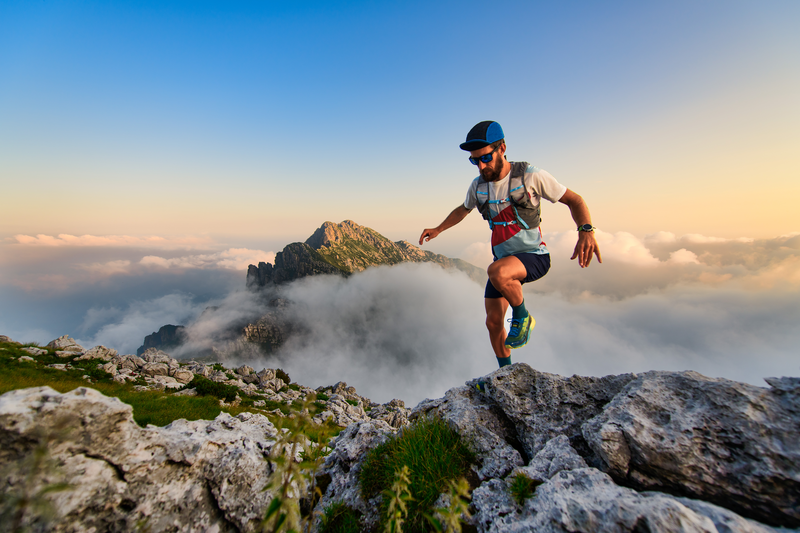
94% of researchers rate our articles as excellent or good
Learn more about the work of our research integrity team to safeguard the quality of each article we publish.
Find out more
EDITORIAL article
Front. Bioeng. Biotechnol. , 14 March 2025
Sec. Biomaterials
Volume 13 - 2025 | https://doi.org/10.3389/fbioe.2025.1568490
This article is part of the Research Topic Tissue Engineering and Regenerative Medicine: Advances, Controversies, and Future Directions View all 6 articles
Editorial on the Research Topic
Tissue engineering and regenerative medicine: advances, controversies, and future directions
Tissue engineering and regenerative medicine have undergone significant advancements over the last years, involving scientists, clinicians, and industrial partners alike. At the intersection of engineering, biology and medical science, interdisciplinary approaches will offer new therapeutic strategies from debilitating conditions to millions of patients suffering (Sahakyants and Vacanti, 2020). Three-dimensional bioprinting has been one of the most striking developments in tissue engineering over the last years. 4D and 5D bioprinting will soon allow for the precise fabrication of more complex tissue structure (Abolhassani et al., 2025). Such technology enabled creating of organoids, simple organs and cutting-edge tissue design, which offers new pathways in personalized medicine. Furthermore, advances in biomaterials design, including hydrogels or bioactive scaffolds, help mimic the extracellular matrix in a dynamic environment, facilitating and expediting tissue regeneration and ingrowth (Choi et al., 2024). Stem cell technology introducing pluripotent stem cells, providing ethically viable and patient-own cells in regenerative medicine. Advancements in acellularization and recolonizing tissues, structures and even organs comprising vasculature gain increasing attention. Using acellular scaffolds helps preserve the intrinsic tissue structure and main components of the formed backbone of the matrix (Verboket et al., 2024). When combined with patient-derived cell lines, such scaffolds offer promising platforms for tissue regeneration due to their low antigenicity. Bioreactors may help provide a highly controlled environment to facilitate the ex vivo growth of tissue-engineered scaffolds, which may soon become suitable at an industrial scale for clinical application.
Despite such developments, controversy and challenges persist. In addition to ethical constrains related to tissue harvesting, accessibility and affordability may form bottlenecks in prospective use. Scalability and reproducibility of tissue engineered scaffolds form further challenges. Lacking clinical trials and long-term experience will all become hurdles to make tissue engineered scaffolds become safe and robust solutions for the patients. Challenges lie even earlier in tissue engineering, as morpho-mechanical properties–both the existing ones and the desired ones for tissue engineered scaffolds–remain largely unknown. Standardized protocols at all steps of tissue engineering are needed to address this aspect.
Tissue mechanical properties are strictly linked to their morphological features, on a scale ranging from macro to nano (Lozano et al., 2019). It is vital to understand how biological materials respond to both intrinsic and external forces to advance applications in tissue engineering. Using the combined knowledge of the relationship between tissue structure, composition, and load-deformation properties will help design tailored models, tissue scaffolds, and treatments for future clinical needs (Kuniakova et al., 2024).
Determining an appropriate sample size is crucial in biomechanical research, as it directly impacts reliability and reproducibility. Small samples reduce stability, while excessive sampling is impractical, particularly for human tissues (Hammer et al., 2023). Sample size depends on error tolerance, statistical methods, and morpho-mechanical properties, with high-precision studies often requiring large samples. Standardized methodologies are essential to account for post-mortem change and tissue heterogeneity, ensuring efficient resource use and reliable results for surgical and tissue-engineering advancements. Tissue anisotropy, heterogeneity, and hydration add complexity to biomechanical testing (Zwirner et al., 2020b). Collagen alignment, fiber density, and extracellular matrix composition influence properties like tensile strength and elastic modulus, while layered tissues enhance load resistance. Hydration also plays a critical role, as water content significantly affects deformation behavior (Lozano et al., 2019). These factors highlight the importance of controlled testing conditions.
Mechanical setups significantly affect testing accuracy, with sample geometry and clamping conditions playing a key role (Zwirner et al., 2020b). Tapered samples improve stress distribution, while advanced clamping technologies, such as resin-enforced and 3D-printed grips, reduce slippage and distortion, enhancing reproducibility (Scholze et al., 2018; Horvath et al., 2024). Load rates influence tissue stiffness and extensibility, reflecting viscoelastic behavior (Zwirner et al., 2023). Measurement innovations like digital image correlation provide accurate, non-intrusive data, further improving biomechanical modeling for surgical and scaffold applications (Zwirner et al., 2020a).
The future of tissue engineering and regenerative medicine lies in converging and leveraging emerging technologies. Artificial intelligence, machine learning, and automation are expected to accelerate progress by optimizing biomaterial design, predicting patient-specific outcomes, and refining bioprinting techniques. Gene-editing tools may unlock new treatment opportunities for genetic disorders, thereby enhancing tissue functionality.
To achieve these goals, effective interdisciplinary collaboration will be essential to overcome the limitations currently faced. Partnerships among engineers, biologists, scientists, and clinicians will play a pivotal role in ensuring that technological advancements are translated into safe, effective, and accessible therapies. Emphasizing collaboration, standardization, and alignment with policymakers could significantly expedite the transition to human trials and clinical practice.
While tissue engineering and regenerative medicine present significant challenges, they also offer vast opportunities. The full potential of this transformative field can only be realized through collaboration, as highlighted by the contributions featured Research Topic entitled “Tissue Engineering and Regenerative Medicine: Advances, Controversies, and Future Directions”.
Our Research Topic includes five articles addressing some of the most emerging questions: The review and research paper by Lin et al. assessed testing parameters for uniaxial testing of human soft tissues, as no standards exist to date for biological materials. They found broad variability in sample shape and clamping conditions, with subsequent numerical simulations suggesting that geometry impacts stress distribution, indicating the need for standardized reporting of the testing environment. Jiang et al. in their review explore the use of gene-modified induced mesenchymal stem cells (iMSCs) and enhanced MSC-derived exosomes as a potential therapeutic approach for the treatment of osteoarthritis and cartilage repair. Ansari et al. review different combinations of hydrogels, biomaterials, combined with advanced strategies such as drug delivery and mechano-signaling for cartilage repair, highlighting their potential to overcome the limitations of traditional osteoarthritis therapies. The study of Josino and Stimamiglio demonstrates that bioactive decellularized extracellular matrix-based hydrogel do support human adipose-derived stem cell maintenance and fibrocartilage phenotype. Jovic et al. found that isolated fibronectin-adherent chondroprogenitor cells did not offer significant benefits, highlighting the need for improved isolation methods and markers in future.
SŽ: Writing–original draft, Writing–review and editing. ĽD: Writing–original draft, Writing–review and editing. NH: Writing–original draft, Writing–review and editing.
The author(s) declare that no financial support was received for the research and/or publication of this article.
The authors declare that the research was conducted in the absence of any commercial or financial relationships that could be construed as a potential conflict of interest.
The author(s) declare that Generative AI was used in the creation of this manuscript.
All claims expressed in this article are solely those of the authors and do not necessarily represent those of their affiliated organizations, or those of the publisher, the editors and the reviewers. Any product that may be evaluated in this article, or claim that may be made by its manufacturer, is not guaranteed or endorsed by the publisher.
Abolhassani, S., Fattahi, R., Safshekan, F., Saremi, J., and Hasanzadeh, E. (2025). Advances in 4D bioprinting: the next frontier in regenerative medicine and tissue engineering applications. Adv. Healthc. Mater 14 (4), e2403065. doi:10.1002/adhm.202403065
Choi, H., Choi, W. S., and Jeong, J. O. (2024). A review of advanced hydrogel applications for tissue engineering and drug delivery systems as biomaterials. Gels 10 (11), 693. doi:10.3390/gels10110693
Hammer, N., Ondruschka, B., Berghold, A., Kuenzer, T., Pregartner, G., Scholze, M., et al. (2023). Sample size considerations in soft tissue biomechanics. Acta Biomater. 169, 168–178. doi:10.1016/j.actbio.2023.07.036
Horvath, P., Gläser, F., Antipova, V., Klug, C., Lin, A. C., Scholze, M., et al. (2024). Advanced 3D-printed capstan clamping system for accurate uniaxial tensile testing of biological soft tissues. Appl. Sci. 14 (24), 11537. doi:10.3390/app142411537
Kuniakova, M., Novakova, Z. V., Haspinger, D., Niestrawska, J. A., Klein, M., Galfiova, P., et al. (2024). Effects of two decellularization protocols on the mechanical behavior and structural properties of the human urethra. Int. J. Mol. Sci. 25 (22), 12361. doi:10.3390/ijms252212361
Lozano, P. F., Scholze, M., Babian, C., Scheidt, H., Vielmuth, F., Waschke, J., et al. (2019). Water-content related alterations in macro and micro scale tendon biomechanics. Sci. Rep. 9 (1), 7887. doi:10.1038/s41598-019-44306-z
Sahakyants, T., and Vacanti, J. P. (2020). Tissue engineering: from the bedside to the bench and back to the bedside. Pediatr. Surg. Int. 36 (10), 1123–1133. doi:10.1007/s00383-020-04722-z
Scholze, M., Singh, A., Lozano, P. F., Ondruschka, B., Ramezani, M., Werner, M., et al. (2018). Utilization of 3D printing technology to facilitate and standardize soft tissue testing. Sci. Rep. 8 (1), 11340. doi:10.1038/s41598-018-29583-4
Verboket, R. D., Henrich, D., Janko, M., Sommer, K., Neijhoft, J., Sohling, N., et al. (2024). Human acellular collagen matrices-clinical opportunities in tissue replacement. Int. J. Mol. Sci. 25 (13), 7088. doi:10.3390/ijms25137088
Zwirner, J., Ondruschka, B., Scholze, M., and Hammer, N. (2020a). Surface coating and speckling of the human iliotibial tract does not affect its load-deformation properties. Sci. Rep. 10 (1), 20747. doi:10.1038/s41598-020-77299-1
Zwirner, J., Ondruschka, B., Scholze, M., Thambyah, A., Workman, J., Hammer, N., et al. (2023). Dynamic load response of human dura mater at different velocities. J. Mech. Behav. Biomed. Mater 138, 105617. doi:10.1016/j.jmbbm.2022.105617
Zwirner, J., Scholze, M., Ondruschka, B., and Hammer, N. (2020b). What is considered a variation of biomechanical parameters in tensile tests of collagen-rich human soft tissues? - critical considerations using the human cranial dura mater as a representative morpho-mechanic model. Med. Kaunas. 56 (10), 520. doi:10.3390/medicina56100520
Keywords: bioprinting, biomaterials, regenerative medicine, stem cells, tissue engineering
Citation: Žiaran S, Danišovič Ľ and Hammer N (2025) Editorial: Tissue engineering and regenerative medicine: advances, controversies, and future directions. Front. Bioeng. Biotechnol. 13:1568490. doi: 10.3389/fbioe.2025.1568490
Received: 29 January 2025; Accepted: 03 March 2025;
Published: 14 March 2025.
Edited and reviewed by:
Candan Tamerler, University of Kansas, United StatesCopyright © 2025 Žiaran, Danišovič and Hammer. This is an open-access article distributed under the terms of the Creative Commons Attribution License (CC BY). The use, distribution or reproduction in other forums is permitted, provided the original author(s) and the copyright owner(s) are credited and that the original publication in this journal is cited, in accordance with accepted academic practice. No use, distribution or reproduction is permitted which does not comply with these terms.
*Correspondence: Niels Hammer, bmxzaGFtbWVyQGdvb2dsZW1haWwuY29t, bmllbHMuaGFtbWVyQG1lZHVuaWdyYXouYXQ=
Disclaimer: All claims expressed in this article are solely those of the authors and do not necessarily represent those of their affiliated organizations, or those of the publisher, the editors and the reviewers. Any product that may be evaluated in this article or claim that may be made by its manufacturer is not guaranteed or endorsed by the publisher.
Research integrity at Frontiers
Learn more about the work of our research integrity team to safeguard the quality of each article we publish.