- 1Department of Bone and Joint Surgery, Peking University Shenzhen Hospital, Shenzhen, China
- 2National and Local Joint Engineering Research Center of Orthopaedic Biomaterials, Peking University Shenzhen Hospital, Shenzhen, China
- 3Shenzhen University School of Medicine, Shenzhen, China
- 4Department of Sport Medicine, Peking University Shenzhen Hospital, Shenzhen, China
- 5Shenzhen Key Laboratory of Orthopaedic Diseases and Biomaterials Research, Shenzhen, China
Osteoarthritis (OA) is a degenerative disease linked to aging and obesity. The global aging population has led to an increasing number of OA patients, imposing a significant economic burden on society. Traditional drugs treatment methods often fail to achieve satisfactory outcomes. With the rapid advancement of nanomaterial delivery systems, numerous studies have focused on utilizing nanomaterials as carriers to achieve efficient OA treatment by effectively loading and delivering bioactive ingredients (e.g., drugs, nucleic acids) tailored to the unique pathological conditions, such as the weakly acidic microenvironment of synovial fluid in OA patients. This review highlights the latest advancements in the use of pH-responsive nanoparticles for OA treatment, emphasizing the principle of targeted drug delivery leveraging the acidic microenvironment of inflamed joints. It further discusses the composition, synthesis, response mechanism, target selection, application, and recent research findings of nanoparticles, while also addressing the challenges and future directions in this promising field.
1 Introduction
Osteoarthritis (OA) is one of the most common joint diseases worldwide, primarily caused by obesity and aging. By 2032, it is estimated that 29.5% of individuals over the age of 45 will have OA, with 15.7% affected by knee OA (Martel-Pelletier et al., 2016; Hunter and Bierma-Zeinstra, 2019). OA is a slowly progressing disease characterized by irreversible structural changes involving the entire joint, including articular cartilage, subchondral bone, ligaments, synovium, and joint capsule. Mechanical, inflammatory, and metabolic factors contribute to the gradual loss of joint function. (Brandt et al., 2006; Martel-Pelletier et al., 2016) The degeneration and destruction of articular cartilage are central to the pathophysiological changes of OA. The disease progression is marked by alterations in cartilage composition, leading to a gradual loss of its integrity. (Loeser et al., 2016).
As the disease advances, symptoms such as pain emerge. Hypertrophic chondrocytes increase their synthetic activity, producing matrix degradation products and pro-inflammatory mediators, including matrix metalloproteinases (MMPs) (Mehana et al., 2019), a disintegrin and metalloproteinase with thrombospondin motifs (ADAMTS) (Bondeson et al., 2008), Interleukin-1β(IL-1β), Interleukin-6(IL-6), and tumor necrosis factor (TNF) (Glyn-Jones et al., 2015). These factors promote the proliferation of adjacent synovial cells and inflammatory responses, with synovitis being a primary source of pain. Ultimately, OA leads to the loss of joint function, severely impacting patients’ quality of life.
Current treatment for osteoarthritis (OA) varies according to the disease’s severity. (Nelson et al., 2014; Bannuru et al., 2019; Gibbs et al., 2023) In early-stage OA, pain symptoms are not prominent, and joint range of motion is not significantly limited. Numerous guidelines recommend non-drug treatments as the first line of treatment, emphasizing patient health education, self-management, exercise, and weight loss. (Block et al., 2014; Nelson et al., 2014) When pain and other symptoms appear, drug therapy becomes the main treatment, including oral non-steroidal anti-inflammatory drugs (NSAIDs) or topical analgesics. Although oral medications effectively relieve pain and improve function, they cannot ensure effective drug concentration in the knee cavity, and local topical drugs are limited by their permeability. Issues such as short action time, rapid metabolism, single efficacy, and low bioavailability are major drawbacks of drug treatments for OA. (Hu et al., 2019; Liu et al., 2020) Moreover, these drugs mainly relieve pain and symptoms without directly treating OA, failing to fundamentally alleviate or inhibit disease progression. (Mao et al., 2021).
Injections of glucocorticoids (Felson et al., 2016; Orchard et al., 2020), hyaluronic acid (HA) (Altman et al., 2015; Kang et al., 2021), and platelet-rich plasma (PRP) into the joint cavity are also widely used. (Katz, 2021) However, due to rapid fluid circulation and drug metabolism in the joint cavity, drugs do not remain long, necessitating repeated injections. This increases the risk of joint infection and reduces patient compliance. Generally, current pharmacological treatments for OA are mostly palliative. For end-stage OA patients, when joint pain cannot be effectively relieved and joint movement is significantly limited, knee replacement surgery becomes a clinically and cost-effective option. (Higashi and Barendregt, 2011; Ruiz et al., 2013) However, the perioperative and lifetime postoperative risks of joint replacement surgery must be objectively evaluated (Ferket et al., 2017). Therefore, early detection and diagnosis of OA, early interventional treatment, and delaying its progression have become the focus of current clinical research. (Little et al., 2013).
With the rapid advancement of nanotechnology, nano-drug delivery systems have been extensively developed for tumor treatment and are gradually being applied to osteoarthritis (OA). These systems can achieve slow drug release in specific areas by leveraging the unique pathophysiological background of OA and responding to specific internal and external stimuli. Through modular combination, multifunctional nanomedicine delivery systems have been constructed. (Jiang and Zhang, 2023) Initially, nanoparticle delivery systems primarily functioned as carriers, loading bioactive ingredients in specific forms and achieving slow drug release in the joint cavity through intra-articular injection to enhance therapeutic effects. As nanotechnology has advanced and our understanding of the OA joint microenvironment has deepened, a series of stimulus-responsive nanoparticle delivery systems targeting OA joint microenvironment signals have been developed. These systems can respond to specific stimuli, target specific cells or tissues, alleviate inflammation, and promote cartilage regeneration. Numerous studies have reported that OA joint cavities are weakly acidic, leading to the development of a series of pH-responsive nanoparticle delivery systems for effective OA treatment. This article primarily reviews the application of pH-responsive nanoparticle delivery platforms in the treatment of osteoarthritis.
2 Pathological changes of pH in the joint of OA
During the progression of osteoarthritis (OA), the enhancement of the local inflammatory response produces numerous inflammatory factors and mediates tissue repair. This vigorous metabolic activity of inflammatory cells leads to a relative shortage of oxygen, inducing chondrocytes to shift towards anaerobic glycolysis. This process produces a large amount of lactic acid, gradually acidifying the joint cavity microenvironment. Additionally, inflammatory damage causes sustained degradation of articular cartilage and increased production of cartilage-degrading enzymes such as metalloproteinases. These enzymes further degrade the cartilage, releasing more degradation products that exacerbate the inflammatory response.
Inflammatory responses, cartilage degradation, and other factors collectively drive the metabolic activities within the joint cavity. Acidic substances like lactic acid are exchanged with the body primarily through synovial fluid and can be excreted. While the pH of synovial fluid in a normal joint cavity is 7.4, it gradually drops to 6.6–7.2, and can reach as low as 6.0, with the progression of OA and cartilage degradation (Treuhaft and MCCarty, 1971; Geborek et al., 1989; Andersson et al., 1999; Lee et al., 2011; Dou et al., 2020; Lombardi et al., 2022). The overall production of H+ in the joint cavity can be comparable to that in rheumatoid arthritis and solid tumor microenvironments. H+-ATPase, Na+-H+ exchange pump, and monocarboxylate transporter (MCT) are involved in the excretion of H+, lactic acid, and other related substances, while the exchange of anions such as Cl− and HCO3− in chondrocytes or organelles helps maintain pH stability in the joint cavity (Matsuyama et al., 2000; Webb et al., 2011; Coleman et al., 2016; Zhang et al., 2022).
On one hand, the relative hypoxia of the chondrocyte matrix aggravates the acidification of the chondrocyte microenvironment, with mitochondria-dependent apoptosis characterized by mitochondrial alkalization and cytoplasmic acidification, continuously lowering the joint microenvironment’s pH (Matsuyama et al., 2000). On the other hand, the surrounding microenvironment produces substances to buffer the H+ concentration, maintaining the overall stability of the joint cavity microenvironment (Vaupel et al., 1989).
3 Application of pH-responsive nano-drug delivery systems in osteoarthritis
In recent years, researchers have developed nanoscale, highly integrated, multifunctional hybrid delivery platforms using various physical and chemical synthesis methods. These systems have different morphologies, such as nanospheres, nanorods, and nanostars, nanoshells, which provide nanoparticles with excellent loading performance for bioactive ingredients such as drugs, peptides, and nucleic acids (Mabrouk et al., 2021). Leveraging the weakly acidic environment of the osteoarthritis (OA) joint cavity, these systems enable active and passive drug delivery with continuous slow release, extending drug residence time and increasing cellular uptake, thus achieving effective OA treatment (Zhang et al., 2022).
These pH-responsive drug delivery systems respond to the low pH changes in the OA joint cavity, causing structural cracking and releasing the bioactive ingredients. Figure 1 illustrates the mechanism of the weak acidic microenvironment in OA patients’ joint cavity and the response mode of pH-responsive nanoparticles. Table 1 summarizes the material composition, bioactive ingredients, response mode and characteristics, experimental models, target sites, and results of current pH-responsive nanomaterials for OA treatment, comparing and evaluating their therapeutic effects and recent advancements.
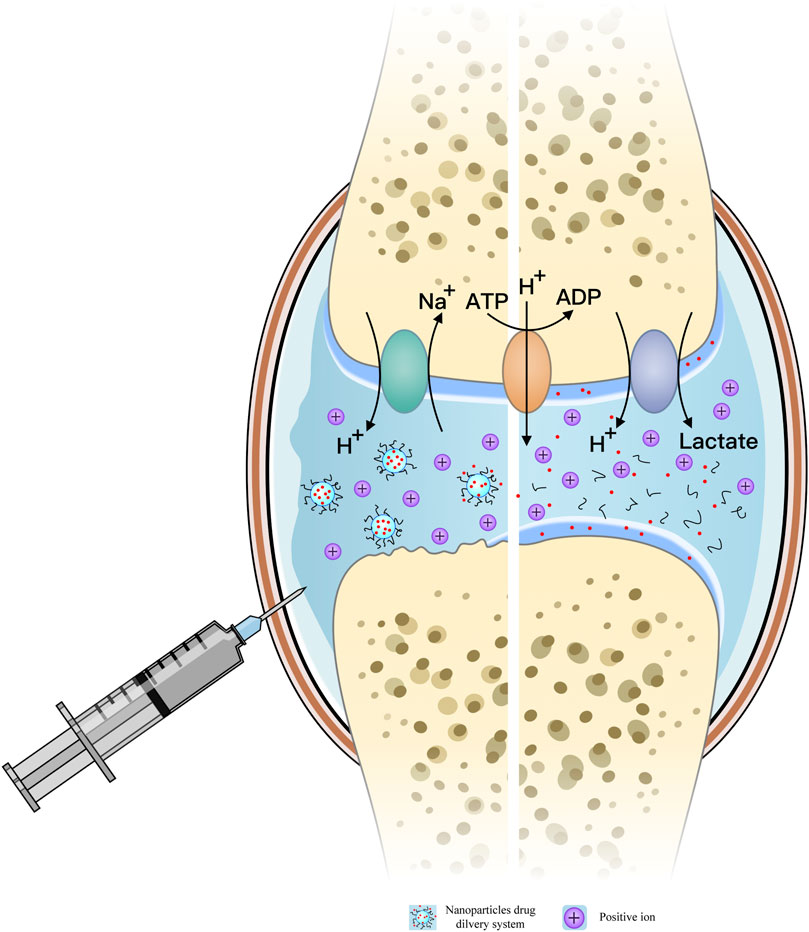
Figure 1. Mechanism of the weak acid microenvironment in the joint cavity of osteoarthritis (OA) patients; pH-responsive release model of nanoparticles (NPs) in the OA joint cavity.
pH-responsive nano-drug delivery systems offer significant advantages in OA treatment:
1. Higher drug delivery efficiency.
2. Controlled drug release rate and enhanced cellular uptake as needed.
3. High drug loading capacity, extending the administration cycle and reducing administration frequency. (Karimi et al., 2016).
4. Adjustable polymer proportions to fit the pH response range, maintaining stability at physiological pH (7.4) and gradually hydrolyzing in acidic environments, thereby mediating a cascade amplification effect for explosive drug release. (Zhou et al., 2017).
The pH response mechanism of nanomaterials primarily involves the breaking of acid-sensitive chemical bonds and the protonation of chemical groups (Liu et al., 2014; Wang et al., 2015; He et al., 2020). The former refers to the introduction of acid-sensitive chemical bonds to connect drugs and the nanoparticle structure or encapsulate drugs within the acid-sensitive nanoparticle structure. These bonds are stable at physiological pH (∼7.4) but hydrolyze in acidic environments (pH 4.5–6.5), then nanoparticles release drugs or bioactive ingredients by cracking acid-sensitive chemical bonds or reversing charges (e.g., hydrazine (Yang et al., 2010), acetate (Schlossbauer et al., 2011), imine (Yu et al., 2016) in response to pH changes in the OA microenvironment. And the latter receives or donates protons and produce pH-dependent structural and hydrophobic changes (e.g., carboxylic acid in weakly acidic and amine groups in weakly alkaline environments) to achieve drug release on demand (Wang et al., 2015; Zhao et al., 2015). Additionally, some pH-sensitive materials, such as polylysine, polyhistidine, poly(dimethyl lactamide), and poly(benzyl glutamate), can mediate the escape of nanoparticle drug delivery systems from endosomes or lysosomes and facilitate drug delivery into cells through protonation (Karimi et al., 2016; Zhou et al., 2017; He et al., 2020).
3.1 pH-responsive nano-drug delivery systems for OA treatment
3.1.1 pH-responsive nanoparticles for OA treatment
Based on the characteristics of the weak acidic microenvironment in the joint cavity of osteoarthritis (OA), researchers have designed various nano-drug delivery systems with different compositions. These pH-responsive nanoparticles are constructed using raw materials with intrinsic pH response characteristics. For instance, Wang et al. designed a pH-responsive nanoparticles(PCL/PEG-NAR) composed of polycaprolactone and PEG naringin. This membrane was prepared by forming an ester bond between the carboxyl group of mPEG-COOH and naringin. In an acidic microenvironment, acid-promoted ester bond breaking effectively “opens” the nanofiber membrane structure, releasing naringin slowly and thereby slowing the progression of OA (Wang et al., 2022).
Hu et al. developed a polymer nanoparticle Rh-PLGA-NPs@NH4, with PLGA as the main body loaded with Rhein (Rh). In the weakly acidic joint cavity, rich in H+, the H+ penetrates the nanoparticle cladding and reacts with NH4HCO3 to produce NH4, CO2, and H2O. The rapid generation of a large amount of gas destroys the nanoparticle cladding, achieving explosive drug release (Hu et al., 2020). Additionally, the precursor material of nanoparticles can also be derived from organisms, offering excellent biocompatibility. Xiong et al. designed a pH-responsive biodegradable hollow structure manganese Prussian blue nanosystems (HMPBzymes). By inducing the phenotypic transformation of macrophages from M1 to M2, this system prevents and reverses the pathological progression of OA. The selection of a mesoporous structure provides a larger surface area, increases the contact area between catalyst and reactant, improves catalytic efficiency, and enhances the drug loading rate (Xiong et al., 2022).
3.1.2 pH/ROS dual responsive nanoparticles for OA treatment
With the deepening of research on the pathology, molecular biology, and pharmacology of osteoarthritis(OA) and the rapid development of nanomaterials, the limitations of nano-drug delivery systems with single stimulus-response, single target, and single drug delivery have become increasingly obvious, including low target specificity, misdelivery in non-disease areas, and potential physical and chemical damage to healthy tissues caused by other stimuli. Consequently, the multi-stimulus-response model combined with a multi-target, multi-drug delivery system has emerged as a new direction for OA treatment.
Based on this approach, Zhao et al. synthesized a pegylated, phenylboric acid-modified levodopa pro-antioxidant (pPAD) that can self-assemble into nanoparticles (pPADN) for specific loading and dual-response delivery of dexamethasone (Dex) (Zhao et al., 2021). When exposed to reactive oxygen species (ROS), pPADN transforms into the active form of levodopa, exerting ROS clearance and antioxidant effects. Simultaneously, the acidic microenvironment in the joint cavity and the structural modification of pPADN promote the specific release of Dex. Multiple stimulus-response modes facilitate specific responses to different environmental changes according to the disease’s pathophysiological characteristics, reducing the incidence of erroneous drug release and incorrect targeting.
3.2 pH responsive nanoparticles as theranostic systems for OA treatment
Due to the lack of appropriate monitoring indicators to reflect the response process of nanoparticles in vivo, and the inability to accurately observe their operation path in vivo, the diagnosis of osteoarthritis (OA), evaluation of therapeutic effects, and tracing of therapeutic drugs remain challenging. An integrated diagnosis and treatment system is essential for achieving these functions and facilitating the efficient treatment of OA.
Kang et al. designed a self-assembled nanomicelle coated with curcumin (ACP), using poly(β-amino ester) as the main component, to achieve a rapid response in an acidic environment, release curcumin, and generate fluorescence signals at the inflammation site (Kang et al., 2020). ACP micelles were injected into the joint cavity of osteoarthritis induced by iodoacetate (MIA), and the inflammatory response was observed through fluorescence imaging. No recognizable fluorescence signal was observed in the normal joint cavity, but a significantly enhanced fluorescence signal was seen at the inflammatory site. This indicates that ACP maintains a stable micelle structure and achieves fluorescence quenching under normal physiological conditions, but under acidic conditions, the structure breaks down to expose fluorescence.
Also, Zerrillo et al. designed a polylactic-co-glycolic acid copolymer nanoparticle (PLGA NPs) coated with near-infrared dye (NIR) to track the release and uptake path of NPs in the joint lumen through optical imaging and evaluate its therapeutic effect on cartilage (Zerrillo et al., 2019). Lan et al. used a nanomicelle (MRC-PPL@PSO) as a response probe loaded with fluorescent dye cy5.5 to observe the drug targeting effect in vivo in real-time and continuously release the drug(Lan et al., 2020). Chen et al. designed a novel chondron-targeting, MMP-13/pH-responsive ferritin nanocage (CMFn), which contains cy5.5 and uses a quenching agent (BHQ-3) to “mask” its fluorescence signal. As OA progresses, MMP-13 enzyme activity is highly expressed, and CMFn responds to the overexpressed MMP-13 level in the OA microenvironment. It can intelligently “turn on” the fluorescence signal and induce drug release, serving both OA imaging and therapeutic functions. The detected fluorescence intensity reflects the severity of OA, enabling precise staging of the disease and ensuring timely treatment (Chen et al., 2019).The self-assembled nanoparticle (pPADN) synthesized by Zhao et al. converts into melanin-like compounds in an acidic microenvironment to activate near-infrared (NIR) photoacoustic (PA) signals, tracking the development of OA. This approach can identify inflammatory areas and monitor treatment outcomes, opening a new avenue for integrating non-invasive examination, diagnosis, and treatment (Zhao et al., 2021).
Numerous studies have confirmed the feasibility and controllability of fluorescent dyes to reflect the real-time response process of nanomaterials, promoting the combined application of various fluorescent dyes and nanomaterials. In the future, the properties of fluorescent dyes may be further utilized to reveal more pathophysiological changes in the development of osteoarthritis.
3.3 pH-responsive nanoparticle/hydrogel composite for OA treatment
Although pH-responsive nanodrug delivery systems have significantly improved drug delivery efficiency in the joint cavity, the pathological changes in the osteoarthritis (OA) joint microenvironment and the frequent exchange of joint fluid make it challenging for drugs to remain in the joint for extended periods. Researchers have developed several specialized material-modified nanomedical drug delivery systems targeting damaged cartilage(Chen et al., 2019; Lan et al., 2020), synovium (Nygaard and Firestein, 2020), or subchondral bone (Zhen and Cao, 2014; Yang et al., 2018) to achieve prolonged retention by anchoring to cells or the extracellular matrix, thereby extending the duration of drug action. However, targeting and lubrication capabilities still need improvement, as adequate lubrication of the articular cartilage surface is fundamental to pain relief (Clarke, 2021; Li et al., 2021). Therefore, the combination of controlled drug release and enhanced lubrication ability has been recognized as a crucial direction to further improve the efficacy of drug therapy. Researchers have introduced hydrogel systems to construct nanoparticle-hydrogel composite systems. The colloidal matrix of the hydrogel hinders the premature release of nanoparticles, allowing them to be released in response to the microenvironment.
For example, Han et al. designed a hydrogel microsphere loaded with IA-ZIF-8 (IA-ZIF-8@HMs), combining a hydrogel matrix with functional nanoparticles to impart IA-ZIF-8 with effective pH response characteristics. The hydrogel matrix further facilitates the slow release of the nanoparticles, creating a secondary structure drug delivery platform with pH response capabilities (Yu et al., 2023). Li et al. developed a pH-responsive, in situ gelatinized nanoparticle (AHCPA NPs) system. Under acidic conditions, the nanoparticle structure decomposes in response to pH changes, releasing various hydrophilic polyvalent host molecules. Enhanced host-guest interactions and the spontaneous assembly of polyvalent guests form an in situ hydrogel within the joint cavity. This hydrogel acts as a lubricant to reduce local wear and provides sustained release of dexamethasone (DEX) to minimize inflammation (Li et al., 2022). Additionally, Zhou et al. designed a dual delivery system using a hyaluronic acid (HA) cross-linked platelet-rich plasma (PRP) hydrogel network carrying bovine serum albumin-manganese dioxide nanosheets (BSA-MnO2). The HA/PRP/BM hydrogel, utilizing traditional raw materials like hyaluronic acid (HA), serves as a lubricant and delivery carrier. The manganese dioxide (MnO2) nanosheets achieve pH response and ROS clearance, triggering the release of multiple payloads under acidic conditions, including the total protein of PRP and the representative growth factor transforming growth factor β1 (TGF-β1). (Zhou et al., 2022).
We have deleted some content of sections 5 and 6 and integrated them into section 7(5) for a brief explanation. We have also revised the relevant content in section 7(5) to make it more academically styled and enhance the readability of the article.
4 Conclusions and prospects
Based on the information summarized in Table 1, we understand that pH-responsive nanoparticles can successfully respond to the weakly acidic microenvironment within the osteoarthritic joint cavity. However, challenges such as burst release and insufficient sustained release remain. By integrating additional response mechanisms, we can achieve more controllable drug release. Furthermore, incorporating hydrogel systems not only facilitates nanoparticle release but also provides lubrication.
Some nanoparticles, either alone or loaded with fluorescent dyes, can serve imaging functions to monitor the progression of osteoarthritis in real-time. Despite extensive research on nanoparticles for osteoarthritis (OA) treatment, current nanoparticle delivery systems have many limitations. Many functionalities of nanoparticles remain unexplored, and several pathophysiological changes in osteoarthritis are still not thoroughly elucidated.
On the one hand, we need to consider the safety of nanoparticle metabolism in the body. Protonation-induced escape from the endoplasmic reticulum and lysosomes can lead to the leakage of hydrolases into the cytoplasm, resulting in autophagy and cell death (Mura et al., 2013). Additionally, pH-responsive nanomaterials based on acid-sensitive chemical bond response mechanisms can be partially cytotoxic due to the presence of ketone or aldehyde functional groups and cationic polymer residues (Sonawane et al., 2017). Some nanoparticles can interact closely with the immune system, potentially inducing hypersensitivity reactions, and trigger the overproduction of reactive oxygen species (ROS) in the body (Mitragotri and Lahann, 2009; Feng et al., 2019).
On the other hand, the preparation of pH-responsive drug delivery systems should comprehensively consider the characteristics of the material, the pathophysiological characteristics of OA, the composition and physical and chemical properties of the drug, and the optimal therapeutic dose. Common targets for pH-responsive nanomaterials in osteoarthritis (OA) treatment, such as cartilage, synovium, and subchondral bone, are insufficient. In addition to exploring drug release at the target, the impact of disease-specific anatomical and physiological barriers (e.g., extracellular matrix (ECM)) on the bioavailability of nanomaterials must be considered (Lavrador et al., 2018; Majumder and Minko, 2021). It is essential to deepen our understanding of OA at the molecular level. Additionally, and promote the development of new materials to discover more target-specific and biocompatible drug carriers. Thus, it opens up a new and effective way for disease treatment (Mura et al., 2013; Lavrador et al., 2018; Raza et al., 2019).
At present, the application of pH-responsive drug delivery systems in the treatment of osteoarthritis (OA) primarily focuses on the low pH characteristic, the designed nanomaterials crack under acidic conditions, causing the release of bioactive components. Some studies have further utilized material characteristics to assemble multifunctional modules, achieving dual and multiple targeting capabilities, such as type II collagen targeting peptide (Lan et al., 2020) and specific gene knockout sequences (siHIF-2α) (Zhang ZJ. et al., 2023) Additionally, fluorescence can be loaded and specifically regulated to “turn on and off,” providing imaging capabilities and real-time visualization of the progression and severity of osteoarthritis (Chen et al., 2019). Although there are changes in pH, reactive oxygen species (ROS), and matrix metalloproteinases (MMPs) in damage tissues(Feng and Chen, 2018; Dou et al., 2020), material characteristics or bioactive ingredients, primarily target cartilage to achieve therapeutic effects for osteoarthritis (OA) by alleviating or improving cartilage lesions. There are still few studies focusing on the synovium and subchondral bone. It is impossible to achieve accurate drug release in a complex microenvironment with multiple signals by relying on a single stimulus mode. This limitation increases the “false positive” rate and the risk of non-targeted delivery (Municoy et al., 2020).
By equipping materials with multiple response capabilities, we can construct multi-stage reaction systems for drug delivery, and combine imaging and treatment through the use of fluorescent dyes. For example, pH and ultrasound (US) response materials can be used to improve both drug release and cellular uptake. Magnetic and US response materials have heating effects, while magnetic response materials also possess unique magnetic permeability properties. The unique advantages of different response systems become the key to optimizing carrier performance and achieving multiple response capabilities. For example, MMP-13/pH-responsive ferritin nanocages are modified to respond to the overexpression of MMP-13 and pH changes in OA joint cavities. These nanocages reflect the progression of osteoarthritis in real-time by intelligently “turning on” fluorescence imaging and releasing anti-inflammatory drugs (Chen et al., 2019). More importantly, the multistage response capability of the composite system allows the carriers to be altered through two or more stimuli, enabling batch and on-demand release of drugs (Costa Lima and Reis, 2015). However, given the uncertainty of interactions between different materials and their interference, it is crucial to objectively evaluate the overall therapeutic effect of dual and multiple response systems. Blindly combining different stimuli could potentially have adverse effects.
In designing new materials, we should consider not only the specificity of target selection but also the accuracy of targeting. For osteoarthritis (OA), the complexity of physiological variables such as disease status, blood flow, and tissue structure must also be considered (Muro, 2012; Noble et al., 2014). Currently, many advanced dual and multiple response systems are concentrated in cancer research, But, due to the common pathological characteristics of tumors and inflammation, these advancements are still of great guiding significance for developing drug delivery systems for OA treatment.
In the future, the development of nanoparticle delivery systems should aim to build multi-drug delivery platforms to overcome the limitations of single-drug delivery, which cannot achieve multi-functional therapeutic effects such as anti-inflammatory, antioxidant, and cartilage repair functions. Although some studies have successfully implemented dual bioactive component delivery systems for the treatment of osteoarthritis (OA), such as the pH-responsive nanoparticle dual drug delivery system based on circular brush zwitterionic polymer that successfully loaded hydrophobic curcumin and hydrophilic loxoprofen sodium (CB@Cur@LXP), the circular brush also has joint lubrication and ROS removal properties (Zhang M. et al., 2023). Additionally, a chondro-targeted dual drug delivery nano platform (RB@MPMW) was developed to conjugate Type II collagen targeting peptide (WYRGRL) and load rapamycin (Rap) and bilirubin (Br) with NIR laser irradiation to produce a photothermal effect for drug release (Xue et al., 2021). However, current dual-drug delivery systems have high requirements on the properties of the drugs themselves (e.g., polarity, hydrophobicity) and cannot achieve broad multi-drug loading and high load efficiency.
In conclusion, to achieve more accurate and efficient treatment of OA with pH-responsive targeted nanomaterials, and further promote the research and development of new materials to find more target-specific and biocompatible drug carriers. We must optimize target selection, targeting capability combinations, and material and drug combinations. Actively exploring a variety of signals of pathophysiological changes in OA patients, and not limiting our focus to targeting cartilage, will be crucial. The development direction for treating various diseases, including OA, will be nanoparticle drug delivery systems with multi-stimulus response modes, multi-functional targeting, and multi-drug delivery capabilities.
Author contributions
SL: Writing–original draft, Writing–review and editing. SJ: Data curation, Writing–review and editing. YY: Investigation, Writing–review and editing. HZ: Visualization, Writing–review and editing. PL: Visualization, Writing–review and editing, Supervision. JL: Supervision, Writing–review and editing.
Funding
The author(s) declare that financial support was received for the research, authorship, and/or publication of this article. This study was supported by grants from Guangdong Basic and Applied Basic Research Foundation (Nos 2021A1515220037, 2022A1515220165, 2023A1515220019, 2022A1515220056), National Natural Science Foundation of China (No. 82172432), Shenzhen Science and Technology Program (Nos JCYJ20210324105806016, JCYJ20210324110214040, JCYJ20220818102815033), Shenzhen Key Laboratory of Orthopaedic Diseases and Biomaterials Research (No. ZDSYS20220606100602005) and Sanming Project of Medicine in Shenzhen (No. SZSM202211038).
Conflict of interest
The authors declare that the research was conducted in the absence of any commercial or financial relationships that could be construed as a potential conflict of interest.
Publisher’s note
All claims expressed in this article are solely those of the authors and do not necessarily represent those of their affiliated organizations, or those of the publisher, the editors and the reviewers. Any product that may be evaluated in this article, or claim that may be made by its manufacturer, is not guaranteed or endorsed by the publisher.
References
Altman, R. D., Manjoo, A., Fierlinger, A., Niazi, F., and Nicholls, M. (2015). The mechanism of action for hyaluronic acid treatment in the osteoarthritic knee: a systematic review. BMC Musculoskelet. Disord. 16, 321. doi:10.1186/s12891-015-0775-z
Andersson, S. E., Lexmüller, K., Johansson, A., and Ekström, G. M. (1999). Tissue and intracellular pH in normal periarticular soft tissue and during different phases of antigen induced arthritis in the rat. J. Rheumatol. 26 (9), 2018–2024.
Bannuru, R. R., Osani, M. C., Vaysbrot, E. E., Arden, N., Bennell, K., Bierma-Zeinstra, S., et al. (2019). OARSI guidelines for the non-surgical management of knee, hip, and polyarticular osteoarthritis. Osteoarthr. Cartil. 27 (11), 1578–1589. doi:10.1016/j.joca.2019.06.011
Block, J. A. (2014). Osteoarthritis: OA guidelines: improving care or merely codifying practice. Nat. Rev. Rheumatol. 10 (6), 324–326. doi:10.1038/nrrheum.2014.61
Bondeson, J., Wainwright, S., Hughes, C., and Caterson, B. (2008). The regulation of the ADAMTS4 and ADAMTS5 aggrecanases in osteoarthritis: a review. Clin. Exp. Rheumatol. 26 (1), 139–145.
Brandt, K. D., Radin, E. L., Dieppe, P. A., and van de Putte, L. (2006). Yet more evidence that osteoarthritis is not a cartilage disease. Ann. Rheum. Dis. 65 (10), 1261–1264. doi:10.1136/ard.2006.058347
Chen, H., Qin, Z., Zhao, J., He, Y., Ren, E., Zhu, Y., et al. (2019). Cartilage-targeting and dual MMP-13/pH responsive theranostic nanoprobes for osteoarthritis imaging and precision therapy. Biomaterials 225, 119520. doi:10.1016/j.biomaterials.2019.119520
Clarke, J. (2021). Restoring cartilage lubrication to heal post-traumatic OA. Nat. Rev. Rheumatol. 17 (12), 707. doi:10.1038/s41584-021-00712-0
Coleman, M. C., Ramakrishnan, P. S., Brouillette, M. J., and Martin, J. A. (2016). Injurious loading of articular cartilage compromises chondrocyte respiratory function. Arthritis Rheumatol. 68 (3), 662–671. doi:10.1002/art.39460
Costa Lima, S. A., and Reis, S. (2015). Temperature-responsive polymeric nanospheres containing methotrexate and gold nanoparticles: a multi-drug system for theranostic in rheumatoid arthritis. Colloids Surf. B Biointerfaces 133, 378–387. doi:10.1016/j.colsurfb.2015.04.048
Dou, Y., Li, C., Li, L., Guo, J., and Zhang, J. (2020). Bioresponsive drug delivery systems for the treatment of inflammatory diseases. J. Control Release 327, 641–666. doi:10.1016/j.jconrel.2020.09.008
Felson, D. T. (2016). Intra-articular corticosteroids and knee osteoarthritis: interpreting different meta-analyses. JAMA 316 (24), 2607–2608. doi:10.1001/jama.2016.17227
Feng, X., and Chen, Y. (2018). Drug delivery targets and systems for targeted treatment of rheumatoid arthritis. J. Drug Target 26 (10), 845–857. doi:10.1080/1061186x.2018.1433680
Feng, X., Xu, W., Li, Z., Song, W., Ding, J., and Chen, X. (2019). Immunomodulatory nanosystems. Adv. Sci. (Weinh) 6 (17), 1900101. doi:10.1002/advs.201900101
Ferket, B. S., Feldman, Z., Zhou, J., Oei, E. H., Bierma-Zeinstra, S. M., and Mazumdar, M. (2017). Impact of total knee replacement practice: cost effectiveness analysis of data from the Osteoarthritis Initiative. BMJ 356, j1131. doi:10.1136/bmj.j1131
Geborek, P., Saxne, T., Pettersson, H., and Wollheim, F. A. (1989). Synovial fluid acidosis correlates with radiological joint destruction in rheumatoid arthritis knee joints. J. Rheumatol. 16 (4), 468–472.
Geng, Z., Wang, X. G., Yu, Y. M., Ji, L., Wang, J., and Liu, C. (2021). Attenuating osteoarthritis by a high efficient anti-bone resorption injectable pH-responsive bisphosphonate-conjugated nano-apatite system. J. Chem. Eng. J. 420, 127674. doi:10.1016/j.cej.2020.127674
Gibbs, A. J., Gray, B., Wallis, J. A., et al. Recommendations for the management of hip and knee osteoarthritis: a systematic review of clinical practice guidelines. Osteoarthr. Cartil. 2023 : S1063–S4584.
Glyn-Jones, S., Palmer, A. J., Agricola, R., Price, A. J., Vincent, T. L., Weinans, H., et al. (2015). Osteoarthr. Lancet 386 (9991), 376–387. doi:10.1016/s0140-6736(14)60802-3
He, M., Qin, Z., Liang, X., He, X., Zhu, B., Lu, Z., et al. (2021). A pH-responsive mesoporous silica nanoparticles-based drug delivery system with controlled release of andrographolide for OA treatment. Regen. Biomater. 8 (4), rbab020. doi:10.1093/rb/rbab020
He, Q., Chen, J., Yan, J., Cai, S., Xiong, H., Liu, Y., et al. (2020). Tumor microenvironment responsive drug delivery systems. Asian J. Pharm. Sci. 15 (4), 416–448. doi:10.1016/j.ajps.2019.08.003
Higashi, H., and Barendregt, J. J. (2011). Cost-effectiveness of total hip and knee replacements for the Australian population with osteoarthritis: discrete-event simulation model. PLoS One 6 (9), e25403. doi:10.1371/journal.pone.0025403
Hu, B., Gao, F., Li, C., Zhang, B., Lu, M., et al. (2020). Rhein laden pH-responsive polymeric nanoparticles for treatment of osteoarthritis. Amb. Express 10 (1), 158. doi:10.1186/s13568-020-01095-3
Hu, Y., Chen, C. H., Ding, Y. Y., Wen, X., Wang, B., Gao, L., et al. (2019). Optimal control nodes in disease-perturbed networks as targets for combination therapy. Nat. Commun. 10 (1), 2180. doi:10.1038/s41467-019-10215-y
Hunter, D. J., and Bierma-Zeinstra, S. (2019). Osteoarthr. Lancet 393 (10182), 1745–1759. doi:10.1016/s0140-6736(19)30417-9
Jiang, Q., and Zhang, S. (2023). Stimulus-responsive drug delivery nanoplatforms for osteoarthritis therapy. Small 19 (23), e2206929. doi:10.1002/smll.202206929
Jin, T., Wu, D., Liu, X. M., Xu, J. T., Ma, B. J., Ji, Y., et al. (2020). Intra-articular delivery of celastrol by hollow mesoporous silica nanoparticles for pH-sensitive anti-inflammatory therapy against knee osteoarthritis. J. Nanobiotechnology 18 (1), 94. doi:10.1186/s12951-020-00651-0
Kang, C., Jung, E., Hyeon, H., Seon, S., and Lee, D. (2020). Acid-activatable polymeric curcumin nanoparticles as therapeutic agents for osteoarthritis. Nanomedicine. 23, 102104. doi:10.1016/j.nano.2019.102104
Kang, L. J., Yoon, J., Rho, J. G., Han, H. S., Lee, S., Oh, Y. S., et al. (2021). Self-assembled hyaluronic acid nanoparticles for osteoarthritis treatment. Biomaterials 275, 120967. doi:10.1016/j.biomaterials.2021.120967
Karimi, M., Ghasemi, A., Sahandi Zangabad, P., Rahighi, R., Moosavi Basri, S. M., Mirshekari, H., et al. (2016). Smart micro/nanoparticles in stimulus-responsive drug/gene delivery systems. Chem. Soc. Rev. 45 (5), 1457–1501. doi:10.1039/c5cs00798d
Katz, J. N. (2021). Platelet-rich plasma for osteoarthritis and achilles tendinitis. JAMA 326 (20), 2012–2014. doi:10.1001/jama.2021.19540
Lan, Q., Lu, R., Chen, H., Pang, Y., Xiong, F., Shen, C., et al. (2020). MMP-13 enzyme and pH responsive theranostic nanoplatform for osteoarthritis. J. Nanobiotechnology 18 (1), 117. doi:10.1186/s12951-020-00666-7
Lavrador, P., Gaspar, V. M., and Mano, J. F. (2018). Stimuli-responsive nanocarriers for delivery of bone therapeutics - barriers and progresses. J. Control Release 273, 51–67. doi:10.1016/j.jconrel.2018.01.021
Lee, G. H., Hwang, J. D., Choi, J. Y., Park, H. J., Kim, K. W., et al. (2011). An acidic pH environment increases cell death and pro-inflammatory cytokine release in osteoblasts: the involvement of BAX inhibitor-1. Int. J. Biochem. Cell. Biol. 43 (9), 1305–1317. doi:10.1016/j.biocel.2011.05.004
Li, Y., Yuan, Z., Yang, H., Zhong, H., Peng, W., and Xie, R. (2021). Recent advances in understanding the role of cartilage lubrication in osteoarthritis. Molecules 26 (20), 6122. doi:10.3390/molecules26206122
Li, Z., Li, G., Xu, J., Li, C., Han, S., Zhang, C., et al. (2022). Hydrogel transformed from nanoparticles for prevention of tissue injury and treatment of inflammatory diseases. Adv. Mater 34 (16), e2109178. doi:10.1002/adma.202109178
Little, C. B., and Hunter, D. J. (2013). Post-traumatic osteoarthritis: from mouse models to clinical trials. Nat. Rev. Rheumatol. 9 (8), 485–497. doi:10.1038/nrrheum.2013.72
Liu, C., Li, C., Pang, C., Li, M., Li, H., Li, P., et al. (2020). Supramolecular drug-drug complex vesicles enable sequential drug release for enhanced combination therapy. ACS Appl. Mater Interfaces 12 (25), 27940–27950. doi:10.1021/acsami.0c04565
Liu, J., Huang, Y., Kumar, A., Tan, A., Jin, S., Mozhi, A., et al. (2014). pH-sensitive nano-systems for drug delivery in cancer therapy. Biotechnol. Adv. 32 (4), 693–710. doi:10.1016/j.biotechadv.2013.11.009
Loeser, R. F., Collins, J. A., and Diekman, B. O. (2016). Ageing and the pathogenesis of osteoarthritis. Nat. Rev. Rheumatol. 12 (7), 412–420. doi:10.1038/nrrheum.2016.65
Lombardi, A. F., Ma, Y., Jang, H., Jerban, S., Tang, Q., Searleman, A., et al. (2022). AcidoCEST-UTE MRI reveals an acidic microenvironment in knee osteoarthritis. Int. J. Mol. Sci. 23 (8), 4466. doi:10.3390/ijms23084466
Mabrouk, M., Das, D. B., Salem, Z. A., and Beherei, H. H. (2021). Nanomaterials for biomedical applications: production, characterisations, recent trends and difficulties. Molecules 26 (4), 1077. doi:10.3390/molecules26041077
Majumder, J., and Minko, T. (2021). Multifunctional and stimuli-responsive nanocarriers for targeted therapeutic delivery. Expert Opin. Drug Deliv. 18 (2), 205–227. doi:10.1080/17425247.2021.1828339
Mao, L., Wu, W., Wang, M., Guo, J., Li, H., Zhang, S., et al. (2021). Targeted treatment for osteoarthritis: drugs and delivery system. Drug Deliv. 28 (1), 1861–1876. doi:10.1080/10717544.2021.1971798
Martel-Pelletier, J., Barr, A. J., Cicuttini, F. M., Conaghan, P. G., Cooper, C., Goldring, M. B., et al. (2016). Osteoarthritis. Nat. Rev. Dis. Prim. 2, 16072. doi:10.1038/nrdp.2016.72
Matsuyama, S., Llopis, J., Deveraux, Q. L., Tsien, R. Y., and Reed, J. C. (2000). Changes in intramitochondrial and cytosolic pH: early events that modulate caspase activation during apoptosis. Nat. Cell. Biol. 2 (6), 318–325. doi:10.1038/35014006
Mehana, E. E., Khafaga, A. F., and El-Blehi, S. S. (2019). The role of matrix metalloproteinases in osteoarthritis pathogenesis: an updated review. Life Sci. 234, 116786. doi:10.1016/j.lfs.2019.116786
Mitragotri, S., and Lahann, J. (2009). Physical approaches to biomaterial design. Nat. Mater 8 (1), 15–23. doi:10.1038/nmat2344
Municoy, S., Álvarez Echazú, M. I., Antezana, P. E., Galdopórpora, J. M., Olivetti, C., Mebert, A. M., et al. (2020). Stimuli-responsive materials for tissue engineering and drug delivery. Int. J. Mol. Sci. 21 (13), 4724. doi:10.3390/ijms21134724
Mura, S., Nicolas, J., and Couvreur, P. (2013). Stimuli-responsive nanocarriers for drug delivery. Nat. Mater 12 (11), 991–1003. doi:10.1038/nmat3776
Muro, S. (2012). Challenges in design and characterization of ligand-targeted drug delivery systems. J. Control Release 164 (2), 125–137. doi:10.1016/j.jconrel.2012.05.052
Nelson, A. E., Allen, K. D., Golightly, Y. M., Goode, A. P., and Jordan, J. M. (2014). A systematic review of recommendations and guidelines for the management of osteoarthritis: the chronic osteoarthritis management initiative of the U.S. bone and joint initiative. Semin. Arthritis Rheum. 43 (6), 701–712. doi:10.1016/j.semarthrit.2013.11.012
Noble, G. T., Stefanick, J. F., Ashley, J. D., Kiziltepe, T., and Bilgicer, B. (2014). Ligand-targeted liposome design: challenges and fundamental considerations. Trends Biotechnol. 32 (1), 32–45. doi:10.1016/j.tibtech.2013.09.007
Nygaard, G., and Firestein, G. S. (2020). Restoring synovial homeostasis in rheumatoid arthritis by targeting fibroblast-like synoviocytes. Nat. Rev. Rheumatol. 16 (6), 316–333. doi:10.1038/s41584-020-0413-5
Orchard, J. W. (2020). Is there a place for intra-articular corticosteroid injections in the treatment of knee osteoarthritis. BMJ 368, l6923. doi:10.1136/bmj.l6923
Raza, A., Rasheed, T., Nabeel, F., Hayat, U., Bilal, M., and Iqbal, H. (2019). Endogenous and exogenous stimuli-responsive drug delivery systems for programmed site-specific release. Molecules 24 (6), 1117. doi:10.3390/molecules24061117
Ruiz, D. Jr, Koenig, L., Dall, T. M., Gallo, P., Narzikul, A., Parvizi, J., et al. (2013). The direct and indirect costs to society of treatment for end-stage knee osteoarthritis. J. Bone Jt. Surg. Am. 95 (16), 1473–1480. doi:10.2106/jbjs.l.01488
Schlossbauer, A., Dohmen, C., Schaffert, D., Wagner, E., and Bein, T. (2011). pH-responsive release of acetal-linked melittin from SBA-15 mesoporous silica. Angew. Chem. Int. Ed. Engl. 50 (30), 6828–6830. doi:10.1002/anie.201005120
Sonawane, S. J., Kalhapure, R. S., and Govender, T. (2017). Hydrazone linkages in pH responsive drug delivery systems. Eur. J. Pharm. Sci. 99, 45–65. doi:10.1016/j.ejps.2016.12.011
Su, W. T., Huang, C. C., and Liu, H. W. (2022). Evaluation and preparation of a designed kartogenin drug delivery system (DDS) of hydrazone-linkage-based pH responsive mPEG-Hz-b-PCL nanomicelles for treatment of osteoarthritis. Front. Bioeng. Biotechnol. 10, 816664. doi:10.3389/fbioe.2022.816664
Treuhaft, P. S., and Mccarty, D. J. (1971). Synovial fluid pH, lactate, oxygen and carbon dioxide partial pressure in various joint diseases. Arthritis Rheum. 14 (4), 475–484. doi:10.1002/art.1780140407
Vaupel, P., Kallinowski, F., and Okunieff, P. (1989). Blood flow, oxygen and nutrient supply, and metabolic microenvironment of human tumors: a review. Cancer Res. 49 (23), 6449–6465.
Wang, J., Mao, W., Lock, L. L., Tang, J., Sui, M., Sun, W., et al. (2015). The role of micelle size in tumor accumulation, penetration, and treatment. ACS Nano 9 (7), 7195–7206. doi:10.1021/acsnano.5b02017
Wang, Z., Zhong, Y., He, S., Liang, R., Liao, C., Zheng, L., et al. (2022). Application of the pH-responsive PCL/PEG-Nar nanofiber membrane in the treatment of osteoarthritis. Front. Bioeng. Biotechnol. 10, 859442. doi:10.3389/fbioe.2022.859442
Webb, B. A., Chimenti, M., Jacobson, M. P., and Barber, D. L. (2011). Dysregulated pH: a perfect storm for cancer progression. Nat. Rev. Cancer 11 (9), 671–677. doi:10.1038/nrc3110
Xiong, F., Qin, Z., Chen, H., Lan, Q., Wang, Z., Lan, N., et al. (2020). pH-responsive and hyaluronic acid-functionalized metal-organic frameworks for therapy of osteoarthritis. J. Nanobiotechnology 18 (1), 139. doi:10.1186/s12951-020-00694-3
Xiong, H., Zhao, Y., Xu, Q., Xie, X., Wu, J., Hu, B., et al. (2022). Biodegradable hollow-structured nanozymes modulate phenotypic polarization of macrophages and relieve hypoxia for treatment of osteoarthritis. Small 18 (32), e2203240. doi:10.1002/smll.202203240
Xue, S., Zhou, X., Sang, W., Wang, C., Lu, H., Xu, Y., et al. (2021). Cartilage-targeting peptide-modified dual-drug delivery nanoplatform with NIR laser response for osteoarthritis therapy. Bioact. Mater 6 (8), 2372–2389. doi:10.1016/j.bioactmat.2021.01.017
Yang, W., Cao, Y., Zhang, Z., Du, F., Shi, Y., Li, X., et al. (2018). Targeted delivery of FGF2 to subchondral bone enhanced the repair of articular cartilage defect. Acta Biomater. 69, 170–182. doi:10.1016/j.actbio.2018.01.039
Yang, X., Grailer, J. J., Rowland, I. J., Javadi, A., Hurley, S. A., Matson, V. Z., et al. (2010). Multifunctional stable and pH-responsive polymer vesicles formed by heterofunctional triblock copolymer for targeted anticancer drug delivery and ultrasensitive MR imaging. ACS Nano 4 (11), 6805–6817. doi:10.1021/nn101670k
Yu, G., Wu, D., Li, Y., Zhang, Z., Shao, L., Zhou, J., et al. (2016). A pillar[5]arene-based [2]rotaxane lights up mitochondria. Chem. Sci. 7 (5), 3017–3024. doi:10.1039/c6sc00036c
Yu, H., Ren, P., Pan, X., Zhang, X., Ma, J., Chen, J., et al. (2023). Intracellular delivery of itaconate by metal-organic framework-anchored hydrogel microspheres for osteoarthritis therapy. Pharmaceutics 15 (3), 724. doi:10.3390/pharmaceutics15030724
Zerrillo, L., Que, I., Vepris, O., Morgado, L., Chan, A., Bierau, K., et al. (2019). pH-responsive poly(lactide-co-glycolide) nanoparticles containing near-infrared dye for visualization and hyaluronic acid for treatment of osteoarthritis. J. Control Release 309, 265–276. doi:10.1016/j.jconrel.2019.07.031
Zhang, M., Hu, W., Cai, C., Wu, Y., Li, J., and Dong, S. (2022). Advanced application of stimuli-responsive drug delivery system for inflammatory arthritis treatment. Mater Today Bio 14, 100223. doi:10.1016/j.mtbio.2022.100223
Zhang, M., Peng, X., Ding, Y., Ke, X., Ren, K., Xin, Q., et al. (2023b). A cyclic brush zwitterionic polymer based pH-responsive nanocarrier-mediated dual drug delivery system with lubrication maintenance for osteoarthritis treatment. Mater Horiz. 10, 2554–2567. doi:10.1039/d3mh00218g
Zhang, Z. J., Hou, Y. K., Chen, M. W., Yu, X. Z., Chen, S. Y., Yue, Y. R., et al. (2023a). A pH-responsive metal-organic framework for the co-delivery of HIF-2α siRNA and curcumin for enhanced therapy of osteoarthritis. J. Nanobiotechnology 21 (1), 18. doi:10.1186/s12951-022-01758-2
Zhao, C., Chen, J., Ye, J., Li, Z., Su, L., Wang, J., et al. (2021). Structural transformative antioxidants for dual-responsive anti-inflammatory delivery and photoacoustic inflammation imaging. Angew. Chem. Int. Ed. Engl. 60 (26), 14458–14466. doi:10.1002/anie.202100873
Zhao, Y., Luo, Z., Li, M., Qu, Q., Ma, X., Yu, S., et al. (2015). A preloaded amorphous calcium carbonate/doxorubicin@silica nanoreactor for pH-responsive delivery of an anticancer drug. Angew. Chem. Int. Ed. Engl. 54 (3), 919–922. doi:10.1002/anie.201408510
Zhen, G., and Cao, X. (2014). Targeting TGFβ signaling in subchondral bone and articular cartilage homeostasis. Trends Pharmacol. Sci. 35 (5), 227–236. doi:10.1016/j.tips.2014.03.005
Zhou, M., Wen, K., Bi, Y., Lu, H., Chen, J., Hu, Y., et al. (2017). The application of stimuli-responsive nanocarriers for targeted drug delivery. Curr. Top. Med. Chem. 17 (20), 2319–2334. doi:10.2174/1568026617666170224121008
Keywords: osteoarthritis, nanoparticles, pH responsive, intra-articular injection, drug delivery
Citation: Liao S, Jia S, Yue Y, Zeng H, Lin J and Liu P (2024) Advancements in pH-Responsive nanoparticles for osteoarthritis treatment: Opportunities and challenges. Front. Bioeng. Biotechnol. 12:1426794. doi: 10.3389/fbioe.2024.1426794
Received: 02 May 2024; Accepted: 06 June 2024;
Published: 01 July 2024.
Edited by:
Bing Guo, Harbin Institute of Technology, Shenzhen, ChinaReviewed by:
Jindan Wu, Zhejiang Sci-Tech University, ChinaQingxian Li, First Affiliated Hospital of Xiamen University, China
Copyright © 2024 Liao, Jia, Yue, Zeng, Lin and Liu. This is an open-access article distributed under the terms of the Creative Commons Attribution License (CC BY). The use, distribution or reproduction in other forums is permitted, provided the original author(s) and the copyright owner(s) are credited and that the original publication in this journal is cited, in accordance with accepted academic practice. No use, distribution or reproduction is permitted which does not comply with these terms.
*Correspondence: Hui Zeng, emVuZ2h1aUBwa3VzemguY29t; Peng Liu, bGl1cGVuZ19wb2x5bWVyQDEyNi5jb20=; Jianjin Lin, bGluamlhbmppbmdAYmptdS5lZHUuY24=
†These authors share first authorship
‡These authors share last authorship