coefficients were weak and non-significant when restricting the analysis to the last two weeks of staging in the St. Lawrence (Supplementary Figure S2B). Mean temperature in Nunavik was significantly correlated with temperature at the next time period on Baffin Island but not during the arrival and pre-laying period on Bylot Island (Table 2; Supplementary Figure S2C). Mean temperature on Baffin Island was also significantly correlated to temperature during the arrival and pre-laying period on Bylot Island. In both cases, correlation coefficients were relatively weak (r = 0.35).
Table 2 Pairwise correlations in temperature between stopovers or the breeding site (Bylot Island) of greater snow geese for subsequent time periods over a 40-year time span (1979–2018).
Analyses based on the rate of change of temperature revealed the same pattern as with mean temperatures except that most correlations were weaker (all r ≤ 0.33) and almost all were non-significant, either for the same or the next time periods between sites (Supplementary Figures S3, S4). The only strong correlation was for the rate of temperature change between Baffin and Bylot Islands for the same time period (r = 0.77. P < 0.001), but it disappeared when comparing successive periods for those sites.
In our analysis of the effect of temperatures encountered by geese at stopover sites on laying date, there were five competitive models (ΔAIC < 2) with various effects (Supplementary Table S7). All these models retained an effect of temperature on Bylot Island during the arrival and pre-laying period, but three models including the top one also retained an effect of temperature at the Baffin Island staging site. The multi-model inference on the 5 models with ΔAIC < 2 showed that laying date was negatively related to temperature during the arrival and pre-laying period on Bylot Island (model-averaged estimate = -1.34, 95% CI = -2.04, -0.65; Figure 3; Supplementary Table S8). However, laying date was not significantly related to temperatures encountered by geese on Baffin Island despite a negative trend (model-averaged estimate = -0.63, 95% CI -1.39, 0.13). Repeating the analysis using temperatures only during the last part of the staging period along the St. Lawrence (1-15 May) instead of the full period yielded the same results (Supplementary Tables S9, S10).
Figure 3 Relationship between annual mean laying date of greater snow geese and temperature during the arrival and pre-laying period (30 May to 15 June) at the Bylot Island colony.
We used a 40-year temperature dataset to determine if conditions along the migratory route could be used by greater snow geese to anticipate those at sites further north. We found that all sites used by geese in spring experienced recent warming trends but that they were generally more intense in the Arctic than at temperate staging areas, as expected. Mean temperature and rate of change in daily temperatures at spring stopovers were weakly related to those at the next stopover, and generally not related to those at more distant areas or at the breeding site. Therefore, geese would only be able to vaguely predict temperatures to be expected at their next stopover at best. Lastly, the largest influence on laying date was from the temperature encountered during the arrival and pre-laying period at the breeding site.
Warming trends along the spring migratory route were heterogeneous across latitudes and time periods, and warming was generally stronger at northern than at lower latitudes (Rantanen et al., 2022). The weakest warming trend occurred in the temperate region of the St. Lawrence Valley, the most southern stopover. In that region, greater snow geese accumulate most of their body reserves for spring migration and subsequent reproduction (Gauthier et al., 1992). Over the last 40 years, departure dates of geese from the St. Lawrence Valley for the Arctic did not change as most geese depart between 15 and 22 May (Supplementary Material 1, Supplementary Table S1). Due to human-related modifications along the St. Lawrence Valley, geese have switched their feeding behavior from predominantly marsh plants (bulrush, cordgrass) to one dominated by agricultural plants (waste corn, new growth in hayfields), which allowed geese to depart in better condition for the Arctic (Gauthier et al., 2005). However, since 1999 a spring hunt combined with efforts from farmers to scare geese away from croplands has increased disturbance on birds along the St. Lawrence Valley. This has changed their distribution, habitat use and reduced their body condition (Béchet et al., 2003; Féret et al., 2003; Béchet et al., 2004; LeTourneux et al., 2021). In contrast to the temperate region, the Arctic staging areas used by geese in Nunavik and on Baffin Island have not been directly affected by human activities although they have experienced a strong warming trend and an increase in primary productivity (Berner et al., 2020). During the short period when geese are present in spring, these Arctic stopovers have warmed by at least 1 to 2°C, enough to potentially advance snowmelt and improve feeding conditions for geese.
Considering all these changes along the migratory route, we could have expected an advance in departure date of geese for the Arctic to escape human disturbance at lower latitudes and benefit from climate warming effects at northern latitudes. However, this apparently did not occur. Our analysis suggests that the weak warming trend experienced by geese at their temperate stopover may be one of the factors preventing them from departing earlier for the Arctic. Similar to geese, purple martins (Progne subis), and pied flycatchers (Ficedula hypoleuca) have experienced uneven warming across their migratory routes (Ahola et al., 2004; Both and Marvelde, 2007; Fraser et al., 2013), which apparently limited their ability to adjust to conditions prevailing at the breeding site. Nonetheless, the positive warming trends found at all staging areas may have improved feeding conditions for geese, especially for early migrants which often face the harshest weather conditions (Tøttrup et al., 2010), thus enhancing their body condition. Improved body stores in early migrants may in turn be an important factor that allow them to breed early (Reséndiz-Infante and Gauthier, 2020).
Not surprisingly, distance was an important factor in explaining the strength of correlations in temperatures between paired sites during the same time period. Indeed, temperatures between north Baffin Island and Bylot Island, two sites ca. 400 km apart, where highly correlated whereas temperatures between the St. Lawrence Valley and Nunavik or between Nunavik and north Baffin Island, each separated by ca. 1,500 km, were weakly correlated. Considering that the St. Lawrence Valley is in a temperate climate zone whereas Nunavik and Baffin Island are in the Arctic, we could have expected a greater correlation between the two sites within the same climate zone than between sites in different zones (temperate vs Arctic) but this was not the case. The gradual decay of correlations in temperatures with distance means that temperature encountered by geese while staging in the temperate St. Lawrence Valley does not provide much information on temperature prevailing in the Arctic and especially at their breeding site. Therefore, an early spring at a southern staging site does not imply an early spring also at a distant northern site. The same applies for geese that have entered the Arctic climate zone and are staging in Nunavik.
In order to adjust their migration schedule based on conditions encountered along the route, local conditions experienced by geese must provide reliable information on conditions to be expected at the next staging site in the coming days/weeks. However, we found that the predictive power of future conditions was very low, either when using mean temperature or its rate of change over time. This supports our prediction that temperature encountered at temperate staging areas or even low Arctic ones in spring are a weak predictor of those to be expected at or near the Arctic breeding site. Without reliable environmental information, it is difficult for geese to anticipate future temperature and time their migration schedule accordingly. This lack of predictability may explain why several goose populations apparently do not surf on the green wave as previously suggested (Wang et al., 2019). Under these circumstances, photoperiod may remain the best cue to use for a good part of the migration. Other less predictable local conditions (e.g. favorable tail wind) may control departure date from stopover sites within a suitable time window determined by photoperiod, like in the long-distance migrant wood thrush (Hylocichla mustelina, Stanley et al., 2012).
The difficulty to anticipate conditions to be encountered at upcoming staging sites based on current conditions, combined with generally weaker warming occurring at lower compared to higher latitudes, has the potential to lead to mistimed arrival on the breeding grounds. This will limit the ability of geese of benefiting from favorable nesting conditions early in the season, especially in years with an early snow-melt. When this happens, goslings experience a trophic mismatch with the food quality peak, which advances due to early spring, and results in reduced growth and survival of goslings (Doiron et al., 2015; Ross et al., 2018). It is only when geese are getting close to the breeding site (Baffin Island in our case) that local temperature can be informative of temperature on the breeding grounds. Based on that, geese may either overfly their last staging area to speed up migration when spring is early or stop and wait if spring is late. However, if spring is early, overflying their last staging area should result only in a minor advance in arrival time on the breeding grounds, which would prevent birds from taking full advantage of suitable feeding conditions. Because females need time to recover body condition to start laying eggs (Bêty et al., 2003), this would explain why in early springs geese lay after the 50% snow melt date, contrary to the situation in late years (Gauthier et al., 2013). Ground counts conducted on Bylot Island for the past 25 years supports this idea as they show no temporal trend in goose arrival date (Supplementary Material 1, Supplementary Table S2).
The most important predictor of laying date was temperature during the arrival and pre-laying period at the breeding site. This is likely because resources acquired on the breeding grounds account for a large part of the energy invested in egg production due to a mixed capital/income breeding strategy (Gauthier et al., 2003). Temperatures prevailing at that time will determine snow-melt, which will have a direct effect on food availability and also on nest site availability, another factor that can affect laying date (Dickey et al., 2008).
We would have expected that temperatures encountered at other stopovers, and especially in the St. Lawrence Valley where accumulation of most body reserves takes place in spring (Gauthier et al., 1992), would have an influence on laying date. Other studies had documented carry-over effects of environmental conditions in spring on the breeding performance in this population (Bêty et al., 2003; Legagneux et al., 2012). However, these carry-over effects appear to be more important when conditions on the breeding grounds are less favorable for breeding (Legagneux et al., 2012; Grandmont et al., 2023). Thus, it is possible that geese evolved their mixed capital/income breeding strategy to cope with the high environmental variability in the Arctic. For instance, poor conditions encountered at southern staging areas in spring could be somewhat compensated by good conditions encountered at arrival on the breeding grounds, or vice versa. Such situations may be frequent as our analysis showed a low correlation between temperatures encountered at distant stopovers and those during the arrival and pre-laying period in the Arctic in the same year. We also found a weak positive effect of temperature encountered at the last staging area on Baffin Island on laying date. Given the proximity between this staging site and the breeding site, egg formation may have started by the time geese arrive in north Baffin Island, and thus feeding taking place at this site may be part of the pre-laying period and may influence egg formation.
In this study, we lacked data at the individual level on migration chronology and subsequent reproductive performance. Detailed information on individual departure dates from temperate staging areas and arrival dates on the Arctic breeding grounds, combined with nesting success data, are available for only a few years and a small number of individuals in this species (Bêty et al., 2003; Grandmont et al., 2023). This is not uncommon considering that tracking migration and reproduction of long-distance migrants at the individual level requires the use of expensive technology such as satellite telemetry, which considerably limits sample size (Shariatinajafabadi et al., 2014; Kölzsch et al., 2015). Some studies nonetheless showed that while migration speed and chronology often differ among individuals within populations, migratory behavior tends to be repeatable within individuals (Bêty et al., 2004; Stanley et al., 2012; Fraser et al., 2013). It is thus important to study directly the effects of warming on individual variation in timing of migration because climate change may not affect early and late migrants equally (Tøttrup et al., 2010).
Our limited knowledge of the behavior of geese at the Arctic staging areas (Nunavik and Baffin Island) also prevents us from determining precisely stopover duration or to what extent geese are adjusting their migration speed (Schmaljohann and Both, 2017). This uncertainty may have weakened some of the correlations between temperatures encountered by geese at these Arctic sites. Finally, we considered only temperature in our analysis and geese may also use other environmental cues such as wind direction in their migratory decisions (Stanley et al., 2012; Covino et al., 2015; Burnside et al., 2021). However, we believe that it is unlikely that other environmental factors not related to temperature could be used as predictors of conditions likely to be encountered by geese at subsequent stopovers.
Overall, our results show that temperatures encountered along the migratory route of the greater snow goose are not a good predictor of temperatures occurring at more northern stopovers or on the breeding grounds. It is only when geese are getting close to their breeding site, i.e. within a few hundred kilometers, that temperatures encountered are similar to those prevailing on the breeding grounds but by then it may be too late to adjust migration speed to significantly advance arrival time on the breeding grounds in early spring years. To our knowlkedge, this is one of the first comprehensive assessments of predictability of conditions encountered along the migration route of an arctic-nesting species. Moreover, their northern staging areas and breeding grounds are warming at a faster rate than southern staging areas, which further increases the potential for mistimed arrival on the breeding grounds. This limits the ability of this species to adjust its breeding phenology to a changing environment and can explain why laying date did not advance over the last three decades despite climate warming (Gauthier et al., 2013; Reséndiz-Infante et al., 2020). It would be interesting to repeat similar analyses in species where laying date has advanced in response to climate warming to determine if this is due to shorter migration distance, and therefore potentially more correlated temperature among stopovers, or differences in plasticity among species.
The original contributions presented in the study are included in the article/Supplementary Material. Further inquiries can be directed to the corresponding author.
The animal study was approved by the Comité de protection des animaux de laboratoire de l’Université Laval. The study was conducted in accordance with the local legislation and institutional requirements.
CR-I: Conceptualization, Data curation, Formal analysis, Writing – original draft. GG: Conceptualization, Funding acquisition, Supervision, Writing – review & editing.
The author(s) declare financial support was received for the research, authorship, and/or publication of this article. Funding was provided by the Natural Sciences and Engineering Research Council of Canada, the ArcticNet Network of Centres of Excellence of Canada, the Canadian Wildlife Service (Environment and Climate Change Canada), the Fonds de recherche Nature et Technologie du Québec, the Northern Student Training Program of Polar Knowledge Canada, the Polar Continental Shelf Program of Natural Resources Canada and the National Council for Science and Technology (CONACYT Mexico).
North American Regional Reanalysis (NARR) data were provided by the NOAA Physical Sciences Laboratory, Boulder, Colorado, USA. We thank Marie-Christine Cadieux for her help with fieldwork logistics and database management, and numerous summer assistants for field data collection. We also thank Joël Bêty and Jean-Pierre Tremblay for their insight and expertise throughout this work. We thank Pierre Legagneux and Thierry Grandmont for providing and compiling data from radio-tracking of snow geese from 2019 to 2022.
The authors declare that the research was conducted in the absence of any commercial or financial relationships that could be construed as a potential conflict of interest.
All claims expressed in this article are solely those of the authors and do not necessarily represent those of their affiliated organizations, or those of the publisher, the editors and the reviewers. Any product that may be evaluated in this article, or claim that may be made by its manufacturer, is not guaranteed or endorsed by the publisher.
The Supplementary Material for this article can be found online at: https://www.frontiersin.org/articles/10.3389/fbirs.2024.1307628/full#supplementary-material
Ahola M., Laaksonen T., Sippola K., Eeva T., Rainio K., Lehikoinen E. (2004). Variation in climate warming along the migration route uncouples arrival and breeding dates. Glob. Change Biol. 10, 1610–1617. doi: 10.1111/j.1365-2486.2004.00823.x
Alerstam T., Hedenström A., Åkesson S. (2003). Long-distance migration: evolution and determinants. Oikos 103, 247–260. doi: 10.1034/j1600-0706.2003.12559x
Bauer S., Gienapp P., Madsen J. (2008). The relevance of environmental conditions for departure decision changes en route in migrating geese. Ecology 89, 1953–1960. doi: 10.1890/07-1101.1
Béchet A., Giroux J.-F., Gauthier G. (2004). The effects of disturbance on behaviour, habitat use and energy of spring staging snow geese. J. Appl. Ecol. 41, 689–700. doi: 10.1111/j.0021-8901.2004.00928.x
Béchet A., Giroux J.-F., Gauthier G., Nichols J. D., Hines J. E. (2003). Spring hunting changes the regional movements of migrating greater snow geese. J. Appl. Ecol. 40, 553–564. doi: 10.1046/j.1365-2664.2003.00812.x
Berner L. T., Massey R., Jantz P., Forbes B. C., Macias-Fauria M., Myers-Smith I., et al. (2020). Summer warming explains widespread but not uniform greening in the Arctic tundra biome. Nat. Commun. 11, 4621. doi: 10.1038/s41467-020-18479-5
Bêty J., Gauthier G., Giroux J.-F. (2003). Body condition, migration, and timing of reproduction in snow geese: a test of the condition-dependent model of optimal clutch size. Am. Nat. 162, 110–121. doi: 10.1086/375680
Bêty J., Giroux J.-F., Gauthier G. (2004). Individual variation in timing of migration: causes and reproductive consequences in greater snow geese (Anser caerulescens atlanticus). Behav. Ecol. Sociobiol. 57, 1–8. doi: 10.1007/s00265-004-0840-3
Both C., Marvelde L. T. (2007). Climate change and timing of avian breeding and migration throughout Europe. Clim. Res. 35, 93–105. doi: 10.3354/cr00716
Burnside R. J., Salliss D., Collar N. J., Dolman P. M. (2021). Birds use individually consistent temperature cues to time their migration departure. Proc. Natl. Acad. Sci. U. S. A. 118, e2026378118. doi: 10.1073/pnas.2026378118
Choinière L., Gauthier G. (1995). Energetics of reproduction in female and male greater snow geese. Oecologia 103, 379–389. doi: 10.1007/BF00328628
Covino K. M., Holberton R. L., Morris S. R. (2015). Factors influencing migratory decisions made by songbirds on spring stopover. J. Avian Biol. 46, 73–80. doi: 10.1111/jav.00463
Dickey M.-H., Gauthier G., Cadieux M.-C. (2008). Climatic effects on the breeding phenology and reproductive success of an arctic-nesting goose species. Glob. Change Biol. 14, 1973–1985. doi: 10.1111/j.1365-2486.2008.01622.x
Doiron M., Gauthier G., Lévesque E. (2015). Trophic mismatch and its effects on the growth of young in an Arctic herbivore. Glob. Change Biol. 21, 4364–4376. doi: 10.1111/gcb13057
Féret M., Gauthier G., Béchet A., Giroux J.-F., Hobson K. A. (2003). Effect of a spring hunt on nutrient storage by greater snow geese in southern Quebec. J. Wildl. Manage. 67, 796–807. doi: 10.2307/3802687
Francis J. A., Vavrus S. J., Cohen J. (2017). Amplified Arctic warming and mid-latitude weather: new perspectives on emerging connections. WIREs Clim. Change 8, e474. doi: 10.1002/wcc474
Fraser K. C., Silverio C., Kramer P., Mickle N., Aeppli R., Stutchbury B. J. M. (2013). A trans-hemispheric migratory songbird does not advance spring schedules or increase migration rate in response to record-setting temperatures at breeding sites. PloS One 8, e64587. doi: 10.1371/journal.pone.0064587
Gauthier G. (1993). Feeding ecology of nesting greater snow geese. J. Wildl. Manage. 57, 216–223. doi: 10.2307/3809416
Gauthier G., Bêty J., Cadieux M. C., Legagneux P., Doiron M., Chevallier C., et al. (2013). Long-term monitoring at multiple trophic levels suggests heterogeneity in responses to climate change in the Canadian Arctic tundra. Philos. Trans. R. Soc B. 368, 20120482. doi: 10.1098/rstb.2012.0482
Gauthier G., Bêty J., Hobson K. A. (2003). Are greater snow geese capital breeders? New evidence from a stable-isotope model. Ecology 84, 3250–3264. doi: 10.1890/02-0613
Gauthier G., Cadieux M. C. (2020). Monitoring of Greater Snow Goose reproduction on Bylot Island, Nunavut, Canada, v. 1.1 (1989-2019). Nordicana, D41. doi: 10.5885/45570CE-2D00DCA728074FA7
Gauthier G., Giroux J.-F., Beídard J. (1992). Dynamics of fat and protein reserves during winter and spring migration in greater snow geese. Can. J. Zool. 70, 2077–2087. doi: 10.1139/z92-280
Gauthier G., Giroux J.-F., Reed A., Beíchet A., Bélanger L. (2005). Interactions between land use, habitat use and population increase in greater snow geese: what are the consequences for natural wetlands? Glob. Change Biol. 11, 856–868. doi: 10.1111/j.1365-2486.2005.00944.x
Grandmont T., Fast P., Grentzmann I., Gauthier G., Bety J., Legagneux P. (2023). Should I breed or should I go? Manipulating individual state during migration influences breeding decisions in a long-lived bird species. Funct. Ecol. 37, 602–613. doi: 10.1111/1365-2435.14256
Gwinner E. (1996). Circannual clocks in avian reproduction and migration. Ibis 138, 47–63. doi: 10.1111/j.1474-919X.1996.tb04312.x
Haest B., Hüppop O., Bairlein F. (2018). The influence of weather on avian spring migration phenology: What, where and when? Glob. Change Biol. 24, 5769–5788. doi: 10.1111/gcb.14450
Harrison X. A., Hodgson D. J., Inger R., Colhoun K., Gudmundsson G. A., McElwaine G., et al. (2013). Environmental conditions during breeding modify the strength of mass-dependent carry-over effects in a migratory bird. PloS One 8, e77783. doi: 10.1371/journal.pone.0077783
Kölzsch A., Bauer S., Boer R., Griffin L., Cabot D., Exo K.-M., et al. (2015). Forecasting spring from afar? Timing of migration and predictability of phenology along different migration routes of an avian herbivore. J. Anim. Ecol. 84, 272–283. doi: 10.1111/1365-2656.12281
Lameris T. K., van der Jeugd H. P., Eichhorn G., Dokter A. M., Bouten W., Boom M. P., et al. (2018). Arctic geese tune migration to a warming climate but still suffer from a phenological mismatch. Curr. Biol. 28, 2467–2473. doi: 10.1016/j.cub.2018.05.077
Legagneux P., Fast P. L., Gauthier G., Beêty J. (2012). Manipulating individual state during migration provides evidence for carry-over effects modulated by environmental conditions. Proc. R. Soc B 279, 876–883. doi: 10.1098/rspb.2011.1351
LeHenaff D., Ouellet R., Lepage M., Maisonneuve C. (1995). Utilisation du territoire du Nouveau-Québec par l'oie des neiges Chen caerulescens caerulescens en période de migration. Can. Field-Nat. 109, 408–412.
Lepage D., Gauthier G., Menu S. (2000). Reproductive consequences of egg-laying decisions in snow geese. J. Anim. Ecol. 69, 414–427. doi: 10.1046/j.1365-2656.2000.00404.x
LeTourneux F., Grandmont T., Dulude-de Broin F., Martin M. C., Lefebvre J., Kato A., et al. (2021). COVID19-induced reduction in human disturbance accelerates fattening of an overabundant goose species. Biol. Cons. 255, 108968. doi: 10.1016/j.biocon.2021.108968
Marra P. P., Francis C. M., Mulvihill R. S., Moore F. R. (2005). The influence of climate on the timing and rate of spring bird migration. Oecologia 142, 307–315. doi: 10.1007/s.00442-004-1725-x
Mazerolle M. J. (2019). AICcmodavg: Model selection and multimodel inference based on (Q)AIC(c) (R package version 2.2-2). Available at: https://cran.r-project.org/web/packages/AICcmodavg/index.html.
Mesinger F., DiMego G., Kalnay E., Mitchell K., Shafran P. C., Ebisuzaki W., et al. (2006). North american regional reanalysis. Bull. Am. Meteorol. Soc 87, 343–360. doi: 10.1175/BAMS-87-3-343
Natural Resources Canada. (2018). “Geogratis,” in Digital Repository (Ottawa, Canada: Government of Canada). Available at: http://geogratis.gc.ca/geogratis.
Nilsson C., Klaassen R. H. G., Alerstam T. (2013). Differences in speed and duration of bird migration between spring and autumn. Am. Nat. 181, 837–845. doi: 10.1086/670335
Ovaskainen O., Skorokhodova S., Yakovleva M., Sukhov A., Kutenkov A., Kutenkova N., et al. (2013). Community-level phenological response to climate change. Proc. Natl. Acad. Sci. U. S. A. 110, 13434–13439. doi: 10.1073/pnas.1305533110
Rantanen M., Karpechko A. Y., Lipponen A., Nordling K., Hyvärinen O., Ruosteenoja K., et al. (2022). The arctic has warmed nearly four times faster than the globe since 1979. Commun. Earth Env. 3, 168. doi: 10.1038/s43247-022-00498-3
R Core Team. (2015). R: A language and Environment for Statistical Computing. Available at: https://www.R-project.org.
Reed A., Giroux J.-F., Gauthier G. (1998). “Population, size, productivity, harvest, and distribution,” in The Greater Snow Goose: Report of the Arctic Goose Habitat Working Group. Ed. Batt B. D. J. (Ottawa, Ont: Arctic Goose Joint Venture Special Publication, U.S. Fish and Wildlife Service, Washington D.C. and Canadian Wildlife Service).
Reséndiz-Infante C., Gauthier G. (2020). Temporal changes in reproductive success and optimal breeding decisions in a long-distance migratory bird. Sci. Rep. 10, 22067. doi: 10.1038/s41598-020-78565-y
Reséndiz-Infante C., Gauthier G., Souchay G. (2020). Consequences of a changing environment on the breeding phenology and reproductive success components in a long-distance migratory bird. Pop. Ecol. 62, 284–296. doi: 10.1002/1438-390X.12046
Ross M. V., Alisauskas R. T., Douglas D. C., Kellett D. K., Drake K. L. (2018). Density-dependent and phenological mismatch effects on growth and survival in lesser snow and Ross's goslings. J. Avian Biol. 49, e01748. doi: 10.1111/jav.01748
Saino N., Szép T., Romano M., Rubolini D., Spina F., Møller A. P. (2004). Ecological conditions during winter predict arrival date at the breeding quarters in a trans-Saharan migratory bird. Ecol. Lett. 7, 21–25. doi: 10.1046/j.1461-0248.2003.00553.x
Schmaljohann H., Both C. (2017). The limits of modifying migration speed to adjust to climate change. Nat. Clim. Change 7, 573–577. doi: 10.1038/nclimate3336
Shaffer S. A., Tremblay Y., Weimerskirch H., Scott D., Thompson D. R., Sagar P. M., et al. (2006). Migratory shearwaters integrate oceanic resources across the Pacific Ocean in an endless summer. Proc. Natl. Acad. Sci. U. S. A. 103, 12799–12802. doi: 10.1073/pnas.0603715103
Shariatinajafabadi M., Wang T., Skidmore A. K., Toxopeus A. G., Kölzsch A., Nolet B. A., et al. (2014). Migratory herbivorous waterfowl track satellite-derived green wave index. PloS One 9, e108331. doi: 10.1371/journal.pone.0108331
Stanley C. Q., MacPherson M., Fraser K. C., McKinnon E. A., Stutchbury B. J. M. (2012). Repeat tracking of individual songbirds reveals consistent migration timing but flexibility in route. PloS One 7, e40688. doi: 10.1371/journal.pone.0040688
Studds C. E., Marra P. P. (2005). Nonbreeding habitat occupancy and population processes: An upgrade experiment with a migratory bird. Ecology 86, 2380–2385. doi: 10.1890/04-1145
Sullivan B. L., Wood C. L., Iliff M. J., Bonney R. E., Fink D., Kelling S. (2009). eBird: a citizen-based bird observation network in the biological sciences. Biol. Conserv. 142, 2282–2292. doi: 10.1016/j.biocon.2009.05.006
Tøttrup A. P., Rainio K., Coppack T., Lehikoinen E., Rahbek C., Thorup K. (2010). Local temperature fine-tunes the timing of spring migration in birds. Integr. Comp. Biol. 50, 293–304. doi: 10.1093/icb/icq028
Tøttrup A. P., Thorup K., Rainio K., Yosef R., Lehikoinen E., Rahbek C. (2008). Avian migrants adjust migration in response to environmental conditions en route. Biol. Lett. 4, 685–688. doi: 10.1098/rsbl.2008.0290
Keywords: Anser caerulescens atlanticus, long distance migrant, staging areas, climate warming, temperature, timing of reproduction
Citation: Reséndiz-Infante C and Gauthier G (2024) Can arctic migrants adjust their phenology based on temperature encountered during the spring migration? The case of the greater snow goose. Front. Bird Sci. 3:1307628. doi: 10.3389/fbirs.2024.1307628
Received: 04 October 2023; Accepted: 18 January 2024;
Published: 02 February 2024.
Edited by:
W. Douglas Robinson, Oregon State University, United StatesReviewed by:
Kate Layton-Matthews, Norwegian Institute for Nature Research (NINA), NorwayCopyright © 2024 Reséndiz-Infante and Gauthier. This is an open-access article distributed under the terms of the Creative Commons Attribution License (CC BY). The use, distribution or reproduction in other forums is permitted, provided the original author(s) and the copyright owner(s) are credited and that the original publication in this journal is cited, in accordance with accepted academic practice. No use, distribution or reproduction is permitted which does not comply with these terms.
*Correspondence: Gilles Gauthier, Z2lsbGVzLmdhdXRoaWVyQGJpby51bGF2YWwuY2E=
Disclaimer: All claims expressed in this article are solely those of the authors and do not necessarily represent those of their affiliated organizations, or those of the publisher, the editors and the reviewers. Any product that may be evaluated in this article or claim that may be made by its manufacturer is not guaranteed or endorsed by the publisher.
Research integrity at Frontiers
Learn more about the work of our research integrity team to safeguard the quality of each article we publish.