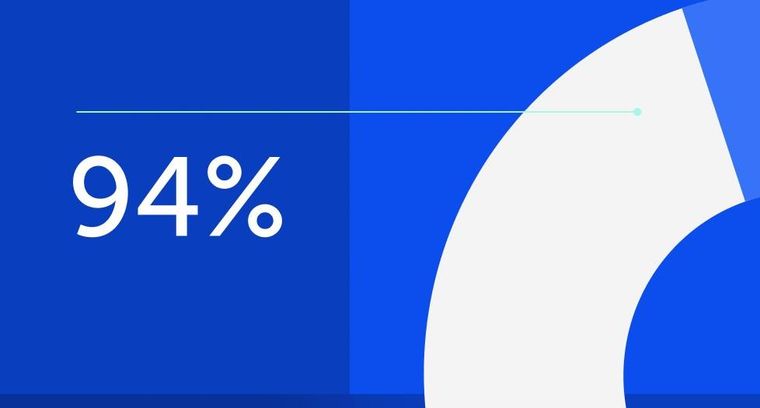
94% of researchers rate our articles as excellent or good
Learn more about the work of our research integrity team to safeguard the quality of each article we publish.
Find out more
ORIGINAL RESEARCH article
Front. Bird Sci., 26 October 2023
Sec. Bird Ecology and Behavior
Volume 2 - 2023 | https://doi.org/10.3389/fbirs.2023.1245853
This article is part of the Research TopicEditors' Showcase: Bird Ecology and BehaviorView all 6 articles
Urban birds’ stress response frequently differs in magnitude from non-urban conspecifics. This urban phenotype may reflect response to selection, sorting during colonization of urban environments, developmental plasticity, or phenotypic flexibility in response to urban environments. We investigated whether exposure to one characteristic of an urban environment, chronic disturbance, could induce an attenuated acute glucocorticoid response over a short time in adult non-urban dark-eyed juncos (Junco hyemalis), which, if true, would support the phenotypic flexibility hypothesis. We tested this during the period of spring gonadal recrudescence. We simulated a high-disturbance urban-like environment by exposing non-urban experimental birds to chronic disturbance (30-min psychological stressors 4x/day for 3 weeks); controls were minimally disturbed. We found that chronically disturbed birds had a lower acute corticosterone response after 3 weeks of treatment. Baseline corticosterone was not affected. Chronically disturbed birds had less body fat and lower body condition than controls at the end of the experiment, although on average all birds gained weight over the course of the experiment. Feathers grown during the experiment did not show an effect of the disturbance treatment on feather corticosterone or fault bars, although captive-grown feathers had lower corticosterone and more fault bars than wild-grown feathers. We conclude that adult male juncos have the capacity to attenuate their acute corticosterone response in an environment with high frequency of disturbance, potentially facilitating colonization of urban habitats. Future research may show whether successful urban colonists differ from unsuccessful species in this regard.
Urban wildlife have repeatedly been shown to have phenotypes that differ from their non-urban conspecifics (e.g., Hendry et al., 2008; Moller, 2008; Bonier, 2012; Lowry et al., 2013; Patankar et al., 2021). The processes that result in these phenotypes are a topic of intense interest (Sol et al., 2013; Ouyang et al., 2019; Bonier, 2023; Deviche et al., 2023). Phenotypic flexibility in adults is one process that could contribute to urban phenotypes, and could facilitate rapid colonization of urban habitats.
Traits relevant to the response to stressors are of special interest in understanding adaptation to urban habitats because frequency of disturbance by potential stressors may be higher in urban habitats (Blumstein, 2014). Urban environments often have a higher density of vertebrate predators such as cats and raccoons compared to rural environments (Haskell et al., 2001), although it is not known whether predation rates differ for urban vs non-urban populations of our study species, the dark-eyed junco (Junco hyemalis). Humans, vehicles, anthropogenic noise, and domestic animals may also be interpreted as potential predators (Frid and Dill, 2002; Davies et al., 2017), and perception of predation risk may affect urban animals regardless of predation rates (Beckerman et al., 2007; Grade et al., 2021; Garitano-Zavala et al., 2022). Here we focus on glucocorticoid hormones because they are known to respond to predation risk (Scheuerlein et al., 2001; Hawlena and Schmitz, 2010; Fischer et al., 2014; Jones et al., 2016) and may mediate physiological and life-history responses to risk such as changes in reproductive rate (Clinchy et al., 2011) and changes in population dynamics (Sheriff et al., 2009; Finn et al., 2023).
Corticosterone is the primary glucocorticoid hormone in birds. It is elevated in response to acute stressors, and coordinates physiological responses that allow animals to survive immediate threats to homeostasis. While corticosterone elevation has short-term benefits, it can come at a cost to long-term processes such as growth and reproduction (Sapolsky et al., 2000), and these costs can accumulate as the duration or frequency of elevations in corticosterone increase (e.g., Busch et al., 2008). Because of these trade-offs, it may be advantageous in some contexts to have a lower corticosterone response to acute stressors (Wingfield, 2013; Romero and Wingfield, 2016). Indeed, studies have shown considerable variation over seasons, species, and individuals in corticosterone physiology (Wingfield et al., 1995; Romero, 2002; Hau et al., 2016; Romero and Wingfield, 2016; Patankar et al., 2021). Novel environments that cause frequent perturbations, such as cities, are hypothesized to favor individuals that down-regulate the acute corticosterone response to avoid the costs of chronic high corticosterone (Wingfield et al., 2015).
Urban animals’ behavioral and physiological responses to anthropogenic disturbance have been studied extensively in birds (e.g., Evans, 2010; Caizergues et al., 2022). Some urban bird species and populations are less reactive than nearby rural birds to human disturbance: they allow closer approach by humans before initiating flight (e.g., Moller, 2008; Atwell et al., 2012), and reduce their territorial behavior less in response to capture stress than rural birds (Abolins-Abols et al., 2016). Urban populations also show differences in the corticosterone response to acute stressors compared to rural birds (Bonier, 2012). These endocrine changes vary in direction: in some species, urban populations are more reactive (e.g., Fokidis et al., 2009) and in others they are less reactive (e.g., Atwell et al., 2012; Rebolo-Ifrán et al., 2015), possibly reflecting differences in the native habitat, populations’ tolerance of urbanization, or the quantity or quality of stressors in urban habitats.
Although urban birds with altered stress-response phenotypes have been found in numerous species, the processes leading to these phenotypes are still poorly understood. An important next step is to experimentally determine how urban environments induce altered phenotypes. Are urban phenotypes the result of natural selection, do they arise developmentally, or can they be induced through phenotypic flexibility in adult individuals? All these processes are non-exclusive and could act together to produce urban phenotypes. We asked whether the latter alternative, phenotypic flexibility in adults, can lead to urban-like phenotypes. To test this, we administered a chronic disturbance treatment during the springtime period of gonadal recrudescence and migration in a migratory sparrow, the dark-eyed junco (Junco hyemalis).
Free-living urban juncos at two locations in southern California have lower acute corticosterone response than neighboring non-urban populations (Atwell et al., 2012, Hanauer et al. in prep, Abolins-Abols et al., 2016), and juncos captured as juveniles and raised in a common garden had lower acute corticosterone response if they were derived from an urban as opposed to a non-urban population (Atwell et al., 2012). Together these findings indicate that some populations of urban juncos exhibit a lower acute corticosterone response than non-urban juncos in the wild, and that the difference persists in a common garden.
Physiology in high-disturbance environments is of particular interest during the spring period of migration and gonadal recrudescence because this is an important time for determining whether birds breed successfully or not (Ramenofsky and Wingfield, 2006; Bauchinger et al., 2007), and colonization of urban habitats often begins with wintering in urban areas and later transitions to breeding in urban habitats (Yeh and Price, 2004; Evans et al., 2010).
If phenotypic flexibility in adults contributes to an urban phenotype, we predicted that juncos exposed to chronic disturbance would attenuate their acute corticosterone response compared to birds in a low-disturbance environment. If attenuation of the acute corticosterone response is advantageous in a high-disturbance environment, we also predicted that within a group of chronically disturbed individuals, birds with a lower acute corticosterone response would maintain higher body condition. Alternatively, if the urban phenotype depends solely on developmental or genetic processes, then we would not expect an effect of disturbance on the acute corticosterone response in non-urban adults captured from the wild.
We captured wild adult male dark-eyed juncos in rural habitat near Bloomington, IN during the winter of 2013-2014. To our knowledge, juncos that winter in Indiana migrate north in spring to breed in non-urban habitats. After capture they were housed indoors, free-flying, in small flocks. We artificially advanced photoperiod so that our treatment would occur during the early springtime period of gonadal recrudescence and migration (Rowan, 1938; Holberton et al., 2008). We matched light cycles to Indiana photoperiod until January 15, 2014, and then gradually increased photoperiod from 10:44-hour days on Jan. 15 to 16:03-hour days on Feb. 22 (light schedule changed three times per week). Day length on February 3, the date disturbance treatment began, was 13:23. Birds were moved to individual cages on January 19. Cages (2’x2’x2’) were arranged 9 per room (9’x7’) in stacks of two or three cages. Birds could see and hear other birds in the room. We provided food (including millet, sunflower seeds, dry dog food, fresh carrots, whole hard-boiled eggs, and mealworms) and fresh water ad lib and replaced them three times per week.
We randomly assigned birds to one of two groups: control (n=18) or chronic disturbance (n=18). The 18 birds from each group were divided between two adjacent rooms. The disturbance treatment was similar to Rich and Romero (2005): birds were subjected to 4 disturbances each day, and each disturbance was 30 min in duration. At least 60 min elapsed between the end of one disturbance and the beginning of the next disturbance. Disturbances began at least 2 hrs after lights-on to allow adequate time for morning feeding. Within these constraints, the timing of disturbances varied day to day. We administered three types of disturbances: hand-waving, cage-tapping, and predator mount. The sequence of disturbances varied each day. For both hand-waving and cage-tapping, a researcher was present in the room during the entire 30 min. The researcher targeted one focal bird for 10-30 sec at a time before moving to the next bird, with the goal of keeping the focal bird moving (hopping or flying) during that time. Birds were targeted in random order within a room to reduce predictability. During hand-waving, the researcher placed one hand inside the cage, waved their hand, and chased the bird. During cage-tapping, the researcher tapped on the outside of the bird’s cage. Each room experienced hand-waving or cage-tapping at least once each day (average: 2.5 times/day). Predator mount consisted of a taxidermic predator being placed in the room for 30 min. Predators were owls, hawks, or squirrels. One or two predators were placed in the room at a time, and the identity and location of the predators were varied to mitigate habituation. We had previously validated in a separate cohort of juncos that exposure to 30 minutes of these disturbances caused acutely increased corticosterone (Figure S1). Disturbances began February 3 and continued until February 25. Capture, handling, restraint, and bleeding were specifically not used as disturbances, to ensure that plasma corticosterone values reflected changes in overall stress-response rather than habituation to the specific sampling techniques. Controls were not disturbed except for standard animal care procedures and the times when birds from both treatments were sampled; control rooms were separated from disturbance rooms so that disturbance treatment did not affect controls.
We collected blood samples just prior to the start of the experiment (round 1; February 1-2), after one week of treatment (round 2; February 8-9), and after three weeks of treatment (round 3; February 23-24). On sampling days, we did not enter bird rooms prior to taking the baseline sample. We captured birds for blood sampling between 7:50 and 13:30. Multiple researchers sampled birds simultaneously, allowing half of the birds in each room to be sampled within 3 min of entering the room; the other half were sampled the next day using the same procedures. Peripheral blood was collected from the brachial vein in heparinized microcapillary tubes. We took two blood samples during each round, one within 3 min of capture (baseline), and one 30 min after capture (restraint-induced). Birds were restrained in bags in between the baseline and restraint-induced samples. We calculated the rise in corticosterone in response to acute stress by subtracting baseline hormone concentrations from restraint-induced concentrations.
Fifteen days prior to the onset of the experiment (Jan. 19), we plucked six rectrices (R2, R4, R6, left and right) to stimulate feather growth during the experiment; after the treatment ended (Feb. 25), we plucked the newly-grown feathers. One of these rectrices (R2) was measured by calipers and weighed, then analyzed for feather corticosterone (see below). The other five rectrices were scored for fault bars by one researcher (BJG), who was blind to treatment. Bars were classified as light (visible discontinuity due to missing or abnormal barbules), medium (translucent line <1mm wide), or strong (translucent line >1mm wide) following Sarasola and Jovani (2006). By comparing feathers grown in the wild to feathers grown in captivity, we were able to address the impacts of both captivity and disturbance.
On each sampling round, we recorded each bird’s mass (Pesola), visually scored pectoral muscle condition following Gosler (1991), and visually scored fat stores in the furculum and abdomen using a system modified from Helms and Drury (1960). We classified fat separately for the furculum and abdomen: 0, no fat visible; 1, fat visible but region is concave; 2, fat fills the space so it is neither concave nor convex; 3, fat deposits are large and convex. The scores from each region (furculum and abdomen) were added together, for a total fat score ranging from 0 to 6.
We quantified corticosterone using EIA (Cayman Chemical Company, cat. No. 500655, Ann Arbor, MI, USA). To measure circulating corticosterone, we diluted 10 ul peripheral blood plasma in water, extracted the non-polar hormones with diethyl ether, and separated ether from water via snap-freezing. To measure corticosterone deposited in feathers, we extracted corticosterone from one rectrix (R2) per bird by cutting the feather (vanes and rachis, excluding calamus) into small pieces (<4mm2) and incubating the cut feather in methanol overnight in a 50°C water bath, beginning with 1 hour of sonication (Bortolotti et al., 2008). After incubation, we centrifuged samples at 3000 rpm for 20 min, collected the supernatant, and then rinsed the remaining pellet of feathers by vortexing with 1 ml fresh methanol, centrifuging, and collecting the rinsed supernatant. This rinse reduced variation in extraction efficiency. Supernatant was dried in a 40 C water bath. Feather hormone samples were then reconstituted and assayed using EIA in the same way as plasma samples. The feather assay showed good parallelism of serial dilutions to the standard curve. Extraction efficiency was measured by recovery of tritium-labeled corticosterone and on average measured >100% for plasma and feather assays; however, efficiency was highly consistent (plasma CV=3.08%, feather CV=2.96%), and extraction efficiency was not used to adjust final corticosterone values. Samples were run in duplicate. A plasma pool standard was run in triplicate on all plates to determine intra- and inter-plate variation. Inter-plate CV was 9.2%, and intra-plate CV was 4.5%. Hormone levels were determined in reference to eight-point standard curve using a curve-fitting program (Microplate Manager, Bio-Rad Laboratories Inc., Hercules, CA). The average of the standard across all plates was used to determine correction factors that were used to scale the results from each plate, mitigating inter-plate variation.
We used JMP 12.0 (SAS) for all analyses. Sample size varies across analyses due to sample loss. We ln-transformed baseline and restraint-induced corticosterone values to meet assumptions of normality. Time of day was negatively correlated with all measures of plasma corticosterone (Figure S2; linear mixed models; baseline: n=91 observations from 35 birds, F=11.99, p<0.001; restraint-induced: n=91 observations from 35 birds, F=8.19, p<0.01; rise: n=81 observations from 35 birds, F=6.82, p=0.01), so we included time of day in our models. Each treatment was divided between two rooms; we tested whether room improved each model (AICc), and if it did not, we omitted room from that model. Each sampling round was divided between two sampling days; sampling day did not improve our models (AICc) and was omitted. To test whether the disturbance treatment affected plasma corticosterone levels, we used a repeated-measures mixed effects model with time of day, treatment, round, and treatment*round as explanatory variables, and individual identity as a random factor (nested within treatment). We used this model to test effects of treatment on both baseline corticosterone and restraint-induced corticosterone, except that the treatment*round interaction did not improve the model (AICc) for baseline corticosterone and was omitted. We used post-hoc one-way ANOVA to confirm the time points when treatments differed. Feather fault bars and feather corticosterone were non-normally distributed, so we used nonparametric tests for one-way analyses of these variables (Wilcoxon test). We tested whether the total number of fault bars differed and also whether the severity of fault bars differed, by calculating the proportion of fault bars that were medium or strong. To account for differences in structural size, we regressed mass on tarsus length, and used the residual mass from this regression for subsequent analyses of body condition. For all parametric tests, we verified normal distribution of residuals.
Baseline corticosterone did not differ between treatment groups, and did not change over the course of the experiment (Figures 1A and S3; repeated-measures mixed effects model, n=91 observations from 35 birds, treatment: F=0.01, p=0.93; round: F=0.47, p=0.63; time of day: F=5.12, p=0.03).
Figure 1 Baseline (A) and restraint-induced (B) plasma corticosterone levels, depicted as residuals after controlling for time of day of sampling. Colors represent treatment groups, sampled across three rounds: before treatment (round 1), in the middle of treatment (2), and post-treatment (3). Box plots show median values (solid horizontal lines), 50th percentile values (box outline), observations within 1.5IQ range (whiskers) and outliers (points).
For restraint-induced corticosterone, the interaction between treatment and round was significant, indicating that the treatments differed in how restraint-induced corticosterone changed over the course of the experiment (Figures 1B, S4; repeated-measures mixed effects model, n=91 observations from 35 birds; treatment*round: F=3.1, p=0.04; treatment: F=1.84, p=0.18; round: F=0.10, p=0.91; time of day: F=5.43, p=0.02). Post-hoc ANOVA found no difference between treatments at round 1 (n=29, r2 = 0.34, treatment: F=2.50, p=0.13; time of day: F=13.32, p=0.001) or round 2 (n=34, r2 = 0.02, treatment: F=0.05, p=0.83; time of day: F=0.51, p=0.48), but at round 3 the disturbance group had significantly lower restraint-induced corticosterone than the control group (n=28, r2 = 0.25, treatment: F=5.14, p=0.03; time of day: F=2.70, p=0.11).
Rise in corticosterone showed similar patterns as restraint-induced corticosterone (Figure S5, repeated-measures mixed effects model: n=81 observations of 35 birds; treatment*round: F=6.95, p=0.002; treatment: F=4.30, p=0.046; round: F=1.23, p=0.30; time of day: F=6.01, p=0.02). Sample size is larger for restraint-induced corticosterone than rise in corticosterone, and so we focus our interpretations of acute corticosterone response on restraint-induced corticosterone.
Feathers grown during the disturbance treatment did not differ in corticosterone concentrations from feathers grown during the control treatment (Figure 2; Wilcoxon test, n=34, X2 = 0.27, p=0.60). Feather corticosterone was lower in feathers grown during the experiment than in feathers grown in the wild (Figure 2, Wilcoxon test, n=68, X2 = 27.05, p<0.01). The decrease in feather corticosterone from feathers grown in the wild to feathers grown in captivity for each individual did not depend on treatment (Wilcoxon test, n=34, X2 = 0.53, p=0.47), and there was no correlation between an individual’s feather corticosterone in the wild and feather corticosterone in captivity (linear regression, n=34, F<0.01, p=0.99).
Figure 2 Feather corticosterone is shown for feathers grown before capture (wild-grown) and feathers grown during treatment (captive-grown). Box plots show median values (solid horizontal lines), 50th percentile values (box outline), observations within 1.5IQ range (whiskers) and outliers (points).
Both groups increased body condition (residual mass) over the course of the experiment, but control birds increased significantly more (Figure 3, repeated-measures mixed effects model; n=36 birds, 107 observations; round: F=63.38, p<0.01; treatment: F=8.97, p<0.01; treatment*round: F=4.00, p=0.02; room[treat]: F=2.37, p=0.10). Both groups of birds gained fat over the course of the experiment, but control birds gained significantly more (ordinal logistic regression with repeated measures; n=36 birds, 108 observations; round: X2 = 91.53, p<0.01; treatment: X2 = 6.92, p<0.01). Pectoral muscle condition did not differ significantly between groups, but there was a trend toward an interaction between round and treatment group (ordinal logistic regression with repeated measures; n=36 birds, 108 observations; treatment: X2 = 0.10, p=0.75; round: X2 = 2.94, p=0.23; treatment*round: X2 = 5.58, p=0.06); that trend was due to the control group showing a small increase in pectoral condition by the end of the experiment (ordinal logistic regression with repeated measures; n=18 birds, 54 observations; round: X2 = 7.38, p=0.03), while the disturbance group showed no change (ordinal logistic regression with repeated measures; n=18 birds, 54 observations; round: X2 = 0.61, p=0.74).
Figure 3 Body condition, defined as residual mass (g) after accounting for tarsus length, increased over the course of the experiment in both groups. The disturbance group (dark red) had lower body condition at the end of the experiment than controls (light blue). Box plots show median values (solid horizontal lines), 50th percentile values (box outline), observations within 1.5IQ range (whiskers) and outliers (points).
Restraint-induced corticosterone did not show the predicted negative correlation with condition in the disturbance group (Figure 4; linear regression of residual mass by residual restraint-induced corticosterone (corrected for time of day); control: n=15, r2 = 0.04, β=0.53, t=0.70, p=0.50; disturb: n=13, r2 = 0.04, β=0.50, t=0.68, p=0.51). Rise in corticosterone showed a positive correlation with condition in the disturbance group (linear regression of residual mass by residual rise in corticosterone (corrected for time of day); control: n=14, r2 = 0.03, F=0.38, p=0.55; disturb: n=9, r2 = 0.48, F=6.39, p=0.04).
Figure 4 Restraint-induced corticosterone (corrected for time of day) at sampling round 3 was not negatively correlated with body condition in either treatment (A: control; B: disturb); trends were weak, nonsignificant, and positive.
Disturbance treatment did not affect number or severity of fault bars grown in captivity, but captive-grown feathers had more fault bars than wild-grown feathers, regardless of treatment. Feathers grown during the disturbance treatment did not differ from feathers grown during the control treatment in number of fault bars (Figure 5, Wilcoxon test, n=34, X2 = 2.20, p=0.14), proportion of fault bars classified “medium” (Wilcoxon test, n=34, X2 = 1.55, p=0.21) or proportion of fault bars classified “strong” (Wilcoxon test, n=34, X2 = 0.20, p=0.65). Despite ad lib food, feathers grown in captivity had significantly more fault bars than feathers grown in the wild (Figure 5, Wilcoxon test, n=70, X2 = 15.92, p<0.01). An individual bird’s change in fault bars from wild to captivity did not depend on treatment (Wilcoxon test, n=34, X2 = 0.25, p=0.62). Within the disturbance group, an individual’s fault bars grown in captivity trended negatively with fault bars grown in the wild (Figure S6, linear regression, n=17, r2 = 0.21, β=-0.67, t=-2.02, p=0.06); there was no relationship in the control group (linear regression, n=17, r2<0.01, β=0.09, t=0.16, p=0.88). In the disturbance group, body condition at the end of the experiment was negatively correlated with the number of fault bars in the feathers grown during treatment (Figure 6, linear regression, n=17, r2 = 0.31, β=-0.50, t=-2.58, p=0.02); there was no relationship between body condition and fault bars in the control group (linear regression, n=17, r2<0.01, β=-0.06, t=-0.21, p=0.83).
Figure 5 Birds grew more feather fault bars in captivity than in the wild, but fault bars not differ between treatment groups (control in light blue, disturbance in dark red). Box plots show median values (solid horizontal lines), 50th percentile values (box outline), observations within 1.5IQ range (whiskers) and outliers (points).
Figure 6 Captive-grown feather faults bars from disturbance group compared to body condition (residual mass, g).
Feathers that grew during the disturbance treatment were significantly shorter than feathers in the control treatment (Figure 7, linear regression: n=35, r2 = 0.47, treat: β=1.01, t=4.07, p<0.01; feather length at capture: β=0.52, t=3.96, p<0.01). Treatment did not affect mass of feathers that grew in captivity (ANOVA: n=35, r2 = 0.03, β=0.14, t=0.94, p=0.36).
We manipulated disturbance frequency of adult juncos to assess phenotypic flexibility in the physiological stress response upon exposure to a high-disturbance environment. We found that chronic disturbance induced an attenuation of acute corticosterone response as compared to the control group. We predicted that such an attenuation would be advantageous in a high-disturbance environment and lead to higher body condition, but this prediction was not supported. Baseline corticosterone was not affected by treatment, and body condition was significantly lower in the disturbance group. Surprisingly, feather corticosterone and feather fault bars did not differ between treatments, despite the different levels of disturbance experienced during feather growth. Juncos are known to have the capacity to adapt to breeding in urban environments in parts of their range, and this study suggests that phenotypic flexibility could potentially account for altered phenotypes early in the urban colonization process.
We found no effect of treatment on baseline corticosterone. In the literature, reports of effects of chronic disturbance on baseline corticosterone are mixed (Dickens and Romero, 2013; Iglesias-Carrasco et al., 2020; Bonier, 2023; Deviche et al., 2023), so this result is not surprising. Juncos have shown seasonal changes in baseline corticosterone in some previous studies conducted in the spring migratory period (Holberton et al., 2008) but not others (Fudickar et al., 2016); we did not observe seasonal change in baseline corticosterone levels in our study.
Acute corticosterone response was attenuated in the disturbance group compared to the control group after three weeks of treatment. This attenuation in the disturbance group is in accordance with previous studies of chronic disturbance in birds (e.g., Rich and Romero, 2005), and it also matches our predictions for the phenotypic flexibility hypothesis for the generation of urban phenotypes. Not all urban-adapting species show this pattern; repeated ten-day chronic disturbance treatments did not affect corticosterone in European blackbirds (Hau et al., 2015), although this lack of effect could be due to the duration of the experiment rather than intrinsic differences in stress physiology between juncos and blackbirds, since in our study we did not see differences after seven days of chronic disturbance.
The mechanism for this attenuation of acute corticosterone is not known in this study. It is possible that juncos habituated to stressors while retaining full capacity to produce corticosterone; this could be investigated in the future via ACTH challenges (Gormally and Romero, 2018). Alternatively, changes to the HPA axis could have taken place to reduce the amount of corticosterone the juncos were able to produce. It is also unknown why in our study acute corticosterone was attenuated after three weeks of disturbance but not after one week.
Urban juncos in southern California are known to demonstrate a lower acute corticosterone response than their non-urban neighbors. The present study adds an exciting layer: a similar pattern can be induced in non-urban birds exposed to high levels of disturbance as adults. Thus our experiment simulates a colonization scenario in which adults raised in non-urban habitats come into reproductive condition in a novel, high-disturbance environment, which is hypothesized to be the process by which juncos in southern California colonized urban habitats (Yeh and Price, 2004). One caveat is that our frequent disturbance protocol may be an extreme stressor compared to predictable urban disturbances such as light pollution or infrequent disturbances such as predation attempts. Our results suggest that phenotypic flexibility in adults could contribute to attenuated acute corticosterone responses in free-living urban birds at the onset of colonization of a novel urban habitat. Many studies of avian stress physiology in response to disturbance treatments use domesticated species or synanthropic species, which thrive in urban environments (Beaugeard et al., 2019); in contrast, juncos avoid breeding in urban habitats across much of their range, but have shown an ability to colonize urban habitats in some places. This makes the junco a useful system for understanding the process by which native wildlife may adapt to human disturbance and colonize urban environments.
Feathers contain corticosterone deposited from the blood during feather growth, and feather corticosterone has attracted interest as a less-invasive, longer-term measure of HPA activity compared to blood sampling (Romero and Fairhurst, 2016). A common interpretation of feather corticosterone is that it reflects the stressors an animal experiences during feather growth, integrated with other factors such as the magnitude of the individual’s HPA response to stressors (Bortolotti et al., 2008; Fairhurst et al., 2011; Beaugeard et al., 2019; Brodin and Watson, 2023). In this study, however, feather corticosterone was not a useful indicator of the frequency of stressors experienced by the birds. Captivity itself had unexpected effects on feather corticosterone: feather corticosterone was lower in feathers grown in captivity during early spring as compared to those grown in the wild during fall molt, despite plasma corticosterone levels frequently being higher in early spring than during fall molt (Romero, 2002). Captive-grown feather corticosterone was not correlated with wild-grown feather corticosterone, in contrast to work in other species that did find such a correlation (Aharon-Rotman et al., 2017). We thus urge caution in future interpretations of feather corticosterone.
Birds in both groups gained fat and body condition over time, likely because they were preparing for migration. Previous studies of captive juncos in spring have shown an increase in food intake, mass, and fat (Holberton et al., 2008). Against this background of seasonal increase in fat and mass over time, we observed that control birds gained more fat and body condition than birds exposed to chronic disturbance, suggesting that some aspect of chronic disturbance interfered with the seasonal advance in migratory condition. Lower mass is the most consistent physiological sign of chronic stress in wild animals (Dickens and Romero, 2013), so the difference we observed in body condition could indicate that our disturbance treatment caused chronic stress; however, the lack of difference in baseline corticosterone makes this interpretation less likely. Alternatively, the exercise of flying and hopping during disturbances may have interfered with mass gain in the disturbance group. A companion study of these same birds found that frequently-disturbed birds were equally reproductively ready as control birds: groups did not differ in testes mass or testosterone in response to GnRH, and cloacal protuberance size was larger in the disturbance group, although baseline testosterone was lower in the disturbance group (Abolins-Abols et al., 2018). These results indicate that birds in the chronic disturbance treatment were likely not in homeostatic overload (Romero et al., 2009), and were instead able to achieve reproductive readiness despite disturbances, suggesting that the phenotypic flexibility we observed was adaptive (Ghalambor et al., 2007).
Disturbed birds grew shorter tail feathers, which could cause a decrease in flying efficiency. Fault bar severity and number did not differ between treatments, and therefore did not reveal any cost of disturbance to feather structure. Fault bars are a long-used method for evaluating nutritional or psychological stress during feather growth (Riddle, 1908; Jovani and Rohwer, 2017), but our results do not provide evidence that fault bars can be used to detect variation in frequency of disturbance. Previous work in starlings also found no effect of chronic psychological stress on fault bars (Strochlic and Romero, 2008). Captivity may be inherently stressful to juncos, and the increased number of fault bars in captivity could reflect this. Consistent with the hypothesis that experimental conditions increase fault bars, white-crowned sparrows formed fewer fault bars in the wild or in large aviaries than they did during experiments that involved handling to collect samples (King and Murphy, 1984). Within individuals, wild-grown and captive-grown fault bars were not positively correlated, and the disturbance group showed a counterintuitive negative correlation between wild-grown and captive-grown fault bars; this contrasts with previous work that found fault bars to be repeatable across years (Bortolotti et al., 2002). In the disturbance group, birds that had higher body condition had fewer captive-grown fault bars; this likely indicates that some birds tolerated the disturbance treatment better than others, and invested in both weight gain and high-quality feathers. Low body condition is sometimes correlated with increased fault barring (Bortolotti et al., 2002; Jovani and Rohwer, 2017).
Contrary to our predictions, an attenuated acute corticosterone response was not associated with improved resilience to the chronic disturbance environment. Instead of the predicted negative relationship between restraint-induced corticosterone and body condition, we saw no significant relationship between these variables; our data therefore do not indicate whether attenuation in the acute corticosterone response is advantageous in terms of maintaining good body condition.
Overall, we saw considerable individual variation in all measures, which may indicate individual variation in response to stressors in the environment: some birds in the control group may have experienced captivity and periodic handling and bleeding as a significant stressor, while some birds in the disturbance group may have experienced chronic disturbance as only a moderate stressor.
As noted there are multiple hypotheses that could explain these phenotypic changes: 1) selection for individuals expressing aspects of the urban phenotype, 2) developmental plasticity of young developing in an urban habitat, 3) epigenetic and maternal effects, or 4) phenotypic flexibility in adult animals (Piersma and Drent, 2003; Partecke et al., 2013; Bonier, 2023). These hypotheses are non-exclusive and may all help to explain phenotypic change in urban habitats. Understanding how these processes lead to urban phenotypes is important for predicting the success and timescale of colonization of urban habitats.
We have shown that a high-disturbance environment can induce attenuation of the acute corticosterone response in adult male juncos, supporting the hypothesis that phenotypic flexibility in adults could contribute to acclimation to urban areas. We hypothesize that behavioral and phenotypic flexibility in adults can lead to faster colonization of urban habitats as compared to development or selection, and that species that have higher flexibility are more likely to successfully colonize urban habitats (Mason et al., 2013). Future work exploring this possibility could enable predictions of which species are most likely to adapt to urban habitats, and which species will require conservation of appropriate wildlands (Winchell et al., 2022).
Although colonization events may not happen frequently, they are critical to maintenance of biodiversity as non-urban habitats are rapidly replaced by urban habitats across the globe (Seto et al., 2011; Isaksson, 2018). Response to chronic disturbance may be especially critical in the springtime period when gonads develop, territories are established, and mates are chosen, because stressed animals may delay or forgo reproduction. Tolerance of human disturbance and successful colonization of new habitats may be species-level traits, dependent on life history, behavior, and hormones (Møller et al., 2015). Here we present evidence that non-urban adult dark-eyed juncos are flexible in their corticosterone response to capture and handling, depending on frequency of disturbance in their environment. This may contribute to successful colonization of urban habitats by juncos.
The raw data supporting the conclusions of this article will be made available by the authors, without undue reservation.
The animal study was reviewed and approved by Bloomington Institutional Animal Care and Use Committee. The study was conducted in accordance with the local legislation and institutional requirements.
Data analysis and manuscript drafts prepared by RH, bird capture, animal care, disturbance treatments, blood sampling, and hormone analyses were done by all except senior author, final draft prepared by lead author and senior author. All authors contributed to the article and approved the submitted version.
The material is based on work supported by the National Science Foundation under Grant Nos. DEB-1406496 and IOS-1257527, and by the National Institutes of Health under training grant 2T32HD49336.
Assistance with bird capture, animal care, disturbance treatments, blood sampling, and hormone analyses was provided by A. Kimmitt, S. Slowinski, A. Fudickar, K. Rosvall, A. McKay, H. Kassab, N. Fletcher, C. Taylor, and S. Calzonzi. R. Stewart, Center for Integrative Study of Animal Behavior (CISAB), and S. Wanamaker helped to develop the feather corticosterone assay.
The authors declare that the research was conducted in the absence of any commercial or financial relationships that could be construed as a potential conflict of interest.
The author EK declared that they were an editorial board member of Frontiers, at the time of submission. This had no impact on the peer review process and the final decision.
All claims expressed in this article are solely those of the authors and do not necessarily represent those of their affiliated organizations, or those of the publisher, the editors and the reviewers. Any product that may be evaluated in this article, or claim that may be made by its manufacturer, is not guaranteed or endorsed by the publisher.
The Supplementary Material for this article can be found online at: https://www.frontiersin.org/articles/10.3389/fbirs.2023.1245853/full#supplementary-material
Abolins-Abols M., Hanauer R. E., Rosvall K. A., Peterson M. P., Ketterson E. D. (2018). The effect of chronic and acute stressors, and their interaction, on testes function: an experimental test during testicular recrudescence. J. Exp. Biol. 221 (17). doi: 10.1242/jeb.180869
Abolins-Abols M., Hope S. F., Ketterson E. D. (2016). Effect of acute stressor on reproductive behavior differs between urban and rural birds. Ecol. Evol. 6 (18), 6546–6555. doi: 10.1002/ece3.2347
Aharon-Rotman Y., Buchanan K. L., Klaassen M., Buttemer W. A. (2017). An experimental examination of interindividual variation in feather corticosterone content in the house sparrow, Passer domesticus in southeast Australia. Gen. Comp. Endocrinol. 244, 93–100. doi: 10.1016/j.ygcen.2015.12.010
Atwell J. W., Cardoso G. C., Whittaker D. J., Campbell-Nelson S., Robertson K. W., Ketterson E. D. (2012). Boldness behavior and stress physiology in a novel urban environment suggest rapid correlated evolutionary adaptation. Behav. Ecol. 23 (5), 960–969. doi: 10.1093/beheco/ars059
Bauchinger U., Van’t Hof T., Biebach H. (2007). Testicular development during long-distance spring migration. Hormones Behav. 51 (3), 295–305. doi: 10.1016/j.yhbeh.2006.10.010
Beaugeard E., Brischoux F., Henry P.-Y., Parenteau C., Trouvé C., Angelier F. (2019). Does urbanization cause stress in wild birds during development? Insights from feather corticosterone levels in juvenile house sparrows (Passer domesticus). Ecol. Evol. 9 (1), 640–652. doi: 10.1002/ece3.4788
Beckerman A. P., Boots M., Gaston K. J. (2007). Urban bird declines and the fear of cats. Anim. Conserv. 10 (3), 320–325. doi: 10.1111/j.1469-1795.2007.00115.x
Blumstein D. T. (2014). “Attention, habituation, and antipredator behaviour: implications for urban birds,” in Avian urban ecology. (Oxford, UK: Oxford University Press), 41–53.
Bonier F. (2012). Hormones in the city: Endocrine ecology of urban birds. Hormones Behav. 61 (5), 763–772. doi: 10.1016/j.yhbeh.2012.03.016
Bonier F. (2023). Future directions in urban endocrinology – The effects of endocrine plasticity on urban tolerance. Mol. Cell. Endocrinol. 565, 111886. doi: 10.1016/j.mce.2023.111886
Bortolotti G. R., Dawson R. D., Murza G. L. (2002). Stress during feather development predicts fitness potential. J. Anim. Ecol. 71 (2), 333–342. doi: 10.1046/j.1365-2656.2002.00602.x
Bortolotti G. R., Marchant T. A., Blas J., German T. (2008). Corticosterone in feathers is a long-term, integrated measure of avian stress physiology. Funct. Ecol. 22 (3), 494–500. doi: 10.1111/j.1365-2435.2008.01387.x
Brodin A., Watson H. (2023). Feather corticosterone reveals that urban great tits experience lower corticosterone exposure than forest individuals during dominance-rank establishment. Conserv. Physiol. 11 (1). doi: 10.1093/conphys/coad033
Busch D. S., Sperry T. S., Peterson E., Do C.-T., Wingfield J. C., Boyd E. H. (2008). Impacts of frequent, acute pulses of corticosterone on condition and behavior of Gambel’s white-crowned sparrow (Zonotrichia leucophrys gambelii). Gen. Comp. Endocrinol. 158 (3), 224–233. doi: 10.1016/j.ygcen.2008.07.010
Caizergues A. E., Grégoire A., Choquet R., Perret S., Charmantier A. (2022). Are behaviour and stress-related phenotypes in urban birds adaptive? J. Anim. Ecol. 91 (8), 1627–1641. doi: 10.1111/1365-2656.13740
Clinchy M., Zanette L., Charlier T. D., Newman A. E. M., Schmidt K. L., Boonstra R., et al. (2011). Multiple measures elucidate glucocorticoid responses to environmental variation in predation threat. Oecologia 166 (3), 607–614. doi: 10.1007/s00442-011-1915-2
Davies S., Haddad N., Ouyang J. Q. (2017). Stressful city sounds: glucocorticoid responses to experimental traffic noise are environmentally dependent. Biol. Lett. 13 (10), 20170276. doi: 10.1098/rsbl.2017.0276
Deviche P., Sweazea K., Angelier F. (2023). Past and future: Urbanization and the avian endocrine system. Gen. Comp. Endocrinol. 332, 114159. doi: 10.1016/j.ygcen.2022.114159
Dickens M. J., Romero L. M. (2013). A consensus endocrine profile for chronically stressed wild animals does not exist. Gen. Comp. Endocrinol. 191, 177–189. doi: 10.1016/j.ygcen.2013.06.014
Evans K. L. (2010). “Individual species and urbanisation,” in Urban ecology (Cambridge: Cambridge University Press), 53–87.
Evans K. L., Hatchwell B. J., Parnell M., Gaston K. J. (2010). A conceptual framework for the colonisation of urban areas: the blackbird Turdus merula as a case study. Biol. Rev. 85, 643–667. doi: 10.1111/j.1469-185X.2010.00121.x
Fairhurst G. D., Frey M. D., Reichert J. F., Szelest I., Kelly D. M., Bortolotti G. R. (2011). Does environmental enrichment reduce stress? An integrated measure of corticosterone from feathers provides a novel perspective. PloS One 6 (3), e17663. doi: 10.1371/journal.pone.0017663
Finn C., Grattarola F., Pincheira-Donoso D. (2023). More losers than winners: investigating Anthropocene defaunation through the diversity of population trends. Biol. Rev. 98 (5), 1732–1748. doi: 10.1111/brv.12974
Fischer E. K., Harris R. M., Hofmann H. A., Hoke K. L. (2014). Predator exposure alters stress physiology in guppies across timescales. Hormones Behav. 65 (2), 165–172. doi: 10.1016/j.yhbeh.2013.12.010
Fokidis H. B., Orchinik M., Deviche P. (2009). Corticosterone and corticosteroid binding globulin in birds: Relation to urbanization in a desert city. Gen. Comp. Endocrinol. 160 (3), 259–270. doi: 10.1016/j.ygcen.2008.12.005
Frid A., Dill L. M. (2002). Human-caused disturbance stimuli as a form of predation risk. Conserv. Ecol. 6 (1), 11. doi: 10.5751/ES-00404-060111
Fudickar A. M., Greives T. J., Atwell J. W., Stricker C. A., Ketterson E. D. (2016). Reproductive allochrony in seasonally sympatric populations maintained by differential response to photoperiod: Implications for population divergence and response to climate change. Am. Nat. 187 (4), 436–446. doi: 10.1086/685296
Garitano-Zavala Á., Calbimonte R., Esteve-Herraiz G. (2022). The behavioral responses of the chiguanco thrush to urbanization in a neotropical city comes from preadapted behavioral traits. Front. Ecol. Evol. 10. doi: 10.3389/fevo.2022.830902
Ghalambor C. K., McKAY J. K., Carroll S. P., Reznick D. N. (2007). Adaptive versus non-adaptive phenotypic plasticity and the potential for contemporary adaptation in new environments. Funct. Ecol. 21 (3), 394–407. doi: 10.1111/j.1365-2435.2007.01283.x
Gormally B. M. G., Romero L. M. (2018). House sparrows (Passer domesticus) adjusted hypothalamic-pituitary-adrenal axis negative feedback and perch hopping activities in response to a single repeated stimulus. J. Exp. Zoology Part A: Ecol. Integr. Physiol. 329 (10), 597–605. doi: 10.1002/jez.2229
Gosler A. (1991). On the use of greater covert moult and pectoral muscle as measures of condition in passerines with data for the Great Tit Parus major. Bird Study 38 (1), 1–9. doi: 10.1080/00063659109477061
Grade A. M., Lerman S. B., Warren P. S. (2021). Perilous choices: landscapes of fear for adult birds reduces nestling condition across an urban gradient. Ecosphere 12 (7), e03665. doi: 10.1002/ecs2.3665
Haskell D. G., Knupp A., Schneider M. (2001). “Nest predator abundance and urbanization,” in Avian ecology and conservation in an urbanizing world (New York, NY: Springer), 243–258.
Hau M., Casagrande S., Ouyang J. Q., Baugh A. T. (2016). Glucocorticoid-mediated phenotypes in vertebrates: Multilevel variation and evolution. Adv. Study Behav. 48, 41–115. doi: 10.1016/bs.asb.2016.01.002
Hau M., Haussmann M. F., Greives T. J., Matlack C., Costantini D., Quetting M., et al. (2015). Repeated stressors in adulthood increase the rate of biological ageing. Front. Zoology 12, 4. doi: 10.1186/s12983-015-0095-z
Hawlena D., Schmitz O. J. (2010). Physiological stress as a fundamental mechanism linking predation to ecosystem functioning. Am. Nat. 176 (5), 537–556. doi: 10.1086/656495
Helms C. W., Drury W. H. Jr. (1960). Winter and migratory weight and fat field studies on some North American buntings. Bird-Banding 31, 1–40.
Hendry A. P., Farrugia T. J., Kinnison M. T. (2008). Human influences on rates of phenotypic change in wild animal populations. Mol. Ecol. 17 (1), 20–29. doi: 10.1111/j.1365-294X.2007.03428.x
Holberton R. L., Boswell T., Hunter M. J. (2008). Circulating prolactin and corticosterone concentrations during the development of migratory condition in the Dark-eyed Junco, Junco hyemalis. Gen. Comp. Endocrinol. 155 (3), 641–649. doi: 10.1016/j.ygcen.2007.11.001
Iglesias-Carrasco M., Aich U., Jennions M. D., Head M. L. (2020). Stress in the city: meta-analysis indicates no overall evidence for stress in urban vertebrates. Proc. R. Soc. B: Biol. Sci. 287 (1936), 20201754. doi: 10.1098/rspb.2020.1754
Isaksson C. (2018). “Impact of urbanization on birds,” in Bird species: how they arise, modify and vanish. Ed. Tietze. D. T. (Cham: Springer International Publishing), 235–257.
Jones B. C., Smith A. D., Bebus S. E., Schoech S. J. (2016). Two seconds is all it takes: European starlings (Sturnus vulgaris) increase levels of circulating glucocorticoids after witnessing a brief raptor attack. Hormones Behav. 78 (Supplement C), 72–78. doi: 10.1016/j.yhbeh.2015.10.017
Jovani R., Rohwer S. (2017). Fault bars in bird feathers: mechanisms, and ecological and evolutionary causes and consequences. Biol. Rev. 92 (2), 1113–1127. doi: 10.1111/brv.12273
King J. R., Murphy M. E. (1984). Fault bars in the feathers of white-crowned sparrows - dietary deficiency or stress of captivity and handling. Auk 101 (1), 168–169. doi: 10.1093/auk/101.1.168
Lowry H., Lill A., Wong B. (2013). Behavioural responses of wildlife to urban environments. Biol. Rev. 88 (3), 537–549. doi: 10.1111/brv.12012
Mason G., Burn C. C., Dallaire J. A., Kroshko J., Kinkaid H. M., Jeschke J. M. (2013). Plastic animals in cages: behavioural flexibility and responses to captivity. Anim. Behav. 85 (5), 1113–1126. doi: 10.1016/j.anbehav.2013.02.002
Moller A. P. (2008). Flight distance of urban birds, predation, and selection for urban life. Behav. Ecol. Sociobiology 63 (1), 63–75. doi: 10.1007/s00265-008-0636-y
Møller A., Díaz M., Flensted-Jensen E., Grim T., Ibáñez-Álamo J., Jokimäki J., et al. (2015). Urbanized birds have superior establishment success in novel environments. Oecologia 178 (3), 943–950. doi: 10.1007/s00442-015-3268-8
Ouyang J. Q., Baldan D., Munguia C., Davies S. (2019). Genetic inheritance and environment determine endocrine plasticity to urban living. Proc. R. Soc. B: Biol. Sci. 286 (1908), 20191215. doi: 10.1098/rspb.2019.1215
Partecke J., Gil D., Brumm H. (2013). “Mechanisms of phenotypic responses following colonization of urban areas: from plastic to genetic adaptation,” in Avian urban ecology. (Oxford, UK: Oxford University Press), 131–142.
Patankar S., Jambhekar R., Suryawanshi K. R., Nagendra H. (2021). Which traits influence bird survival in the city? A review. Land 10 (2), 92. doi: 10.3390/land10020092
Piersma T., Drent J. (2003). Phenotypic flexibility and the evolution of organismal design. Trends Ecol. Evol. 18 (5), 228–233. doi: 10.1016/S0169-5347(03)00036-3
Ramenofsky M., Wingfield J. C. (2006). Behavioral and physiological conflicts in migrants: the transition between migration and breeding. J. Ornithology 147 (2), 135. doi: 10.1007/s10336-005-0050-4
Rebolo-Ifrán N., Carrete M., Sanz-Aguilar A., Rodríguez-Martínez S., Cabezas S., Marchant T. A., et al. (2015). Links between fear of humans, stress and survival support a non-random distribution of birds among urban and rural habitats. Sci. Rep. 5 (1), 13723. doi: 10.1038/srep13723
Rich E. L., Romero L. M. (2005). Exposure to chronic stress downregulates corticosterone responses to acute stressors. Am. J. Physiol. - Regul. Integr. Comp. Physiol. 288 (6), R1628–R1636. doi: 10.1152/ajpregu.00484.2004
Riddle O. (1908). The genesis of fault-bars in feathers and the cause of alternation of light and dark fundamental bars. Biol. Bull. 14 (6), 328–370. doi: 10.2307/1535869
Romero L. M., Dickens M. J., Cyr N. E. (2009). The reactive scope model — A new model integrating homeostasis, allostasis, and stress. Hormones Behav. 55 (3), 375–389. doi: 10.1016/j.yhbeh.2008.12.009
Romero L. M., Fairhurst G. D. (2016). Measuring corticosterone in feathers: Strengths, limitations, and suggestions for the future. Comp. Biochem. Physiol. Part A Mol. Integr. Physiol. 202, 112–122. doi: 10.1016/j.cbpa.2016.05.002
Romero L. M., Wingfield J. C. (2016). Tempests, poxes, predators, and people: Stress in wild animals and how they cope (New York, NY, US: Oxford University Press).
Romero M. L. (2002). Seasonal changes in plasma glucocorticoid concentrations in free-living vertebrates. Gen. Comp. Endocrinol. 128 (1), 1–24. doi: 10.1016/S0016-6480(02)00064-3
Rowan W. (1938). Light and seasonal reproduction in animals. Biol. Rev. 13 (4), 374–401. doi: 10.1111/j.1469-185X.1938.tb00523.x
Sapolsky R. M., Romero L. M., Munck A. U. (2000). How do glucocorticoids influence stress responses? Integrating permissive, suppressive, stimulatory, and preparative actions. Endocrine Rev. 21 (1), 55–89. doi: 10.1210/edrv.21.1.0389
Sarasola J. H., Jovani R. (2006). Risk of feather damage explains fault bar occurrence in a migrant hawk, the Swainson’s hawk Buteo swainsoni. J. Avian Biol. 37 (1), 29–35. doi: 10.1111/j.2006.0908-8857.03693.x
Scheuerlein A., Van’t Hof T., Gwinner E. (2001). Predators as stressors? Physiological and reproductive consequences of predation risk in tropical stonechats (Saxicola torquata axillaris). Proc. R. Soc. London B Biol. Sci. 268 (1476), 1575–1582. doi: 10.1098rspb.2001.1691
Seto K. C., Fragkias M., Güneralp B., Reilly M. K. (2011). A meta-analysis of global urban land expansion. PloS One 6 (8), e23777. doi: 10.1371/journal.pone.0023777
Sheriff M. J., Krebs C. J., Boonstra R. (2009). The sensitive hare: sublethal effects of predator stress on reproduction in snowshoe hares. J. Anim. Ecol. 78 (6), 1249–1258. doi: 10.1111/j.1365-2656.2009.01552.x
Sol D., Lapiedra O., Gonzalez-Lagos C. (2013). Behavioural adjustments for a life in the city. Anim. Behav. 85 (5), 1101–1112. doi: 10.1016/j.anbehav.2013.01.023
Strochlic D. E., Romero L. M. (2008). The effects of chronic psychological and physical stress on feather replacement in European starlings (Sturnus vulgaris). Comp. Biochem. Physiol. Part A Mol. Integr. Physiol. 149 (1), 68–79. doi: 10.1016/j.cbpa.2007.10.011
Winchell K. M., Aviles-Rodriguez K. J., Carlen E. J., Miles L. S., Charmantier A., De León L. F., et al. (2022). Moving past the challenges and misconceptions in urban adaptation research. Ecol. Evol. 12 (11), e9552. doi: 10.1002/ece3.9552
Wingfield J. C. (2013). The comparative biology of environmental stress: behavioural endocrinology and variation in ability to cope with novel, changing environments. Anim. Behav. 85 (5), 1127–1133. doi: 10.1016/j.anbehav.2013.02.018
Wingfield J., Krause J., Perez J., Chmura H., Németh Z., Word K., et al. (2015). A mechanistic approach to understanding range shifts in a changing world: What makes a pioneer? Gen. Comp. Endocrinol. 222, 44–53. doi: 10.1016/j.ygcen.2015.08.022
Wingfield J. C., Oreilly K. M., Astheimer L. B. (1995). Modultion of the adrenocortical responses to acute stress in arctic birds - a possible ecological basis. Am. Zoologist 35 (3), 285–294. doi: 10.1093/icb/35.3.285
Keywords: corticosterone, glucocorticoid, stress, urban, feather corticosterone, fault bar, recrudescence, phenotypic flexibility
Citation: Hanauer RE, Abolins-Abols M, Brenner AM, Gall BJ and Ketterson ED (2023) Chronic disturbance induces attenuation of the acute glucocorticoid response in an urban adapter, the dark-eyed junco. Front. Bird Sci. 2:1245853. doi: 10.3389/fbirs.2023.1245853
Received: 23 June 2023; Accepted: 25 September 2023;
Published: 26 October 2023.
Edited by:
David Koons, Colorado State University, United StatesReviewed by:
Michaela Hau, Max Planck Institute for Biological Intelligence, GermanyCopyright © 2023 Hanauer, Abolins-Abols, Brenner, Gall and Ketterson. This is an open-access article distributed under the terms of the Creative Commons Attribution License (CC BY). The use, distribution or reproduction in other forums is permitted, provided the original author(s) and the copyright owner(s) are credited and that the original publication in this journal is cited, in accordance with accepted academic practice. No use, distribution or reproduction is permitted which does not comply with these terms.
*Correspondence: Ellen D. Ketterson, a2V0dGVyc29AaW5kaWFuYS5lZHU=
Disclaimer: All claims expressed in this article are solely those of the authors and do not necessarily represent those of their affiliated organizations, or those of the publisher, the editors and the reviewers. Any product that may be evaluated in this article or claim that may be made by its manufacturer is not guaranteed or endorsed by the publisher.
Research integrity at Frontiers
Learn more about the work of our research integrity team to safeguard the quality of each article we publish.