- 1Departamento de Biodiversidade, Universidade Estadual Paulista, Rio Claro, Brazil
- 2Department of Biology, Indiana University, Bloomington, IN, United States
- 3Departamento de Ecología, Genética y Evolución, Instituto de Ecología, Genética y Evolución (CONICET-UBA), Facultad de Ciencias Exactas y Naturales, Universidad de Buenos Aires, Buenos Aires, Argentina
Migratory birds depend on resources located in distantly separated ecosystems, potentially making them vulnerable to rapid environmental changes across their range. Yet, we understand little about how such changes may impact birds in different migratory systems, in large part because of a lack of individual-level research throughout their annual cycle and on different continents. The Fork-tailed Flycatcher (Tyrannus savana) is a widely distributed Neotropical bird, whose nominate subspecies is composed of populations that breed at tropical and south-temperate latitudes of South America and then spend the non-breeding season in northern South America. Using movement data collected with light-level geolocators on individual migratory Fork-tailed Flycatchers from breeding populations at tropical and south-temperate latitudes, we evaluated whether different flycatcher populations vary in their vulnerability to future climate change. Results suggest that populations breeding at south-temperate and tropical latitudes will lose at least 22% of their breeding area, with most losses of south-temperate breeding areas occurring in the northern portions of those areas. Both south-temperate breeders and tropical breeders will also lose non-breeding area, with south-temperate breeders experiencing a loss of over a third of their non-breeding area, whereas tropical breeders will lose up to ~16% of that area. These results are the first to demonstrate population- and season-dependent risks to climate change for a migratory bird breeding in the Neotropics, and suggest that mitigating the impacts of climate change for birds in South America will require conservation planning that accounts for both seasonal and regional processes.
1 Introduction
Bird migration in the New World is composed of a variety of systems, including 1) Nearctic-Neotropical migration, in which birds breed in North America and overwinter in the Neotropics, 2) intra-tropical migration, in which birds migrate wholly within tropical latitudes, and 3) Neotropical austral migration, in which birds breed at south-temperate latitudes of South America and overwinter closer to the Equator (Chesser, 1994; Newton, 2010; Jahn et al., 2020). However, research on bird migration in South America has lagged behind that on Nearctic-Neotropical migration (Jahn et al., 2004; Faaborg et al., 2010). Results of recent research in South America have shown that bird migration there is more common than previously thought (reviewed by Jahn et al., 2020); nevertheless, seasonal distributions of most species at the population and individual levels still remain poorly understood, hampering our understanding of population-specific challenges and adaptations to survival across a rapidly changing landscape.
Climate change is expected to result in significant changes to temperature and precipitation regimes across South America (Torres et al., 2022). It is likely to have disproportionate effects on different populations of migratory birds, given that impacts of climate change on individual organisms depend on such factors as latitude, proximity to oceans, elevation and habitat (Newton, 2010) and operate along spatial, temporal, and individual axes (Bellard et al., 2012). For migratory organisms, impacts of abrupt climate change may lead to range losses, changes in migratory routes, and in departure and arrival dates, which can ultimately negatively affect their reproductive success (Parmesan, 2006; Zurell et al., 2018). Given the context-dependent nature of climate change impacts, predictions of its potential to affect organisms requires research on as fine a temporal, spatial and taxonomic scale as possible.
Previous research on how future climate change may affect migratory birds in South America suggest that closely related taxa (e.g., congeners) are likely to be affected in different ways, depending on their breeding and non-breeding ranges (Da Silveira et al., 2021). Our goal was to evaluate the population- and season-specific risks to future climate change of a common migratory bird in South America, to provide the first evaluation of risks to climate change between conspecific migratory bird populations on a continent where such risks are still poorly understood.
The Fork-tailed Flycatcher (Tyrannus savana) is a New World flycatcher (Tyrannidae) whose nominate subspecies has both intra-tropical migratory populations and Neotropical austral migrant populations (Tuero et al., 2019; Jahn and Tuero, 2020). Intra-tropical migration of Fork-tailed flycatchers includes populations that breed in central and southern Brazil and spend the non-breeding season in northern South America (Jahn et al., 2016; Jahn and Tuero, 2020). In contrast, Neotropical austral migration of Fork-tailed flycatchers involves migration of populations that breed at south-temperate latitudes, primarily southern Brazil, Uruguay, Paraguay and Argentina, and spend the non-breeding season in northern South America (Jahn and Tuero, 2020).
Future changes in both temperature and rainfall are expected across this species’ range (Ortega et al., 2021; Torres et al., 2022) and because it is a widespread species, populations breeding in different regions of the continent are likely to be differentially impacted as a result of regional differences in future climatic changes, as found in Turdus thrushes (Da Silveira et al., 2021). Furthermore, because Fork-tailed flycatchers track temperature during the breeding season, but primarily track rainfall during the non-breeding season (MacPherson et al., 2018), future challenges to their survival as a result of climate change are likely to be season-specific.
Specifically, we evaluated whether future climate change will lead to modifications in the size of breeding and non-breeding areas of Neotropical austral and intra-tropical migrant Fork-tailed flycatchers. We chose to study the changes in size of these areas, since the size of the area occupied by a species has been shown to be a key predictor of future risk of extinction due to climate change (Pearson et al., 2014). A recent study on the potential for future climate change to impact migratory patterns of the New World flycatchers found that Neotropical austral migratory flycatchers would lose most of their breeding and non-breeding areas and that intra-tropical migratory flycatcher species would on average lose all of both ranges (Braga et al., 2022). Because that study used species-level distribution data, we aimed to use data collected from flycatchers across conspecific populations to test whether the broader patterns seen at the family level were supported by data collected at the population level. Given the results of Braga et al. (2022), we expected losses of breeding and non-breeding areas of Fork-tailed flycatchers breeding at both south-temperate latitudes (Neotropical austral migrants) and tropical latitudes (intra-tropical migrants). We also expected that breeding area losses would be more severe in the latter.
2 Materials and methods
We used data on the location of Fork-tailed flycatchers (hereafter, “flycatchers”) using fixes derived from light-level geolocators attached to 28 individual flycatchers (see Jahn et al., 2013; Jahn et al., 2019 for details on geolocator deployment and data processing methods). Geolocators were deployed on flycatchers at two breeding sites of intra-tropical migrant flycatchers: the city of Brasília (n=4 geolocators) and the interior of São Paulo State (n=7), Brazil, both located in the Cerrado, a biome located in central Brazil that is characterized by highly seasonal dry and wet seasons, resulting in strong seasonality of insects (Amorim et al., 2009), and fruits (Batalha and Martins, 2004), which are a central part of the flycatcher diet (Jahn and Tuero, 2020). Geolocators were also deployed on breeding Neotropical austral migrant flycatchers in Buenos Aires Province (n=9) and La Pampa Province (n=8), Argentina. These two sites are located in the Pampas biome, which is a fertile plain primarily located in central and northern Argentina and Uruguay, and is characterized by both dry and wet seasons, as well as warm (summer) and cool (winter) seasons (Aliaga et al., 2017). This resulted in 4,721 fixes distributed across breeding and non-breeding seasons (see Supplementary Figure 1 for population- and season-specific locations and Supplementary Table 1 for sample sizes and definitions of start and end of seasons).
Using South America as a background, records containing geolocator errors and outliers (i.e., inaccurate coordinates) were removed. Records containing the same ID, date, and location were also removed. Additionally, all points contained in each cell of a grid with cells 1 km on a side were removed, resulting in a single point occurrence within each randomly chosen cell in the point rarefaction process. We conducted spatial rarefaction of occurrence data with a minimum distance of 5 km between pixels to further even out sample bias (clustering) in observer effort. We used program R v. 3.6.3 (R Core Team, 2018) for applying the filters and rarefaction of the occurrence points and program QGis (QGIS Development Team 3.12.0, 2015) for projecting the maps containing the records (Supplementary Figure 2).
2.1 Bioclimatic variables
Ecological niche models are commonly projected onto paleoclimates simulated with General Circulation Models (GCMs). A GCM is generally a mathematical representation of the physical processes operating in the atmosphere, ocean, cryosphere, and land surfaces and are widely applied in testing and predicting global climate responses to variations in planetary parameters on climate dynamics (Varela et al., 2015). For this, the respective GCMs from CMPI5 (Coupled Model Intercomparison Project, Phase 5 (http://cmip-pcmdi.llnl.gov/) hosted on the WorldClim platform (https://worldclim.org/) version 1 were used, at a resolution of approximately 2.5 km x 2.5 km at the Equator. The GCMs are: CCSM4 (University of Miami) - RSMAS, United States; MIROC-ESM Atmosphere and Ocean Research Institute (University of Tokyo), National Institute for Environmental Studies, and Japan Agency for Marine-Earth Science and Technology, Japan; MRI-CGCM3 Meteorological Research Institute, Japan). The GCMs obtained are for the present and future scenarios and were cut to exclusively comprise South America as background.
For the future scenario, we used the climate projections based on emission scenario IPCC AR-CMIP 5/RCP 8.5 (i.e., maximum energy requirement, balanced emissions between fossil and non-fossil sources; Intergovernmental Panel On Climate Change, 2014), which was also used by Braga et al. (2022). Braga et al. (2022) based their model simulations on the years 2080-2100, whereas we based ours on years 2041-2060 so as to inform conservation strategies that can be implemented before future impacts are too severe to mitigate. To reduce the dimensionality and collinearity of the 19 bioclimatic variables available on WorldClim (Giannini et al., 2013), we constructed a Principal Component Analysis (PCA) with standardized values, and for the axes with an eigenvalue above 1 (first five axes with 90% explanation of the variation), we selected the variables with the highest contribution through the loading values (Supplementary Figures 3, 4). For the non-breeding area models, we selected the following variables: BIO04 = Seasonal Temperature (standard deviation ×100), BIO06 = Minimum Temperature of the Coldest Month, BIO15 = Seasonal Precipitation (Coefficient of Variation), BIO17 = Precipitation of the Driest Quarter and BIO19 = Precipitation of the Coldest Quarter. For breeding area models, we selected: BIO04 = Seasonal Temperature, BIO05 = Maximum Temperature of the Warmest Month, BIO15 = Seasonal Precipitation, BIO16 = Precipitation of the Wettest Quarter and BIO18 = Precipitation of the Warmest Quarter.
All analyses were performed in program R with the raster (Hijmans and van Etten, 2016) and rgdal (Bivand et al., 2019) packages used to perform raster management. PCA was performed using the “prcomp” function of the vegan package. Maps containing occurrence points were processed using QGIS (QGIS Development Team 3.12.0, 2015), using the land cover mask “Land Use” from the EarthEnv platform (earthenv.org/landcover; Tuanmu and Jetz, 2014).
2.2 Ecological niche modeling
To predict the current potential distribution of breeding and non-breeding areas of Fork-tailed flycatcher populations and to simulate the effects of future climate change on these areas, we applied ecological niche modeling (ENM) approaches (Supplementary Figure 2). We used ensemble forecasting approaches based on the use of different ENM algorithms (Araújo and New, 2007), which is recommended as a protocol for building ENMs (Araújo et al., 2019) and is a widely used technique (Hao et al., 2019). The algorithms used to estimate the potential distributions of breeding and non-breeding areas are: MAXENT, Random Forests (RF) and Support Vector Machine (SVM). We used a machine learning (MLE) approach and cluster analysis (classification of observations into homogeneous groups; i.e., two or more), in which clustering is an unsupervised learning method (Guisan et al., 2017). These are based on presence and background data (MAXENT) and presence/pseudo absence (SVM and RF; Li et al., 2011). MAXENT is based on presence and background data, and uses maximum entropy density estimation in which the true distribution of the species is represented as a probability of distribution Y over a set X of locations in the study area (Phillips et al., 2006; Li et al., 2011). RF combines many classification trees to produce more accurate classifications. Byproducts of RF calculations include measures of variable importance and similarity measures of data points that can be used for clustering, multidimensional scaling, graphical representation, and missing value imputation (Cutler et al., 2006). The SVM algorithm maps the data into a new hyperspace, in which more complicated patterns can be represented simply, reducing the entry of outliers (Tax, 2001). For each population, ten True Skill Statistic (TSS) replicates were generated for each scenario (present day and one RCP85 scenario with 3 future GCMs for 2050), and for each scenario, three SVM algorithms were used (see above; Supplementary Figure 2; Supplementary Table 2). We used the TSS method for validation because it is not influenced by the prevalence of the study species (Allouche et al., 2006) and incorporates a threshold of maximum sensitivity and specificity, which is most appropriate for presence-only methods (Liu et al., 2016). To calculate this threshold, we divided the occurrence records into training and test folding, counting 70% and 30% of occurrence records, respectively (Peterson et al., 2011).
All resulting maps were overlaid using the frequency ensemble forecasting approach, using each constructed model’s threshold value of maximum sensitivity and specificity to transform the continuous map into a binary map to minimize omission errors, and balance sensitivity and specificity (Guisan et al., 2017). We then concatenated the replicates of the same algorithms as well as the replicates between algorithms and obtained a final continuous suitability map for the present and future projections (Supplementary Figure 2). Finally, we evaluated the biological meaning of the models, comparing the partial results obtained from the present with each future projection, and compared the influence of those variables on the choice of available areas and the geographical distribution of the species.
To calculate future stability, losses and gains of suitable areas, we transformed the final suitability maps with continuous values into binary maps using the 10th percentile threshold (Da Silveira et al., 2021), which estimates the suitable area by considering 90% of occurrences and omitting areas with low suitability generated by the remaining 10% of presences. It assumes that 10% of occurrence records in the least suitable habitat are not occurring in areas representative of the general distribution of the species and therefore should be omitted. Future projections of suitable breeding areas for populations breeding in Brasília and São Paulo extended to regions that we considered unrealistic (e.g., Venezuela), such that we restricted future suitable breeding areas for those two populations to within Brazil.
3 Results
3.1 Ecological niche modeling
In all, 480 models were generated, with 120 per population separated according to season, with the six models from different algorithms for the same season and projection (present vs. future) concatenated into a final continuous suitability map.
Populations breeding at south-temperate latitudes are projected to experience large breeding area losses (22.8%), primarily in the northern portions of their breeding areas (Table 1; Figure 1). Populations breeding at tropical latitudes will also experience large losses in breeding area (27.5%) (Table 1; Figure 2). Overall, both south-temperate and tropical breeders are projected to lose at least a fifth of their current breeding area (Table 1).
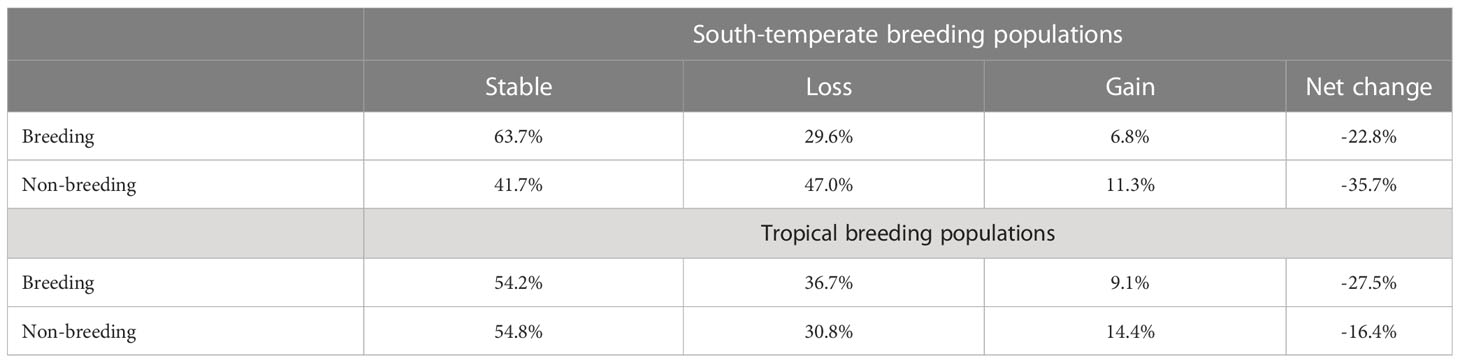
Table 1 Mean percent of migratory Fork-tailed Flycatcher breeding and non-breeding areas projected to remain stable, lose or gain area under RCP 8.5 by 2050.
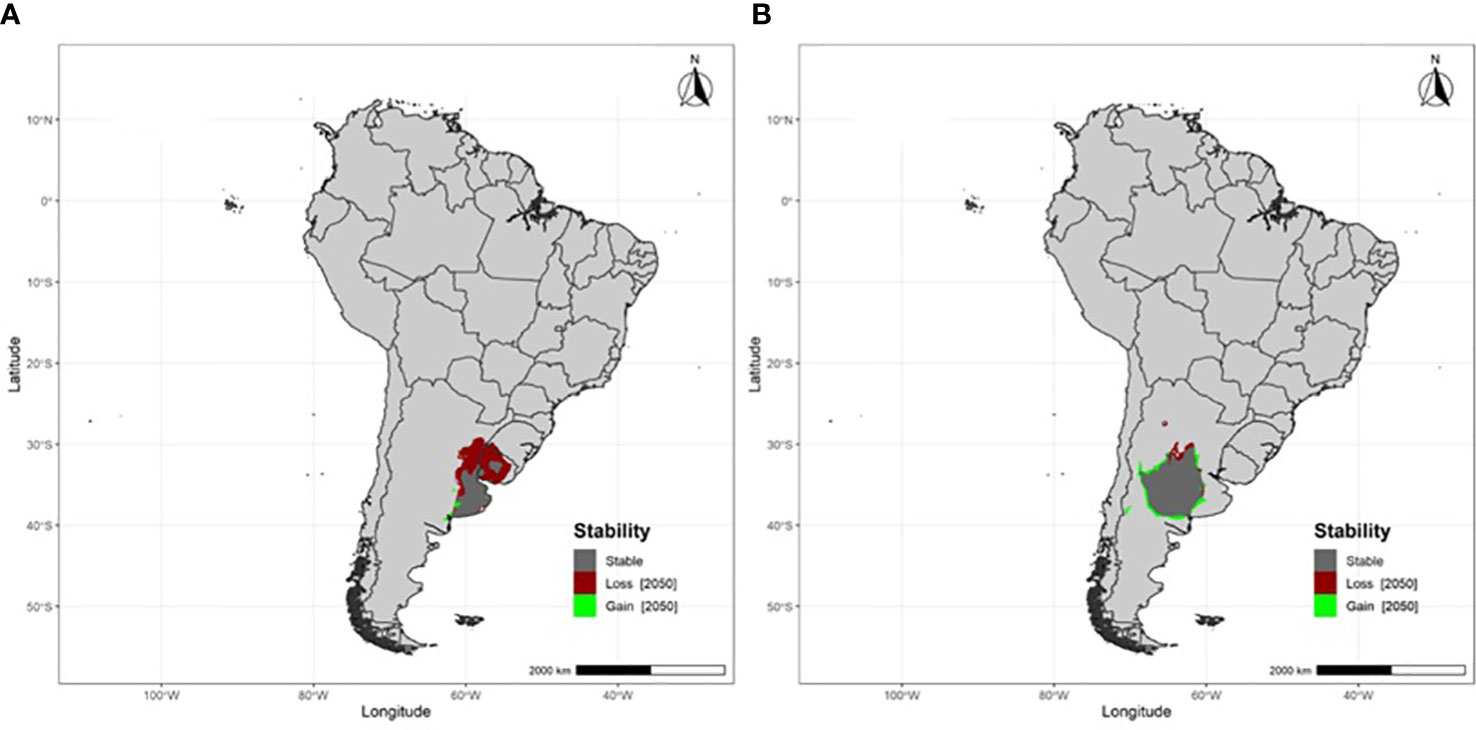
Figure 1 Projected breeding area stability, losses and gains under RCP 8.5 by 2050 for migratory Fork-tailed flycatchers breeding at south-temperate latitudes (A) Buenos Aires and (B) La Pampa, Argentina.
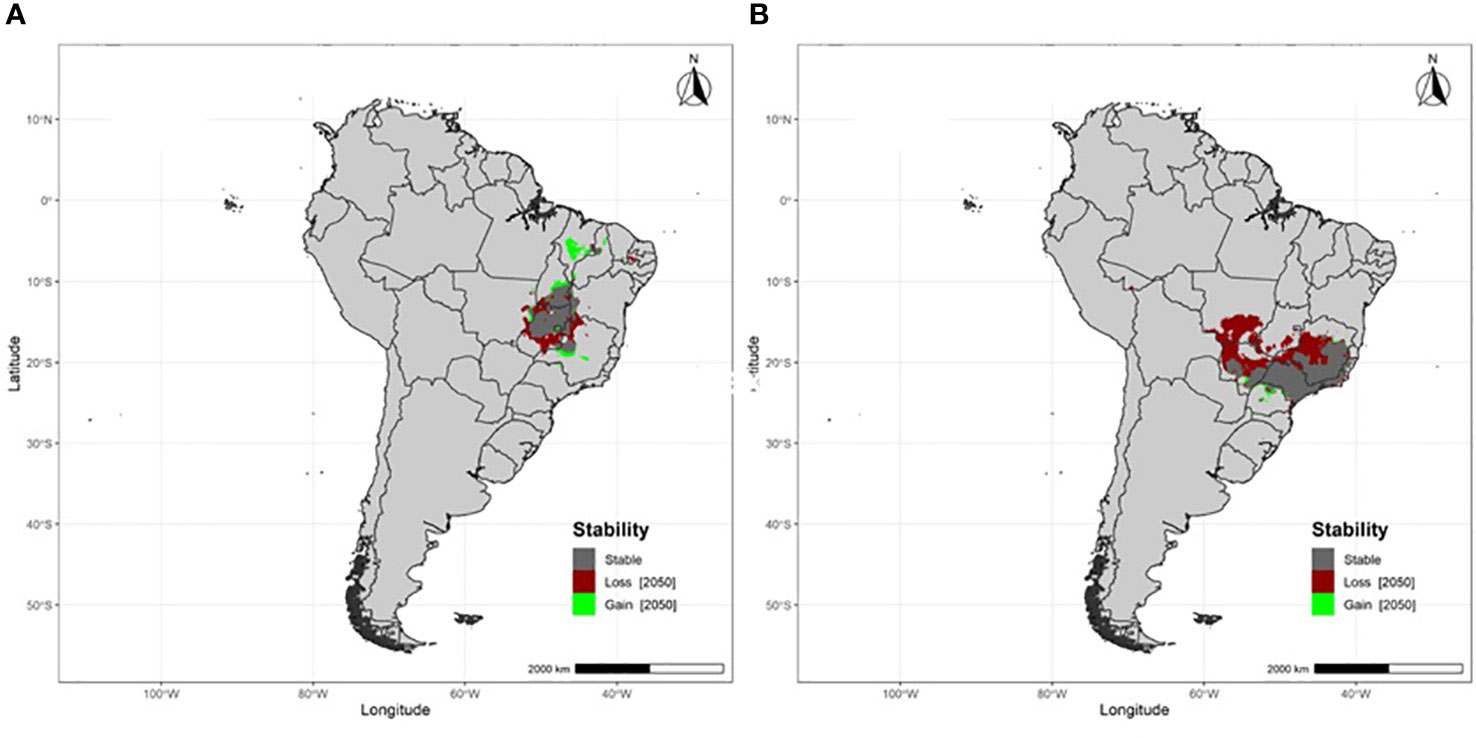
Figure 2 Projected breeding area stability, losses and gains under RCP 8.5 by 2050 for migratory Fork-tailed flycatchers breeding at tropical latitudes (A) Brasília and (B) São Paulo, Brazil.
Flycatchers breeding at south-temperate latitudes are projected to lose at least a third of their non-breeding area (Table 1), which will primarily occur across northwestern Amazonia and the grasslands (llanos) of the Orinoco River basin (Figure 3). Flycatchers breeding at tropical latitudes will experience fewer non-breeding area losses, losing 16.4% of that area (Table 1; Figure 4).
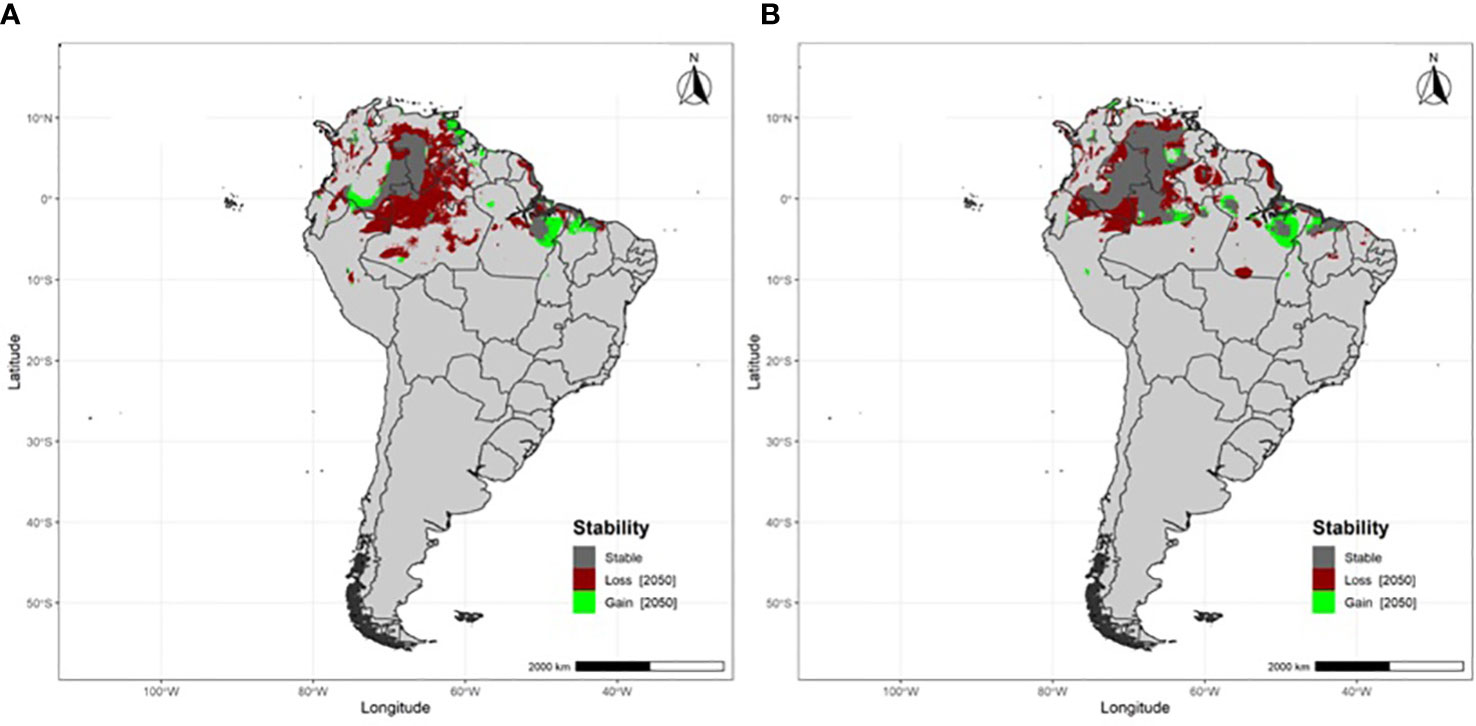
Figure 3 Projected non-breeding area stability, losses and gains under RCP 8.5 by 2050 for migratory Fork-tailed flycatchers breeding at south-temperate latitudes (A) Buenos Aires and (B) La Pampa, Argentina.
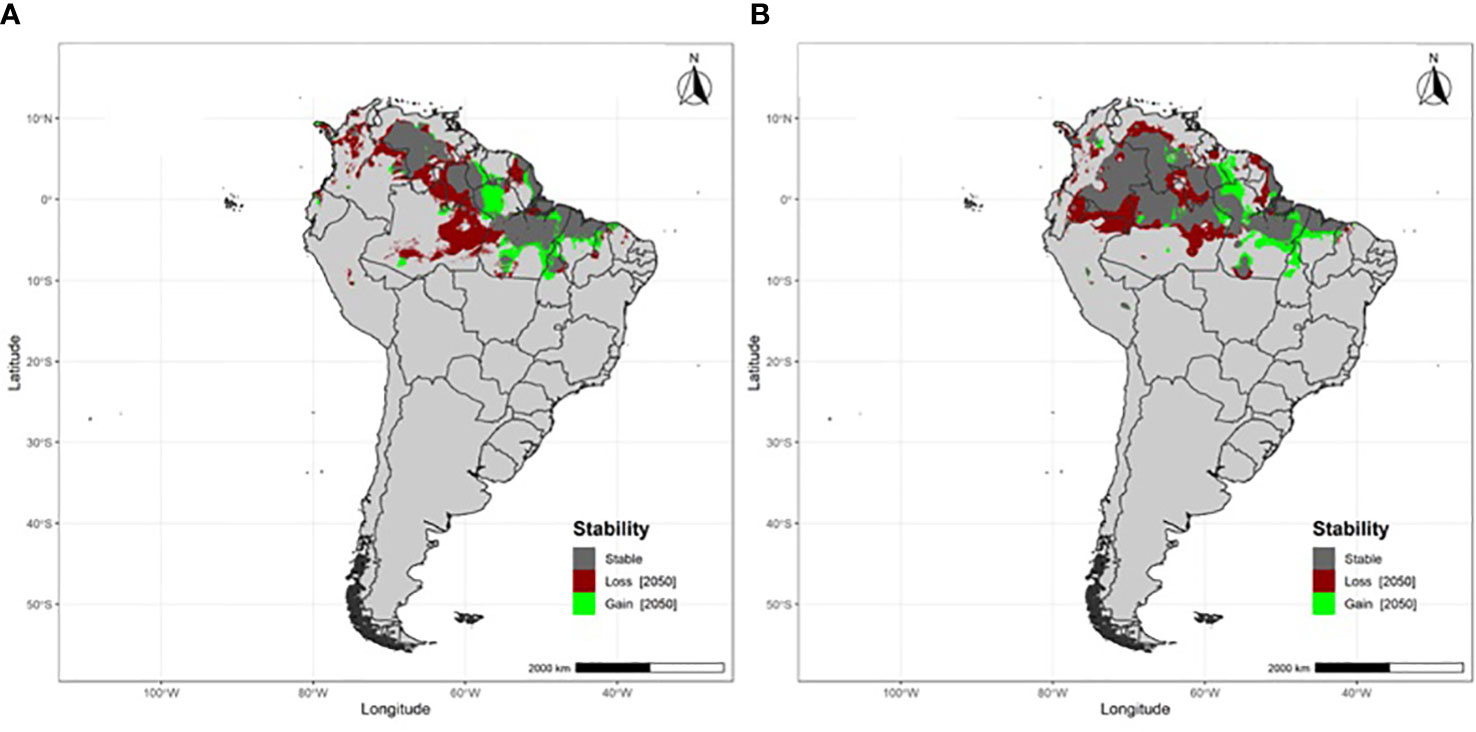
Figure 4 Projected non-breeding area stability, losses and gains under RCP 8.5 by 2050 for migratory Fork-tailed flycatchers breeding at tropical latitudes (A) Brasília and (B) São Paulo, Brazil.
4 Discussion
Our results suggest that there will be a substantial reduction in the size of the breeding and non-breeding areas of Fork-tailed Flycatcher populations breeding at south-temperate and tropical latitudes, as we expected. Flycatchers breeding at tropical latitudes will also lose a slightly larger fraction of their breeding area than will those breeding at south-temperate latitudes, supporting our expectations. Overall, our results suggest negative future impacts of climate change on Fork-tailed flycatchers, which will vary by season and population.
A recent study that evaluated future impacts of climate change on New World flycatchers at the species level found that migratory flycatcher species breeding in South America are likely to lose most and in some cases, all of their non-breeding area as a result of future climate change (Braga et al., 2022). Our results also project that Fork-tailed flycatchers will experience non-breeding area losses, although to a lesser degree as that found by Braga et al. (2022). Although that disparity may be due to a difference in analytical methods between the two studies (i.e., Braga et al., 2022 model simulations were for years 2080-2100, vs. those in our study for 2041-2060), it could also be because Fork-tailed flycatchers migrate longer distances than most other migrants that breed in South America. Only 32 bird species that breed in South America overwinter as far as Amazonia and only 14 overwinter north of the Amazon Basin (Stotz et al., 1996). Thus, Fork-tailed flycatchers may be at less of a risk to climate change during the non-breeding season than most austral migrants because they overwinter in northern South America (northern Amazonia and the Orinoco Basin, which are primarily in the Northern Hemisphere), where they would be impacted by climate changes occurring in a different part of the continent – and in a different hemisphere – than where the majority of austral and intra-tropical migratory bird species overwinter.
The higher stability projected for the non-breeding vs. breeding area of Fork-tailed Flycatchers breeding in Brasília and São Paulo holds potential consequences for future changes in their migratory behavior. Three non-migratory subspecies of Fork-tailed Flycatchers currently inhabit the non-breeding area of the migratory (nominate) subspecies in northern South America (T. savana monachus, T. sanctaemartae and T. circumdatus; Jahn and Tuero, 2020), which are derived via loss of migration from a migratory ancestor (Gómez-Bahamón et al., 2020). If future climatic conditions in northern South America are relatively favorable for residency of populations of Fork-tailed flycatchers breeding in central and southeastern Brazil, then they may become increasingly resident further north in South America, where they currently only overwinter. Indeed, areas exposed to long-term climate stability (refugia) are known to play a critical role in the persistence of biodiversity, particularly when regional or global climate conditions change (Terribile et al., 2012). Given that our results project that at least a fifth of the breeding area will be lost for the migratory subspecies, and that the non-migratory subspecies of Fork-tailed Flycatchers are derived from migratory ancestors (Gómez-Bahamón et al., 2020), a shift to residency (i.e., loss of migration) may be a favorable strategy for the migratory subspecies in the future.
The migratory subspecies may also be more vulnerable to future climate change than the resident subspecies as a result of the potential for a mismatch in the timing of food resource availability and the timing of migration (Both et al., 2010; Jones and Cresswell, 2010). Given that migratory Fork-tailed flycatchers track temperature during the breeding season and rainfall during the non-breeding season (MacPherson et al., 2018), they may find it increasingly challenging to match their migration timing to that of the resources they require throughout the year. This challenge may be heightened for populations that migrate the longest distances (i.e., those breeding in Argentina; Jahn et al., 2019), since long-distance migrants may be generally less able to advance their spring migration phenology (Jones and Cresswell, 2010). However, Fork-tailed Flycatchers breeding in Brazil may also be at risk to mismatching the timing of their migration relative to local phenology. The breeding success of intra-tropical migratory Fork-tailed flycatchers in southeastern Brazil rapidly declines as a function of a later arrival date at the breeding site (Bejarano and Jahn, 2018). Thus, with climate change, flycatchers breeding there may need to continually adjust their migratory schedule so as to avoid a mismatch between the timing of their arrival at those sites and that of local resource phenology, as has been found in migratory birds in the Northern Hemisphere (e.g., Both and Visser, 2001).
Notably, the populations that are projected to experience the greatest loss in breeding area (São Paulo and Buenos Aires) are those in closest proximity to the Atlantic Ocean, which is thought to currently provide climatic buffering to the southern portions of the continent (Paruelo et al., 2007). In contrast, populations breeding further inland (Brasília and La Pampa) are projected to lose the least area. Understanding the processes responsible for these differences will require an evaluation of the impact of climate change on flycatcher phenology, which will in turn require information on variation in phenological sensitivity and climate change exposure (Bailey et al., 2022), and variation in functional traits between populations (Schleuning et al., 2023).
Migratory birds breeding in South America may be able to successfully respond to future change by moving their breeding ranges towards higher, more southerly latitudes, as seen in a variety of terrestrial species (Chen et al., 2011). However, that option would potentially be limited by available space, given that South America is increasingly narrower towards its southern portions (Chesser, 1994). Another limitation may be that the life history strategy adopted by a population in a given region may not be adaptive if it needs to shift its range to another region, since life history traits can mediate phenological responses to environmental change (reviewed by Iler et al., 2021). For example, Fork-tailed flycatcher clutch sizes increase with latitude in South America (Jahn et al., 2014), such that a shift to breed at higher latitudes may require a larger annual investment in energy into reproduction. Sex-specific phenological responses to environmental change may also exist, resulting in a mismatch between the sexes in their ability to properly time life history events when responding to change (Williams et al., 2022).
This represents the first study to evaluate risks to climate change between conspecific migratory bird populations in South America, which is difficult to do without individual-level movement data, since intra-tropical and austral migrant flycatcher populations breed in allopatry, but spend the non-breeding season in sympatry, a common pattern among migratory birds in South America (Chesser, 1994; Jahn and Cueto, 2012). Thus, assessing season- and population-dependent risks to climate change for such migratory bird species requires an ability to distinguish between populations across seasons, since species-level distribution data are unable to do so. More generally, assessments of the risks of species to climate change often fail to incorporate season- and population-specific data, which our results suggest is important.
5 Conclusions
The effects of climate change on migratory birds that breed in the Neotropics have been little studied (Şekercioğlu et al., 2012; Jahn et al., 2020), and this study provides new evidence suggesting that populations of Fork-tailed Flycatchers and other ecologically similar migratory songbirds (e.g., insectivores inhabiting woodlands and grasslands) breeding in South America may be at risk to climate change. Nevertheless, the models we used are strictly climatic, such that further modeling that includes habitat, accounts for biotic interactions (e.g., intra- and inter-specific competition), season-specific behavioral ecology (e.g., dietary requirements; MacPherson et al., 2021), and pre- and post-breeding migration seasons are necessary to paint a more complete picture of these processes. Our results also point to the need for full annual cycle research on migratory birds in South America, which is a gap in research in this system (Tuero et al., 2019) and in ecological research generally (Marra et al., 2015). A priority should be research that incorporates various techniques (e.g., ecological genomics; Ruegg et al., 2018) to identify populations of migratory birds in South America that are most vulnerable to climate change, and ultimately evaluate their long-term population dynamics (e.g., Nater et al., 2023). These results provide novel insights into the complex challenges to survival that migratory birds at tropical and south-temperate latitudes will face in coming years, and point to an urgent need for research in these understudied systems to inform future conservation planning.
Data availability statement
The raw data supporting the conclusions of this article will be made available by the authors, without undue reservation.
Ethics statement
The animal study was reviewed and approved by Animal Use Ethics Commission at UNESP–Rio Claro, Brazil (#3/2014).
Author contributions
TH, NS, and MP contributed to conception and design of the study. TH and NS performed the statistical analysis. All authors contributed to manuscript writing and revision, and approved the submitted version.
Funding
This research was funded by São Paulo Research Foundation (FAPESP; grant # 2019/22228-0). MP receives a Research Grant (#304742/2019-8) from the Brazilian Council for Development of Science and Technology (CNPq). Part of the results presented were originally produced as part of a thesis project conducted at Universidade Estadual Paulista-Rio Claro: Hindi, T.S.E., 2022. Futuros efeitos climáticos sobre as rotas migratórias de uma ave sul-americana (Tyrannus savana; Passeriformes: Tyrannidae). AEJ was funded by Indiana University's Prepared for Environmental Change Initiative.
Conflict of interest
The authors declare that the research was conducted in the absence of any commercial or financial relationships that could be construed as a potential conflict of interest.
Publisher’s note
All claims expressed in this article are solely those of the authors and do not necessarily represent those of their affiliated organizations, or those of the publisher, the editors and the reviewers. Any product that may be evaluated in this article, or claim that may be made by its manufacturer, is not guaranteed or endorsed by the publisher.
Supplementary material
The Supplementary Material for this article can be found online at: https://www.frontiersin.org/articles/10.3389/fbirs.2023.1214458/full#supplementary-material
References
Aliaga V. S., Ferrelli F., Piccolo M. C. (2017). Regionalization of climate over the Argentine Pampas. Int. J. Climatol. 37, 1237–1247. doi: 10.1002/joc.5079
Allouche O., Tsoar A., Kadmon R. (2006). Assessing the accuracy of species distribution models: Prevalence, kappa and the true skill statistic (TSS). J. @ App. Ecol. 43, 1223–1232. doi: 10.1111/j.1365-2664.2006.01214.x
Amorim F. W., de Ávila R. S. Jr., de Camargo A. J. A., Vieira A. L., Oliveira P. E. (2009). A hawkmoth crossroads? Species richness, seasonality and biogeographical affinities of Sphingidae in a Brazilian Cerrado. J. @ Biogeog. 36, 662–674. doi: 10.1111/j.1365-2699.2008.02033.x
Araújo M. B., Anderson R. P., Barbosa A. M., Beale C. M., Dormann C. F., Early R., et al. (2019). Standards for distribution models in biodiversity assessments. Sci. Adv. 5, 5.40–5.45. doi: 10.1126/sciadv.aat4858
Araújo M. B., New M. (2007). Ensemble forecasting of species distributions. Trends Ecol. Evol. 22, 42–47. doi: 10.1016/j.tree.2006.09.010
Bailey L. D., van de Pol M., Adriaensen F., Arct A., Barba E., Bellamy P. E., et al. (2022). Bird populations most exposed to climate change are less sensitive to climatic variation. Nat. Commun. 13, 2112. doi: 10.1038/s41467-022-29635-4
Batalha M. A., Martins F. R. (2004). Reproductive phenology of the cerrado plant community in Emas National Park (central Brazil). Austral. J. @ Bot. 52, 149–161. doi: 10.1071/BT03098
Bejarano V., Jahn A. E. (2018). Relationship between arrival timing and breeding success of intra-tropical migratory Fork-tailed Flycatchers (Tyrannus savana). J. Field Ornith. 89, 109–116. doi: 10.1111/jofo.12251
Bellard C., Bertelsmeier C., Leadler P., Thuiller W., Courchamp F. (2012). Impacts of climate change on the future of biodiversity. Ecol. Lett. 15, 365–377. doi: 10.1111/j.1461-0248.2011.01736.x
Bivand R., Keitt T., Rowlingson B., Pebesma E., Summer M., Hijmans R., et al. (2019) Package rgdal: Bindings for the “Geospatial” Data Abstraction Library. R package version 1.4-8 0. Available at: https://cran.rproject.org/package=rgdal.
Both C., Van Turnhout C. A., Bijlsma R. G., Siepel H., Van Strien A. J., Foppen R. P. (2010). Avian population consequences of climate change are most severe for long-distance migrants in seasonal habitats. Proc. R. Soc B.: Biol. Sci. 277, 1259–1266. doi: 10.1098/rspb.2009.1525
Both C., Visser M. E. (2001). Adjustment to climate change is constrained by arrival date in a long-distance migrant bird. Nature 411, 296–298. doi: 10.1038/35077063
Braga M. R., Jorge L. R., Jahn A. E., Loyola R., Varela S. (2022). Future climate change will impact the migration of New World migrant flycatchers (Tyrannidae). Ornith. Res. 30, 63–74. doi: 10.1007/s43388-022-00081-6
Chen I., Hill J. K., Ohlermüller R., Roy D. B., Thomas C. D. (2011). Rapid range shifts of species associated with high levels of climate warming. Science 333, 1024. doi: 10.1126/science.1206432
Chesser R. T. (1994). Migration in South America: an overview of the austral system. Bird. Cons. Int. 4, 91–107. doi: 10.1017/S0959270900002690
Cutler D. R., Edwards T. C., Beard K. H., Cutler A., Hess K. T., Gibson J., et al. (2006). Random Forests for classification in ecology. Ecology 88, 2783–2792. doi: 10.1890/07-0539.1
Da Silveira N. S., Vancine M. H., Jahn A. E., Pizo M. A., Sobral-Souza T. (2021). Future climate change will impact the size and location of breeding and wintering areas of migratory thrushes in South America. Ornith. Appl. 123, 1–16. doi: 10.1093/ornithapp/duab006
Faaborg J., Holmes R. T., Anders A. D., Bildstein K. L., Dugger K. M., Gauthreaux S. A. Jr., et al. (2010). Recent advances in understanding migration systems of New World land birds. Ecol. Monog. 80, 3–48. doi: 10.1890/09-0395.1
Giannini T. C., Chapman D. S., Saraiva A. M., Alves-dos-Santos I., Biesmeijer J. C. (2013). Improving species distribution models using biotic interactions: a case study of parasites, pollinators and plants. Ecography 36, 649–656. doi: 10.1111/j.1600-0587.2012.07191.x
Gómez-Bahamón V., Marquez R., Jahn A. E., Miyaki C. Y., Tuero D. T., Laverde-R O., et al. (2020). Speciation associated with shifts in migratory behavior in an avian radiation. Curr. Biol. 30, 1312–1321. doi: 10.1016/j.cub.2020.01.064
Guisan A., Thuiller W., Zimmermann N. E. (2017). Habitat suitability and distribution models with applications in R (Cambridge: Cambridge University Press).
Hao T., Elith J., Guillera-Arroita G., Lahoz-Monfort J. J. (2019). A review of evidence about use and performance of species distribution modelling ensembles like BIOMOD. Diversity Distributions. 25, 839–852. doi: 10.1111/ddi.12892
Hijmans R. J., van Etten J. (2016). raster: Geographic Data Analysis and Modeling. R package version, 2.5-8.
Iler A. M., CaraDonna P. J., Forrest J. R., Post E. (2021). Demographic consequences of phenological shifts in response to climate change. Ann. Rev. Ecol. Evol. Syst. 52, 221–245. doi: 10.1146/annurev-ecolsys-011921-032939
Intergovernmental Panel On Climate Change (2014). Climate change 2014: Synthesis Report. Contribution of Working Groups I, II and III to the Fifth Assessment Report of the Intergovernmental Panel on Climate Change. Eds. Pachauri R. K., Meyer L. A. (Geneva, Switzerland: IPCC), 151. Core Writing Team.
Jahn A. E., Cereghetti J., Cueto V. R., Hallworth M. T., Levey D. J., Marini M. A., et al. (2019). Breeding latitude predicts timing but not rate of spring migration in a widespread migratory bird in South America. Ecol. Evol. 9, 5752–5765. doi: 10.1002/ece3.5159
Jahn A. E., Cueto V. R. (2012). The potential for comparative research across New World bird migration systems. J. Ornithol. 153, 199–205. doi: 10.1007/s10336-012-0849-8
Jahn A. E., Cueto V. R., Fontana C. S., Guaraldo A. C., Levey D. J., Marra P. P., et al. (2020). Bird migration within the Neotropics. Auk 137, ukaa033. doi: 10.1093/auk/ukaa033
Jahn A. E., Levey D. J., Cueto V. R., Ledezma J. P., Tuero D. T., Fox J. W., et al. (2013). Long-distance bird migration within South America revealed by light-level geolocators. Auk 130, 223–229. doi: 10.1525/auk.2013.12077
Jahn A. E., Levey D. J., Smith K. G. (2004). Reflections across hemispheres: a system-wide approach to New World bird migration. Auk 121, 1005–1013. doi: 10.1093/auk/121.4.1005
Jahn A. E., Seavy N. E., Bejarano V., Guzmán M. B., Provinciato I. C. C., Pizo M. A., et al. (2016). Intra-tropical migration and wintering areas of Fork-tailed Flycatchers (Tyrannus savana) breeding in São Paulo, Brazil. Orn. Res. 24, 116–121. doi: 10.1007/BF03544339
Jahn A. E., Tuero D. T. (2020)Fork-tailed Flycatcher (Tyrannus savana), version 1.0. In: Birds of the World (Ithaca, NY, USA: Cornell Lab of Ornithology) (Accessed April 20, 2023).
Jahn A. E., Tuero D. T., Mamani A. M., Bejarano V., Masson D. A., Aguilar E. (2014). Drivers of clutch-size in Fork-tailed Flycatchers (Tyrannus savana) at temperate and tropical latitudes in South America. Emu-Austral. Ornithol. 114, 337–342. doi: 10.1071/MU13084
Jones T., Cresswell W. (2010). The phenology mismatch hypothesis: are declines of migrant birds linked to uneven global climate change? J. Anim. Ecol. 79, 98–108. doi: 10.1111/j.1365-2656.2009.01610.x
Li W., Guo Q., Elkan C. (2011). Can we model the probability of presence of species without absence data? Ecography 34, 1096–1105. doi: 10.1111/j.1600-0587.2011.06888.x
Liu C., Newell G., White M. (2016). On the selection of thresholds for predicting species occurrence with presence-only data. Ecol. Evol. 6, 337–348. doi: 10.1002/ece3.1878
MacPherson M. P., Jahn A. E., DeFreitas J., Looknauth K., Wilson A., Baird L., et al. (2021). A trophic niche shift in a South American migrant: Stable nitrogen isotope signatures in feathers of Fork-tailed Flycatchers (Tyrannus savana). Wilson. J. @ Ornith. 133, 527–710. doi: 10.1676/20-00134
MacPherson M. P., Jahn A. E., Murphy M. T., Kim D. H., Cueto V. R., Tuero D. T., et al. (2018). Follow the rain? Environmental drivers of Tyrannus migration across the New World. Auk 135, 881–894. doi: 10.1642/AUK-17-209.1
Marra P. P., Cohen E. B., Loss S. R., Rutter J. E., Tonra C. M. (2015). A call for full annual cycle research in animal ecology. Biol. Lett. 11, 20150552. doi: 10.1098/rsbl.2015.0552
Nater C. R., Burgess M. D., Coffey P., Harris B., Lander F., Price D., et al. (2023). Spatial consistency in drivers of population dynamics of a declining migratory bird. J. @ Anim. Ecol. 92, 97–111. doi: 10.1111/1365-2656.13834
Ortega G., Arias P. A., Villegas J. C., Marquet P. A., Nobre P. (2021). Present-day and future climate over central and South America according to CMIP5/CMIP6 models. Int. J. Clim. 41, 6713–6735. doi: 10.1002/joc.7221
Parmesan C. (2006). Ecological and evolutionary responses to recent climate change. Ecol. Evol. 37, 637–639. doi: 10.1146/annurev.ecolsys.37.091305.110100
Paruelo J. M., Jobbágy E. G., Oesterheld M., Golluscio R. A., Aguiar M. R. (2007). “The grasslands and steppes of Patagonia and the Rio de la Plata Plains,” in The physical Geography of South America. Eds. Veblen T. T., Young K. R., Orme A. R. (Oxford, UK: Oxford Univ. Press), 232–248.
Pearson R. G., Stanton J. C., Shoemaker K. T., Aiello-Lammens M. E., Ersts P. J., Horning N., et al. (2014). Life history and spatial traits predict extinction risk due to climate change. Nat. Climate Change 4, 217–221. doi: 10.1038/nclimate2113
Peterson A. T., Soberón J., Pearson R. G., Anderson R. P., Martínez-Meyer E., Nakamura M., et al. (2011). Ecological niches and geographic Distributions (New Jersey: Princeton University Press).
Phillips S. J., Anderson R. P., Schapire R. E. (2006). Maximum entropy modeling of species geographic distributions. Ecol. Model. 190, 231–259. doi: 10.1016/j.ecolmodel.2005.03.026
QGIS Development Team 3.12.0 (2015) QGIS Geographic Information System. Open Source Geospatial Foundation Project. Available at: http://qgis.osgeo.org.
R Core Team (2018). R: A Language and Environment for Statistical Computing (Vienna: R Foundation for Statistical Computing). Available at: https://www.r-project.org/.
Ruegg K., Bay R. A., Anderson E. C., Saracco J. F., Harrigan R. J., Whitfield M., et al. (2018). Ecological genomics predicts climate vulnerability in an endangered southwestern songbird. Ecol. Lett. 21, 1085–1096. doi: 10.1111/ele.12977
Şekercioğlu Ç.H., Primack R. B., Wormworth J. (2012). The effects of climate change on tropical birds. Biol. Cons. 148, 1–18. doi: 10.1016/j.biocon.2011.10.019
Schleuning M., García D., Tobias J. A. (2023). Animal functional traits: Towards a trait-based ecology for whole ecosystems. Func. Ecol. 37, 4–12. doi: 10.1111/1365-2435.14246
Stotz D. F., Fitzpatrick F. W., Parker T. A. III, Moskovits D. K. (1996). Neotropical birds: Ecology and conservation (Chicago, Illinois, USA: University of Chicago Press).
Tax D. M. J. (2001). One-class classification: concept-learning in the absence of counter-examples [dissertation thesis] (Delft (NL: Delft University of Technology).
Terribile L. C., Lima-Ribeiro R. S., Araújo M. B., Bizão N., Collevatti R. G., Dobrovolski R., et al. (2012). Areas of climate stability of species ranges in the Brazilian cerrado: disentangling uncertainties through time. Natur. Conserv. 10, 152–159. doi: 10.4322/natcon.2012.025
Torres R. R., Benassi R. B., Martins F. B., Lapola D. M. (2022). Projected impacts of 1.5 and 2 C global warming on temperature and precipitation patterns in South America. Int. J. @ Clim. 42, 1597–1611. doi: 10.1002/joc.7322
Tuanmu M. N., Jetz W. (2014). A global 1-km consensus land cover product for biodiversity and ecosystem modelling. Global Ecol. Biogeog. 23, 1031–1045. doi: 10.1111/geb.12182
Tuero D. T., Jahn A. E., MacPherson M. (2019). “Bird migration in South America: The Fork-tailed Flycatcher (Tyrannus savana) as a case study,” in Behavioral Ecology of Neotropical Birds. Eds. Reboreda J. C., Fiorini V. D., Tuero D. T. (Cham, Switzerland: Springer), 133–154.
Varela S., Lima-Ribeiro M. S., Terribile L. C. (2015). A short guide to the climatic variables of the last glacial maximum for biogeographers. PloS One 10, e0129037. doi: 10.1371/journal.pone.0129037
Williams C. T., Chmura H. E., Deal C. K., Wilsterman K. (2022). Sex-differences in phenology: a tinbergian perspective. Int. Comp. Biol. 62, 980–997. doi: 10.1093/icb/icac035
Keywords: austral, fork-tailed flycatcher, intra-tropical migration, neotropical, species distribution models
Citation: El Hindi TS, Jahn AE, Tuero DT, Pizo MA and Da Silveira NS (2023) Projected population- and season-dependent impacts of climate change on a migratory songbird in South America. Front. Bird Sci. 2:1214458. doi: 10.3389/fbirs.2023.1214458
Received: 29 April 2023; Accepted: 24 July 2023;
Published: 14 August 2023.
Edited by:
Frank A. La Sorte, Cornell University, United StatesReviewed by:
Nadinni Sousa, Ministry of the Environment and Climate Change, BrazilJesús Domínguez, University of Santiago de Compostela, Spain
Copyright © 2023 El Hindi, Jahn, Tuero, Pizo and Da Silveira. This is an open-access article distributed under the terms of the Creative Commons Attribution License (CC BY). The use, distribution or reproduction in other forums is permitted, provided the original author(s) and the copyright owner(s) are credited and that the original publication in this journal is cited, in accordance with accepted academic practice. No use, distribution or reproduction is permitted which does not comply with these terms.
*Correspondence: Alex E. Jahn, YWxleGphaG5AaXUuZWR1