- 1Institute of Kinesiology, Faculty of Sport, University of Ljubljana, Ljubljana, Slovenia
- 2Science and Research Centre Koper, Institute for Kinesiology Research, Koper, Slovenia
- 3Faculty of Sports Studies, Masaryk University, Brno, Czechia
Introduction: The electromechanical efficiency of skeletal muscle represents the dissociation between electrical and mechanical events within a muscle. It has been widely studied, with varying methods for its measurement and calculation. For this reason, the purpose of this literature review was to integrate the available research to date and provide more insights about this measure.
Methods: A systematic search of the literature was performed across three online databases: PubMed, ScienceDirect, and SPORTDiscus. This yielded 1284 reports, of which 10 met the inclusion criteria. Included studies have used different methods to measure the electromechanical efficiency (EME) index, including electromyography (EMG), mechanomyography and tensiomyography (TMG).
Results: The EME index was used to assess muscle conditions such as muscle atrophy, pain syndromes, or to monitor rehabilitation in patients with knee problems, fatigue and the effects of exercise and rehabilitation. TMG has been shown to be one of the most reliable methods to obtain the EME index, but its use precludes obtaining the index during voluntary muscle contractions.
Conclusion: Standardizing the EME index is crucial for its diverse applications in clinical, sport, and rehabilitation contexts. Future research should prioritize standardization of measurement protocols for establishing the most repeatable, and reliable approach that can be used for inter-individual comparisons or for assessing an individual for multiple times over a longer period.
Systematic Review Registration: https://www.crd.york.ac.uk/prospero/display_record.php?ID=CRD42023440333 Identifier: CRD42023440333.
1 Introduction
Muscle contraction occurs as a result of electromechanical coupling, which determines the shortening of sarcomeres and hence of entire muscle fibers, while the simultaneous contraction of thousands of muscle fibers causes the muscle to shorten in the longitudinal direction (Valencic et al., 2001; Macgregor et al., 2018). Mechanical muscle function can be quantified using a relatively new non-invasive technique, mechanomyography (MMG), that evolved from sensing muscle vibrations into a sophisticated tool that can capture changes in the geometry of muscle fibers during contractions, specifically lateral oscillations generateded by active muscle, and its own resonance frequency (Ibitoye et al., 2014). These oscilations are independent of the electrical activity of the motor units as measured by electromyography (EMG) (Beck et al., 2005). Specifically, the MMG amplitude is thought to reflect motor unit recruitment, whereas the EMG amplitude reflects both motor unit recruitment and the degree of motor unit integration (Farina and Enoka, 2023). Recent advances in sensor technology and signal processing have improved the capabilities of MMG, giving it insight into muscle mechanics and motor unit activation patterns. Specifically, the MMG amplitude is thought to reflect motor unit recruitment, whereas the EMG amplitude reflects both motor unit recruitment and the degree of motor unit integration (Ebersole and Malek, 2008).
The electromechanical efficiency of skeletal muscle represents the dissociation between electrical and mechanical events of muscle function (i.e., electromechanical coupling), which can be captured by examining the changes in the ratio between MMG and EMG amplitudes, thus creating an electromechanical efficiency (EME) index (Barry et al., 1990). The importance of quantitative insight into the electromechanical function of the vastus medialis muscle during ergometer cycling was highlighted in the study Oka and colleagues (Oka et al., 2014). Authors combined EMG and MMG assessment to objectively quantify muscle performance during dynamic exercise. Several authors have emphasized this approach as simple and desirable in sports and rehabilitation due to different advantages, such as simultaneous assessment of muscle activation and mechanical properties (Beck et al., 2005; Anders et al., 2019; Fukuhara et al., 2021). This provides a comprehensive insight into muscle function and its non-invasive nature, making it practical for use in a variety of settings, from clinical rehabilitation to athletic training (Beck et al., 2005; Anders et al., 2019; Fukuhara et al., 2021).
The EME analysis can provide important information about the status of various muscles including facial and limb muscles (Ioi et al., 2006). It has been used in clinical settings to assess muscle atrophy after disease (Berg et al., 1997), or as a potential indicator of patellofemoral pain syndrome and to assess muscle adaptation after exercise (Ebersole and Malek, 2008). A quite recently, EME index has also been used to assess lower limbs muscle function changes following total knee replacement in end-stage osteoarthritis patients (Paravlic et al., 2020). The EME index can discriminate concentric and eccentric contraction, as well as changes in intrinsic contractile muscle properties after experimentally induced muscle pain (Madeleine and Arendt-Nielsen, 2005). The later findings arguing the importance of EME index, as EMG alone was not being sensitive enough to detect those changes as MMG did during simultaneous measurements (Madeleine and Arendt-Nielsen, 2005). The EME index showed to be useful tool to discriminate between apparently healthy and subjects diagnosed with muscular dystrophy, as reduced EME index has been found in symptomatic compared to healthy subjects (Barry et al., 1990). The age-related muscular dysfunction was investigated using the EME index on patients with chronic low back pain to understand the electrical and mechanical aspects of the pain induced (Sakai et al., 2019). A comparison of EME between vastus medialis and vastus lateralis muscles was performed in a study conducted by (Ebersole and Malek, 2008). EME measurements offer a distinctive perspective on the impact of fatigue on skeletal muscle contractile properties, encompassing changes in intrinsic electric and mechanical components. EME might be valuable in evaluating clinically significant asymmetries in vastus medialis and vastus lateralis muscle function among individuals with knee injuries. The utility of the above has been applied to evaluation of plyometric training effect on the gastrocnemius muscle electromechanical properties (Zubac et al., 2019). Authors reported improvement of EME index in gastrocnemius muscle following 9 weeks of plyometric training in older adults.
The aforementioned studies substantially varied in methods used to evaluate the EME index. The most common methods of MMG signal acquisition refer to acoustomyography using microphones (Jaskólski et al., 2007), vibromyography using piezoelectric accelerometers (Madeleine and Arendt-Nielsen, 2005; Ebersole and Malek, 2008; Sakai et al., 2019); and measurement of perceived skin over muscle movements by detecting changes in the magnetic field (Ioi et al., 2006). A novel method of obtaining the EME index is using tensiomyography (TMG), designed to assess the evoked contraction of individual superficial skeletal muscles (Paravlić et al., 2017; Paravlic et al., 2020). TMG is essentially an MMG method where a TMG sensor is used to detect radial displacement of muscle belly. In study of Paravlic and colleagues, it was used simultaneously with EMG signal (M-wave) to obtain EME index. From the TMG response, the peak-to-peak amplitude of muscle radial displacement (Dm, measured in millimetres [mm]) and the peak-to-peak amplitude of the M-wave (Mptp) are analyzed. It can be said that TMG uses the same principles as mechanomyography, but is designed to work only when the muscle contraction is electrically elicited and uses a unique sensor to detect radial muscle displacement (Valencic et al., 2001). The EME index obtained using tensiomyography was calculated as the ratio of Dm to Mptp (Paravlić et al., 2017).
By overview of the published literature, we found that different methods were used to measure and calculate the EME index. These includes EMG, MMG, and TMG. Moreover, the EME index was used for different purposes and among different populations. For this reason, the purpose of this literature review was to integrate the available research to date and to provide the answers on the following questions: a) What methods have been used so far to measure and calculate the EME index; b) Evaluate the strengths and weaknesses of these methods; c) Suggest guidelines for future research in this field.
2 Methods
The review was carried out in accordance with PRISMA 2020 guidelines (Page et al., 2021). The protocol for present study was prospectively registered on PROSPERO online registry (ID: CRD42023440333).
2.1 Exploratory search strategy
The literature review was carried out by searching PubMed, ScienceDirect, and SPORTDiscus databases from 17 to 27 July 2023. No restriction on the year of publication or language was applied. In all databases, the following keywords or phrases were used: “Electromechanical efficiency,” “Electromechanical efficiency index,” “EME,” “EME index,” “Muscle,” “Skeletal muscle.” The Boolean “OR” and “AND” where used where possible.
2.2 Eligibility criterion for selecting a study
The inclusion criteria were formulated based on the PICO approach, which covers population (P), intervention (I), comparison (C) and outcome (O) (Page et al., 2021): P—men or women of all ages; I—any intervention that used an electromechanical index for neuromuscular status assessment regardless of the method of measurement; C—the case of original research designs with a control group, the control group would be a stand-alone intervention, and in the case of cross-sectional studies, the comparison would look at different individuals (older versus younger, left versus right limb, non-affected versus affected limb, etc.), and; O—electromechanical efficiency of skeletal muscles.
Studies were excluded according to the following criteria: 1) studies that did not investigate the electromechanical efficiency of skeletal muscle and, 2) not meeting inclusion criteria mentioned before.
2.3 Screening strategy
The screening was performed by the first author (GT). After the first screening by GT, the screening was performed by the second author (AP), and based on a compromise between the two authors, the study was included or excluded from the review. As the original studies did not include randomized controlled trials with an experimental design, the methodological quality of the studies was assessed using the Quality Assessment Tool for Observational Cohort and Cross-Sectional Studies developed by National Heart, Lung, and Blood Institute, National Institute of Health (National Heart Lung, and Blood Institute, 2021).
2.4 Data extraction
The information about study design, population (sample type, size and age), perceived variable—EME (mode of integration and which muscles/locations it was performed on) and additional information (tools used in the study and its purpose) were extracted from the original studies included in present review.
2.5 Methodological quality assessment
The Quality Assessment Tool for Observational Cohort and Cross-Sectional Studies (National Heart Lung, and Blood Institute, 2021) was used to assess the methodological strength and risk of bias of the 9 studies included in the review. The methodological assessment of the studies was performed independently by both authors. The NIH Quality Assessment Tool consists of a checklist of 14 questions designed to assess the internal validity (potential risk of selection, information, or measurement bias) of cross-sectional and cohort studies. The criteria were answered “yes,” “no,” or other (not specified; not applicable; not reported). The total score would be the number of affirmative responses. For the qualitative assessment of the final score, scores higher than 12 were considered good, those lower than 9 were considered weak, and those falling in the range 9–12 represented moderate-quality studies. All included studies were rated as good, fair, or poor quality on the basis of a rating sheet with quality assessment instructions.
3 Results
3.1 Basic characteristics of the included studies
The process of searching and selecting articles is presented in Figure 1. In total, ten articles met the inclusion criteria. The basic characteristics of the studies and subjects are presented in Table 1. The total number of subjects in all studies was 267, and their mean age was 38.69 ± 4.02 years. In all studies, the main inclusion criterion was that the EME index reflects the excitation-contraction process in active skeletal muscle. In most studies, the EME index was obtained by EMG and MMG analysis. An exception was the use of the new TMG technology (Zubac et al., 2019; Paravlic et al., 2020). The EME index obtained using TMG technology was shown to be highly reliable in the study by Paravlić et al. (2017). The EME index was used over large superficial muscles in all included studies.
3.2 Methodological quality assessment
Both authors (GT and AP) blinded to each other’s results, screened the full text against the NLHBI criteria and rated methodological quality independently. The median NIH score was 9.9 (SD:1.4), with values ranging from 8 to 12, suggesting that the included studies were generally of fair quality (Table 2).
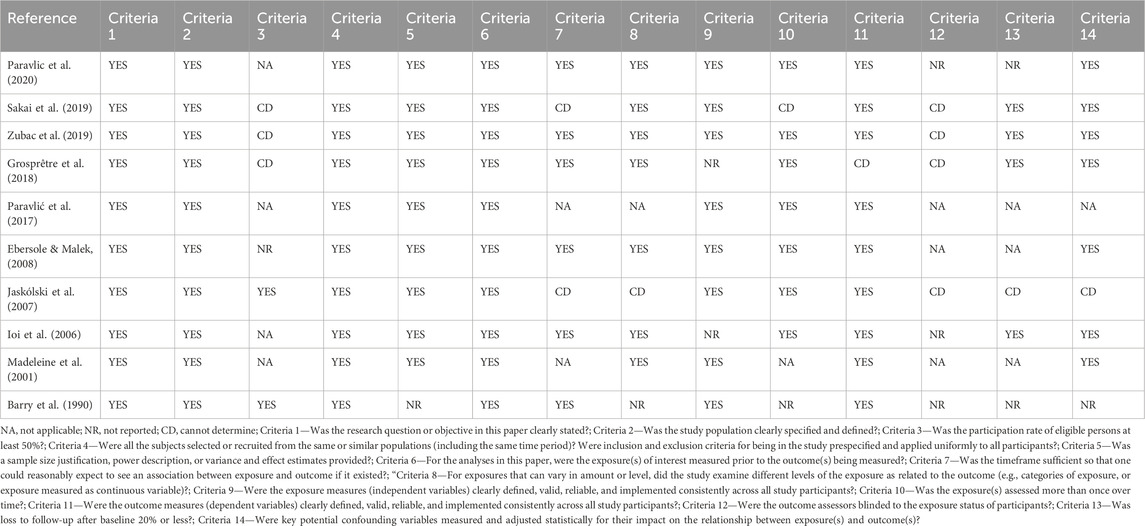
Table 2. The methodological quality of included studies assessed by the quality assessment tool for observational cohort and cross-sectional studies.
3.3 Detailed presentation of the results of the included studies
The experimental research to gain complementary knowledge from EMG and MMG signal recordings was conducted by Madeleine and colleagues in 2001 (Madeleine et al., 2001). Grosprêtre and colleagues compared EME index of triceps surae muscles between power trained athletes engaged in parkour and untrained individuals. Authors found a higher electromechanical efficiency in parkour athletes confirming the greater excitation-contraction coupling efficiency in power trained athletes then non-trained individuals (Grosprêtre et al., 2018). The reliability of the EME obtained by TMG was presented in study of (Paravlić et al., 2017). EME was used to compare muscles before and after muscle fatigue in two studies (Ioi et al., 2006; Ebersole and Malek, 2008), and for comparison between healthy muscles and affected muscles due to chronic knee osteoarthritis (Paravlic et al., 2020) or lower back pain (Sakai et al., 2019). Finally, Zubac and colleagues used EME index to evaluate the effects of plyometric training on medial gastrocnemii muscle in older adults, whereas Jaskólski and colleagues (Jaskólski et al., 2007) used EME index to investigate the effects of acute eccentric training on agonist and antagonist muscles during the elbow flexion movement.
4 Discussion
The aim of this systematic literature review was to investigate various aspects of the EME index, including its utility in assessing muscle conditions, muscle atrophy, pain syndromes, and the effects of exercise and rehabilitation. Additionally, it aimed to provide guidelines for further research and use of the EME index. Ten relevant studies were identified that employed the EME index for diverse purposes and utilized different methods to capture electro-mechanical signals of skeletal muscle. Overall, the EME index appears to offer detailed insights into muscle function and holds potential clinical relevance, as discussed in the following sections.
4.1 Methodology for estimating the EME index
The EME index is a measure of muscle function derived from the assessment of electrical and mechanical muscle actions. The most common calculation technique is the ratio of MMG RMS (root mean square) to EMG RMS [mV/uV] (Madeleine et al., 2001; Jaskólski et al., 2007; Sakai et al., 2019). The Ebersole & Malek, (2008) used the relationship between the normalized MMG amplitude and the normalized EMG amplitude (each repetition separately), by incorporating linear, quadratic and cubic polynomial regression models. A specific calculation of the EME index appears in the study of Ioi and colleagues, where the calculation is made from average rectified value for MMG and EMG values in order to determine how much muscle action potential is converted into muscle contraction. In other studies used a new MMG technique called TMG, where the EME index was calculated using the parameter extracted from the TMG (Dm) and the M-waves (Mptp) to form the EME index (Dm/Mptp) (Paravlić et al., 2017; Zubac et al., 2019; Paravlic et al., 2020). A similar extraction strategy was used in the study of Grosprêtre et al. (2018) where the EME index was obtained from the ratio of the average muscle twitches measured by MMG obtained at the maximum M-wave (Mmax). Given that both methods used in later studies calculate the EME index from electrically elicited muscle contraction in isometric conditions, it can be said that these two methods are the most similar, differing primarily in the assessment of the maximum mechanical response of the muscle. Common to all studies is the introduction of the EME index, representing the ratio of the mechanical response normalized to the electrical muscle activity. However, the question arises as to which method of EME index derivation is the most reliable, a factor dependent on how the signals are acquired and processed. The measurement of the mechanical response of muscles varies between studies, utilizing accelerometers, piezoelectric contact sensors, condenser microphones, laser distance sensors, etc., but the reliability of these methods poses a challenge (Ibitoye et al., 2014). For instance, a considerable amount of the variation in the reliability among the above-mentioned methods might be explained by the initial setting of the measurements, which differs between methods studied. This includes factors such as the type of muscle contraction studied (isometric vs dynamic; voluntary or electrically elicited). For example, Al-Zahrani and colleagues (2009) employed the isometric contractions, where the reliability of the MMG signal in the assessment of quadriceps fatigue was investigated and found to be high (ICC = 0.79–0.83) and low (ICC = 0.43–0.66) for intra-day and inter-day assessments, respectively. Paravlić and colleagues (2017) summarised the findings of other studies where different muscles are studied but under the same conditions (isometric contraction) and reported a very high coefficient of variance among studies. Authors also found the highest reliability measured by acoustomyography (excluding the TMG technique) that is achieved at the lowest levels of muscle activity. During the analysis of dynamic muscle function, which is of greater importance in clinical applications numerous factors affecting MMG have been identified (Stokes, 1993; Herda et al., 2008). These include changes in the length of the muscle and consequently torque, the ambient temperature, and the thickness of the tissue overlying the muscle being studied (Herda et al., 2008; Ibitoye et al., 2014). MMG obtained by means of a capturing radial muscle displacement triggered by an electrical stimulation pulse has been shown to be much more reliable compared to voluntary contractions (Ibitoye et al., 2014). These parameters seem to be reliable physiological parameters of the measured muscles that highly correlate with torque measurements and have also been used to assess muscle fatigue, stiffness and endurance, respectively (Uwamahoro et al., 2021).
A TMG represents a specific MMG technique that captures radial displacement of muscle belly after electrically elicited muscle contractions. The results of a quantitative summary of individual reliability studies confirm high to excellent relative reliability for all the basic TMG parameters including (muscle displacement (ICC, Dm = 0.98), time of contraction (Tc = 0.95) and time of delay (Td = 0.91)) (Lohr et al., 2019). Thus, Dm was used to calculate the EME index when utilizing the TMG method. Paravlic and colleagues showed very high reliability with an average ICC of 0.88 for Dm, 0.90 for Mptp and 0.92 for the EME index (Paravlić et al., 2017). This was also the first study to demonstrate the high reliability of the EME index obtained using the TMG and M-wave techniques. It can be said that the most appropriate and reliable method of obtaining the EME index is by TMG technique. It must be stressed that TMG operates during isometric muscle conditions and electrically induced muscle contraction with submaximal electrical stimuli. Further studies aimed at investigating the discriminative validity of EME index are warranted.
4.2 The EME index’s applied relevance
The EME index has been shown to have clinical relevance (Ebersole and Malek, 2008; Paravlic et al., 2020). The authors reported that EME provides insight into the impact of muscle fatigue on skeletal muscle function and is a useful tool to assess and quantify clinically relevant asymmetries in vastus medialis and vastus lateralis muscle function in patients with knee problems (Ebersole and Malek, 2008). Based on these findings, the EME index can be used as a screening tool to monitor rehabilitation protocols, in particular patellofemoral syndrome, or to improve exercise control and muscle function. Furthermore, Paravlic and colleagues assessed the EME index of gastrocnemii medialis muscle following the total knee arthroplasty that decreased for 38% (Paravlic et al., 2020). Orizio and colleagues (Orizio et al., 1997) investigated difference in muscle function between healthy and affected muscles in patients with cerebral palsy and muscular dystrophy. Observed differences in reduced electromechanical coupling in affected muscles were prescribed to reduction in type II muscle fiber content and overall number of efficient motor units in affected subjects (Orizio et al., 1997). Similarly, Barry and colleagues, suggested that the reduction in the EME index in adolescents with various neuromuscular disorders may be due to atrophy of muscle fibers which, although generating electrical activity, have very little mechanical activity (Barry et al., 1990). By providing objective measurements of muscle function and quality, clinicians can assess asymmetries, monitor progress, and tailor interventions to individual patient needs to improve outcomes across different clinical settings.
Given that the EME index has been shown to be clinically relevant, it would be reasonable to translate its usefulness into sports diagnostic practice. It has been shown to be a valid tool for the diagnosis of muscle fatigue, and this was confirmed in a study Ioi et al. (2006) where the mean EME values after fatigue were lower than those of the pre-fatigue trials at all levels expressed as a percentage of maximal voluntary contraction, namely, for the masseter muscle. In the study Kapadia, (2022) the authors report similar findings, namely, that the EME index has the potential to help characterise the influence of fatigue on force production. These factors cannot be accurately determined from EMG and MMG data alone, as a decrease in the index may be explained by impaired coupling of actin and myosin during muscle contraction, as a result of the accumulation of metabolic by-products, namely, hydrogen ions and diprotonated phosphate (Layzer, 1990). The sport-diagnostic relevance of the EME index has been demonstrated in a study by Jaskólski et al. (2007) who observed a reduction in EME values following 25 eccentric contractions at 50% MVC compared to pre-protocol. The authors reported that EMG and MMG recordings were differently altered immediately after and 120 h after the eccentric protocol, suggesting that several factors, including a) reduced rate of calcium release from the sarcoplasmic reticulum (acute effect); b) altered motor control (chronic effect); and c) increased muscle stiffness (chronic effect), mediated these results. These findings suggest that simultaneous measurements of electromechanical coupling may provide more insights into the mechanisms driving changes in neuromuscular function following the fatiguing protocols, than either parameter alone.
Despite all the sports-diagnostic advantages brought about by the introduction of the EME index, we cannot avoid the fact that the latter can be the simplest tool for evaluating the training cycle, which is the foundation of any successful training process. A 10-week plyometric training program has been shown to increase the EME index by 22.9% compared to a control group that continue with their habitual physical activity (Zubac et al., 2019). Therefore, we can conclude that the EME index can serve as useful tool for evaluating the electromechanical changes after physical training, as well as an indicator of improved jumping performance. Moreover, in the study conducted by Grosprêtre et al. (2018), EME index showed that parkour athletes had a significantly higher EME index compared to untrained athletes. Thus, suggesting that EME index may be used to distinguish more and less trained athletes.
4.3 Limitations of this review article
The findings of all the inclusion studies demonstrate the benefits offered by the introduction of an EME index, but some limitations should also be highlighted. One of these is certainly the number included studies. However, it is important to note that the introduction of an EME index is still a relatively new method with a limited body of research. For this reason, we believe that more studies are needed to investigate other emerging questions where EME index can serve its purpose in differentiating between several muscle groups, various exercise modalities and populations. The difficulties arising from crosstalk from adjacent muscles, which can affect the reliability of EMG and MMG signal capture, have been highlighted and this alone represents a major limitation for incorporating EME index into clinical practice. This could lead to misinterpretation of the results and limit the usefulness of the EME index. Further research should focus more on developing and testing methods to reduce the error of measurement in order to provide more reliable assessment. A limitation can also be seen in the limited generalisability of the results obtained. Many of the included studies focused on specific populations, such as athletes or people with specific health conditions. Despite all the results pointing in favour of the EME index, it would be reasonable to generalise the meaning in the sense that the EME index could be used as a measurement tool to determine muscle status in the general population. Also, one of the limitations is that included studies is extremely difficult to compare, as they use different approaches to obtain the EME index. For the latter reason, it would be useful to consider standardising the methods used to measure the EME index, as this would allow better comparability of results and facilitate the future research in this field.
5 Conclusion
The EME index is a promising parameter that provides a comprehensive and in-depth view of muscle function by describing the excitation-contraction process of skeletal muscle. The EME index is calculated by comparing the mechanical muscle response with its electrical potentials. The latter can be used to assess muscle conditions such as muscle atrophy, pain syndromes, or to monitor rehabilitation in patients with knee problems, fatigue and the effects of exercise and rehabilitation. Therefore, it can be said that the EME index has both clinical and sport-diagnostic relevance.
Various studies have used different methods to measure the EME index, including EMG, MMG, and TMG. TMG has been shown to be one of the most reliable methods to obtain the EME index, but its use precludes obtaining the index during voluntary muscle contractions.
Given the diversity of applicability and the different methods of capturing the EME index, which are presented in the paper, we expect advances in technology to obtain the EME index and thus improvements in psychometric properties. With standardized protocols, EME index can facilitate widespread use in clinical settings. In addition, ongoing research may uncover a new holistic insight into muscle function that helps with targeted clinical conditions.
Improved understanding and use of the EME Index is likely to lead to specific changes in clinical, sports and rehabilitation settings. Clinically, it could facilitate earlier detection and more targeted interventions for muscle dysfunction whereas in sports, it could be used for optimization of training regimes and injury prevention strategies. From arehabilitation point of view, it could help with personalised treatment plans and improved patient outcomes.
Data availability statement
The original contributions presented in the study are included in the article/Supplementary Material, further inquiries can be directed to the corresponding author.
Author contributions
GT: Conceptualization, Data curation, Formal Analysis, Investigation, Project administration, Writing–original draft, Writing–review and editing. AP: Conceptualization, Data curation, Investigation, Methodology, Project administration, Supervision, Validation, Writing–original draft, Writing–review and editing.
Funding
The author(s) declare that financial support was received for the research, authorship, and/or publication of this article. This research is a part of a projects funded by University of Ljubljana, Development Fund titled “The role of physical Activity in Cardiovascular disease risk reducTiOn for Non-communicable diseases. Do non-responders to exercise really exist?” (project ID: 005-1/2023, principal investigator: AP) and “The role of physical Activity in Cardiovascular disease risk reducTiON – On the way to personalized exercise prescription (ACT-ON)” (project ID: 802-15/2023-5, principal investigator: AP), from which the open access publishing of this article was supported. This research also took place within the kinesiology of mono-structured, poly-structured and conventional sports research and infrastructure programme funded by Slovenian Research Agency (ARIS) (project No. P5-0147).
Conflict of interest
The authors declare that the research was conducted in the absence of any commercial or financial relationships that could be construed as a potential conflict of interest.
Publisher’s note
All claims expressed in this article are solely those of the authors and do not necessarily represent those of their affiliated organizations, or those of the publisher, the editors and the reviewers. Any product that may be evaluated in this article, or claim that may be made by its manufacturer, is not guaranteed or endorsed by the publisher.
References
Anders, J. P. V., Smith, C. M., Keller, J. L., Hill, E. C., Housh, T. J., Schmidt, R. J., et al. (2019). Inter- and intra-individual differences in EMG and MMG during maximal, bilateral, dynamic leg extensions. Sports 7 (7), 175. doi:10.3390/sports7070175
Barry, D., Gordon, K., and Hinton, G. (1990). Acoustic and surface EMG diagnosis of pediatric muscle disease. Muscle Nerve 13, 286–290. doi:10.1002/mus.880130403
Beck, T. W., Housh, T. J., Cramer, J. T., Weir, J. P., Johnson, G. O., Coburn, J. W., et al. (2005). Mechanomyographic amplitude and frequency responses during dynamic muscle actions: a comprehensive review. Biomed. Eng. OnLine 4, 67. doi:10.1186/1475-925X-4-67
Berg, H. E., Larsson, L., and Tesch, P. A. (1997). Lower limb skeletal muscle function after 6 wk of bed rest. J. Appl. Physiology 82 (1), 182–188. doi:10.1152/jappl.1997.82.1.182
Ebersole, K. T., and Malek, D. M. (2008). Fatigue and the electromechanical efficiency of the vastus medialis and vastus lateralis muscles. J. Athl. Train. 43 (2), 152–156. doi:10.4085/1062-6050-43.2.152
Farina, D., and Enoka, R. M. (2023). Evolution of surface electromyography: from muscle electrophysiology towards neural recording and interfacing. J. Electromyogr. Kinesiol. 71, 102796. doi:10.1016/J.JELEKIN.2023.102796
Fukuhara, S., Kawashima, T., and Oka, H. (2021). Indices reflecting muscle contraction performance during exercise based on a combined electromyography and mechanomyography approach. Sci. Rep. 11 (1), 21208. doi:10.1038/S41598-021-00671-2
Grosprêtre, S., Gimenez, P., and Martin, A. (2018). Neuromuscular and electromechanical properties of ultra-power athletes: the traceurs. Eur. J. Appl. Physiology 118 (7), 1361–1371. doi:10.1007/s00421-018-3868-1
Herda, T. J., Ryan, E. D., Beck, T. W., Costa, P. B., DeFreitas, J. M., Stout, J. R., et al. (2008). Reliability of mechanomyographic amplitude and mean power frequency during isometric step and ramp muscle actions. J. Neurosci. Methods 171 (1), 104–109. doi:10.1016/j.jneumeth.2008.02.017
Ibitoye, M. O., Hamzaid, N. A., Zuniga, J. M., and Wahab, A. K. A. (2014). Mechanomyography and muscle function assessment: a review of current state and prospects. Clin. Biomech. (Bristol, Avon) 29 (6), 691–704. doi:10.1016/J.CLINBIOMECH.2014.04.003
Ioi, H., Kawakatsu, M., Nakata, S., Nakasima, A., and Counts, A. L. (2006). Mechanomyogram and electromyogram analyses for investigating human masseter muscle fatigue. Orthod. Waves 65 (1), 15–20. doi:10.1016/j.odw.2005.12.002
Jaskólski, A., Andrzejewska, R., Marusiak, J., Kisiel-Sajewicz, K., and Jaskólska, A. (2007). Similar response of agonist and antagonist muscles after eccentric exercise revealed by electromyography and mechanomyography. J. Electromyogr. Kinesiol. 17 (5), 568–577. doi:10.1016/j.jelekin.2006.05.002
Layzer, R. B. (1990). 3 Muscle metabolism during fatigue and work. Baillière’s Clin. Endocrinol. Metabolism 4 (3), 441–459. doi:10.1016/S0950-351X(05)80064-3
Lohr, C., Schmidt, T., Medina-Porqueres, I., Braumann, K. M., Reer, R., and Porthun, J. (2019). Diagnostic accuracy, validity, and reliability of Tensiomyography to assess muscle function and exercise-induced fatigue in healthy participants. A systematic review with meta-analysis. J. Electromyogr. Kinesiol. 47, 65–87. doi:10.1016/J.JELEKIN.2019.05.005
Macgregor, L. J., Hunter, A. M., Orizio, C., Fairweather, M. M., and Ditroilo, M. (2018). Assessment of skeletal muscle contractile properties by radial displacement: the case for tensiomyography. Sports Med. 48 (7), 1607–1620. doi:10.1007/s40279-018-0912-6
Madeleine, P., and Arendt-Nielsen, L. (2005). Experimental muscle pain increases mechanomyographic signal activity during sub-maximal isometric contractions. J. Electromyogr. Kinesiol. 15 (1), 27–36. doi:10.1016/j.jelekin.2004.06.006
Madeleine, P., Bajaj, P., Søgaard, K., and Arendt-Nielsen, L. (2001). Mechanomyography and electromyography force relationships during concentric, isometric and eccentric contractions. J. Electromyogr. Kinesiol. 11 (2), 113–121. doi:10.1016/S1050-6411(00)00044-4
National Heart Lung, and Blood Institute (2021) NHLBI: Quality assessment tool for observational cohort and crosssectional studies. Available at: https://www.nhlbi.nih.gov/health-topics/study-quality-assessment-tools (Accessed July 28, 2023)
Oka, H., Konishi, Y., and Kitawaki, T. (2014). Simultaneous measurement of displacement-MMG/EMG during exercise. SICE J. Control, Meas. Syst. Integration 7 (6), 332–336. doi:10.9746/JCMSI.7.332
Orizio, C., Esposito, F., Sansone, V., Parrinello, G., Meola, G., and Veicsteinas, A. (1997). Muscle surface mechanical and electrical activities in myotonic dystrophy. Electromyogr. Clin. Neurophysiology 37 (4), 231–239.
Page, M. J., McKenzie, J. E., Bossuyt, P. M., Boutron, I., Hoffmann, T. C., Mulrow, C. D., et al. (2021). The PRISMA 2020 statement: an updated guideline for reporting systematic reviews. Bmj-British Med. J. 372, n71. doi:10.1136/bmj.n71
Paravlic, A. H., Pisot, R., and Simunic, B. (2020). Muscle-specific changes of lower extremities in the early period after total knee arthroplasty: insight from tensiomyography. J. Musculoskelet. Neuronal Interact. 20 (3), 390–397.
Paravlić, D., Zubac, D., and Šimunič, B. (2017). Reliability of the twitch evoked skeletal muscle electromechanical efficiency: a ratio between tensiomyogram and M-wave amplitudes. J. Electromyogr. Kinesiol. 37, 108–116. doi:10.1016/j.jelekin.2017.10.002
Sakai, Y., Matsui, H., Ito, S., Hida, T., Ito, K., Koshimizu, H., et al. (2019). Electrophysiological function of the lumbar multifidus and erector spinae muscles in elderly patients with chronic low back pain. Clin. Spine Surg. 32 (1), E13–E19. doi:10.1097/BSD.0000000000000709
Stokes, M. J. (1993). Acoustic myography: applications and considerations in measuring muscle performance. Isokinet. Exerc. Sci. 3 (1), 4–15. doi:10.3233/IES-1993-3101
Uwamahoro, R., Sundaraj, K., and Subramaniam, I. D. (2021). Assessment of muscle activity using electrical stimulation and mechanomyography: a systematic review. Biomed. Eng. OnLine 20 (1), 1. doi:10.1186/S12938-020-00840-W
Valencic, V., Knez, N., and Simunic, B. (2001). Tenziomiography: detection of skeletal muscle response by means of radial muscle belly displacement. Fac. Electr. Eng. 1, 1–10.
Keywords: EME index, muscle function, tensiomyography, electromyography, rehabilitation, athletic performance
Citation: Turnsek G and Paravlic AH (2024) Electromechanical efficiency index of skeletal muscle and its applicability: a systematic review. Front. Bioeng. Biotechnol. 12:1398047. doi: 10.3389/fbioe.2024.1398047
Received: 08 March 2024; Accepted: 17 April 2024;
Published: 09 May 2024.
Edited by:
Elvira Padua, Università telematica San Raffaele, ItalyCopyright © 2024 Turnsek and Paravlic. This is an open-access article distributed under the terms of the Creative Commons Attribution License (CC BY). The use, distribution or reproduction in other forums is permitted, provided the original author(s) and the copyright owner(s) are credited and that the original publication in this journal is cited, in accordance with accepted academic practice. No use, distribution or reproduction is permitted which does not comply with these terms.
*Correspondence: Armin Huso Paravlic, armin.paravlic@hotmail.com