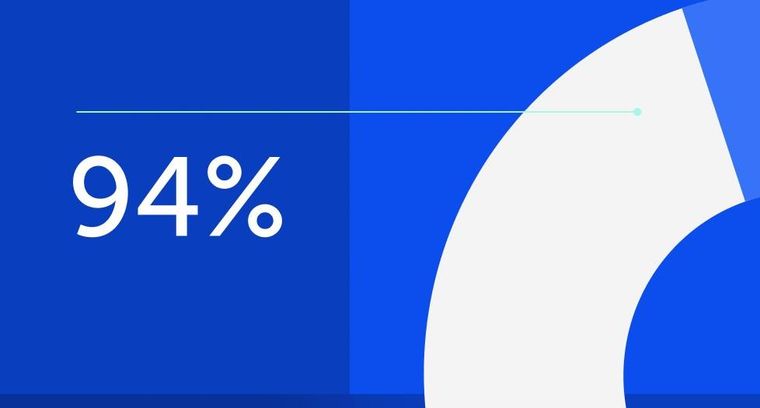
94% of researchers rate our articles as excellent or good
Learn more about the work of our research integrity team to safeguard the quality of each article we publish.
Find out more
ORIGINAL RESEARCH article
Front. Bioeng. Biotechnol., 04 April 2025
Sec. Nanobiotechnology
Volume 13 - 2025 | https://doi.org/10.3389/fbioe.2025.1458965
This article is part of the Research TopicInsights In Nanobiotechnology 2024: Novel Developments, Current Challenges and Future PerspectivesView all articles
Introduction: Pesticides such as isoproturon are widely employed and represent a considerable environmental concern. The development of sustainable and efficient degrading techniques is crucial. Photocatalytic degradation employing semiconductor materials is a compelling solution. This study examines the synergistic advantages of heterojunction formation by synthesizing, characterizing, and improving the photocatalytic efficacy of Ag3PO4/SnO2 nanocomposites for the degradation of isoproturon.
Methods: The Ag3PO4/SnO2 nanocomposite was characterised using powder X-ray diffraction (PXRD), Fourier Transform Infrared Spectroscopy (FTIR), Scanning Electron Microscopy (SEM), Ultraviolet-Diffuse Reflectance Spectroscopy (UV-DRS) and X-ray Photoelectron Spectroscopy (XPS). The effective synthesis of the Ag3PO4/SnO2 heterojunction was confirmed by characterization data from various techniques (PXRD, FTIR, SEM, UV-DRS, XPS).
Results and Discussion: Elemental mapping confirmed uniform distribution of O, P, Ag, and Sn. High-resolution mass spectrometry (HRMS) was employed to analyse degradation products. The Ag3PO4/SnO2 nanocomposite exhibited improved photocatalytic degradation of isoproturon compared to its precursors. In contrast to 25% for pure SnO2 and 41% for Ag3PO4, over 97% degradation was achieved using Ag3PO4/SnO2 nanocomposite within 120 min of light irradiation under identical conditions. The synergistic effects of heterojunction formation significantly enhanced isoproturon degradation using the Ag3PO4/SnO2 nanocomposite. The heterojunction reduces electron-hole recombination rate and enhances photogenerated charge carriers for degradation via effective charge separation. The improved photocatalytic activity is ascribed to the increased surface area of the nanocomposite. The analysis of HRMS data revealed the degradation products. The findings demonstrate the efficacy of Ag3PO4/SnO2 nanocomposites as photocatalysts for environmental remediation, namely in the breakdown of pesticides.
Pesticides serve as chemical agents designed to safeguard crops from detrimental pests and diseases affecting humans. The positive ramifications of employing pesticides render them a crucial tool for upholding and elevating the global population’s quality of life. The annual consumption of pesticides across the world is about two million tons (De et al., 2014). The term pesticide conventionally includes herbicides, insecticides, rodenticides, and fungicides, etc., depending upon the targeted species (Sharma et al., 2019). Herbicides and insecticides represent the predominant types of pesticides, constituting 47.5% and 29.5%, respectively, of the overall pesticide utilization (De et al., 2014). Globally, countries like India, China, Japan, Canada, and Brazil, etc., are the major consumers of pesticides (Sharma et al., 2019). Also, most of the pesticides undergo biological magnification which means their concentration increases on moving to higher trophic level. This makes the aquatic organism hazardous for consumption. Significantly, prolonged exposure to pesticides through water ingestion can emulate the hormonal functions within the human body, thereby compromising immune response, disrupting hormone equilibrium, eliciting reproductive-related complications, imparting carcinogenic effects, and diminishing cognitive abilities, particularly among children in the developmental stage (Yadav et al., 2015).
Use of pesticides in agriculture is concerning as these chemicals are water soluble, and the rate of chemical and biological degradation is very slow. Ground and surface water are contaminated due to poor agricultural practices, reckless dumping of empty containers, and equipment washing (Spliid and Køppen, 1998). In the interest of public health protection, various nations have published guidelines for permissible levels of pesticides in drinking water. Discrepancies in permissible pesticide levels in drinking water may arise due to socio-economic, dietary, geographical, and industrial variations (Hamilton et al., 2003). Agricultural soils around the world are heavily treated with phenyl urea herbicides and Isoproturon (IPU) to control weeds. IPU is a systemic herbicide used to manage broad-leaved weeds and annual grasses in agricultural fields. As a result, various methods for removing these contaminants from water have been developed such as ozonation (Magara et al., 1995), electrical discharge (Malik et al., 2001), activated carbon nanofiltration (Appleman et al., 2013), etc. The application, efficiency, and cost of these operations are all constrained by their very nature (Yeoh et al., 2022). Ozonation does not lead to mineralisation of organic contaminants (Von Sonntag and Von Gunten, 2012) and oxidised byproducts are formed (von Gunten, 2003) while electrical discharge has shown problems in scaling up (Zeghioud et al., 2020). One of the most promising methods to treat contaminated water is photocatalysis. Photocatalysis is an environmentally friendly degradation method which utilizes a photocatalyst to decompose pollutants using oxidation or reduction process in the presence of light irradiation (Ahmed et al., 2021; El-Saeid et al., 2021; Dhenadhayalan et al., 2022). AOPs typically rely on the production of OH• radicals that interact with organic contaminants to cause gradual deterioration and ultimately whole-body mineralization (Verma et al., 2014). An efficient photocatalyst must have various characteristics, including non-toxicity, high efficiency, low cost, recyclable, and an effective light absorber. Metal oxide nanoparticles have semiconducting characteristics and having large surface area. As a result, these materials serve an important role as photocatalysts for the degradation of contaminants.
There have been a lot of photocatalysts used for photocatalysis over the years. But recently a p-type semiconducting material silver phosphate (Ag3PO4), has witnessed a surge in popularity due to its capability of utilizing visible light to decompose water molecules and disintegrate organic pollutants (Kamiuchi et al., 2010). Nevertheless, the practical application of Ag3PO4 is hindered by photo corrosion due to reduction of Ag+ ions by the photoexcited electrons during the photocatalytic process (Chen et al., 2015). A widely employed technique to mitigate photo corrosion and enhance the overall photocatalytic efficiency involves the integration of Ag3PO4 with other semiconductors, resulting in the formation of an Ag3PO4-semiconductor composite. Zheng et al. (2017) reported higher efficiency of Ag3PO4/Bi4Ti3O12 as compared to pure Ag3PO4 and Bi4Ti3O12 for breakdown of Rhodamine B (RhB) under simulated solar irradiation. The degradation rate of composite containing 10% molar Bi4Ti3O12 was 2.6 times higher as compared to pure Ag3PO4. Formation of heterojunction facilitate increased charge separation between both the molecules which resulted in high photocatalytic efficiency. Qi et al. studied the photocatalytic performance of Ag3PO4-BiOCl1-xBrx for the degradation of phenol under artificial sunlight (Qi et al., 2018). Ag3PO4 has recently been found to couple with additional wide-band gap semiconductors, including WO3 (Lu et al., 2017), TiO2 (Liu and Perng, 2020), and ZnO (Yu et al., 2020). In order to create effective photocatalysts, much effort has been put into synthesising Ag3PO4-semiconductor composites with acceptable band gap. One of the most significant n-type semiconductors is tin dioxide (SnO2). By interacting with other semiconductors, SnO2 is known to be effective owing to formation of p-n junctions (We et al., 2019; Wen et al., 2017). Additionally, there aren't a lot of studies on the synthesis of Ag3PO4/ SnO2 composites and the analysis of their characteristics in the literature (Liu et al., 2020; Zhang et al., 2012; Li et al., 2019a). For instance, when subjected to visible light irradiation, the Ag3PO4/SnO2 composite synthesised by Zhang et al. (Liu et al., 2020) showed excellent photocatalytic activity to facilitate the photodegradation of methyl orange dye. The efficient e−-h+ separation was attributed for the enhancement in the photocatalytic performance. Li et al. also synthesized Ag3PO4/SnO2 catalyst using hydrothermal method (Li et al., 2019a). The catalyst exhibits significantly improved tetracycline degradation under visible light irradiation compared to Ag3PO4 and SnO2. Optimum conditions yielded a 74% degradation within 60 min. Gabriela et al. also synthesized Ag3PO4/SnO2 composite with varying SnO2 ratios (Silva et al., 2021). Min Liu et al. prepared Ag3PO4/SnO2 heterojunctions on carbon cloth using a simple two-step process (Liu et al., 2020). The synthesis method involved deposition of SnO2 on carbon cloth followed by in-situ growth of Ag3PO4 nanoparticles. The catalyst demonstrated significantly improved photocatalytic activity degrading 95% of RhB within 60 min.
In this study, Ag3PO4/ SnO2 nanocomposite was prepared via hydrothermal method. The composite was analysed using various analytical techniques. The composite was used for the photocatalytic degradation of IPU. The nanocomposite degraded 97% of pesticide in 120 min. This work opens up new pathways for the utilisation of Ag3PO4/ SnO2 nanocomposite in environmental remediation methods.
The reagents used in this study were commercially available. The chemicals were directly used without further purification. SnCl2•2H2O (≥99.0% purity) and CH3OH (≥99.0% purity) from Merck Chemicals, H2O2 (Fisher Scientific, 30% w/v), AgNO3 (99.9% purity) from Rehsiff scientific, and Na2HPO4 (99.5% purity) were purchased from Fisher scientific.
For the synthesis of Ag3PO4/SnO2 nanocomposites, 1.32 mM methanolic solution of SnO2 (S.I.1) was sonicated for 60 min. To this solution, 3 mM aqueous solution of AgNO3 (S.I.2) was added and sonicated for 40 min. It was followed by dropwise addition of 49.3 mM aqueous Na2HPO4 solution. Subsequently, the reaction solution was kept in Teflon vessel in hydrothermal at 150°C for 15 h. Then the synthesized Ag3PO4/SnO2 nanocomposite were washed with water several times and dried in an oven at 80°C. Pure Ag3PO4 and SnO2 nanoparticles were synthesized using same method at same conditions and the synthesis method have been described in Supplementary Material.
A specially designed photocatalytic reactor equipped with water circulatory system was used for the study of IPU degradation as described in our previous work (Kumar et al., 2022). Water circulation around the reactor helps in cooling. A 125-W mercury lamp (Philips, India) was utilised as a source of UV light (Bhawna et al., 2023). A 100 mL solution of 50 μM isoproturon was taken in photocatalytic reactor along with 100 mg of synthesized photocatalyst. The solution was stirred for 30 min in dark in order to reach the adsorption/desorption equilibrium before light irradiation. A 5 mL of the IPU solution was pipetted out at regular intervals and was centrifuged. The UV-visible spectrum was recorded to check the degradation efficiency.
The Powder X-Ray Diffraction pattern of Ag3PO4/SnO2 nanocomposite (NC) and is pure Ag3PO4 depicted in Figures 1a, b, respectively. The characteristic planes (110), (101) and (211) corresponding to SnO2 phase reveal a tetragonal crystal system with a rutile structure having space group P42/mnm (JCPDS No. 41-1445) (Kumar et al., 2019). Similarly, the identified feature planes of Ag3PO4, (210) and (211) exhibits a well-defined crystalline body-centred cubic structure with the space group
SEM imaging was used to analyse the topography of synthesised NCs (Figure 2). Figure 2A shows that the nanoparticles of Ag3PO4 are agglomerated and spherical shaped. The combined effect of different attractive-repulsive forces and some weak forces is responsible for the nanoparticle agglomeration. The formation of irregular shaped SnO2 NPs is illustrated in Figure 2B. In Figure 2C shows the SEM image of Ag3PO4/SnO2 nanocomposite featuring both spherical and irregularly shaped nanoparticles. This observation highlights the amalgamation of SnO2 and Ag3PO4 structures, culminating in the formation of a composite material. This may be advantageous for the facile transfer of charge carriers between Ag3PO4 and SnO2. The uniform distribution of Sn, Ag, P, and O elements is demonstrated through elemental mapping (Figure 2D).
Figure 2. SEM images of (a) Ag3PO4 NPs (b) SnO2 NPs (c) Ag3PO4/SnO2 NPs and (d) Elemental mapping of Ag3PO4/SnO2 nanocomposite.
TEM image of nanocomposite has been represented in Figure 3A. TEM image shows different types of nanoparticles ranging between 20–50 nm which are spherical as well as irregular in nature. The crystal lattice fringe spacings of 0.29, 0.24, and 0.17 nm corresponds to Ag3PO4 (200), Ag3PO4 (211), and SnO2 (211) planes, respectively (Figure 3B). Additionally, the selected area electron diffraction (SAED) pattern in Figure 3C, shows the highly crystalline structure of Ag3PO4 and SnO2.
Figure 3. (a) TEM image of Ag3PO4/SnO2 NPs (b) lattice fringes and (c) selected area electron diffraction pattern of Ag3PO4/SnO2 NPs.
X-ray photoelectron spectroscopy was utilized to examine the composition and the valence state of different elements in synthesized Ag3PO4/SnO2 nanocomposite. The XPS analysis of the sample (Figure 4A) displayed the presence of Sn, Ag and O elements in the synthesized nanocomposite. The spectrum was calibrated with reference to the C 1s peak.
Figure 4. (a) XPS survey spectrum of Ag3PO4/SnO2 nanocomposite; De-convoluted XPS core level spectra of (b) Ag 3d (c) Sn 3d and (d) O 1s.
The core level XPS spectrum of Ag 3d, Sn 3d and O 1s (Figure 4) was deconvoluted using gaussian function. Ag exhibits two characterstic peaks of binding energy approximately at 368.2 eV and 374.3 eV (Figure 4B) corrosponding to Ag 3d3/2 and Ag3d5/2. It confirms that Ag is present as Ag(I) (Li et al., 2019b; Chai et al., 2014; Li et al., 2019c). The XPS peak of Sn 3d (Figure 4C) was deconvoluted in two prominent peaks having binding energy of 487.2 eV and 495.6 eV, corresponding to the Sn 3d5/2 and Sn 3d3/2 states, respectively. This indicate that Sn is present as Sn+4 in the nanocomposite (Wang et al., 2019; Ma et al., 2019; Kamboj et al., 2022). Additionally, the deconvoluted peak of P is observed at 135 eV (Supplementary Figure S2) which is attributed to P 2p3/2. The peak around 532.5 eV is attributed to O 1s band (Figure 4D). These peaks confirms that P is present as P5+ (Li et al., 2019c) and O is present as O2- in the form of PO43- as well as in SnO2 lattice. Moreover, in the XPS spectra of Ag3PO4/SnO2, apart from Sn, Ag, O and P, no distinct impurity peaks were observed, which confirms the formation of pure Ag3PO4/SnO2 nanocomposite.
The Brunauer-Emmett-Teller is an important analytical method for calculating the average surface area of materials. BET characterization was performed using 80 mg Ag3PO4/SnO2 nanocomposite. nanoparticles.
Figure 5 shows that the adsorption-desorption isotherms for both the Ag3PO4/SnO2 nanocomposite and Ag3PO4 nanoparticles are of Type IV according to IUPAC classification (Thommes et al., 2015). The hysteresis loop for Ag3PO4/SnO2 is slightly wider than the hysteresis loop for Ag3PO4. This suggests that the pores in the Ag3PO4/SnO2 material are slightly more ink-bottle shaped (Thommes et al., 2015; Yamaguchi et al., 2020; Cychosz et al., 2017) which can absorb more light and thus results in higher photocatalytic activity. Moreover, the mean pore radius, total pore volume, and average surface area of the Ag3PO4/SnO2 nanocomposite was found to be 1.5261 nm, 0.0266 cm3/g, and 37.674 m2/g, respectively. The mean pore radius, total pore volume, and average surface area of the pure Ag3PO4 nanoparticles are 1.706 nm, 0.021 cm3/g, and 7.021 m2/g, respectively. Table 1 summarizes the compared values from BET analysis for Ag3PO4 and Ag3PO4/SnO2. Table 1 depicts that the average surface area of the Ag3PO4/SnO2 nanocomposite is almost 5.36 times higher than that of Ag3PO4 nanoparticles (Figure 5). This increase in surface area indicates that more surface-active sites are available in Ag3PO4/SnO2 nanocomposite than the pure Ag3PO4.
Figure 5. Adsorption-desorption isotherm of Ag3PO4/SnO2 nanocomposite and pure Ag3PO4 nanoparticles.
Using DRS, the optical property of the Ag3PO4/SnO2 nanocomposite was investigated to evaluate the effect of Ag3PO4/SnO2 heterojunction on light absorption activity. To determine the optical band gap energy, the linear fit of the graph between (F(R)hυ)2 and photon energy was extrapolated using Kubelka Munk function. The observed band gaps for pure Ag3PO4, SnO2 and Ag3PO4/SnO2 composite are 2.27 eV, 3.89 eV and 2.51 eV respectively, as shown in Figure 6A. The formation of a heterojunction in between Ag3PO4 and SnO2 changes the overall band gap and enhance the light absorption property resulting in high photocatalytic activity.
Figure 6. (A) Band gap of Ag3PO4 and Ag3PO4/SnO2, (inset) UV-DRS absorption spectrum of Ag3PO4 and Ag3PO4/SnO2 (B) FTIR spectrum of Ag3PO4 and Ag3PO4/SnO2.
FTIR spectrum was used to study the bonding characteristics of the nanocomposite (Figure 6B). The broad shoulder peak at 500 cm−1 corresponds to O–Sn–O stretching vibrations, and the peaks at 627 cm−1 is the characteristic of Sn–O bond stretching (Kumar et al., 2011). The discernible peak at 544 cm−1 signifies the asymmetric bending vibration associated with O=P–O bonds (Nagajyothi et al., 2019).The peak at 663 cm−1 indicate the P-O-P bond stretching (Mroczkowska et al., 2007). Additionally, a prominent peak observed at 949 cm-1 is attributed to the P=O stretching due to presence of PO43− ions (Nagajyothi et al., 2019). The peak at 1119 cm-1 represents the antisymmetric stretching of P-O bonds (Kumar et al., 2013). A pristine phase of Ag3PO4 exhibits characteristic vibrational modes at 929 cm⁻1 and 538 cm⁻1. Upon the formation of a nanostructured composite, these characteristic vibrational modes experience a minor blue shift or hypsochromic shift. This indicates that SnO2 changes the structure of Ag3PO4 and there is strong interaction between both phases (Pant et al., 2021; Saud et al., 2017). Based on the FTIR spectra, it can be deduced that the introduction of SnO2 does not significantly alter the fundamental composition of Ag3PO4. One hypothesis suggests that Sn+4 ions attach themselves to the negative end of the P-O bond which results in slight shift of the stretching frequency of P-O bonds in nanocomposite. Similar effect has also been observed in other composites of Ag3PO4 (Selim et al., 2023).
Ag3PO4/SnO2 NC was used to degrade the IPU solution under UV light irradiation (shown in Figure 7). To attain adsorption-desorption equilibrium, IPU solution is stirred with catalyst in dark for 30 min. Then the solution was irradiated with UV-Visible light for 120 min. Aliquots were collected at 20-min intervals and subjected to centrifugation to remove suspended nanoparticles before UV-visible spectroscopic analysis. Irradiation of IPU solution in absence of photocatalyst resulted in negligible degradation. This owes to the requirement of an efficient photocatalyst. Under UV light irradiation, the degradation efficiency of SnO2 NPs was about 25% and that of Ag3PO4 NPs was 41% with the variation of ± 2%–4%. Compared to them, the composite exhibited the degradation efficiency of 97% with an error bar of ±2%. The higher efficiency of the nanocomposite can be attributed to the larger average surface area and formation of heterojunction that inhibits the electron-hole pair recombination rate. Supplementary Table S2 depicts comparison of synthesized nanocomposite with nano-catalysts reported in literature which have been used for photocatalytic IPU degradation.
SnO2/Ag3PO4 photocatalyst showed high stability and recyclability. In terms of recycling the nanocomposite degraded 87% of IPU even after four consecutive cycles (Figure 8). Recyclability experiments demonstrate the potential of Ag3PO4/SnO2 photocatalyst to be an economical option for IPU degradation. PXRD pattern was taken after four consecutive cycles (Figure 9). It was observed to be unchanged which demonstrate high stability of the nanocomposite.
The degradation mechanism involves the reaction photogenerated holes with water molecules to generate hydroxyl radicals. The conduction band as well as valence band potentials of the SnO2 are higher than those of Ag3PO4 (Niu et al., 2010). As a result, photogenerated electrons in Ag3PO4 are easily transferred to the surface of SnO2, while photoinduced holes stay on the surface of Ag3PO4, as shown in Figure 13. The band structure of the Ag3PO4/SnO2 photocatalysts must be responsible for the higher photocatalytic activity than pure Ag3PO4. Furthermore, electronic acceptors such as adsorbed O2 may easily capture electrons (which was transferred onto the surface of SnO2) to generate a superoxide anion radical
Based on the foregoing, it is possible to infer that the suggested Ag3PO4/SnO2 fabrication is an effective and universal technique for developing highly stable and active photocatalysts under UV irradiation.
To explore the species responsible for degradation of IPU, trapping reagents were used. For this purpose, EDTA-Na2 (disodium-EDTA), terephthalic acid (TPA) and benzoquinone (BQ) were used for trapping h+, OH•, and
Figure 10. Effect of various trapping/sacrificial agents on degradation efficiency of the photocatalyst.
Figure 11. Photoluminescence spectrum of terepthalic acid under light irradiation in presence of Ag3PO4/SnO2.
Equations used for kinetic study have been provided in Supplementary Material. Langmuir Hinshelwood and pseudo first order kinetic model are two of the most common models used for photocatalytic degradation processes. Degradation of IPU was followed Langmuir Hinshelwood kinetics and Equation 1 is known as L-H model (Kumar et al., 2008). On integrating Equation 1, we get Equation 2 which represents another form of L-H model in which graph of
On the other hand, Equation 3 represents pseudo first order reaction. From literature it has been found that when substrate concentration is lower than 10–3 mol L-1, KCP << 1 and kap ∼ kdeg K = k1 indicating that at very low substrate concentration pseudo first order kinetics is followed (Tekin et al., 2019). In current work it was found that the nanocomposite follows Equation 3 with kap value of 0.02746 min-1. So the degradation rate follows pseudo first order kinetics (Figure 12).
The degradation mechanism involves the reaction of photogenerated holes with water molecules to generate hydroxyl radicals. The conduction band as well as valence band potentials of the SnO2 are higher than those of Ag3PO4 (Niu et al., 2010). As a result, photo-excited electrons in Ag3PO4 are easily transferred to the surface of SnO2, while photo-induced holes stay on the surface of Ag3PO4, as shown in Figure 13. The band structure of the Ag3PO4/SnO2 photocatalysts must be responsible for the higher photocatalytic activity than pure Ag3PO4. Furthermore, electronic acceptors such as adsorbed molecular O2 may easily capture electrons (which was transferred onto the surface of SnO2) to generate a superoxide anion radical
To investigate the degradation pathway of IPU, HRMS was performed to analyse the degradation products and intermediates. Supplementary Figures S4A, B shows the HRMS spectrum of sample before and after UV light irradiation for 120 min, respectively. Based on HRMS analysis, the possible products and the proposed degradation pathway have been shown in Figure 14. The results indicate that the degradation pathway of IPU include reactions such as hydroxylation, demethylation, deamination, oxidation, reduction and decarboxylation (Galichet et al., 2002; Khan et al., 2023; Sharma et al., 2008; Boucheloukh et al., 2017). Photogenerated h+, OH•, were found to be the main active species responsible for degradation of IPU. The photogenerated e‾ further produced
This work elucidates the successful synthesis, comprehensive characterization, and remarkable photocatalytic degradation of Isoproturon using Ag3PO4/SnO2 nanocomposite. The nanocomposite synthesis was validated using a variety of analytical techniques such as X-ray diffraction, FTIR, SEM, Elemental mapping, DRS and X-ray photoelectron spectroscopy. PXRD spectrum as well as XPS showed successful synthesis of the nanocomposite. DRS showed a decrease in overall band gap of the nanocomposite. Elemental mapping indicates a homogeneous distribution of Ag, P, Sn, and O elements, which further supports the structural integrity of the nanocomposite. The Ag3PO4/SnO2 nanocomposite showed better photocatalytic activity as compared to both pure Ag3PO4 & pure SnO2. The nanocomposite performed approximately 2.3 times greater than its precursor nanoparticles. Formation of heterojunction enhance the light harvesting ability of the nanocomposite and also enhance the charge separation of generated electron-hole pair. This result along with large average surface area of NC responsible in enhanced photocatalytic activity. The mechanistic insights highlight the promise of Ag3PO4/SnO2 nanocomposites for expanded environmental applications in pesticide clean-up, while also providing a deeper understanding of the enhanced photocatalytic activity. Thus, this study opens the door for the creation of effective and long-lasting remedies to the problem of pesticide pollution in the environment.
The original contributions presented in the study are included in the article/Supplementary Material, further inquiries can be directed to the corresponding authors.
RR: Writing–original draft, Data curation, Formal Analysis, Investigation, Software. Bhawna: Formal Analysis, Software, Writing–original draft, Validation. SK: Formal Analysis, Software, Writing–review and editing. AG: Conceptualization, Formal Analysis, Writing–review and editing. RK: Data curation, Formal Analysis, Resources, Writing–review and editing, Software. KD: Formal Analysis, Resources, Validation, Visualization, Writing–review and editing. VK: Writing–original draft, Conceptualization, Supervision.
The author(s) declare that no financial support was received for the research, authorship, and/or publication of this article.
Author Rishi Ram thanks Council of Scientific and Industrial Research for Junior Research Fellowship (NTA Ref. No.: 231610271964). Author wants to sincerely thank to special centre for nanoscience and advance instrumentation research facility for providing instrumentation and working space.
The authors declare that the research was conducted in the absence of any commercial or financial relationships that could be construed as a potential conflict of interest.
All claims expressed in this article are solely those of the authors and do not necessarily represent those of their affiliated organizations, or those of the publisher, the editors and the reviewers. Any product that may be evaluated in this article, or claim that may be made by its manufacturer, is not guaranteed or endorsed by the publisher.
The Supplementary Material for this article can be found online at: https://www.frontiersin.org/articles/10.3389/fbioe.2025.1458965/full#supplementary-material
Ahmed, S., Khan, F. S. A., Mubarak, N. M., Khalid, M., Tan, Y. H., Mazari, S. A., et al. (2021). Emerging pollutants and their removal using visible-light responsive photocatalysis – a comprehensive review. J. Environ. Chem. Eng. 9 (6), 106643. doi:10.1016/j.jece.2021.106643
Appleman, T. D., Dickenson, E. R. V., Bellona, C., and Higgins, C. P. (2013). Nanofiltration and granular activated carbon treatment of perfluoroalkyl acids. J. Hazard Mater 260, 740–746. doi:10.1016/j.jhazmat.2013.06.033
Bhawna, S. R., Kumar, S., Kumar, R., Sahu, P. K., Kumari, V., et al. (2023). Unlocking the potential of N-doped SnO2 for sustainable photocatalytic degradation of carcinogenic dyes. Separations 10 (6), 322. doi:10.3390/separations10060322
Boucheloukh, H., Remache, W., Parrino, F., Sehili, T., and Mechakra, H. (2017). The effect of natural iron oxide and oxalic acid on the photocatalytic degradation of isoproturon: a kinetics and analytical study. Photochem Photobiol. Sci. 16 (5), 759–765. doi:10.1039/c6pp00441e
Chai, B., Li, J., and Xu, Q. (2014). Reduced graphene oxide grafted Ag3Po4 composites with efficient photocatalytic activity under visible-light irradiation. Ind. Eng. Chem. Res. 53 (21), 8744–8752. doi:10.1021/ie4041065
Chen, X., Dai, Y., and Wang, X. (2015). Methods and mechanism for improvement of photocatalytic activity and stability of Ag3PO4: a review. J. Alloys Compd. 649, 910–932. doi:10.1016/j.jallcom.2015.07.174
Cychosz, K. A., Guillet-Nicolas, R., García-Martínez, J., and Thommes, M. (2017). Recent advances in the textural characterization of hierarchically structured nanoporous materials. Chem. Soc. Rev. 46 (2), 389–414. doi:10.1039/c6cs00391e
De, A., Bose, R., Kumar, A., and Mozumdar, S. (2014). Worldwide pesticide use. In: targeted delivery of pesticides using biodegradable polymeric nanoparticles. SpringerBriefs Mol. Sci., 99. doi:10.1007/978-81-322-1689-6_2
Dhenadhayalan, N., Chauhan, A., Lin, K.-C., and AlFantazi, A. (2022). Architecting 3D prism shaped carbon dots/germanium/germanium oxide nanohybrid for photocatalytic degradation of pendimethalin and dinotefuran pesticides. Mater Today Chem. 24, 100913. doi:10.1016/j.mtchem.2022.100913
El-Saeid, M. H., Alotaibi, M. O., Alshabanat, M., Alharbi, K., Altowyan, A. S., and Al-Anazy, M. (2021). Photo-catalytic remediation of pesticides in wastewater using uv/tio2. WaterSwitzerl. 13 (21), 3080–3111. doi:10.3390/w13213080
Galichet, F., Mailhot, G., Bonnemoy, F., Bohatier, J., and Bolte, M. (2002). Iron(III) photo-induced degradation of isoproturon: correlation between degradation and toxicity. Pest Manag. Sci. 58 (7), 707–712. doi:10.1002/ps.505
Hamilton, D. J., Ambrus, Á., Dieterle, R. M., Felsot, A. S., Harris, C. A., Holland, P. T., et al. (2003). Regulatory limits for pesticide residues in water (IUPAC Technical Report). Pure Appl. Chem. 75 (8), 1123–1155. doi:10.1351/pac200375081123
Kamboj, N., Debnath, B., Bhardwaj, S., Paul, T., Kumar, N., Ogale, S., et al. (2022). Ultrafine mix-phase SnO-SnO2Nanoparticles anchored on reduced graphene oxide boost reversible Li-ion storage capacity beyond theoretical limit. ACS Nano 16 (9), 15358–15368. doi:10.1021/acsnano.2c07008
Kamiuchi, N., Mitsui, T., Yamaguchi, N., Muroyama, H., Matsui, T., Kikuchi, R., et al. (2010). Activation of Pt/SnO2 catalyst for catalytic oxidation of volatile organic compounds. Catal. Today 157 (1–4), 415–419. doi:10.1016/j.cattod.2010.02.063
Khan, K., Khitab, F., Shah, J., and Jan, M. R. (2023). Ultrasound assisted photocatalytic degradation of isoproturon and triasulfuron herbicides using visible light driven impregnated zinc oxide catalysts. Sustain Environ. Res. 33 (1), 24. doi:10.1186/s42834-023-00184-9
Kumar, K. V., Porkodi, K., and Rocha, F. (2008). Langmuir-Hinshelwood kinetics - a theoretical study. Catal. Commun. 9 (1), 82–84. doi:10.1016/j.catcom.2007.05.019
Kumar, S., Bhawna, Y. S. K., Gupta, A., Kumar, R., Ahmed, J., et al. (2022). B-doped SnO2 nanoparticles: a new insight into the photocatalytic hydrogen generation by water splitting and degradation of dyes. Environ. Sci. Pollut. Res. 29 (31), 47448–47461. doi:10.1007/s11356-022-18946-0
Kumar, S., Surendar, T., Baruah, A., and Shanker, V. (2013). Synthesis of a novel and stable g-C3N4-Ag 3PO4 hybrid nanocomposite photocatalyst and study of the photocatalytic activity under visible light irradiation. J. Mater Chem. A 1 (17), 5333–5340. doi:10.1039/c3ta00186e
Kumar, V., Govind, A., and Nagarajan, R. (2011). Optical and photocatalytic properties of heavily F--doped SnO2 nanocrystals by a novel single-source precursor approach. Inorg. Chem. 50 (12), 5637–5645. doi:10.1021/ic2003436
Kumar, V., Yadav, S. K., Gupta, A., Dwivedi, B., Kumar, A., Singh, P., et al. (2019). Facile synthesis of Ce–doped SnO2 nanoparticles: a promising photocatalyst for hydrogen evolution and dyes degradation. ChemistrySelect 4 (13), 3722–3729. doi:10.1002/slct.201900032
Li, D., Wang, H., Tang, H., Yang, X., and Liu, Q. (2019c). Remarkable enhancement in solar oxygen evolution from MoSe2/Ag3PO4 heterojunction photocatalyst via in situ constructing interfacial contact. ACS Sustain Chem. Eng. 7 (9), 8466–8474. doi:10.1021/acssuschemeng.9b00252
Li, F., Zhang, G., and Song, Y. (2019a). Preparation and photocatalytic mechanism of Ag3PO4/SnO2 composite photocatalyst. Nano 14 (7), 1950092–1950099. doi:10.1142/s1793292019500929
Li, T., Wei, H., Jia, H., Xia, T., Guo, X., Wang, T., et al. (2019b). Mechanisms for highly efficient mineralization of bisphenol A by heterostructured Ag 2 WO 4/Ag 3 PO 4 under simulated solar light. ACS Sustain Chem. Eng. 7 (4), 4177–4185. doi:10.1021/acssuschemeng.8b05794
Li, Y., Niu, J., Yin, L., Wang, W., Bao, Y., Chen, J., et al. (2011). Photocatalytic degradation kinetics and mechanism of pentachlorophenol based on Superoxide radicals. J. Environ. Sci. 23 (11), 1911–1918. doi:10.1016/S1001-0742(10)60563-3
Liu, C. F., and Perng, T. P. (2020). Fabrication and band structure of Ag3PO4–TiO2 heterojunction with enhanced photocatalytic hydrogen evolution. Int. J. Hydrogen Energy 45 (1), 149–159. doi:10.1016/j.ijhydene.2019.10.182
Liu, M., Wang, G., Xu, P., Zhu, Y., and Li, W. (2020). Construction of Ag3PO4/SnO2 heterojunction on carbon cloth with enhanced visible light photocatalytic degradation. Appl. Sci. 10 (9), 3238. doi:10.3390/app10093238
Lu, J., Wang, Y., Liu, F., Zhang, L., and Chai, S. (2017). Fabrication of a direct Z-scheme type WO 3/Ag 3 PO 4 composite photocatalyst with enhanced visible-light photocatalytic performances. Appl. Surf. Sci. 393, 180–190. doi:10.1016/j.apsusc.2016.10.003
Ma, J., Fan, H., Ren, X., Wang, C., Tian, H., Dong, G., et al. (2019). A simple absorbent cotton biotemplate to fabricate SnO2 porous microtubules and their gas-sensing properties for chlorine. ACS Sustain Chem. Eng. 7 (1), 147–155. doi:10.1021/acssuschemeng.8b02235
Magara, Y., Itoh, M., and Morioka, T. (1995). Application of ozone to water treatment and power consumption of ozone generating systems. Prog. Nucl. energy 29, 175–182. doi:10.1016/0149-1970(95)00041-h
Malik, M. A., Ghaffar, A., and Malik, S. A. (2001). Water purification by electrical discharges. Plasma sources Sci. Technol. 10 (1), 82–91. doi:10.1088/0963-0252/10/1/311
Mroczkowska, M., Nowinski, J. L., Zukowska, G. Z., Mroczkowska, A., Garbarczyk, J. E., Wasiucionek, M., et al. (2007). Micro Raman, FT-IR/PAS, XRD and SEM studies on glassy and partly crystalline silver phosphate ionic conductors. J. Power Sources 173 (2 SPEC. ISS.), 729–733. doi:10.1016/j.jpowsour.2007.05.048
Nagajyothi, P. C., Sreekanth, T. V. M., Ramaraghavulu, R., Devarayapalli, K. C., Yoo, K., Vattikuti, S. V. P., et al. (2019). Photocatalytic dye degradation and hydrogen production activity of Ag3PO4/g-C3N4 nanocatalyst. J. Mater Sci. Mater Electron 30 (16), 14890–14901. doi:10.1007/s10854-019-01860-6
Niu, M., Huang, F., Cui, L., Huang, P., Yu, Y., and Wang, Y. (2010). Hydrothermal synthesis, structural characteristics, and enhanced photocatalysis of SnO2/α-Fe2O3 semiconductor nanoheterostructures. ACS Nano. 4 (2), 681–688. doi:10.1021/nn901119a
Pant, B., Prasad, O. G., Acharya, J., and Park, M. (2021). Ag3PO4-TiO2-Carbon nanofiber Composite: an efficient Visible-light photocatalyst obtained from electrospinning and hydrothermal methods. Sep. Purif. Technol. 276 (April), 119400. doi:10.1016/j.seppur.2021.119400
Qi, Y. L., Han, G., and Song, X. C. (2018). Enhanced photocatalytic degradation of phenol over Ag3PO4-BiOCl1−xBrx composites. Mater Res. Bull. 102 (2010), 16–23. doi:10.1016/j.materresbull.2018.02.019
Saud, P. S., Pant, B., Ojha, P., Kim, D., Kuk, Y., Park, S., et al. (2017). One-pot synthesis of Ag3PO4/MoS2 nanocomposite with highly efficient photocatalytic activity. Biochem. Pharmacol. 5 (6), 5521–5527. doi:10.1016/j.jece.2017.10.040
Selim, H., Sheha, E. R., Elshypany, R., Raynaud, P., El-Maghrabi, H. H., and Nada, A. A. (2023). Superior photocatalytic activity of BaO@Ag3PO4 nanocomposite for dual function degradation of methylene blue and hydrogen production under visible light irradiation. Catalysts 13 (2), 363. doi:10.3390/catal13020363
Sen, S. K., Barman, U. C., Manir, M. S., Mondal, P., Dutta, S., Paul, M., et al. (2020). X-ray peak profile analysis of pure and Dy-doped α-MoO3 nanobelts using Debye-Scherrer, Williamson-Hall and Halder-Wagner methods. Adv. Nat. Sci. Nanosci. Nanotechnol. 11 (2), 025004. doi:10.1088/2043-6254/ab8732
Sharma, A., Kumar, V., Shahzad, B., Tanveer, M., Sidhu, G. P. S., Handa, N., et al. (2019). Worldwide pesticide usage and its impacts on ecosystem. SN Appl. Sci. 1 (11), 1446–1516. doi:10.1007/s42452-019-1485-1
Sharma, M. V. P., Durgakumari, V., and Subrahmanyam, M. (2008). Solar photocatalytic degradation of isoproturon over TiO2/H-MOR composite systems. J. Hazard Mater 160 (2–3), 568–575. doi:10.1016/j.jhazmat.2008.03.042
Silva, G. N., Martins, T. A., Nogueira, I. C., Santos, R. k., Li, M. S., Longo, E., et al. (2021). Synthesis of Ag3PO4/SnO2 composite photocatalyst for improvements in photocatalytic activity under visible light. Mater Sci. Semicond. Process 135 (December 2020), 106064. doi:10.1016/j.mssp.2021.106064
Simonsen, M. E. (2014). Heterogeneous photocatalysis. Chem. Adv. Environ. Purif. Process Water Fundam. Appl., 135–170. doi:10.1016/b978-0-444-53178-0.00004-3
Spliid, N. H., and Køppen, B. (1998). Occurrence of pesticides in Danish shallow ground water. Chemosphere 37 (7), 1307–1316. doi:10.1016/s0045-6535(98)00128-3
Tekin, D., Tekin, T., and Kiziltas, H. (2019). Photocatalytic degradation kinetics of Orange G dye over ZnO and Ag/ZnO thin film catalysts. Sci. Rep. 9 (1), 17544–17547. doi:10.1038/s41598-019-54142-w
Thommes, M., Kaneko, K., Neimark, A. V., Olivier, J. P., Rodriguez-Reinoso, F., Rouquerol, J., et al. (2015). Physisorption of gases, with special reference to the evaluation of surface area and pore size distribution (IUPAC Technical Report). Pure Appl. Chem. 87 (9–10), 1051–1069. doi:10.1515/pac-2014-1117
Verma, A., Prakash, N. T., and Toor, A. P. (2014). An efficient TiO2 coated immobilized system for the degradation studies of herbicide isoproturon: durability studies. Chemosphere 109, 7–13. doi:10.1016/j.chemosphere.2014.02.051
von Gunten, U. (2003). Ozonation of drinking water: Part II. Disinfection and by-product formation in presence of bromide, iodide or chlorine. Water Res. 37 (7), 1469–1487. doi:10.1016/s0043-1354(02)00458-x
Von Sonntag, C., and Von Gunten, U. (2012). Chemistry of ozone in water and wastewater treatment. London, United Kingdom: IWA publishing.
Wang, Z., Gao, S., Fei, T., Liu, S., and Zhang, T. (2019). Construction of ZnO/SnO2 heterostructure on reduced graphene oxide for enhanced nitrogen dioxide sensitive performances at room temperature. ACS Sensors 4 (8), 2048–2057. doi:10.1021/acssensors.9b00648
We, Z., Zho, Q., Wang, J., Lu, Z., Xu, L., and Zeng, W. (2019). Hydrothermal synthesis of sno2 nanoneedle-anchored nio microsphere and its gas sensing performances. Nanomaterials 9 (7), 1015. doi:10.3390/nano9071015
Wen, X. J., Niu, C. G., Zhang, L., and Zeng, G. M. (2017). Fabrication of SnO2 nanopaticles/BiOI n-p heterostructure for wider spectrum visible-light photocatalytic degradation of antibiotic oxytetracycline hydrochloride. ACS Sustain Chem. Eng. 5 (6), 5134–5147. doi:10.1021/acssuschemeng.7b00501
Yadav, I. C., Devi, N. L., Syed, J. H., Cheng, Z., Li, J., Zhang, G., et al. (2015). Current status of persistent organic pesticides residues in air, water, and soil, and their possible effect on neighboring countries: a comprehensive review of India. Sci. Total Environ. 511, 123–137. doi:10.1016/j.scitotenv.2014.12.041
Yamaguchi, Y., Aono, R., Hayashi, E., Kamata, K., and Hara, M. (2020). Template-free synthesis of mesoporous β-MnO2 nanoparticles: structure, formation mechanism, and catalytic properties. ACS Appl. Mater Interfaces 12 (32), 36004–36013. doi:10.1021/acsami.0c08043
Yeoh, J. X., Jamil Sna, Md., Syukri, F., Koyama, M., and Nourouzi Mobarekeh, M. (2022). Comparison between conventional treatment processes and advanced oxidation processes in treating slaughterhouse wastewater: a review. Water 14 (22), 3778. doi:10.3390/w14223778
Yu, Y., Yao, B., He, Y., Cao, B., Ren, Y., and Sun, Q. (2020). Piezo-enhanced photodegradation of organic pollutants on Ag3PO4/ZnO nanowires using visible light and ultrasonic. Appl. Surf. Sci. 528 (March), 146819. doi:10.1016/j.apsusc.2020.146819
Zeghioud, H., Nguyen-Tri, P., Khezami, L., Amrane, A., and Assadi, A. A. (2020). Review on discharge Plasma for water treatment: mechanism, reactor geometries, active species and combined processes. J. Water Process Eng. 38 (September), 101664. doi:10.1016/j.jwpe.2020.101664
Zhang, L., Zhang, H., Huang, H., Liu, Y., and Kang, Z. (2012). Ag3PO4/SnO2 semiconductor nanocomposites with enhanced photocatalytic activity and stability. New J. Chem. 36 (8), 1541–1544. doi:10.1039/c2nj40206h
Zheng, C., Yang, H., Cui, Z., Zhang, H., and Wang, X. (2017). A novel Bi4Ti3O12/Ag3PO4 heterojunction photocatalyst with enhanced photocatalytic performance. Nanoscale Res. Lett. 12, 608–612. doi:10.1186/s11671-017-2377-1
Keywords: photocatalysis, nanoparticles, photochemistry, heterojunction, pesticide
Citation: Ram R, Bhawna , Kumar S, Gupta A, Kumar R, Dubey KK and Kumar V (2025) Synergistic heterojunction effects in Ag3PO4/SnO2 nanocomposites: a photocatalytic study on isoproturon degradation. Front. Bioeng. Biotechnol. 13:1458965. doi: 10.3389/fbioe.2025.1458965
Received: 03 July 2024; Accepted: 31 January 2025;
Published: 04 April 2025.
Edited by:
Gianni Ciofani, Italian Institute of Technology (IIT), ItalyReviewed by:
Arshid Mahmood Ali, King Abdulaziz University, Saudi ArabiaCopyright © 2025 Ram, Bhawna, Kumar, Gupta, Kumar, Dubey and Kumar. This is an open-access article distributed under the terms of the Creative Commons Attribution License (CC BY). The use, distribution or reproduction in other forums is permitted, provided the original author(s) and the copyright owner(s) are credited and that the original publication in this journal is cited, in accordance with accepted academic practice. No use, distribution or reproduction is permitted which does not comply with these terms.
*Correspondence: Vinod Kumar, dmt1bWFyMkBjaGVtaXN0cnkuZHUuYWMuaW4=, dmlub2Q3Njc0QGdtYWlsLmNvbQ==; Ravinder Kumar, cmF2aW5kZXIua3VtYXJAZ2t2LmFjLmlu
Disclaimer: All claims expressed in this article are solely those of the authors and do not necessarily represent those of their affiliated organizations, or those of the publisher, the editors and the reviewers. Any product that may be evaluated in this article or claim that may be made by its manufacturer is not guaranteed or endorsed by the publisher.
Research integrity at Frontiers
Learn more about the work of our research integrity team to safeguard the quality of each article we publish.