- 1Scientific Research Department, Inner Mongolia Fourth Hospital (Chest Hospital), Hohhot, China
- 2The Department of Infectious Diseases, Inner Mongolia Fourth Hospital (Chest Hospital), Hohhot, China
Piezoelectric materials, as a class of materials capable of generating electrical charges under mechanical vibration, have special piezoelectric effects and have been widely applied in various disease treatment fields. People generate vibrations in the oral cavity during daily activities such as brushing teeth, using electric toothbrushes, chewing, and speaking. These natural vibrations (or external ultrasound) provide ideal conditions for activating piezoelectric materials, leading to their high potential applications in protecting oral health and treating oral diseases. Based on this, this review reports on the research progress and trends of piezoelectric materials in the protection of oral health and the treatment of oral diseases in the past 5 years, and discusses its treatment mechanism, challenges and shortcomings, aiming to provide theoretical basis and new ideas for the future application of piezoelectric materials in the field of oral cavity. Finally, a brief outlook is provided, suggesting that the potential of piezoelectric materials may enable them to quickly move towards real clinical applications.
1 Introduction
Oral health is significant for people’s daily physiological activities (WHO, 2022). More and more people are suffering from oral health problems and oral diseases due to poor personal dietary and hygiene habits (Baker et al., 2024). Oral diseases have become a public health issue, seriously affecting people’s physiological and health functions (WHO, 2022). In particular, some oral diseases may also lead to systemic disease risks (Botelho et al., 2022), such as cardiovascular disease (Tonelli et al., 2023) and diabetes (Li Y. et al., 2024). In addition, oral infections and the growth of bacteria in the mouth may also be transmitted through saliva, increasing the risk of infectious diseases (Jiang et al., 2021). Therefore, how to maintain oral health and achieve efficient treatment of oral diseases has become an urgent challenge in the global public health field.
Currently, many dental materials have been applied clinically to maintain oral health and treat oral diseases (Jia et al., 2024). However, due to the complex oral environment and the presence of a large number of oral microorganisms, many dental materials fail to perform as intended (Jia et al., 2024). For example, dental resin materials are easily degraded by enzymes from saliva and bacteria, as well as acid produced by bacteria (Montoya et al., 2021a). To address the aforementioned oral issues, an increasing number of functional biomaterials are applied in the treatment of oral diseases (Ding et al., 2024; Wei et al., 2024a; Wang Y. et al., 2024). Among them, piezoelectric materials, due to their good biocompatibility and biosafety, as well as their unique properties compared to other biomaterials, can generate charge separation under mechanical stress or ultrasound, which can stimulate the production of reactive oxygen species in the environment and other special functions (electrical stimulation effect, improvement of cell function, anti-inflammatory effect, acceleration of tissue repair and regeneration, etc.), have become a new emerging material with strong application potential in the treatment of oral diseases (Wang et al., 2020; Roldan et al., 2023; Liu X. et al., 2024; Chernova et al., 2024).
In the past 5 years, a large number of review articles have been published on piezoelectric materials as a type of biomaterial with special functions, detailing their preparation process, mechanism, and applications in biomedical fields such as tumor treatment and tissue repair and regeneration (Wang J. et al., 2024; Dai et al., 2024; Zheng et al., 2024). Wang et al. review the basic principles and applications of piezoelectric materials in tumor therapy (Wang Y. et al., 2023). Nain et al. discuss the role of piezoelectric materials in tissue remodeling (Nain et al., 2024). However, to our knowledge, there is currently no comprehensive systematic review solely dedicated to the application of piezoelectric materials in the field of oral. Existing reviews mainly focus on the applications of piezoelectric materials in other biomedical fields, with only a small portion briefly mentioning their potential application of oral therapy (Nain et al., 2024; Chen S. et al., 2023; Wang L. et al., 2023). Here, this review will not specifically discuss aspects such as the preparation methods of piezoelectric materials that have already been extensively covered in other reviews.
In this review, we aim to focus on the latest research progress of emerging piezoelectric materials in protecting oral health and treating oral diseases. Firstly, the definition, classification and working mechanism of piezoelectric materials are briefly introduced. Then, the application of piezoelectric materials in protecting oral health and treating different oral diseases is mainly summarized and discussed (Figure 1). Meanwhile, a detailed discussion is also conducted on the treatment mechanism by which piezoelectric materials play a role, and the shortcomings are analyzed. Finally, the major challenges and future prospects facing the current applications of piezoelectric materials in the field of oral are discussed and proposed, aiming to promote the development of this emerging material in oral and provide insights and inspiration for its future clinical applications.
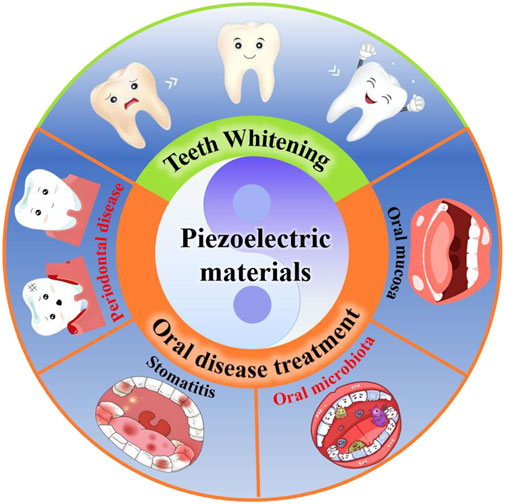
Figure 1. Schematic diagram of piezoelectric materials in protecting oral health and treating oral diseases.
2 Piezoelectric materials
Piezoelectric materials are a type of functional material that can convert mechanical stress into electrical signals, as well as electrical signals into mechanical stress (Xu et al., 2021; Wang X. et al., 2023). The former is known as the piezoelectric effect and was first discovered by the Curie brothers in 1880 (Kapat et al., 2020; Chen S. et al., 2024). Without mechanical strain, the surface of the piezoelectric material does not show polarization, with the centers of positive and negative charges coinciding. When subjected to external force along a certain direction, causing deformation by compression or stretching, the internal polarization will occur, generating an electric current. Meanwhile, positive and negative charges will migrate and appear on the relative surfaces of the material, thus generating a piezoelectric potential and realizing the transformation of mechanical energy into electrical energy. The latter is also called inverse piezoelectric effect (Kapat et al., 2020). When the electric field is applied to the polarization direction of the piezoelectric material, the material will undergo mechanical deformation. When the electric field is removed, the deformation of the material will disappear, so as to realize the transformation from electrical energy to mechanical energy. These characteristics and good biocompatibility of piezoelectric materials lead to their wide application in biomedicine.
2.1 Classification
Piezoelectric materials can be roughly divided into the following four categories according to their chemical composition and structure:
(1) Inorganic piezoelectric materials. Inorganic piezoelectric materials mainly include piezoelectric crystals and piezoelectric ceramics (Xu et al., 2023). Single crystals with piezoelectric properties are called piezoelectric crystals. These crystals have a central asymmetric structure, so they have piezoelectric properties, such as quartz crystals. Due to the poor piezoelectric properties and the difficulty of preparation, the research of piezoelectric crystal in biomedical field is relatively less.
Ceramics with piezoelectric properties are called piezoelectric ceramics (Wei et al., 2024b). Compared with piezoelectric single crystal, piezoelectric ceramics have better piezoelectric properties, and have the characteristics of simple preparation and easy modification. In particular, their piezoelectric properties can be improved by morphology control, structure control, doping, defect engineering and other means, which leads to their very good application potential in the biomedical field (Wu et al., 2023). Among them, barium titanate (BaTiO3), zinc oxide and other piezoelectric ceramics are widely used in a variety of biomedical treatment fields (Wu et al., 2023). However, inorganic piezoelectric materials also have obvious disadvantages, such as relatively poor biocompatibility, difficult degradation and poor plasticity.
(2) Organic piezoelectric materials. Organic piezoelectric materials, also known as piezoelectric polymers, are a class of piezoelectric materials composed of organic polymers (Smith and Kar-Narayan, 2022). Polyvinylidene fluoride is the most widely used piezoelectric polymer (Mokhtari et al., 2021). In addition, polylactic acid (PLA) and its derivatives have good piezoelectric properties and have been certified and approved by the U.S. Food and Drug Administration (FDA), which may make it the first piezoelectric material to be used in clinical treatment (Zhang S. et al., 2024). Compared with inorganic piezoelectric materials, organic piezoelectric materials have better biocompatibility, flexibility and plasticity, which is convenient for subsequent processing and application, but it also has a certain disadvantage that the piezoelectric properties are relatively weak.
(3) Composite piezoelectric materials. Inorganic piezoelectric materials have relatively high dielectric constant and good piezoelectric properties, but they have high physical stiffness and poor plasticity. Although organic piezoelectric materials have certain flexibility, they are limited by low dielectric constant and poor piezoelectric properties. In view of this, researchers have developed composite piezoelectric materials, which are mainly composed of inorganic piezoelectric materials and organic piezoelectric materials, aiming to obtain piezoelectric materials with excellent comprehensive properties such as piezoelectric properties, mechanical properties and biocompatibility through composite methods (Dai et al., 2022; Dong et al., 2024; Kabakov et al., 2023). Composite piezoelectric materials can overcome the shortcomings of organic-inorganic piezoelectric materials, but it also increases the preparation process and the difficulty of preparation. Especially for the composite ratio, the wrong ratio may lead to worse piezoelectric properties.
(4) Natural piezoelectric materials. Natural piezoelectric materials refer to some proteins, tissues and bone components with piezoelectric properties from organisms (Kim et al., 2020; Bai et al., 2024). Compared with the above three synthetic piezoelectric materials, natural piezoelectric materials have the highest biological safety, but their piezoelectric properties are relatively weak, and the extraction and synthesis process is relatively complex, which seriously limits their application in the biomedical field.
2.2 Working mechanisms
The basis for the role of piezoelectric materials in the biomedical field is their piezoelectric effect. When piezoelectric materials undergo piezoelectric effect under the action of external mechanical force, the positive and negative charges inside the piezoelectric material separate and migrate to the surface of the material, resulting in the formation of induced potential. The contact of charged particles on the surface of piezoelectric materials with different substances results in two different working mechanisms.
(1) Electrical stimulation. When the charged particles on the surface of piezoelectric materials directly contact with biological tissues and cells, they often produce certain electrical stimulation to tissues and cells, which can well promote cell proliferation and tissue regeneration (Wu et al., 2023; Das et al., 2024). Compared with the traditional electrical stimulation therapy, piezoelectric materials get rid of the shackles of external power supply and electrode, which makes it have great application prospects in the biomedical field, especially in the field of tissue regeneration.
(2) Piezoelectric catalysis. When the charged particles on the surface of piezoelectric materials react with the surrounding medium, especially the redox reaction, reactive oxygen species (ROS) will be generated, which is called piezoelectric catalysis (Chen S. et al., 2023; Chen W. et al., 2024). The ROS produced by this piezoelectric catalytic process will cause certain damage to chemical substances, cells, tissues and bacteria, which makes it show very good application potential in decontamination, tumor treatment and antibacterial fields. At present, there are still controversies about the mechanism of piezoelectric catalysis, but the energy band theory and the screening charge effect have been recognized by many scholars. Wang et al. have discussed these two mechanisms of piezoelectric catalysis in detail, providing some guidance for people to understand the mechanism of piezoelectric catalysis (Wang K. et al., 2022).
The movement of the oral cavity itself (chewing and other behaviors), daily brushing, and the direct application of ultrasound to the affected area of oral diseases are all external mechanical forces generated and received by the oral cavity in daily life. These daily external mechanical forces provide natural conditions for piezoelectric materials to excite their piezoelectric effects. When the piezoelectric effect occurs, the piezoelectric material will have the function of piezoelectric catalysis (ROS) or electrical stimulation, which makes it have great application potential in the field of maintaining oral health and treating oral diseases (Figure 2).
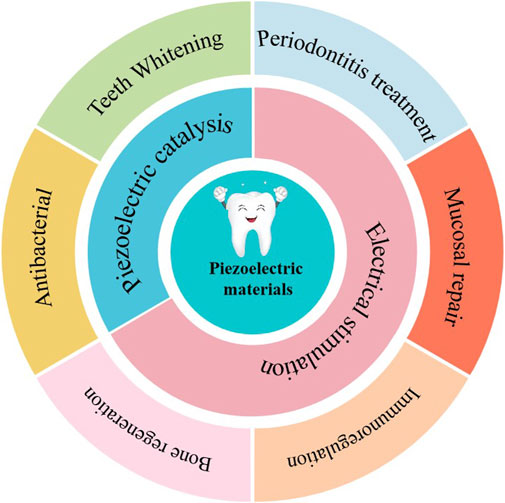
Figure 2. Schematic diagram of working mechanism of piezoelectric materials in maintaining oral health and treating oral diseases.
3 Teeth whitening
Brightening teeth can not only bring about the appearance of improvement, but also improve people’s self-confidence and reflect oral health problems (Goettems et al., 2021; Wang Y. et al., 2022). Common teeth whitening methods include enamel polishing, crown replacement, and tooth bleaching (Wang et al., 2020). Among them, tooth bleaching is the most widely used and effective technique, mainly achieved through the decomposition of stains on the surface of teeth by free radicals released by high concentrations of peroxides for whitening (Wang et al., 2020). However, high concentrations of hydrogen peroxide can lead to oral sensitivity, gum irritation, and enamel damage (Wang et al., 2020; Zhang et al., 2022; Kim et al., 2022). Therefore, developing a mild method of releasing ROS is a better way to achieve tooth whitening. The piezoelectric materials can generate ROS under the excitation of vibration and friction, demonstrating great potential in the field of tooth whitening. The application and mechanism of piezoelectric materials in tooth whitening is shown in Table 1.
Wang et al. (2020) report a non-destructive, harmless, and convenient tooth whitening strategy based on piezoelectric catalytic effect . Using polarized piezoelectric BaTiO3 to replace abrasives in toothpaste, combine with electric toothbrush vibration, can effectively remove staining compounds and achieve teeth whitening (Figures 3A, B). Meanwhile, they also found that BaTiO3 has lower cytotoxicity compared to commonly use hydrogen peroxide whitening agents (Figure 3C) and does not damage the surface of teeth. However, in order to fully utilize the piezoelectric catalytic whitening ability of BaTiO3, specialized equipment is needed to add the electric polarization step, which significantly affects its use. Meanwhile, the piezoelectric catalytic ability of a single BaTiO3 is also very weak. To further enhance the piezoelectric catalytic capability for better teeth whitening effects, different approaches have been proposed. Sharma et al. develop a NaNbO3/ZnO heterojunction piezoelectric catalyst for teeth whitening, showing reaction rates 8.5 times higher than ZnO and 1.7 times higher than NaNbO3 (Sharma et al., 2022). He et al. combine photocatalysis and piezoelectric catalysis for teeth whitening by designing Z-scheme g-C3N4-x/Bi2O3-y heterostructures (Figure 3D) (He et al., 2023). This design enhances internal charge separation, significantly boosting its piezo-photocatalytic efficiency and achieving degradation rates approximately four times than piezoelectric catalysis alone and 2.6 times than photocatalysis alone for typical food colorants.
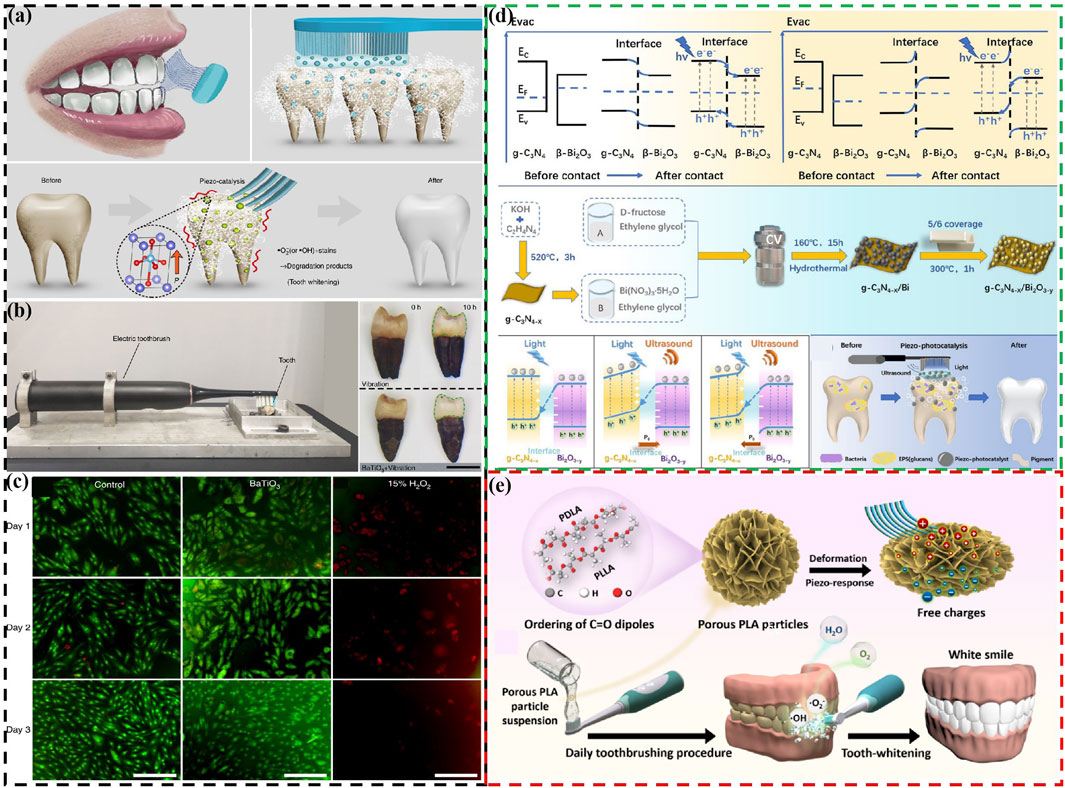
Figure 3. (A) Schematic diagram of using piezoelectric materials instead of toothpaste abrasives to achieve tooth whitening (B) The whitening effect of piezoelectric materials on teeth. (C) Cell morphology of different samples cultured for different days (Wang et al., 2020). Copyright 2020, Springer Nature. (D) Schematic energy band diagrams, fabrication procedure, the direct Z-scheme charge transfer mechanism of Z-scheme g-C3N4-x/Bi2O3-y heterostructures, and simplified schematic for piezo-photocatalytic treatment of tooth whitening and biofilm eradication using Z-scheme g-C3N4-x/Bi2O3-y heterostructures (He et al., 2023). Copyright 2023, Royal Society of Chemistry. (E) Schematic diagram of biodegradable polylactic acid particles used for piezoelectric catalytic teeth whitening (Deng et al., 2023). Copyright 2023, American Chemical Society.
Although there is extensive research on the use of inorganic piezoelectric materials for teeth whitening, the safety of certain ions such as Ba, Nb, and Bi in these materials still requires further investigation. Specifically, using these inorganic piezoelectric materials directly as abrasives in toothpaste may pose biosecurity concerns as they could enter the human body through the oral cavity. Based on this, relatively safe biodegradable piezoelectric materials are also used in teeth whitening. Deng et al. develop biodegradable PLA particles for teeth whitening (Figure 3E) (Deng et al., 2023). Furthermore, researchers have also used piezoelectric materials as fillers or coatings, such as for toothbrush bristles, to achieve teeth whitening (Ma et al., 2024). This prevents piezoelectric materials from entering the human body as toothpaste abrasives.
4 Oral disease treatment
Under the mechanical forces exerted during daily oral activities such as chewing, speaking, and brushing teeth, piezoelectric materials can undergo mechanical deformation effectively, thereby activating their piezoelectric effect to achieve the goal of treating oral diseases. Table 2 summarizes the application and mechanism of piezoelectric materials in maintaining oral health and treating oral diseases.
4.1 Antibacterial
The oral microbiota includes a variety of bacteria, fungi, and viruses, which form a complex ecosystem in the oral environment and interact with the host to positively or negatively impact oral health (Lu et al., 2019). When oral microorganisms proliferate excessively, they can form dental plaque and calculus, leading to the occurrence and development of oral diseases such as gingivitis and periodontitis (Sedghi et al., 2021). Especially, some studies have shown that oral microbiota is also associated with other systemic diseases (Peng et al., 2022). Therefore, effective oral sterilization can not only remove dental plaque and tartar, reduce the number and types of bacteria, but also reduce the risk of oral inflammation and maintain the health of teeth and gums.
Piezoelectric materials are used in the field of oral antimicrobials in two main ways: one approach involves direct use of piezoelectric materials, similar to toothpaste grinding agent, for oral antimicrobial (Sharma et al., 2022; He et al., 2023). Sharma et al. also directly apply NaNbO3/ZnO piezoelectric material used for tooth whitening to antibacterial applications (Sharma et al., 2022), but only briefly studied its antibacterial ability against Escherichia coli, lacking in-depth research, especially its antibacterial performance against major pathogenic bacteria in the oral cavity. In response to this, He et al. apply Z-scheme g-C3N4-x/Bi2O3-y heterostructures with piezoelectric photocatalytic ability to oral antibacterial applications (He et al., 2023). It could not only directly kill planktonic S. mutans (Streptococcus mutans) (Figure 4A) (Antibacterial rate: 63%), but also kill S. mutans embedded in the biofilm formed by S. mutans (Antibacterial rate: 48.2%). In addition, the antibacterial mechanism of the g-C3N4-x/Bi2O3-y is also proposed, mainly by stimulating the production of ROS in the environment, leading to bacterial death. Meanwhile, due to the presence of positive charges on the surface of g-C3N4-x/Bi2O3-y, they can better adsorb negatively charged S. mutans, thereby enhancing their piezoelectric photocatalytic ability and resulting in high antibacterial performance.
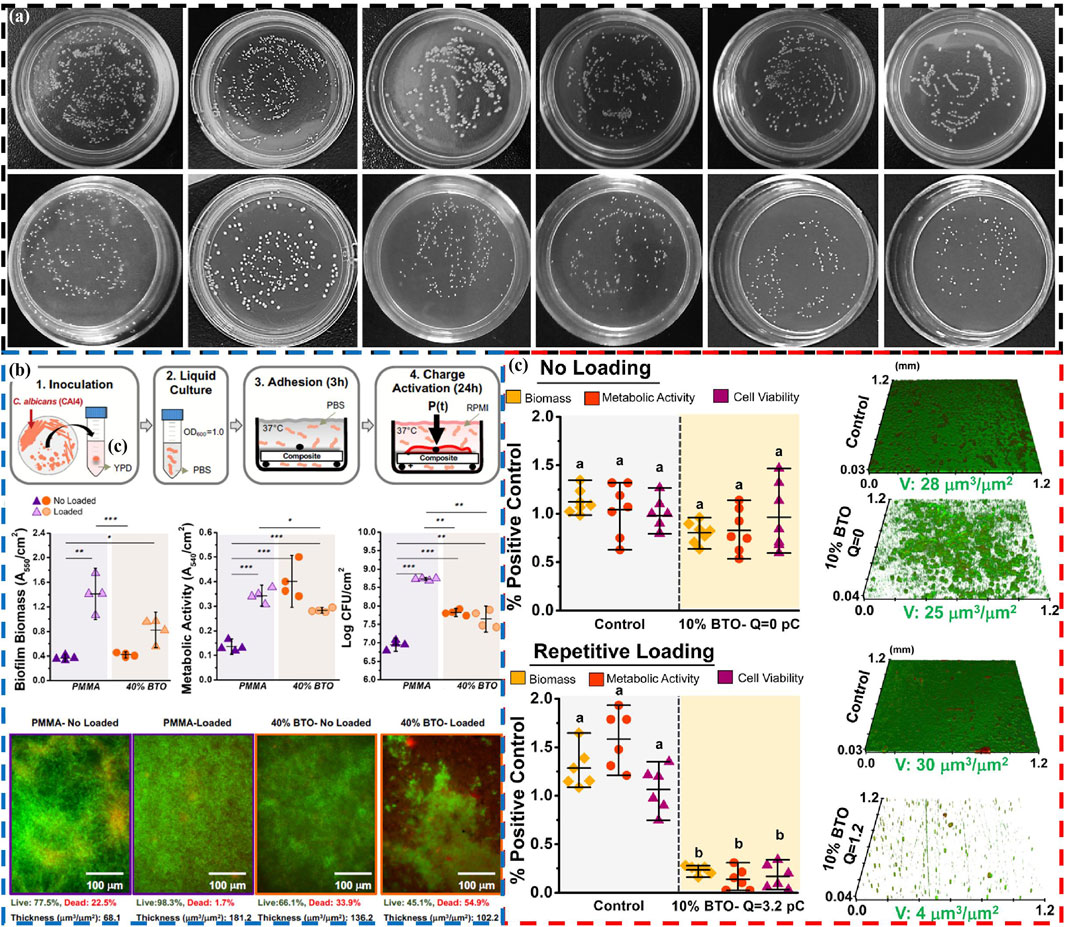
Figure 4. (A) Antibacterial activity of Z-scheme g-C3N4-x/Bi2O3-y heterostructures: Photos of planktonic bacterial colonies and bacterial colonies from disrupted biofilms under different conditions and materials (He et al., 2023). Copyright 2023, Royal Society of Chemistry. (B) The antifungal effect of piezoelectric PMMA dentures: Schematic of the biofilm model, microbiological evaluations (biofilm biomass, metabolic activity and cell viability), and fluorescence microscopy z-stack images of C. albicans biofilms (Montoya et al., 2021b). Copyright 2021, American Chemical Society. (C) Antibacterial activity of dental piezoelectric resin composites: Biofilm biomass, metabolic activity, cell viability, and CLSM images of S. mutans biofilms under no loading and repetitive loading (Montoya et al., 2021a). Copyright 2021, American Chemical Society.
Another approach targets the susceptibility of dental implants to bacterial infection-induced damage (Zhou et al., 2024; Jayasree et al., 2024), using piezoelectric materials as fillers or coatings on dental implants to achieve antimicrobial effects. Wei et al. provide a new strategy to enhance the antibacterial effect (90.41%) of implants using biocompatible BaTiO3/chitosan multiporous surface piezoelectric coating (Wei et al., 2024c). The main reasons for this high antibacterial effect are as follows: On the one hand, the generation of ROS under ultrasonic stimulation in piezoelectric coating kills bacteria. On the other hand, the contact potential difference of bacteria by surface charges on piezoelectric coating leads to the production of ROS inside the bacteria, which also kills bacteria. Meanwhile, the coating promotes increased deposition of hydroxyapatite and adhesion of plasma albumin, facilitated by its abundant positive charges and numerous pores on the surface. In addition, Pan et al. design a surface ultrasonic response coating of Al3+ ion doped strontium titanate/titanium dioxide nanotubes (Al-SrTiO3/TiO2 nanotubes) for antibacterial properties of dental implants (Pan et al., 2024). The introduction of Al3+ ions induces oxygen vacancies, destroys the lattice of SrTiO3, and enables Al-SrTiO3/TiO2 nanotubes to produce more ROS, thereby achieving efficient antibacterial (P. gingivalis: 80.4% and F. nucleatum: 82.1%) and inhibiting the growth of biofilms. In particular, the presence of Sr2+ ions in SrTiO3 can effectively promote osteogenic activity and facilitate the formation of rigid bone fusion between the implant surface and alveolar bone. The multifunctionality of piezoelectric materials increase through ion doping or the addition of functional ions has important guiding significance for the application of piezoelectric materials in the oral field.
In addition to the aforementioned primary applications, piezoelectric materials are also used as fillers for antibacterial purposes in dentures (Montoya et al., 2021b), dental composite restorative materials (Montoya et al., 2021a), and orthodontic appliances (Shi et al., 2023). Montoya et al. demonstrate for the first time that piezoelectric nanoparticles of BaTiO3 can serve as fillers for dentures, achieving antifungal effects (Biofilm volume decreased by 43%) (Figure 4B) (Montoya et al., 2021b). The main reason for achieving antifungal effects may be the interaction (surface charge to induce the production of ROS) between the surface charges of BaTiO3 and fungi, leading to fungal death. The research team also apply BaTiO3 nanoparticles as fillers to dental composite repair materials (Montoya et al., 2021a). The BaTiO3 can not only impart antibacterial (up to 90%) to composite materials (Figure 4C), but also promote the remineralization of dental tissues. Under the stimulation of mechanical external forces, a piezoelectric charge is generated on the surface of the composite material, which interacts with bacteria through electrostatic interactions, leading to bacterial repulsion, preventing further adhesion, and ultimately inhibiting the growth of biofilms. Meanwhile, these charges also promote the production of ROS in cells, thereby killing bacteria. And its mineralization mechanism is also due to the presence of surface charges of piezoelectric materials promoting the nucleation of calcium phosphate, leading to its re-mineralization. Here, piezoelectric materials may also have other functions such as promoting tooth tissue remineralization during antibacterial processes, which also needs further development and utilization.
4.2 Treatment of periodontitis
Periodontitis (PD) is a local chronic inflammatory disease of periodontal tissue caused by pathogenic microorganisms (Mi et al., 2024). Under the continuous stimulation of bacteria and their metabolites, periodontal tissue undergoes inflammatory reactions and immune regulation imbalances, ultimately leading to structural damage of periodontal tissue such as alveolar bone loss (Huang et al., 2020). In the pathogenesis of PD, in addition to pathogenic bacteria, host immune response is also a key mediator of periodontal damage (Lai et al., 2023; Yang et al., 2021).
Current treatment strategies mainly focus on reducing bacterial adhesion or killing bacteria (Mei et al., 2024), as well as regulating the host immunity to inhibit the progression of chronic inflammation (Zheng et al., 2023; Peng et al., 2024). For alveolar bone resorption, a prominent feature of periodontal disease, the treatment plan is to promote bone regeneration to achieve the repair of missing bone (Luan et al., 2023; Song et al., 2024; Dong et al., 2023). Based on this, Roldan et al. develop an injectable piezoelectric hydrogel (PiezoGEL), comprising methacryloyl gelatin (GelMA) and biocompatible BaTiO3 piezoelectric filler (BTO), which generates charges in response to biomechanical vibrations like chewing and movement (Roldan et al., 2023). This new type of PiezoGEL exhibits good biological activity, which can promote bone tissue regeneration (Figures 5A, B) and achieve high antibacterial effects (Figure 5C). PiezoGEL significantly reduce pathogenic biofilm biomass (∼41%), metabolic activity (∼75%), and viable cell count (∼2-3 log) compared to BTO-free hydrogels in vitro. The antibacterial mechanism is mainly due to the surface charge of BTO and the generation of ROS. Molecular analysis of the antibacterial effect attributes it to decreased cell adhesion (downregulation of porP and fimA) and increased oxidative stress (upregulation of oxyR). The primary mechanism of bone tissue regeneration is attributed to electrical stimulation generated by the surface charge of BTO, which promotes differentiation and proliferation of bone marrow stem cells (BMSCs) and facilitates the formation of new bone tissue (upregulating RUNX2, COL1A1, and ALP) (Figure 5D). Additionally, these charges also stimulate necessary vascularization for bone regeneration. Especially, in vivo experiments have shown that PiezoGEL can effectively reduce periodontal inflammation and increase bone tissue regeneration, thus having good application prospects in the treatment of periodontal disease.
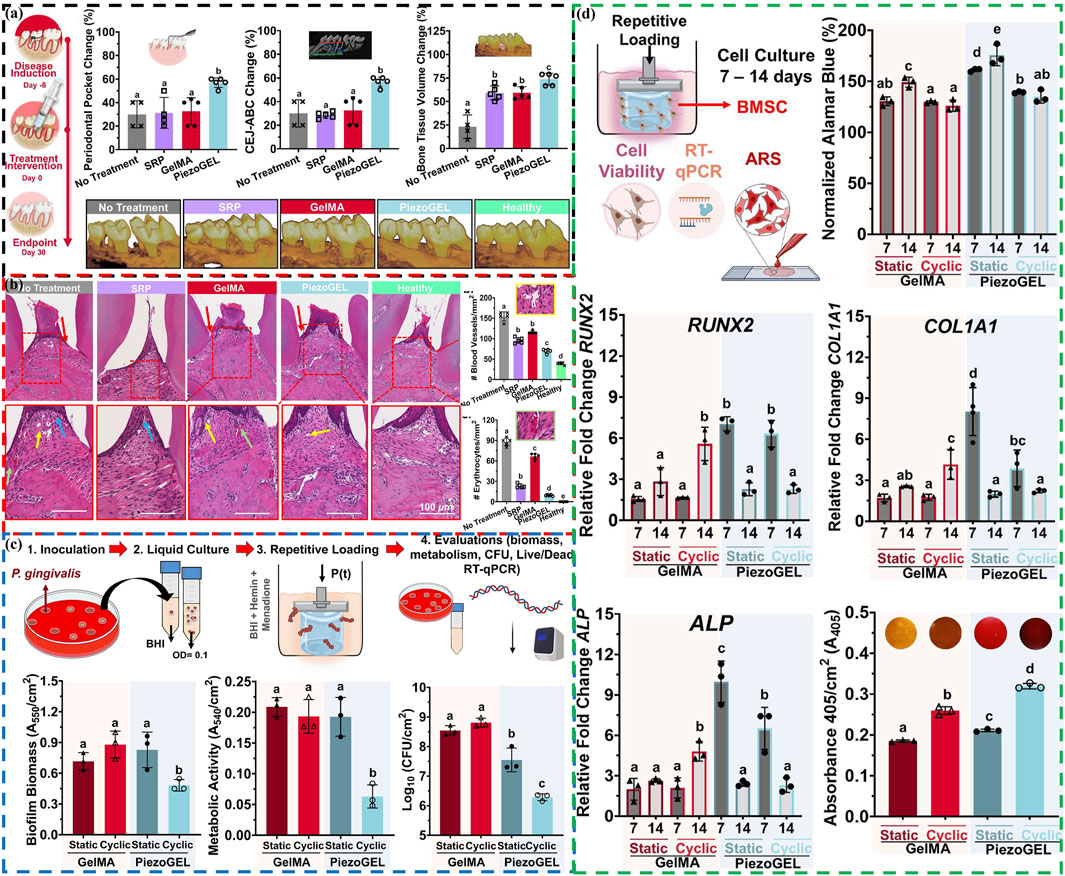
Figure 5. (A) Periodontal bone tissue regeneration evaluation: Schematic representation of the ligature-induced model, changes of periodontal pocket depth, cementum-enamel junction to alveolar bone crest distance and bone volume, and reconstructed 3D micro-CT images of the maxillary alveolar bone. (B) Histological evaluation: H&E images of periodontal tissue sections, number of blood vessels and erythrocytes quantified in the regenerated area. (C) Antibacterial evaluation of PiezoGEL hydrogels in vitro: Schematics of the model, biofilm biomass, metabolic activity, and cell viability. (D) Osteogenic differentiation evaluation of PiezoGEL hydrogels in vitro: Schematics of the model, changes of Cell viability (RUNX2, COL1A1, ALP and ECM minerals) (Roldan et al., 2023). Copyright 2023, American Chemical Society.
The impaired differentiation ability of resident cells and the disruption of immune microenvironment in PD seriously affect the regeneration of alveolar bone (Li et al., 2022; Li et al., 2023). Research has found that electrical stimulation can reduce inflammation, regulate macrophage polarization and promote bone regeneration by improving mitochondrial function and inducing more adenosine triphosphate (ATP) synthesis (Luo et al., 2024; Hollenberg et al., 2021). Inspired by this, Liu et al. develop a wireless piezoelectric stimulation system using a piezoelectric hydrogel composed of tetragonal BaTiO3 nanoparticles (t-BTO NPs) and tilapia fish gelatin hydrogel to activate bioenergetics for injured tissue regeneration in PD (Figure 6A) (Liu X. et al., 2024). Under mechanical activation, the triggered piezoelectric potential induces osteogenic differentiation of inflammatory periodontal ligament stem cells (PDLSCs) by regulating energy metabolism and enhancing ATP synthesis. Additionally, under the synergistic action of piezoelectric stimulation and the intrinsic anti-inflammatory activity of the hydrogel, macrophage polarization is shifted from the pro-inflammatory M1 phenotype to the anti-inflammatory M2 phenotype, promoting osteogenesis (Figure 6B). This ultimately achieve in situ tissue regeneration of rat periodontitis bone defects (Figure 6C), paving new pathways for treating PD and other immune-related bone defects through piezoelectric stimulation to regulate energy metabolism and immune modulation.
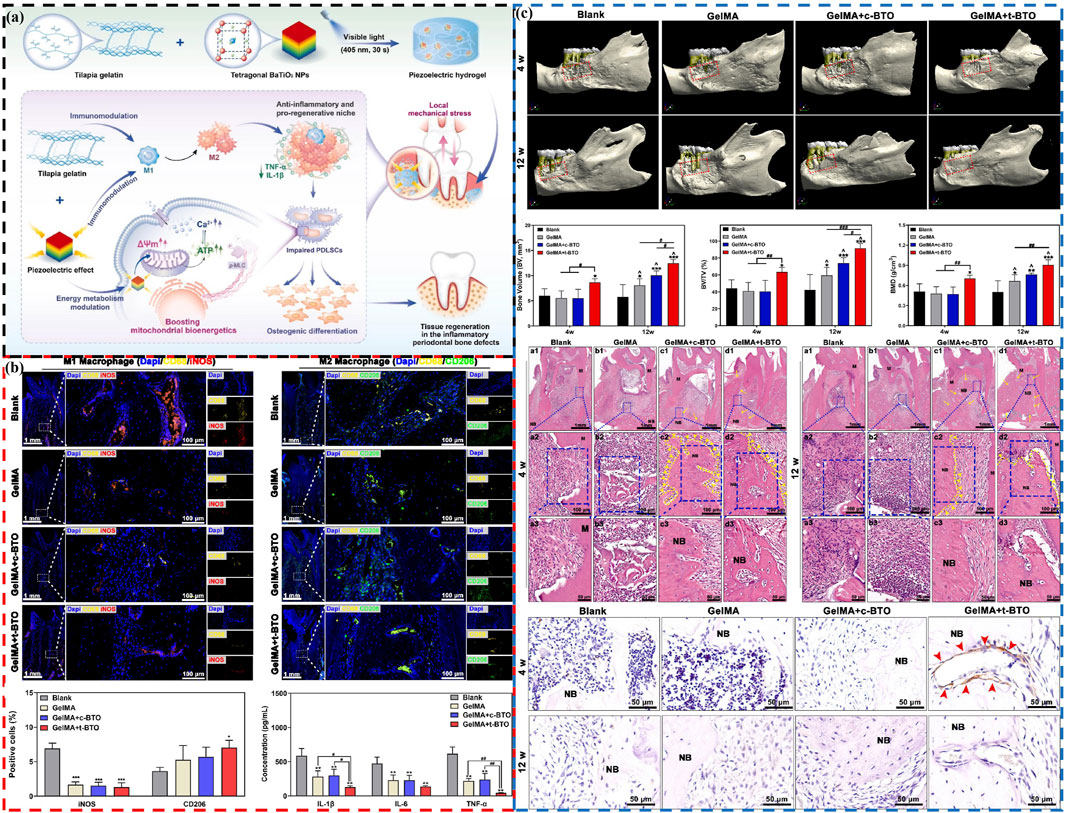
Figure 6. The piezoelectric hydrogel’s role in promoting osteogenesis and immunomodulation in PD by activating bioenergetics. (A) Schematic diagram of piezoelectric hydrogel preparation and treatment of PD. (B) Piezoelectric stimulation modulates M1/M2 polarization of macrophages: Representative fluorescence images and positive percentage of M1 and M2 macrophages, and salivary inflammatory factors after 12 weeks (C) The piezoelectric hydrogel enhances bone regeneration in periodontal defects associated with PD: Micro-CT 3D construction images, new bone volume, bone volume/total volume fraction, bone mineral density, H&E staining images and p-MLC immunohistochemistry images at 4 weeks and 12 weeks (Liu X. et al., 2024). Copyright 2024, Elsevier.
In addition, for the repair and regeneration of dentin tissue, Li et al. design a P(VDF-TrFE) piezoelectric film with 2 wt% SrCl2 addition (Li J. et al., 2024). Piezoelectric film creates an electric microenvironment conducive to recruiting dental pulp stem cells (DPSCs) and guiding their differentiation into odontoblasts during everyday activities like chewing and speaking. Moreover, the gradual release of Sr2+ ions from the film promotes the odonto-differentiation of DPSCs. The mutual assistance of electrical stimulation and Sr2+ ions enables the regeneration of dentin tissue, providing a new treatment approach for the repair of damaged teeth such as alveolar bone loss.
4.3 Oral mucosal regeneration
Oral mucosa not only serves as a protective barrier inside the mouth, effectively preventing bacteria and viruses from invading deep tissues, but also maintains comfort and normal function in the mouth through sensory functions (Moutsopoulos and Konkel, 2018; Zhao et al., 2022). Therefore, wound healing of the oral mucosa is an important measure to prevent oral diseases and promote oral health.
Piezoelectric materials can promote cell growth and repair due to their unique electrical stimulation effects, especially during wound healing, where appropriate electrical stimulation can accelerate tissue repair and regeneration (Dai et al., 2024; Liu Q. et al., 2024; Ren et al., 2024). Chernova et al. conduct a comparative study on two different types of polymeric membranes in promoting wound healing processes in oral mucosa: dielectric poly(tetrafluoroethylene) (PTFE) membrane and piezoelectric membrane of vinylidene fluoride and tetrafluoroethylene (VDF-TeFE) (Chernova et al., 2024). Comparative experiments have shown that piezoelectric VDF-TeFE membranes have high oral mucosal regeneration ability (Figure 7). Badaraev et al. also confirm that piezoelectric polymer membranes (copolymer of vinylidene fluoride with tetrafluoroethylene) can be used for oral mucosal regeneration (Badaraev et al., 2020), especially by coating them with antibacterial Cu2+ ion coatings, which can further enhance their oral mucosal regeneration ability. These indicate that piezoelectric materials have broad application prospects in oral mucosal repair.
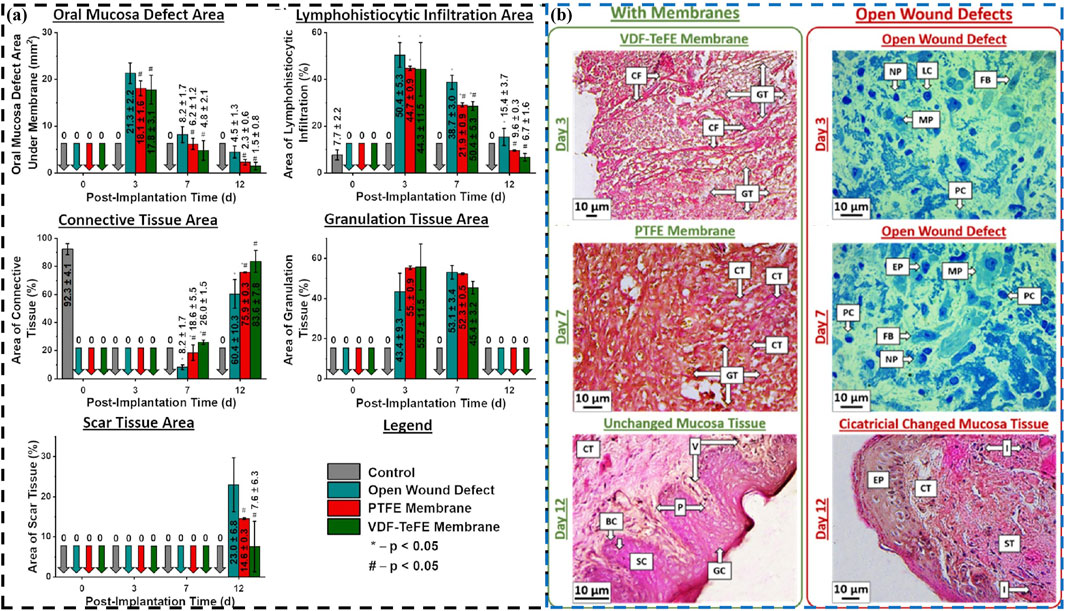
Figure 7. (A) The wound defect healing of PTFE (red) and VDF-TeFE (green): Area of the oral mucosa defect under the membranes, and specific area of lymphohistiocytic infiltration, connective tissue, granulation tissue and scar tissue. (B) Histological examination of the oral mucosa: Changes in wound defect areas (connective tissue fibers, cellular infiltration, granulation tissue, mucosa and cicatricial) under different films at different times (day 3, day 7 and day 12) (Chernova et al., 2024). Copyright 2024, American Chemical Society.
5 Treatment mechanism
According to the role of the above piezoelectric materials in the treatment of oral diseases and the working mechanism of piezoelectric materials, the treatment mechanism (Table 2) is as follows:
(1) The mechanism of piezoelectric materials in tooth whitening is mainly to generate charges on the surface under mechanical force stimulation, which reacts with the external environment, generates ROS (Piezoelectric catalysis), and realizes the degradation of tooth surface staining and stains, achieving the whitening effect (Wang et al., 2020; Deng et al., 2023).
(2) The inhibitory mechanism of piezoelectric materials on oral microbiota is similar to that of teeth whitening, mainly relying on the reaction (Piezoelectric catalysis) of piezoelectric materials with surrounding media under external mechanical forces such as ultrasound to generate ROS (He et al., 2023; Wei et al., 2024c; Pan et al., 2024). The ROS can cause bacterial death through pathways such as oxidative stress, lipid peroxidation, protein dysfunction, and DNA damage. However, another antibacterial property of piezoelectric materials has also been proposed, which is the charge generated on the surface of the piezoelectric material, which generates a certain stimulation on cells, leading to the production of ROS inside bacteria and ultimately causing bacterial death (Montoya et al., 2021a; Wei et al., 2024c). In addition, the surface charge of piezoelectric materials may also interact with the surface charge of bacteria, leading to bacterial death or inhibiting the formation of bacterial biofilms (Montoya et al., 2021a). Therefore, further research is needed on the antibacterial mechanism of piezoelectric materials to find their biological mechanisms.
(3) In the treatment of PD, one mechanism is that piezoelectric materials can inhibit bacteria to treat PD, and its treatment mechanism is antibacterial mechanism (Roldan et al., 2023). Another mechanism is to generate electric charges on the surface of piezoelectric materials, which can produce electrical stimulation (Roldan et al., 2023; Liu X. et al., 2024). Under the action of electrical stimulation, it can improve mitochondrial function and induce more ATP synthesis to alleviate inflammatory response, promote osteoblast differentiation, regulate macrophage polarization to control the immune microenvironment, and ultimately achieve regeneration and repair of damaged dental bone tissues.
(4) The mechanism of oral mucosal repair is basically consistent with the mechanism of treating PD, mainly through the electrical stimulation effect of piezoelectric materials (Chernova et al., 2024; Badaraev et al., 2020). Under the action of electrical stimulation, it improves the function of intracellular mitochondria, increases energy production of cells (such as ATP synthesis), and promotes the improvement of cell metabolism and repair ability. In addition, reducing local inflammatory response and controlling the release of inflammatory mediators under electrical stimulation can help reduce pain and discomfort during wound healing process.
Based on the above analysis, the fundamental role of piezoelectric materials in treating oral diseases lies in their ability to generate electric charges and fields when subjected to mechanical force or pressure. It is precisely this electrical stimulation that facilitates the treatment of oral diseases. However, further in-depth research is needed on the detailed effects and mechanisms of electrical stimulation on the internal cells or tissues of living organisms, which has certain guiding significance for the application of piezoelectric materials. In addition, it can also be found that antibacterial is the basis and auxiliary for the treatment of many oral diseases, and the addition of antibacterial process can increase the therapeutic effect on other oral diseases.
6 Shortcomings and improvements
Piezoelectric materials have shown great potential in the treatment of oral diseases, but there are still shortcomings that need improvement. The specific details are as follows:
(1) The bottleneck limiting the application of piezoelectric materials is that their piezoelectric performance is too weak. Chemical preparation methods such as ion doping (Pan et al., 2024; Wang X. et al., 2022; Chen T. et al., 2023) and heterojunction (Sharma et al., 2022; He et al., 2023) can improve piezoelectric performance or develop new piezoelectric materials with high voltage capability (Zhang H. et al., 2024).
(2) At present, the application of piezoelectric materials in oral treatment is too limited, which is a typical acoustic dynamic therapy (Roldan et al., 2023; Chernova et al., 2024). The single treatment method may affect its effectiveness, and it can be combined with other treatment methods such as photothermal, photoelectric, and drug methods to achieve multifunctional treatment of oral diseases and improve efficacy.
(3) The mechanism of piezoelectric materials in tooth whitening has been basically understood (Wang et al., 2020), but the mechanism of their effects in the treatment of other oral diseases is not clear and and controversial (Montoya et al., 2021a; Wei et al., 2024c; Montoya et al., 2021b). The latest biological methods such as proteomics and metabolomics can be used to further study and explore their deep therapeutic mechanisms.
(4) Piezoelectric materials have been widely studied in tumor treatment and have shown good therapeutic effects (Zheng et al., 2024; Zhang L. et al., 2024; Hao et al., 2024). However, there is currently no application of piezoelectric materials in the treatment of oral cancer, which is a new direction for the future application of piezoelectric materials in the field of oral treatment.
(5) The biological safety and metabolic process of piezoelectric materials entering the body are the real problems that need to be solved, and are the prerequisites for their clinical application.In particular, the metal ions contained in some inorganic piezoelectric materials may have specific toxicity to the human body (Bhasin et al., 2019). Therefore, exploring the toxicological research of piezoelectric materials is of utmost importance.
7 Conclusion and perspective
This review comprehensively summarizes and classifies the research on piezoelectric materials in protecting oral health and treating oral diseases in the past 5 years. Among them, representative studies are discussed in detail and compared, and their underlying therapeutic mechanisms and innovation points are explored. Then, each mechanism of the application of piezoelectric materials in the field of oral cavity is discussed and analyzed in detail, which provides a new treatment idea and a new method for the treatment of oral diseases by piezoelectric materials in the future. Finally, the current deficiencies of piezoelectric materials in the field of oral therapy are analyzed, and improvement measures are proposed to provide solid support for the real clinical application of piezoelectric materials.
Although the application of piezoelectric materials in this field is still in the research and development stage, their high-performance characteristics and multi-functional application potential provide a new prospect for personalized treatment and precision medicine for oral health in the future. With the continuous advancement of technology and in-depth exploration of theories, it is believed that the application of piezoelectric materials in the field of oral disease treatment will become more and more extensive, bringing people a healthier and more comfortable oral care experience.
Author contributions
TY: Writing–review and editing, Writing–original draft, Methodology, Investigation, Data curation, Conceptualization. RS: Writing–original draft, Investigation, Formal Analysis, Writing–review and editing, Funding acquisition. FW: Supervision, Investigation, Formal Analysis, Writing–review and editing. CC: Writing–review and editing, Methodology, Investigation, Formal Analysis. LZ: Writing–review and editing, Resources, Project administration, Funding acquisition, Conceptualization.
Funding
The author(s) declare that financial support was received for the research, authorship, and/or publication of this article. We gratefully acknowledge support of this work by the Natural Science Foundation of Inner Mongolia (Grant No. 2023LHMS08035), the 2023 High-level Clinical Specialty Construction Science and Technology Project of Public Hospitals in the Capital Region, Health Commission of the Autonomous Region (Grant No. 2023SGGZ147), and the 2024 High-level Clinical Specialty Construction Science and Technology Project of Public Hospitals in the Capital Region, Health Commission of the Autonomous Region (Grant No. 2024SGGZ061).
Conflict of interest
The authors declare that the research was conducted in the absence of any commercial or financial relationships that could be construed as a potential conflict of interest.
Publisher’s note
All claims expressed in this article are solely those of the authors and do not necessarily represent those of their affiliated organizations, or those of the publisher, the editors and the reviewers. Any product that may be evaluated in this article, or claim that may be made by its manufacturer, is not guaranteed or endorsed by the publisher.
References
Badaraev, A. D., Koniaeva, A., Krikova, S. A., Shesterikov, E. V., Bolbasov, E. N., Nemoykina, A. L., et al. (2020). Piezoelectric polymer membranes with thin antibacterial coating for the regeneration of oral mucosa. Appl. Surf. Sci. 504, 144068. doi:10.1016/j.apsusc.2019.144068
Bai, Y., Meng, H., Li, Z., and Wang, Z. L. (2024). Degradable piezoelectric biomaterials for medical applications. MedMat 1 (1), 40–49. doi:10.1097/mm9.0000000000000002
Baker, J. L., Welch, J. L. M., Kauffman, K. M., McLean, J. S., and He, X. (2024). The oral microbiome: diversity, biogeography and human health. Nat. Rev. Microbiol. 22, 89–104. doi:10.1038/s41579-023-00963-6
Bhasin, A. K. K., Chauhan, P., and Chaudhary, S. (2019). A novel sulfur-incorporated naphthoquinone as a selective “turn-on” fluorescence chemical sensor for rapid detection of Ba2+ ion in semi-aqueous medium. Sens. Actuator. B Chem. 294, 116–122. doi:10.1016/j.snb.2019.04.098
Botelho, J., Mascarenhas, P., Viana, J., Proença, L., Orlandi, M., Leira, Y., et al. (2022). An umbrella review of the evidence linking oral health and systemic noncommunicable diseases. Nat. Commun. 13, 7614. doi:10.1038/s41467-022-35337-8
Chen, S., Tong, X., Huo, Y., Liu, S., Yin, Y., Tan, M., et al. (2024a). Piezoelectric biomaterials inspired by nature for applications in biomedicine and nanotechnology. Adv. Mater. 36, 2406192. doi:10.1002/adma.202406192
Chen, S., Zhu, P., Mao, L., Wu, W., Lin, H., Xu, D., et al. (2023a). Piezocatalytic medicine: an emerging frontier using piezoelectric materials for biomedical applications. Adv. Mater. 35, 2208256. doi:10.1002/adma.202208256
Chen, T., Zhu, Z., Chen, F., Hu, C., and Huang, H. (2023b). Low-valence B-site cation substitution triggering polarization and oxygen vacancy enhancement for elevating piezocatalytic activity on Bi2MoO6. Appl. Surf. Sci. 616, 156467. doi:10.1016/j.apsusc.2023.156467
Chen, W., Chen, Q., Song, F., Hua, M., Chang, M., Feng, W., et al. (2024b). Engineering heterostructured piezoelectric nanorods with rich oxygen vacancy-mediated piezoelectricity for ultrasound-triggered piezocatalytic cancer therapy. Adv. Funct. Mater., 2405929. doi:10.1002/adfm.202405929
Chernova, U. V., Varakuta, E.Yu., Koniaeva, A. D., Leyman, A. E., Sagdullaeva, S. A., Plotnikov, E., et al. (2024). Piezoelectric and dielectric electrospun fluoropolymer membranes for oral mucosa regeneration: a comparative study. ACS Appl. Mater. Interfaces 16, 20245–20259. doi:10.1021/acsami.4c01867
Dai, J., Shao, J., Zhang, Y., Hang, R., Yao, X., Bai, L., et al. (2024). Piezoelectric dressings for advanced wound healing. J. Mater. Chem. B 12, 1973–1990. doi:10.1039/d3tb02492j
Dai, X., Yao, X., Zhang, W., Cui, H., Ren, Y., Deng, J., et al. (2022). The osteogenic role of barium titanate/polylactic acid piezoelectric composite membranes as guiding membranes for bone tissue regeneration. Int. J. Nanomed. 17, 4339–4353. doi:10.2147/ijn.s378422
Das, K. K., Basu, B., Maiti, P., and Dubey, A. K. (2024). Interplay of piezoelectricity and electrical stimulation in tissue engineering and regenerative medicine. Appl. Mater. Today 39, 102332. doi:10.1016/j.apmt.2024.102332
Deng, S., Zhang, Y., Qiao, Z., Wang, K., Ye, L., Xu, Y., et al. (2023). Hierarchically designed biodegradable polylactide particles with unprecedented piezocatalytic activity and biosafety for tooth whitening. Biomacromolecules 24, 797–806. doi:10.1021/acs.biomac.2c01252
Ding, Y., Zhu, Z., Zhang, X., and Wang, J. (2024). Novel functional dressing materials for intraoral wound care. Adv. Healthc. Mater., 2400912. doi:10.1002/adhm.202400912
Dong, H., Zhu, Z., Li, Z., Li, M., and Chen, J. (2024). Piezoelectric composites: state-of-the-art and future prospects. JOM 76, 340–352. doi:10.1007/s11837-023-06202-w
Dong, Z., Lin, Y., Xu, S., Chang, L., Zhao, X., Mei, X., et al. (2023). NIR-triggered tea polyphenol-modified gold nanoparticles-loaded hydrogel treats periodontitis by inhibiting bacteria and inducing bone regeneration. Mater, Des. 225, 111487. doi:10.1016/j.matdes.2022.111487
Goettems, M. L., Fernandez, M. d.S., Donassollo, T. A., Donassollo, S. H., and Demarco, F. F. (2021). Impact of tooth bleaching on oral health-related quality of life in adults: a triple-blind randomised clinical trial. J. Dent. 105, 103564. doi:10.1016/j.jdent.2020.103564
Hao, Z., Guo, S., Tu, W., Wang, Q., Wang, J., Zhang, X., et al. (2024). Piezoelectric catalysis induces tumor cell senescence to boost chemo-immunotherapy. Small 20, 2309487. doi:10.1002/smll.202309487
He, J., Cui, S., Hou, Y., Liu, S., Zhang, Z., Zhao, M., et al. (2023). Bifunctional defect mediated direct Z-scheme g-C3N4-x/Bi2O3-y heterostructures with enhanced piezo-photocatalytic properties for efficient tooth whitening and biofilm eradication. J. Mater. Chem. B 11, 7103–7116. doi:10.1039/d3tb01044a
Hollenberg, A. M., Huber, A., Smith, C. O., and Eliseev, R. A. (2021). Electromagnetic stimulation increases mitochondrial function in osteogenic cells and promotes bone fracture repair. Sci. Rep. 11 (1), 19114. doi:10.1038/s41598-021-98625-1
Huang, X., Xie, M., Xie, Y., Mei, F., Lu, X., Li, X., et al. (2020). The roles of osteocytes in alveolar bone destruction in periodontitis. J. Transl. Med. 479, 18. doi:10.1186/s12967-020-02664-7
Jayasree, A., Cartmell, S., Ivanovski, S., and Gulati, K. (2024). Electrically stimulated dental implants triggers soft-tissue integration and bactericidal functions. Adv. Funct. Mater. 34, 2311027. doi:10.1002/adfm.202311027
Jia, B., Zhang, B., Li, J., Qin, J., Huang, Y., Huang, M., et al. (2024). Emerging polymeric materials for treatment of oral diseases: design strategy towards a unique oral environment. Chem. Soc. Rev. 53, 3273–3301. doi:10.1039/d3cs01039b
Jiang, W., Deng, Z., Dai, X., and Zhao, W. (2021). PANoptosis: a new insight into oral infectious diseases. Front. Immunol. 12, 789610. doi:10.3389/fimmu.2021.789610
Kabakov, P., Kim, T., Cheng, Z., Jiang, X., and Zhang, S. (2023). The versatility of piezoelectric composites. Annu. Rev. Mater. Res. 53, 165–193. doi:10.1146/annurev-matsci-080921-092839
Kapat, K., Shubhra, Q. T. H., Zhou, M., and Leeuwenburgh, S. (2020). Piezoelectric nano-biomaterials for biomedicine and tissue regeneration. Adv. Funct. Mater. 30, 1909045. doi:10.1002/adfm.201909045
Kim, D., Bae, J., Heo, J. H., Park, C. H., Kim, E. B., and Lee, J. H. (2022). Nanoparticles as next-generation tooth-whitening agents: progress and perspectives. ACS Nano 16 (7), 10042–10065. doi:10.1021/acsnano.2c01412
Kim, D., Han, S. A., Kim, J. H., Lee, J., Kim, S., and Lee, S. (2020). Biomolecular piezoelectric materials: from amino acids to living tissues. Adv. Mater. 32, 1906989. doi:10.1002/adma.201906989
Lai, D., Ma, W., Wang, J., Zhang, L., Shi, J., Lu, C., et al. (2023). Immune infiltration and diagnostic value of immune-related genes in periodontitis using bioinformatics analysis. J. Periodont. Res. 58, 369–380. doi:10.1111/jre.13097
Li, J., Zhao, X., Xia, Y., Qi, X., Jiang, C., Xiao, Y., et al. (2024b). Strontium-containing piezoelectric biofilm promotes dentin tissue regeneration. Adv. Mater. 36, 2313419. doi:10.1002/adma.202313419
Li, P., Ou, Q., Shi, S., and Shao, C. (2023). Immunomodulatory properties of mesenchymal stem cells/dental stem cells and their therapeutic applications. Cell. Mol. Immunol. 20 (6), 558–569. doi:10.1038/s41423-023-00998-y
Li, X., Tian, B. M., Deng, D. K., Liu, F., Zhou, H., Kong, D. Q., et al. (2022). LncRNA GACAT2 binds with protein PKM1/2 to regulate cell mitochondrial function and cementogenesis in an inflammatory environment. Bone Res. 10 (1), 29. doi:10.1038/s41413-022-00197-x
Li, Y., Liu, Y., Cui, J., Zhu, M., Wang, W., Chen, K., et al. (2024a). Oral-gut microbial transmission promotes diabetic coronary heart disease. Cardiovasc. Diabetol. 23, 123. doi:10.1186/s12933-024-02217-y
Liu, Q., Liu, L., Fan, D., Xie, S., Wang, C., Gou, X., et al. (2024b). Self-powered biodegradable piezoelectric fibrous composites as antibacterial and wound healing dressings. Appl. Mater. Today 37, 102120. doi:10.1016/j.apmt.2024.102120
Liu, X., Wan, X., Sui, B., Hu, Q., Liu, Z., Ding, T., et al. (2024a). Piezoelectric hydrogel for treatment of periodontitis through bioenergetic activation. Bioact. Mater. 35, 346–361. doi:10.1016/j.bioactmat.2024.02.011
Lu, M., Xuan, S., and Wang, Z. (2019). Oral microbiota: a new view of body health. Food Sci. Hum. well. 8 (1), 8–15. doi:10.1016/j.fshw.2018.12.001
Luan, J., Li, R., Xu, W., Sun, H., Li, Q., Wang, D., et al. (2023). Functional biomaterials for comprehensive periodontitis therapy. Acta Pharm. Sin. B 13 (6), 2310–2333. doi:10.1016/j.apsb.2022.10.026
Luo, S., Zhang, C., Xiong, W., Song, Y., Wang, Q., Zhang, H., et al. (2024). Advances in electroactive biomaterials: through the lens of electrical stimulation promoting bone regeneration strategy. J. Orthop. Transl. 47, 191–206. doi:10.1016/j.jot.2024.06.009
Ma, G., Wu, A., Zhou, S., Wang, M., Zhang, B., Liu, Y., et al. (2024). Tooth whitening and caries prevention toothbrush based on PTFE electret. J. Mater. Sci. 59, 2522–2533. doi:10.1007/s10853-024-09355-4
Mei, H., Liu, H., Sha, C., Lv, Q., Song, Q., Jiang, L., et al. (2024). Multifunctional metal-phenolic composites promote efficient periodontitis treatment via antibacterial and osteogenic properties. ACS Appl. Mater. Interfaces 16 (11), 13573–13584. doi:10.1021/acsami.3c19621
Mi, J., Zhi, M., Kang, W., Liang, Q., Tang, D., Wang, T., et al. (2024). Succession of the oral microbiome with the increasing severity of periodontitis. VIEW 5, 20230118. doi:10.1002/viw.20230118
Mokhtari, F., Azimi, B., Salehi, M., Hashemikia, S., and Danti, S. (2021). Recent advances of polymer-based piezoelectric composites for biomedical applications. J. Mech. Behav. Biomed. 122, 104669. doi:10.1016/j.jmbbm.2021.104669
Montoya, C., Jain, A., Londono, J. J., Correa, S., Lelkes, P. I., Melo, M. A., et al. (2021a). Multifunctional dental composite with piezoelectric nanofillers for combined antibacterial and mineralization effects. ACS Appl. Mater. Interfaces 13, 43868–43879. doi:10.1021/acsami.1c06331
Montoya, C., Kurylec, J., Baraniya, D., Tripathi, A., Puri, S., and Orrego, S. (2021b). Antifungal effect of piezoelectric charges on PMMA dentures. ACS Biomater. Sci. Eng. 7 (10), 4838–4846. doi:10.1021/acsbiomaterials.1c00926
Moutsopoulos, N. M., and Konkel, J. E. (2018). Tissue-specific immunity at the oral mucosal barrier. Trends Immunol. 39 (4), 276–287. doi:10.1016/j.it.2017.08.005
Nain, A., Chakraborty, S., Barman, S. R., Gavit, P., Indrakumar, S., Agrawal, A., et al. (2024). Progress in the development of piezoelectric biomaterials for tissue remodeling. Biomaterials 307, 122528. doi:10.1016/j.biomaterials.2024.122528
Pan, Q., Zheng, Y., Zhou, Y., Zhang, X., Yuan, M., Guo, J., et al. (2024). Doping engineering of piezo-sonocatalytic nanocoating confer dental implants with enhanced antibacterial performances and osteogenic activity. Adv. Funct. Mater. 34, 2313553. doi:10.1002/adfm.202313553
Peng, S., Fu, H., Li, R., Li, H., Wang, S., Li, B., et al. (2024). A new direction in periodontitis treatment: biomaterial-mediated macrophage immunotherapy. J. Nanobiotechnol. 359, 22. doi:10.1186/s12951-024-02592-4
Peng, X., Cheng, L., You, Y., Tang, C., Ren, B., Li, Y., et al. (2022). Oral microbiota in human systematic diseases. Int. J. Oral. Sci. 14, 14. doi:10.1038/s41368-022-00163-7
Ren, J., Wang, X., Bao, T., Shen, X., Yin, D., Liang, Q., et al. (2024). Piezoelectric dual network dressing with adaptive electrical stimulation for diabetic infected wound repair via antibacterial, antioxidant, anti-inflammation, and angiogenesis. Chem. Eng. J. 491, 151801. doi:10.1016/j.cej.2024.151801
Roldan, L., Montoya, C., Solanki, V., Cai, K. Q., Yang, M., Correa, S., et al. (2023). A novel injectable piezoelectric hydrogel for periodontal disease treatment. ACS Appl. Mater. Interfaces 15 (37), 43441–43454. doi:10.1021/acsami.3c08336
Sedghi, L., DiMassa, V., Harrington, A., Lynch, S. V., and Kapila, Y. L. (2021). The oral microbiome: role of key organisms and complex networks in oral health and disease. Periodontol 87, 107–131. doi:10.1111/prd.12393
Sharma, A., Bhardwaj, U., Jain, D., and Kushwaha, H. S. (2022). NaNbO3/ZnO piezocatalyst for non-destructive tooth cleaning and antibacterial activity. iScience 25, 104915. doi:10.1016/j.isci.2022.104915
Shi, Y., Zhang, N., Liu, J., Wang, J., Shen, S., Zhang, J., et al. (2023). Preparation of nanocomposites for antibacterial orthodontic invisible appliance based on piezoelectric catalysis. Sensors 23, 5336. doi:10.3390/s23115336
Smith, M., and Kar-Narayan, S. (2022). Piezoelectric polymers: theory, challenges and opportunities. Int. Mater. Rev. 67, 65–88. doi:10.1080/09506608.2021.1915935
Song, J., Lu, Y., Pan, T., Wang, J., Liu, Z., Xu, L., et al. (2024). Manipulation of surface electrical charge on nanocomposite membranes confers wide spectrum bactericidal effects and promotes tissue regeneration. Adv. Funct. Mater. 34 (22), 2314024. doi:10.1002/adfm.202314024
Tonelli, A., Lumngwena, E. N., and Ntusi, N. A. B. (2023). The oral microbiome in the pathophysiology of cardiovascular disease. Nat. Rev. Cardiol. 20, 386–403. doi:10.1038/s41569-022-00825-3
Wang, J., Wu, J., Zhang, J., Guan, L., Feng, H., Zhu, K., et al. (2024b). Bibliometric and visualized analysis of piezoelectric materials in biomedical application. ACS Appl. Electron. Mater. 6 (3), 1562–1573. doi:10.1021/acsaelm.3c01596
Wang, K., Han, C., Li, J., Qiu, J., Sunarso, J., and Liu, S. (2022a). The mechanism of piezocatalysis: energy band theory or screening charge effect? Angew. Chem. Int. Ed. 134 (6), e202110429. doi:10.1002/ange.202110429
Wang, L., Zhang, S., Zhang, Y., and An, Q. (2023b). Piezodynamic therapy: mechanisms and biomedical applications. Nano Energy 110, 108342. doi:10.1016/j.nanoen.2023.108342
Wang, X., Dai, X., and Chen, Y. (2023c). Sonopiezoelectric nanomedicine and materdicine. Small 19, 2301693. doi:10.1002/smll.202301693
Wang, X., Huan, Y., Ji, S., Zhu, Y., Wei, T., and Cheng, Z. (2022c). Ultra-high piezoelectric performance by rational tuning of heterovalent-ion doping in lead-free piezoelectric ceramics. Nano Energy 101, 107580. doi:10.1016/j.nanoen.2022.107580
Wang, Y., Chang, L., Gao, H., Yu, C., Gao, Y., and Peng, Q. (2024a). Nanomaterials-based advanced systems for photothermal/photodynamic therapy of oral cancer. Eur. J. Med. Chem. 272 (5), 116508. doi:10.1016/j.ejmech.2024.116508
Wang, Y., Wang, S., Meng, Y., Liu, Z., Li, D., Bai, Y., et al. (2022b). Pyro-catalysis for tooth whitening via oral temperature fluctuation. Nat. Commun. 13, 4419. doi:10.1038/s41467-022-32132-3
Wang, Y., Wen, X., Jia, Y., Huang, M., Wang, F., Zhang, X., et al. (2020). Piezo-catalysis for nondestructive tooth whitening. Nat. Commun. 11, 1328. doi:10.1038/s41467-020-15015-3
Wang, Y., Zang, P., Yang, D., Zhang, R., Gai, S., and Yang, P. (2023a). The fundamentals and applications of piezoelectric materials for tumor therapy: recent advances and outlook. Mater. Horiz. 10, 1140–1184. doi:10.1039/d2mh01221a
Wei, Y., Hu, X., Shao, J., Wang, S., Zhang, Y., Xie, W., et al. (2024c). Daily sonic toothbrush triggered biocompatible BaTiO3/chitosan multiporous coating with enhanced piezocatalysis for intraoral antibacterial activity. Mater. Today Commun. 38, 107715. doi:10.1016/j.mtcomm.2023.107715
Wei, Y., Liang, Y., Qi, K., Gu, Z., Yan, B., and Xie, H. (2024b). Exploring the application of piezoelectric ceramics in bone regeneration. J. Biomater. Appl., 8853282241274528. doi:10.1177/08853282241274528
Wei, Y., Zheng, L., Xie, X., Yang, X., and Liao, J. (2024a). Recent advances in stimuli responsive hydrogels for oral disease treatment. Mater. Des. 240, 112817. doi:10.1016/j.matdes.2024.112817
WHO (2022). Global oral health status report: towards universal health coverage for oral health by 2030. Geneva: World Health Organization.
Wu, L., Gao, H., Han, Q., Guan, W., Sun, S., Zheng, T., et al. (2023). Piezoelectric materials for neuroregeneration: a review. Biomater. Sci. 11, 7296–7310. doi:10.1039/d3bm01111a
Xu, H., Wang, L., Zhao, B., Zhao, C., and Lin, K. (2023). Research and application progress of piezoelectric biomaterials in tissue regeneration. Prog. Biomed. Eng. 44, 1674–1242. doi:10.3969/j.issn.1674-1242.2023.02.001
Xu, Q., Gao, X., Zhao, S., Liu, Y. N., Zhang, D., Zhou, K., et al. (2021). Construction of bio-piezoelectric platforms: from structures and synthesis to applications. Adv. Mater. 33, 2008452. doi:10.1002/adma.202008452
Yang, B., Pang, X., Li, Z., Chen, Z., and Wang, Y. (2021). Immunomodulation in the treatment of periodontitis: progress and perspectives. Front. Immunol. 12, 781378. doi:10.3389/fimmu.2021.781378
Zhang, H., Tang, Y., Gu, Z., Wang, P., Chen, X., Lv, H., et al. (2024b). Biodegradable ferroelectric molecular crystal with large piezoelectric response. Science 383, 1492–1498. doi:10.1126/science.adj1946
Zhang, L., Pan, J., and Zhang, J. (2022). Integrated two-phase free radical hydrogel: safe, ultra-fast tooth whitening and antibacterial activity. J. Mater. Sci. Technol. 100, 59–66. doi:10.1016/j.jmst.2021.05.029
Zhang, L., Yang, T., Ding, L., Chang, M., Yin, X., Chen, Y., et al. (2024c). Engineering 2D Bi4NbO8Br single crystalline nanosheets for piezoelectric and piezodynamic tumor nanotherapy. Chem. Eng. J. 484, 149445. doi:10.1016/j.cej.2024.149445
Zhang, S., Zhang, H., Sun, J., Javanmardi, N., Li, T., Jin, F., et al. (2024a). A review of recent advances of piezoelectric poly-L-lactic acid for biomedical applications. Int. J. Biol. Macromol. 276, 133748. doi:10.1016/j.ijbiomac.2024.133748
Zhao, R., Han, W., Tang, K., Shao, R., Zhu, P., Zhang, S., et al. (2022). Function of normal oral mucosa revealed by single-cell RNA sequencing. J. Cell. Biochem. 123, 1481–1494. doi:10.1002/jcb.30307
Zheng, H., Lin, H., Tian, H., Lin, K., Yang, F., Zhang, X., et al. (2024). Steering piezocatalytic therapy for optimized tumoricidal effect. Adv. Funct. Mater. 34, 2400174. doi:10.1002/adfm.202400174
Zheng, H., Zhou, Y., Zheng, Y., and Liu, G. (2023). Advances in hydrogels for the treatment of periodontitis. J. Mater. Chem. B 11, 7321–7333. doi:10.1039/d3tb00835e
Keywords: piezoelectric material, teeth whitening, oral disease treatment, treatment mechanism, ROS, electrical stimulation
Citation: Yang T, Sa R, Wang F, Chen C and Zheng L (2024) Research progress of piezoelectric materials in protecting oral health and treating oral diseases: a mini-review. Front. Bioeng. Biotechnol. 12:1473126. doi: 10.3389/fbioe.2024.1473126
Received: 08 August 2024; Accepted: 02 September 2024;
Published: 13 September 2024.
Edited by:
Chuang Liu, Harvard Medical School, United StatesReviewed by:
Yufen Xiao, University of Texas Southwestern Medical Center, United StatesXiao Xu, University of California, Los Angeles, United States
Copyright © 2024 Yang, Sa, Wang, Chen and Zheng. This is an open-access article distributed under the terms of the Creative Commons Attribution License (CC BY). The use, distribution or reproduction in other forums is permitted, provided the original author(s) and the copyright owner(s) are credited and that the original publication in this journal is cited, in accordance with accepted academic practice. No use, distribution or reproduction is permitted which does not comply with these terms.
*Correspondence: Lanbing Zheng, bGFuYmluZ3poZW5nQHllYWgubmV0
†These authors have contributed equally to this work