- 1Musculoskeletal Tumor Center, Peking University People’s Hospital, Beijing, China
- 2Beijing Key Laboratory of Musculoskeletal Tumor, Beijing, China
- 3Beijing AK Medical Co., Ltd., Beijing, China
Introduction: Femur and tibia are the most commonly affected sites for primary malignant bone tumors in children. The wide resection of the tumor frequently requires the physis to be resected. The normal growth of the unaffected limb will result in a significant limb length discrepancy at skeletal maturity. To compensate for this resulting LLD, different generations of extendible endoprostheses have been developed. Non-invasive extendable prostheses eliminate the need for surgical procedures and general anesthesia, enabling gradual and painless lengthening. Currently available non-invasive extendable prostheses focus on joint reconstruction, and no case series analysis of intercalary non-invasive extendable prosthesis has been reported. Therefore, we have designed a novel non-invasive electromagnetic extendable intercalary endoprosthesis.
Methods: In vitro mechanical experiments and in vivo animal experiments were conducted.
Results: In vitro experiments have confirmed that the prosthetics can extend at a constant rate, increasing by 4.4 mm every 10 min. The average maximum extension force during prosthetic elongation can reach 1306N. In animal in vivo experiments, the extension process is smooth and non-invasive, and the sheep is in a comfortable state.
Discussion: The in vitro and in vivo animal studies provide evidence to support the extension reliability, laying the foundation for future large-scale validation experiments.
Introduction
Osteosarcoma and Ewing sarcoma are the most common primary malignant bone tumor in children. The incidence accounts for approximately 10% of all malignant tumors in children. The most commonly affected sites are the femur and tibia. Limb salvage surgery has now become the main treatment for patients with bone tumors (Weitao et al., 2012). Tumors frequently involve the distal femur or proximal tibia, thus requiring the resection of the physis or growth plates. The normal growth of the unaffected limb will result in a significant limb length discrepancy (LLD) at skeletal maturity. Different generations of the extendible endoprosthesis (EPR) have been developed to compensate for the resulting LLD (Huang et al., 2023).
Invasive extendable prostheses can be extended through an open invasive surgical procedure. Modular constructs are believed to provide the benefit of enhanced structural integrity of the prosthesis during maximum lengthening. The drawback lies in the necessity for a surgical procedure with extensive exposure for each lengthening event. This may result in excessive scar tissue formation and an elevated susceptibility to infection. Minimally invasive extendable prostheses can be extended through nail-tract incisions. Nevertheless, the drawbacks include the potential for expansion mechanism failure, prosthesis failure at maximum lengthening, and postoperative nail-tract infections (Nystrom and Morcuende, 2010). The latest non-invasive extendable prosthesis, eliminates the need for surgical procedures and general anesthesia to facilitate lengthening. Stanmore JTS noninvasive prosthesis (produced by Stanmore Implants Worldwide Ltd., UK) features an internal lengthening mechanism activated by an external electromagnetic field, enabling smooth and painless lengthening can be undertaken in the outpatient clinic (Dukan et al., 2022).
With the improvement of preoperative imaging examination and surgical osteotomy accuracy, some patients who previously needed joint replacement can now undergo joint preservation surgery (Guder et al., 2017; Takeuchi et al., 2019; Li et al., 2023). Especially, with advancement of 3D printing technology which enables manufacture highly custom-made endoprosthesis and metallic porous structure which facilitating bone ingrowth, joint preservation limb salvage for bone tumor resection increased in recent years (Li et al., 2023). Available non-invasive extendable prostheses focus on joint reconstruction, and no case series analysis of intercalary non-invasive extendable prosthesis has been reported. Thus, we design a novel, non-invasive electromagnetic extendable intercalary endoprosthesis.
The endoprosthesis shaft consists of two parts that slide relatively during the lengthening procedure (Figure 1). The two stemmed ends of the endoprosthesis are secured into the remaining bone segment using cement. The main shaft includes a magnetic disc, a gearbox, and a power screw. The magnetic disc is connected to the gearbox, while the gearbox is connected to a threaded screw that links to the inner telescopic segment. When the screw rotates, it causes the inner and outer segments of the telescoping shaft to separate and extending. The magnetic disc rotates when the prosthesis is placed in a rotating electromagnetic field. The external drive unit creates the rotating electromagnetic field by a power unit, which operates from a single-phase 220-V power source. To verify the extension mechanism and mechanical stability of the prosthesis, both in vitro and in vivo animal experiments were conducted.
Materials and methods
In vitro experiment
The in vitro mechanical experiments were conducted to investigate the extension rate and maximum extension force. The prosthesis was put at the center of the external drive unit (Figure 2A). Extension operation was started by launching the external drive unit. The magnetic generator was stopped every 10 min, and the extension length was measured. The experiment was repeated 6 times. Linear regression was used to calculate the relationship between the extension time and extension length. Then, the prosthesis was placed in the extension force testing device (Figure 2B). The maximum extension force at intervals of 5 mm for each extended length was recorded. The experiment was repeated 6 times.
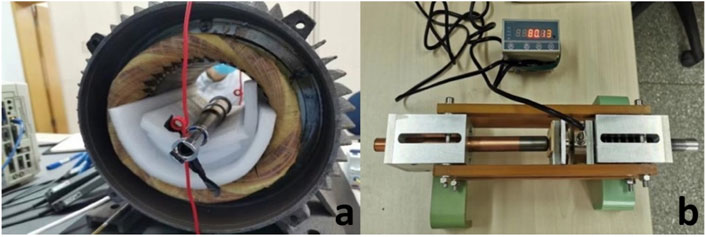
Figure 2. (A), endoprosthesis placed in the center of the external drive unit; (B), extension force testing device.
In vivo experiment
Sheep provide a reliable surrogate for human clinical conditions, with bone mineral composition, macro- and microstructure, remodeling capacity, and biomechanics very similar to those of humans. The load on the entire hind limbs of sheep is approximately half of the load encountered by humans during a gait cycle (Reichert et al., 2009). Therefore, we chose small-tailed Han sheep weighing about 50 Kg for the experiment.
The intercalary prosthesis was designed based on sheep CT scan data and simulated mid-shaft defects in the tibia. To induce anesthesia, a single intravenous dose of 5 mg/kg 1% Provive was injected. Then tracheal intubation was performed and a respirator machine was connected. 2% isoflurane in a mixture of oxygen/air (40:60) was used to maintain anesthesia. After general anesthesia, the sheep were placed supinely on the operation table using bandage fixation. An intercalary bone defect of 16 cm was made on the tibia and was reconstructed with the intercalary prosthesis. The stems at both ends of the prosthesis were fixed to the tibia through bone cement. Lengthening and shortening tests were performed immediately post-surgery, at 4 weeks post-surgery, and at 12 weeks post-surgery. The sheep limb was X-rayed during the assessment period to ensure the length of the prosthesis. The sheep were euthanized at 12 weeks post-surgery. The method of euthanasia for sheep was excessive intravenous injection of pentobarbital. The prosthesis was retrieved. HE staining slices were made from the bone callus around the prosthesis.
Results
In vitro experiment
The fitted regression equation of extension time (T/minute) and extension length (L/mm) was as follows: L = 0.4408T + 0.0425 (Figure 3). The regression coefficient was 0.9993. Under the rotating magnetic field, the prosthesis was extended by 4.4 mm every 10 min. The high regression coefficient indicated that the extension process was very smooth. The average maximum extension force was 1,306 ± 67N (Figure 4), which is sufficient to counteract the tension of soft tissue and extend by approximately 5 mm both in sheep and human (Meswania et al., 1998).
In vivo experiment
After the surgery, the prosthesis was extended a 1.8 mm-length within 4 min. Then, we shortened the prosthesis to its initial length to reduce the wound tension. The sound of the gearbox could be heard through a stethoscope placed on the surface of the sheep’s leg in order to monitor the working status of the gearbox (rotating of the magnetic disc) (Supplementary Material S1). The wound healed well, and the sheep gradually resumed walking.
In the second lengthening procedure, the prosthesis was extended for 4.4 mm, with the lengthening process taking 10 min. Subsequently, the prosthesis was shortened for 3.2 mm. The net elongation in this experiment was 1.2 mm (Figure 5). Then the sheep was allowed to ambulate freely.
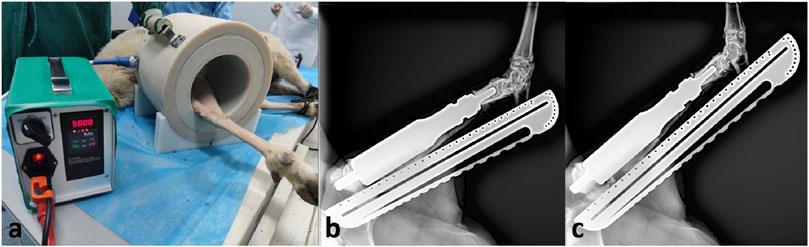
Figure 5. Lengthening procedure at 4 weeks after surgery. (A), the sheep leg was placed in the device; (B), radiograph before lengthening; (C), radiograph after lengthening.
In the third lengthening process, the prosthesis was extended for 4.4 mm. The total elongation of the endoprosthesis was 5.6 mm. The prosthesis has been functioning normally during three lengthening process. The affected limb of the sheep could bear weight and walk. No complication of the prosthesis has been observed. There were no metal particles or foreign body granulomas in the HE slices (Figure 6).
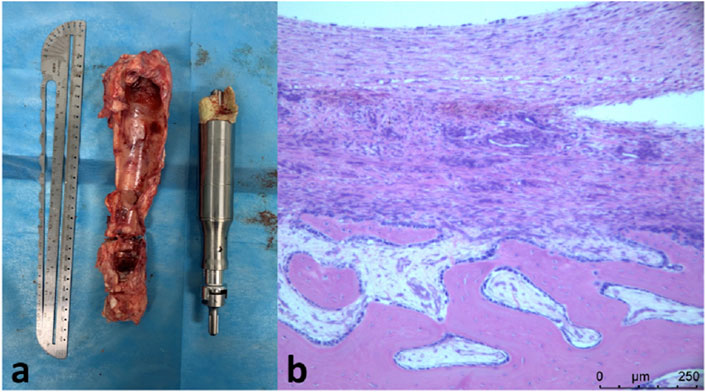
Figure 6. (A), retrieved prosthesis and bone callus; (B), H&E staining showing newly-formed bone callus with no metal particles or foreign body granulomas.
Discussion
Osteosarcoma and Ewing sarcoma are the most common primary malignant bone tumor in children. Tumors typically grow at the metaphyseal ends of long bones such as the distal femur and proximal tibia (Andreou et al., 2015; Ferguson and Turner, 2018). Limb salvage surgery has now become the main treatment for patients with bone tumors (Grinberg et al., 2020). When the joint surface is not invaded, we can excise the tumor-affected bone segment to preserve the joint. The reconstruction of bone defects mainly involves two methods. One is non-biological reconstruction, which typically involves the use of prosthetics (Smolle et al., 2019). The other method is biological reconstruction (such as allograft (Zucchini et al., 2022), vascularized (Hsu et al., 1997) or nonvascularized fibular graft (Kaewpornsawan and Eamsobhana, 2017), distraction osteogenesis (Lesensky and Prince, 2017), recycling tumor-bearing autograft (Tsuchiya et al., 2005)). Due to the inherent growth potential of the unaffected limb being higher than that of the affected limb, patients may experience a discrepancy in limb length when the bones mature.
The following method is commonly used to address postoperative limb length discrepancies in patients. The initial approach involves combining epiphysiodesis fixation with distraction osteogenesis and providing long-term support and protection using an external fixator (Watanabe et al., 2013). This method is direct and effective, but it often presents issues such as extensive trauma, prolonged recovery periods, postoperative infections, nonunion, and, most significantly, a higher risk of nerve damage, which contradicts the philosophy of limb salvage treatment. However, it cannot be denied that this procedure effectively resolves postoperative limb length discrepancies and maximally preserves the patient’s own bones (Scales, 1983). The first-generation lengthening prosthesis was designed by Dr. Scales and Stanmore in 1976. This prosthesis requires open surgery, which entails significant trauma, causing considerable discomfort, especially for patients who require multiple lengthening procedures (Lex et al., 2021). A related study indicates that the infection of the prosthesis and postoperative complications, such as loosening of the prosthesis, is relatively high (Schindler et al., 1997). The second generation of lengthening prostheses is minimally invasive. Its unique extendable screw structure allows the lengthening procedure to be completed through a small incision, but similar to the criticisms of the first-generation lengthening prostheses, it still requires the procedure to be performed in the operating room under anesthesia (Unwin and Walker, 1996). The latest generation of lengthening prostheses is designed to minimize trauma to patients even further. These prostheses contain telescopic segments that can slide past each other when placed in a specific condition, such as an electromagnetic field. By placing the prosthesis in a rotating electromagnetic field, the lengthening procedure can be performed. The lengthening is steady and painless, without the need for anesthesia or an incision (Dotan et al., 2010). Compared to the previous two generations of lengthening prostheses, the design of non-invasive extendable prostheses greatly reduces issues such as the increased risk of infection associated with surgical procedures. Additionally, it alleviates pain during the lengthening process and reduces the anxiety patients may experience when undergoing the lengthening procedure (Jeys et al., 2005; Henderson et al., 2011).
Here are several non-invasive expandable prostheses that have been applied clinically, along with the results of clinical follow-up: Wilkins RM et al. studied Phoenix expandable prosthesis. Seven Phoenix prostheses were used to reconstruct bone defects in six patients. After the implantation of the prostheses, a total of 21 extension procedures were performed in six patients. According to statistics, the average extension time was 20–30 s, with an average extension amount of 8 mm. No patients experienced acute complications during the extension procedure, but most patients still require oral analgesics during the extension process (Wilkins and Soubeiran, 2001). However, it has already been phased out by the market. Said Saghieh and colleagues reported their 7-year experience using the Repiphysis® implant—all patients undergoing non-invasive lengthening procedures achieved successful elongation. But more than two-thirds of patients have developed complications, which mainly focus on infections and mechanical issues (Saghieh et al., 2010). The Juvenile Tumour System (JTS; Stanmore Implants Worldwide, Stanmore, United Kingdom) was another type of non-invasive extendible prosthesis and was first used in 2002. Hwang et al. conducted a study on 34 pediatric patients using the Stanmore prosthesis for defect reconstruction. Their early results suggest that the Stanmore prosthesis can be lengthened non-surgically. However, there still exists a relatively high infection rate (Hwang et al., 2012).
We have developed a novel non-invasive electromagnetic extendable intercalary endoprosthesis, and conducted in vitro and in vivo animal validation. There is no case series analysis of intercalary non-invasive extendable prosthesis. The application of 3 Stanmore JTS non-invasive extendable intercalary prostheses was reported (Gundavda and Agarwal, 2019). Both the novel prosthesis and JTS prosthesis utilize electromagnetic drive technology. However, the gear reduction ratio of the novel prosthesis is different from the JTS prosthesis. The magnet and gearbox of the novel prosthesis are fully sealed within a titanium alloy chamber, which has passed rigorous in vitro testing and meets national standards, whereas the JTS prosthesis does not have this sealed structure. The biocompatibility of the prosthesis is not the main focus of this study. Therefore, no serum metal ion test was conducted after long-term animal feeding. In vivo experiments on biocompatibility and ion release can be further refined in subsequent experiments.
The study confirmed the reliability and usability of the endoprosthesis. According to Meswania’s publication (Meswania et al., 1998), at 6 mm extension the load on the prosthesis due to soft tissues and muscles in human varied between 42 N and 1513 N with a mean of 476 N. There was a linear relationship between extension and load. The average maximum extension force of the novel prosthesis is 1,306 ± 67 N, which is sufficient to counteract the tension of soft tissue and extend by approximately 5 mm. The in vitro and in vivo animal studies provide evidence to support the extension reliability, laying the foundation for future large-scale validation experiments.
Data availability statement
The raw data supporting the conclusions of this article will be made available by the authors, without undue reservation.
Ethics statement
The animal study was approved by the Animal Ethics Committee of Peking University People’s Hospital. The study was conducted in accordance with the local legislation and institutional requirements.
Author contributions
SH: Data curation, Methodology, Writing–original draft, Writing–review and editing. JY: Writing–original draft, Writing–review and editing. XL: Methodology, Writing–original draft, Writing–review and editing. XT: Supervision, Writing–original draft, Writing–review and editing. TJ: Conceptualization, Funding acquisition, Supervision, Validation, Writing–original draft, Writing–review and editing.
Funding
The author(s) declare that financial support was received for the research, authorship, and/or publication of this article. Peking University People’s Hospital Scientific Research Development Funds (RDL2023-01, RDJP2022-55) Beijing Natural Science Foundation (L234063) Capital Medical Science and Technology Innovation Promotion Plan (YC202301QX0066).
Conflict of interest
Author XL was employed by Beijing AK Medical Co., Ltd.
The remaining authors declare that the research was conducted in the absence of any commercial or financial relationships that could be construed as a potential conflict of interest.
The author(s) declared that they were an editorial board member of Frontiers, at the time of submission. This had no impact on the peer review process and the final decision.
Publisher’s note
All claims expressed in this article are solely those of the authors and do not necessarily represent those of their affiliated organizations, or those of the publisher, the editors and the reviewers. Any product that may be evaluated in this article, or claim that may be made by its manufacturer, is not guaranteed or endorsed by the publisher.
Supplementary material
The Supplementary Material for this article can be found online at: https://www.frontiersin.org/articles/10.3389/fbioe.2024.1400428/full#supplementary-material
References
Andreou, D., Hardes, J., Gosheger, G., Henrichs, M. P., Nottrott, M., and Streitbürger, A. (2015). Interdisciplinary diagnostic and treatment of bone sarcomas of the extremities and trunk. Handchir Mikrochir Plast. Chir. 47 (2), 90–99. doi:10.1055/s-0034-1396853
Dotan, A., Dadia, S., Bickels, J., Nirkin, A., Flusser, G., Issakov, J., et al. (2010). Expandable endoprosthesis for limb-sparing surgery in children: long-term results. J. Child. Orthop. 4 (5), 391–400. doi:10.1007/s11832-010-0270-x
Dukan, R., Mascard, E., Langlais, T., Ouchrif, Y., Glorion, C., Pannier, S., et al. (2022). Long-term outcomes of non-invasive expandable endoprostheses for primary malignant tumors around the knee in skeletally-immature patients. Arch. Orthop. Trauma Surg. 142 (6), 927–936. doi:10.1007/s00402-020-03712-z
Ferguson, J. L., and Turner, S. P. (2018). Bone cancer: Diagnosis and treatment principles. Am. Fam. Physician 98 (4), 205–213.
Grinberg, S. Z., Posta, A., Weber, K. L., and Wilson, R. J. (2020). Limb salvage and reconstruction options in osteosarcoma. Adv. Exp. Med. Biol. 1257, 13–29. doi:10.1007/978-3-030-43032-0_2
Guder, W. K., Hardes, J., Gosheger, G., Nottrott, M., and Streitbürger, A. (2017). Ultra-short stem anchorage in the proximal tibial epiphysis after intercalary tumor resections: analysis of reconstruction survival in four patients at a mean follow-up of 56 months. Archives Orthop. Trauma Surg. 137 (4), 481–488. doi:10.1007/s00402-017-2637-7
Gundavda, M. K., and Agarwal, M. G. (2019). Growing without pain: the noninvasive expandable prosthesis is boon for children with bone cancer, as well as their surgeons!. Indian J. Orthop. 53, 174–182. doi:10.4103/ortho.ijortho_53_17
Henderson, E. R., Groundland, J. S., Pala, E., Dennis, J. A., Wooten, R., Cheong, D., et al. (2011). Failure mode classification for tumor endoprostheses: retrospective review of five institutions and a literature review. J. Bone Jt. Surg. Am. 93 (5), 418–429. doi:10.2106/jbjs.j.00834
Hsu, R. W., Wood, M. B., Sim, F. H., and Chao, E. Y. S. (1997). Free vascularised fibular grafting for reconstruction after tumour resection. J. Bone Jt. Surg. Br. 79 (1), 36–42. doi:10.1302/0301-620x.79b1.0790036
Huang, J., Cheng, J., Bi, W., Xu, M., Jia, J., Han, G., et al. (2023). Neoadjuvant chemotherapy and expandable prosthesis reconstruction to treat osteosarcoma around the knee in children. Orthop. Surg. 15 (1), 162–168. doi:10.1111/os.13563
Hwang, N., Grimer, R. J., Carter, S. R., Tillman, R. M., Abudu, A., and Jeys, L. M. (2012). Early results of a non-invasive extendible prosthesis for limb-salvage surgery in children with bone tumours. J. Bone Jt. Surg. Br. 94 (2), 265–269. doi:10.1302/0301-620x.94b2.27536
Jeys, L. M., Grimer, R., Carter, S., and Tillman, R. (2005). Periprosthetic infection in patients treated for an orthopaedic oncological condition. J. Bone Jt. Surg. Am. 87 (4), 842–849. doi:10.2106/jbjs.c.01222
Kaewpornsawan, K., and Eamsobhana, P. (2017). Free non-vascularized fibular graft for treatment of large bone defect around the elbow in pediatric patients. Eur. J. Orthop. Surg. Traumatol. 27 (7), 895–900. doi:10.1007/s00590-017-1955-3
Lesensky, J., and Prince, D. E. (2017). Distraction osteogenesis reconstruction of large segmental bone defects after primary tumor resection: pitfalls and benefits. Eur. J. Orthop. Surg. Traumatol. 27 (6), 715–727. doi:10.1007/s00590-017-1998-5
Lex, J. R., Adlan, A., Tsoi, K., Evans, S., and Stevenson, J. D. (2021). Frequency and reason for reoperation following non-invasive expandable endoprostheses: a systematic review. J. Bone Oncol. 31, 100397. doi:10.1016/j.jbo.2021.100397
Li, Y., Xu, H., Shan, H., Ma, K., Liu, W., and Niu, X. (2023). A comparative study of reconstruction modalities after knee joint-preserving tumor resection: reconstruction with a custom-made endoprosthesis versus reconstruction with a liquid nitrogen-inactivated autologous bone graft. J. Orthop. Surg. Res. 18 (1), 908. doi:10.1186/s13018-023-04402-3
Meswania, J. M., Walker, P. S., Sneath, R. S., and Grimer, R. J. (1998). In vivo distraction forces in extendible endoprosthetic replacement—a study of 34 patients. Proc. Inst. Mech. Eng. H. 212 (3), 151–155. doi:10.1243/0954411981533926
Nystrom, L. M., and Morcuende, J. A. (2010). Expanding endoprosthesis for pediatric musculoskeletal malignancy: current concepts and results. Iowa Orthop. J. 30, 141–149.
Reichert, J. C., Saifzadeh, S., Wullschleger, M. E., Epari, D. R., Schütz, M. A., Duda, G. N., et al. (2009). The challenge of establishing preclinical models for segmental bone defect research. Biomaterials 30 (12), 2149–2163. doi:10.1016/j.biomaterials.2008.12.050
Saghieh, S., Abboud, M. R., Muwakkit, S. A., Saab, R., Rao, B., and Haidar, R. (2010). Seven-year experience of using Repiphysis® expandable prosthesis in children with bone tumors. Pediatr. Blood Cancer. 55 (3), 457–463. doi:10.1002/pbc.22598
Scales, J. T. (1983). Bone and joint replacement for the preservation of limbs. Br. J. Hosp. Med. 30 (4), 220–232.
Schindler, O. S., Cannon, S. R., Briggs, T. W. R., and Blunn, G. W. (1997). Stanmore custom-made extendible distal femoral replacements. Clinical experience in children with primary malignant bone tumours. J. Bone Jt. Surg. Br. 79 (6), 927–937. doi:10.1302/0301-620x.79b6.7164
Smolle, M. A., Andreou, D., Tunn, P. U., and Leithner, A. (2019). Advances in tumour endoprostheses: a systematic review. EFORT Open Rev. 4 (7), 445–459. doi:10.1302/2058-5241.4.180081
Takeuchi, A., Yamamoto, N., Hayashi, K., Matsubara, H., Miwa, S., Igarashi, K., et al. (2019). Joint-preservation surgery for pediatric osteosarcoma of the knee joint. Cancer Metastasis Rev. 38 (4), 709–722. doi:10.1007/s10555-019-09835-z
Tsuchiya, H., Wan, S. L., Sakayama, K., Yamamoto, N., Nishida, H., and Tomita, K. (2005). Reconstruction using an autograft containing tumour treated by liquid nitrogen. J. Bone Jt. Surg. Br. 87 (2), 218–225. doi:10.1302/0301-620x.87b2.15325
Unwin, P. S., and Walker, P. S. (1996). Extendible endoprostheses for the skeletally immature. Clin. Orthop. Relat. Res. 322 (322), 179–193. doi:10.1097/00003086-199601000-00023
Watanabe, K., Tsuchiya, H., Yamamoto, N., Shirai, T., Nishida, H., Hayashi, K., et al. (2013). Over 10-year follow-up of functional outcome in patients with bone tumors reconstructed using distraction osteogenesis. J. Orthop. Sci. 18 (1), 101–109. doi:10.1007/s00776-012-0327-4
Weitao, Y., Qiqing, C., Songtao, G., and Jiaqiang, W. (2012). Epiphysis preserving operations for the treatment of lower limb malignant bone tumors. Eur. J. Surg. Oncol. 38 (12), 1165–1170. doi:10.1016/j.ejso.2012.05.005
Wilkins, R. M., and Soubeiran, A. (2001). The Phenix expandable prosthesis: early American experience. Clin. Orthop. Relat. Res. 382 (382), 51–58. doi:10.1097/00003086-200101000-00009
Zucchini, R., Staals, E. L., Fiore, M., Campanacci, L., Giannini, C., Manfrini, M., et al. (2022). Intercalary reconstruction of the distal femur with or without physeal preservation: results and impact on limb growth. Eur. J. Orthop. Surg. Traumatol. 32 (8), 1651–1659. doi:10.1007/s00590-021-03149-z
Keywords: extendable endoprosthesis, intercalary endoprosthesis, electromagnetic drive, limb length discrepancy, non-invasive extendable endoprothesis
Citation: Huang S, Yang J, Li X, Tang X and Ji T (2024) A novel non-invasive electromagnetic extendable intercalary endoprosthesis: a proof-of-concept study. Front. Bioeng. Biotechnol. 12:1400428. doi: 10.3389/fbioe.2024.1400428
Received: 13 March 2024; Accepted: 26 June 2024;
Published: 18 July 2024.
Edited by:
Chao Zhao, University of Alabama, United StatesReviewed by:
Hong Duan, Sichuan University, ChinaManish Agarwal, Nanavati max superspecialty hospital, India
Copyright © 2024 Huang, Yang, Li, Tang and Ji. This is an open-access article distributed under the terms of the Creative Commons Attribution License (CC BY). The use, distribution or reproduction in other forums is permitted, provided the original author(s) and the copyright owner(s) are credited and that the original publication in this journal is cited, in accordance with accepted academic practice. No use, distribution or reproduction is permitted which does not comply with these terms.
*Correspondence: Tao Ji, jitaomd@163.com