- 1The First Department of Hand and Foot Surgery, Central Hospital of Dalian University of Technology, Dalian, China
- 2Department of Engineering Mechanics, School of Mechanics and Aerospace Engineering, Dalian University of Technology, Dalian, China
- 3DUT-BSU Joint Institute, Dalian University of Technology, Dalian, China
- 4Department of Mechanical Engineering, New York Institute of Technology, New York, NY, United States
The escalating prevalence of diabetes has accentuated the significance of addressing the associated diabetic foot problem as a major public health concern. Effectively offloading plantar pressure stands out as a crucial factor in preventing diabetic foot complications. This review comprehensively examines the design, manufacturing, and evaluation strategies employed in the development of diabetic foot insoles. Furthermore, it offers innovative insights and guidance for enhancing their performance and facilitating clinical applications. Insoles designed with total contact customization, utilizing softer and highly absorbent materials, as well as incorporating elliptical porous structures or triply periodic minimal surface structures, prove to be more adept at preventing diabetic foot complications. Fused Deposition Modeling is commonly employed for manufacturing; however, due to limitations in printing complex structures, Selective Laser Sintering is recommended for intricate insole designs. Preceding clinical implementation, in silico and in vitro testing methodologies play a crucial role in thoroughly evaluating the pressure-offloading efficacy of these insoles. Future research directions include advancing inverse design through machine learning, exploring topology optimization for lightweight solutions, integrating flexible sensor configurations, and innovating new skin-like materials tailored for diabetic foot insoles. These endeavors aim to further propel the development and effectiveness of diabetic foot management strategies. Future research avenues should explore inverse design methodologies based on machine learning, topology optimization for lightweight structures, the integration of flexible sensors, and the development of novel skin-like materials specifically tailored for diabetic foot insoles. Advancements in these areas hold promise for further enhancing the effectiveness and applicability of diabetic foot prevention measures.
1 Introduction
Diabetic foot represents a severe condition characterized by infection, ulceration, or tissue destruction in the foot of individuals with diabetes, often concomitant with lower extremity neuropathy, peripheral artery disease (particularly lower extremity arterial disease) (Jodheea-Jutton et al., 2022), or Charcot foot. According to the comprehensive analysis conducted by Zhang et al. (2017), the aggregated global prevalence of diabetic foot ulceration stands at 6.3% (95% confidence intervals: 5.4%–7.3%). This prevalence is on a continual rise, mirroring the escalating numbers of individuals afflicted by diabetes and those at risk, thereby establishing the diabetic foot as a burgeoning global public health challenge (van Netten et al., 2020; Edmonds et al., 2021).
The surge in diabetic foot cases has intensified the demand for effective prevention and treatment strategies. Diabetic foot ulcers (DFU), infections, or soft tissue damage often evade early detection, particularly in neuropathic feet, as the typical indicators of local infection, such as redness, pain, or tenderness, may be absent (Edmonds et al., 2021). Complicating matters, diabetic patients with deep foot infections may not exhibit traditional signs like an elevated white blood cell count and temperature, leading to delayed diagnosis and treatment (Apelqvist, 2012; Yu et al., 2022). Common imaging tests prove effective primarily in diagnosing diabetic foot conditions in the presence of bone alterations, abscess formation, and soft tissue destruction, missing the optimal window for intervention (Senneville et al., 2020; Yufeng and Yanwu, 2021).
Treatment and care pose challenges, including intricate cures, high recurrence rates, and elevated treatment costs (Kerr et al., 2019; Edmonds et al., 2021). The 5-year mortality and direct care costs for patients with diabetic foot complications rival those of cancer (Armstrong et al., 2020). Lack of awareness about prevention further compounds the issue, making treatment more challenging once diagnosed. In the initial stages of diabetic foot, lower limb pain during walking may be alleviated or disappear after resting, leading high-risk groups to neglect prevention services, increasing the likelihood of severe ulcers or amputations (Chatwin et al., 2020). Therefore, early prevention and intervention during the initial stages of disease development are crucial for diabetic foot patients (Lim et al., 2017). The utilization of specialized therapeutic insoles emerges as a commendable preventive measure (Schaper et al., 2020). These insoles are widely employed to mitigate plantar pressure peaks or optimize pressure distribution, effectively reducing the likelihood of complications and amputations (Korada et al., 2020). Nevertheless, challenges persist in further optimizing pressure offloading performance, enhancing comfort, and streamlining the design and manufacturing cycle and cost of customized insoles (Collings et al., 2021).
In the realm of diabetic foot insoles, notable advancements in design approaches and manufacturing techniques have brought about significant transformations, leading to a marked improvement in their performance, as illustrated in Figure 1. The triad of design, manufacturing, and performance evaluation stands as a critical nexus within the domain of diabetic foot insoles, with each facet exerting a profound influence on the others. Advanced manufacturing technologies now facilitate the creation of diabetic foot insoles boasting intricate structures, including honeycomb porous configurations and triply periodic minimal surface (TPMS) structures. These elaborate structures encounter challenges in precision and production costs when subjected to conventional manufacturing methods such as hand forming or numerical control techniques. Concurrently, the evaluation of diabetic foot insole performance serves as a driving force behind advancements in manufacturing and design. Thus, the current study offers a comprehensive review of the design, manufacturing, and performance evaluation of diabetic foot insoles, aiming to propel further breakthroughs in this dynamic field.
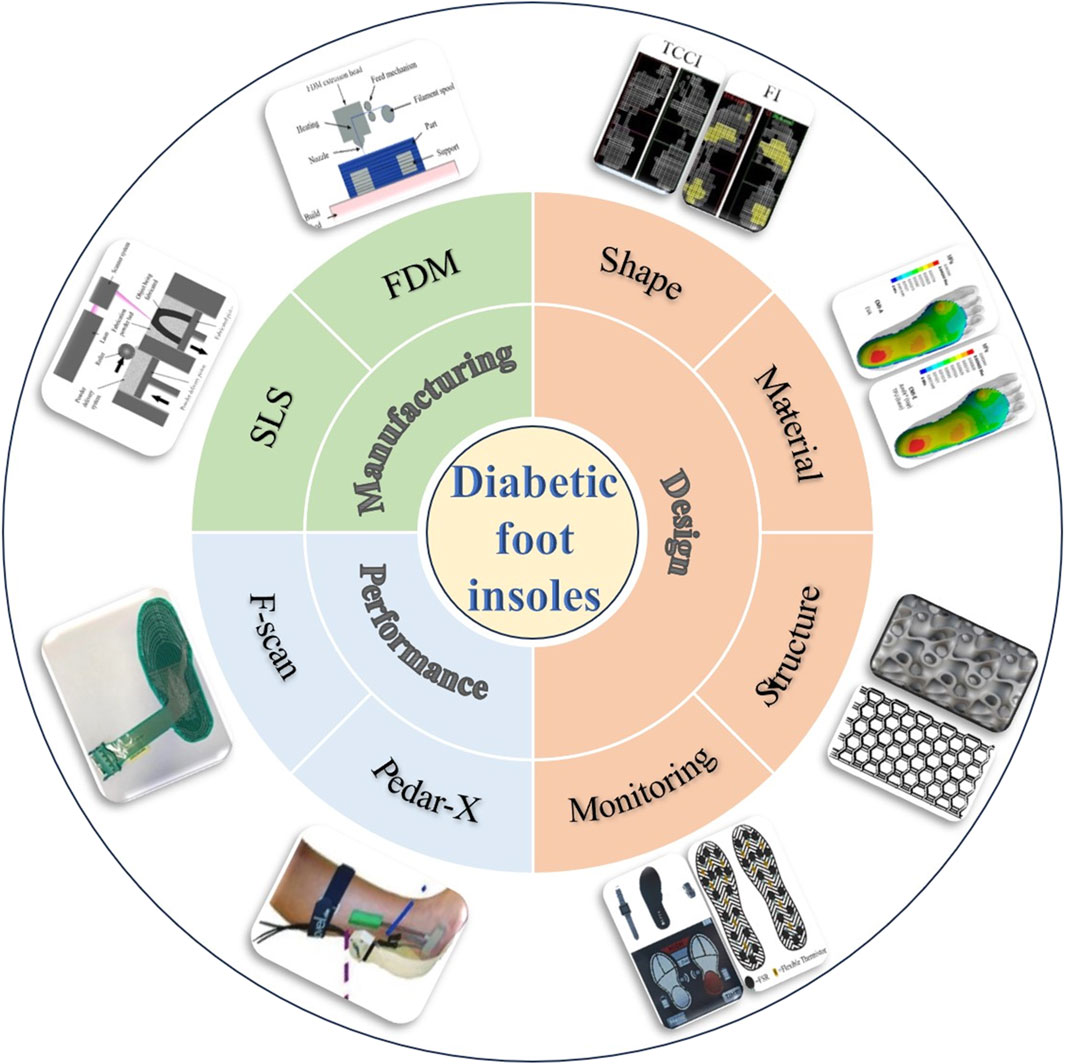
Figure 1. Schematic diagram of design, manufacture, and evaluation of diabetic foot insoles (D’Amico et al., 2021; Nouman et al., 2021; Mondal et al., 2023; Claybrook et al., 2022; Khandakar et al., 2022; Abbott et al., 2019; Chatwin et al., 2020; Aliberti et al., 2011; Alafaghani et al., 2017; Abdulhameed et al., 2019).
2 Review methods
2.1 Literature search strategy and selection criteria
We conducted searches in English-language databases, including PubMed, Web of Science, Elsevier ScienceDirect, SpringerLink, and Wiley Online Library. The search terms used were “diabetic foot” OR “"diabetic feet” OR “diabetic foot ulcer” OR “diabetic foot problem” AND “insoles” OR “smart insoles” OR “orthotic”; “diabetic foot insoles” OR “diabetic foot ulcer” AND “design” OR “biomechanics” OR “finite element” OR “pressure offloading”; “diabetic foot insoles” OR “diabetic foot ulcer” AND “manufacturing” OR “additive manufacturing”; “diabetic foot insoles” AND “evaluation”. We did not restrict the study design or the geographic level (national or regional) of the studies. All databases were searched from January 2000 to January 2023. We also reviewed the references of all included articles to identify other potentially relevant surveys.
The study inclusion criteria were as follows: firstly, the data needed to be presented in English; secondly, detailed methodology for the design, manufacturing, and evaluation of diabetic foot insoles needed to be described; thirdly, the study had to provide sufficient information to enable the design of diabetic foot smart insoles. If multiple articles were based on the same methodology, only the study with the most complete data was included. Furthermore, abstracts meeting the inclusion criteria were also included. The study exclusion criteria were as follows: firstly, duplicate methodologies needed to be excluded; secondly, studies without objective or quantitative data needed to be excluded; finally, studies lacking design and analysis details, or accurate conclusions needed to be excluded. The PRISMA flow diagram of identifying studies via databases is shown in Figure 2.
In case of disagreement during the study selection process, we followed pre-established inclusion and exclusion criteria to minimize subjectivity and improve consistency. If consensus could not be reached, we consulted relevant experts. Inclusion and exclusion criteria were reconsidered if necessary to ensure appropriateness and consensus for final selection.
2.2 Results of searching
The number of studies included about 18 studies on the topic of “Introduction”; about 62 studies on the topic of “Design of Diabetic Foot Insoles”; about 26 studies on the topic of “Additive Manufacturing of Diabetic Foot Insoles”; and about 21 studies on the topic of “Performance Evaluation of Diabetic Foot Insoles”. There were 12 studies that contained multiple topics, so they were included twice. Among the general participants in these included studies, researchers in Europe and North America were more active than those in other regions. Some major research centers and laboratories are located in countries such as the US, UK, Germany, and the Netherlands. Additionally, many researchers included in the studies are affiliated with international organizations and associations, such as the International Diabetic Foot Association and the European Association for the Study of Diabetes Foot.
3 Design of diabetic foot insoles
The development of diabetic foot is not solely linked to neuropathy and changes in blood glucose but is also influenced by biomechanics (Asghar and Naaz, 2022). The plantar pressure distribution in healthy individuals versus diabetic foot patients differs significantly, as depicted in Figure 3A (Deschamps et al., 2013; Fernando et al., 2016; Bus et al., 2021). Moreover, variations exist in pressure conditions across different plantar areas, as illustrated in Figure 3B (Perren et al., 2021). Ground pressure, excessive plantar shear stress (Jones et al., 2022), and compressive stress from shoes or adjacent toes collectively impact diabetic foot development (Allan et al., 2016). Designing specialized insoles for diabetic foot patients becomes imperative due to these complexities. Diabetic foot insoles can be broadly categorized into two groups: pressure offloading insoles and smart monitoring insoles.
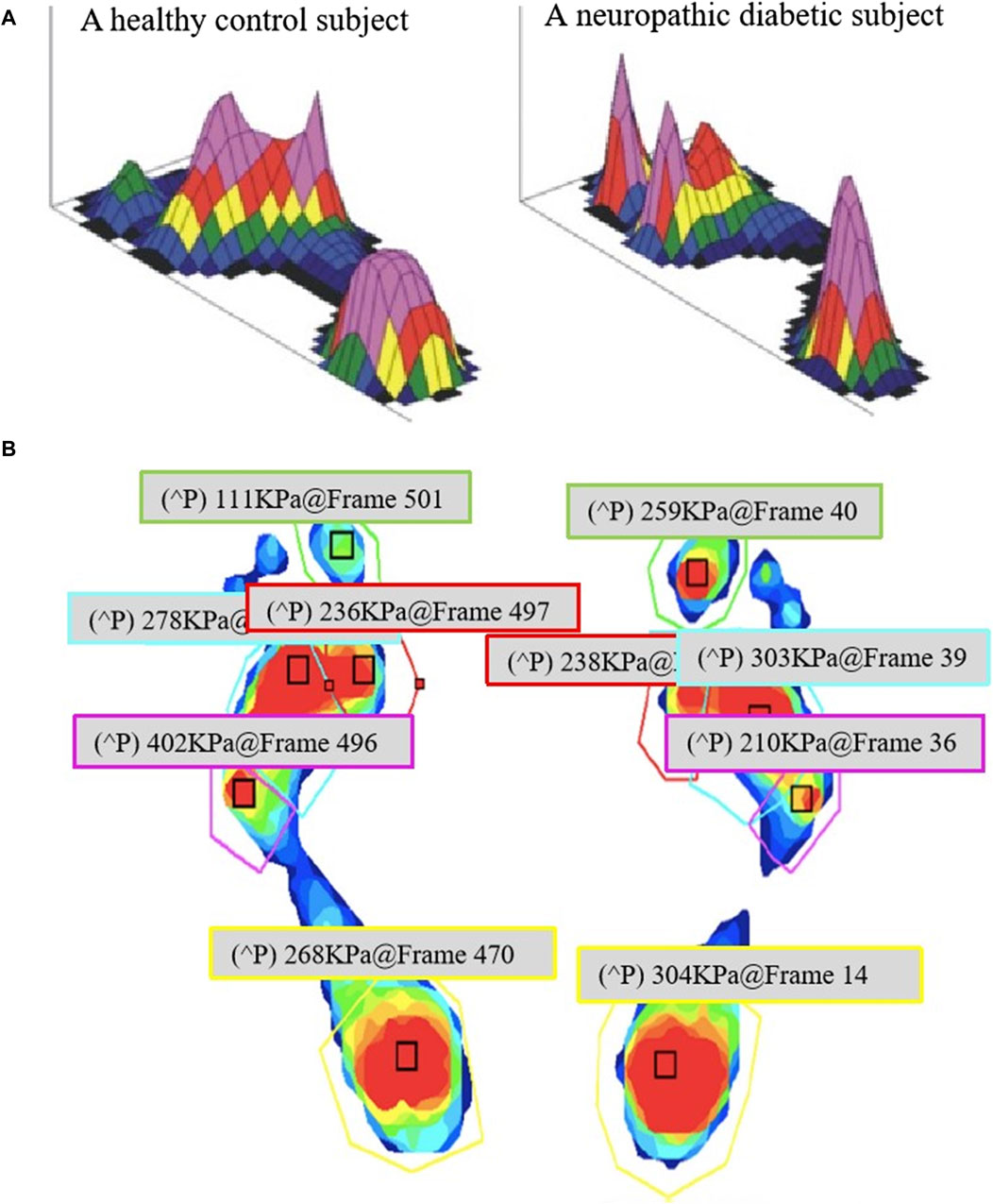
Figure 3. Comparison of the plantar pressure: (A) Comparison of peak plantar pressure in a healthy subject and a neuropathic diabetic subject (Bus et al., 2021), and (B) Different regions with different plantar pressures (Perren et al., 2021).
3.1 Design of pressure offloading diabetic foot insoles
Local lesions exacerbate foot ulcers when certain sole parts are over-pressed (Singh et al., 2021). Therefore, offloading plantar pressure or optimizing pressure distribution effectively prevents and treats diabetic foot (Bellomo et al., 2022). Enhancing the pressure offloading performance involves optimizing the insole’s shape, material, and structure (Haris et al., 2021). Additionally, the comfort levels of insoles with different shapes, materials, and structures vary. Considering that diabetic patients may need to wear insoles for extended periods, discomfort significantly affects their regular usage, potentially compromising prevention or treatment effectiveness.
The finite element (FE) method has emerged as a key tool in designing diabetic foot insoles (Moayedi et al., 2021). These simulations aim to enhance our understanding of foot mechanics in health and disease, guiding the design of personalized insoles (Erdemir et al., 2012). The FE model for insole design is depicted in Figure 4. Through finite element analysis (FEA) (Zeng et al., 2021), we can predict the pressure offloading performance of insoles with different shapes, materials, and structures, optimizing their design for improved diabetic foot insole performance (Ghassemi et al., 2015). However, these simulations have limitations, and future research needs to consider additional biomechanical variables. Complex processes involving the interaction and response of various foot tissues, such as bones, muscles, and fascia, necessitate the consideration of biomechanical variables like joint angles, moments, muscle activity, skeletal stress, and strain for a comprehensive evaluation of foot motion, stability, muscle coordination, functionality, and the prediction of foot disease risks.
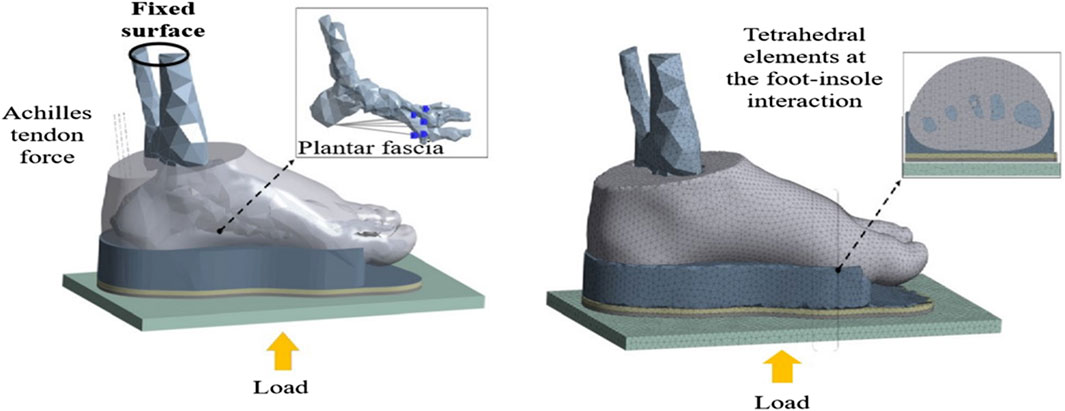
Figure 4. The FE foot model with customized insole and ground (Nouman et al., 2023).
3.1.1 Shape of the diabetic foot insole
In designing the insole, determining the insole’s shape is crucial. Due to individual foot differences, custom-molded shapes are critical for reducing peak plantar pressure in diabetic foot insoles (Martinez-Santos et al., 2019). Jafarzadeh et al. (2021) optimized insole shape using Python and MATLAB programming, starting from a 25.0 mm flat insole. ABAQUS was then used for contact pressure analysis, revealing a 25% reduction in maximum plantar pressure compared to the flat insole. Studies indicate that total contact insoles offer more benefits to diabetic foot than flat insoles (Ahmed et al., 2020; Shim et al., 2021). El-Hilaly et al. (2013) found the efficacy of total contact insoles in offloading foot pressures in diabetic patients following partial first-ray amputations, leading to significant pressure reduction compared to flat insoles during standing and walking. D’Amico et al. (2021) compared Computer Aided Design-Computer Aided Manufacturing (CAD-CAM) and traditional total contact customized insoles (TCCI), showing both reduced risk areas and optimized plantar pressure distribution compared to flat insoles (Figure 5). Arch-supported insoles were found beneficial for diabetic feet with a high arch, while total contact insoles were less favorable for flat feet (Niu et al., 2020). Table 1 summarizes studies on the shape of diabetic foot insoles. Multidisciplinary design methods incorporating computational and mathematical approaches have been explored for insole design. Shalamberidze et al. (2021) applied integral curves of solutions to Dirichlet singular boundary differential equations (Rachůnková et al., 2013) to accurately describe the geometric shapes of overpressure regions. A computer program based on this mathematical algorithm designed individualized orthopedic insoles, offering new avenues for future research.
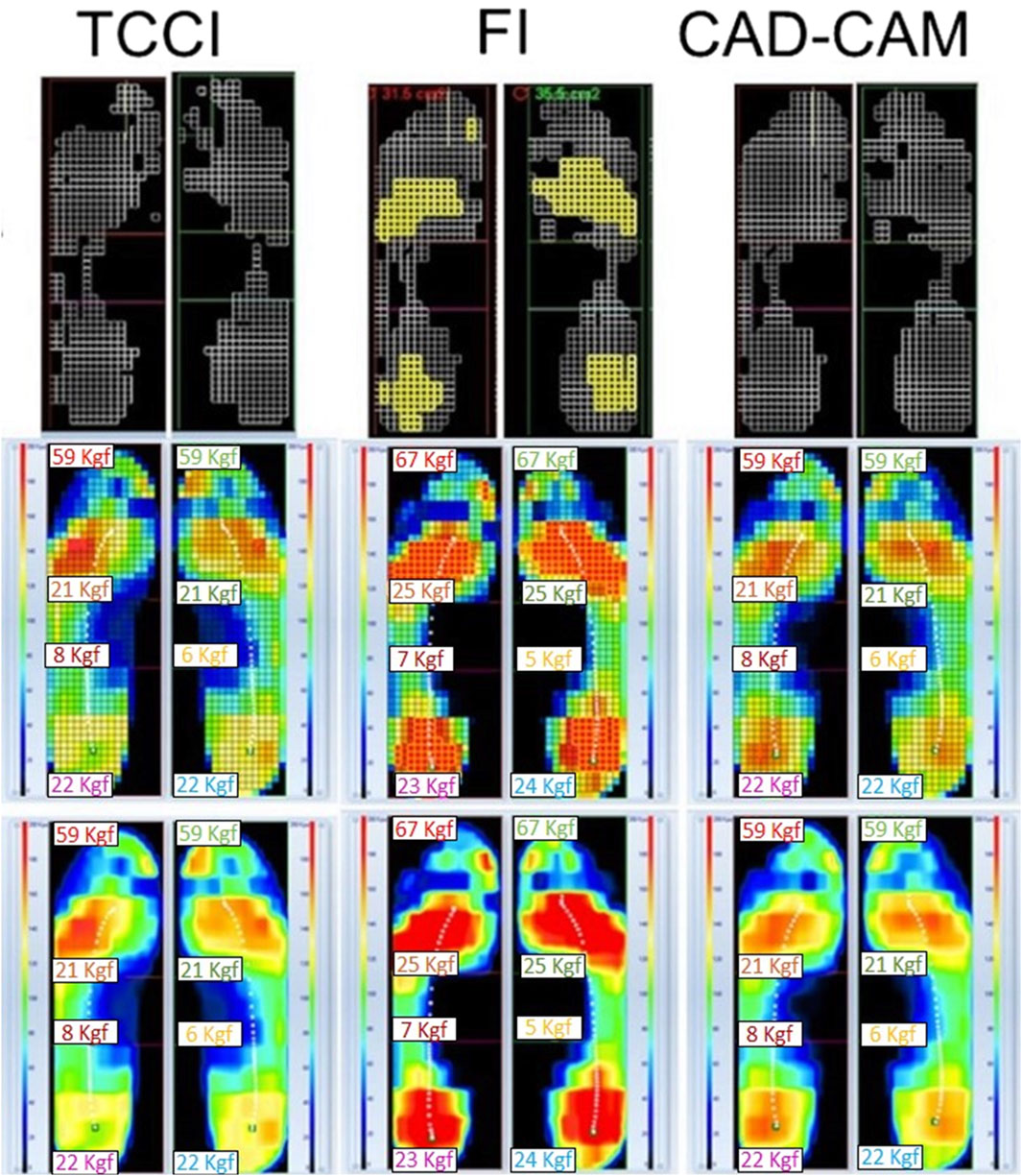
Figure 5. Comparison of pressure redistribution effects of three different shapes of insoles–flat insoles (FI), total contact customized insoles (TCCI), Computer Aided Design-Computer Aided Manufacturing (CAD-CAM) insoles (D’Amico et al., 2021).
3.1.2 Material of the diabetic foot insole
Mechanical property differences among materials affect the pressure offloading performance of customized insoles (Nouman et al., 2019; Haris et al., 2021). Telfer et al. (2014) identified footwear material elasticity as the most influential factor in pressure offloading. Studies comparing pressure offloading performance across different insole materials (Ma et al., 2019; Nouman et al., 2019; Tang et al., 2019; Chhikara et al., 2022) provide practical information for clinical prevention, guiding the design of more suitable insoles for diabetic patients. Soft insole materials, such as PORON Medical 4,708 and Nora Lunalastik EVA, outperformed rigid materials like Nora Lunalight A fresh and Pe-Lite in plantar pressure offloading for diabetic elderly individuals (Shi et al., 2022). Additionally, uniform softening of a homogeneous insole led to a 30% decrease in peak plantar pressure (Jafarzadeh et al., 2021). Nouman et al. (2021) found that a combination of soft and hard materials achieved a more uniform distribution of contact pressure (Figure 6). Table 2 provides a comparison of materials used in diabetic foot insoles.
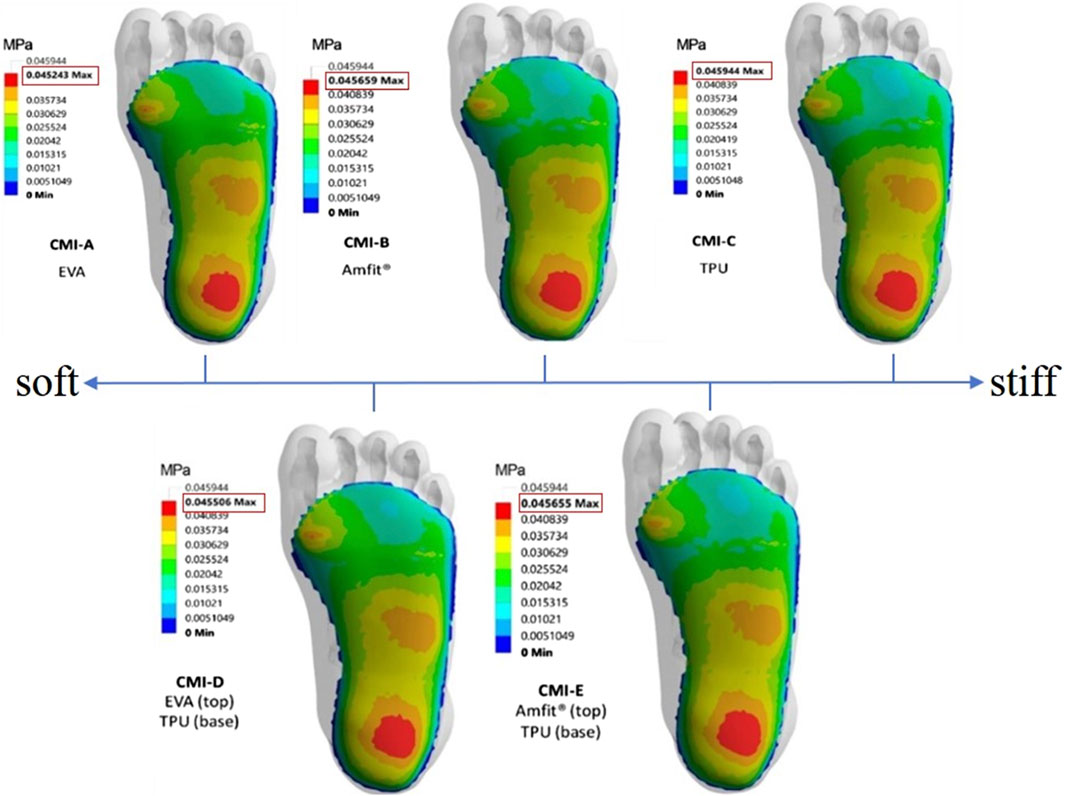
Figure 6. The contact pressure (MPa) distribution with soft material to stiffer material and its combinations (Nouman et al., 2021).
Commonly used plastic, rubber, and silicone-based diabetic foot insoles are non-degradable, non-absorbent, and lack comfort due to their inclusion of magnetic protrusions (Ning et al., 2022). To address these shortcomings, researchers focus on developing new materials with high comfort and superior mechanical performance. Chanda and Unnikrishnan (2018) proposed a novel customized insole made of a synthetic skin material, demonstrating increased effectiveness in stress offloading at ulcers compared to conventional materials. Polyvinyl alcohol/carboxymethyl cellulose (PVA/CMC) based magnetic hydrogels exhibited improved flexibility and tensile strength over conventional materials, providing enhanced comfort (Patwa et al., 2020). Material choice significantly influences insole pressure-offloading capabilities and comfort (Lo et al., 2014; Park et al., 2014) found that materials with low-density structures and large foam cell sizes were suitable for direct contact with the foot, and Rajan et al. (2016) investigated air and water vapor permeability, as well as thermal properties, relative to the porosity of spacer fabric insoles. Porosity influenced air and water vapor permeability.
3.1.3 Structure of the diabetic foot insole
The structure and material of diabetic foot insoles are closely intertwined. Single-layer insoles prioritize comfort, dual-layer insoles combine soft and rigid materials for both comfort and support, and multi-layered insoles use diverse materials for comprehensive support, control, and comfort. Different structures and materials yield varied effects on insole performance. Changing material alone may not simultaneously meet requirements for pressure offloading, comfort, and air permeability, necessitating structural optimization. Designing different porous structures within the insole can enhance pressure unloading and air permeability, offering adjustable parameters for personalized insoles. The internal structure of the insole also impacts the manufacturing process, constituting a crucial aspect of insole design.
Porous structures have gained traction in recent years for diabetic foot insole design. Tang et al. (2019) established relationships between equivalent modulus and structural parameters, enabling adjustable mechanical properties through various porous structure characteristics. Elliptical porous structural units, as proposed by Ma et al. (2019), demonstrated flexibility in adjusting geometric parameters and effective modulus for designing diabetic foot insoles (Shi et al., 2021). The honeycomb structure, employed for diabetic foot insoles, provides high stiffness and load-bearing capacity (Panda et al., 2018). Chen et al. (2021) developed an insole with a honeycomb and auxetic structure, effectively reducing pressure in the forefoot and rearfoot areas. Claybrook et al. (2022) suggested using a Split P TPMS structure to mimic medical-grade foams in diabetic foot orthoses, offering a range of compressive strengths by adjusting porosity. This structure can create a new generation of diabetic foot insoles adaptable to unique loading conditions. Compared to other porous structures, the elliptical porous structure and TPMS structure exhibit superior flexibility in adjusting geometric parameters, effective modulus, and porosity, facilitating personalized diabetic foot insole design. Table 3 summarizes representative porous structures.
Given the unique foot shape and condition of each individual, standardized pressure offloading diabetic foot insoles may not suffice to meet every patient’s specific needs. Personalized insole design tailored to individual foot characteristics is crucial to minimizing the risks of pain and injury. Additionally, assessing other mechanical indicators, such as pressure gradient angle, pressure time integral, pressure mean value, and pressure fluctuation index (Lung et al., 2016), alongside peak plantar pressure, can offer a more comprehensive evaluation of diabetic foot in future research.
3.2 Design of smart monitoring diabetic foot insoles
Advancements in intelligent and informative technology have paved the way for combining emerging technologies with traditional medical interventions for diabetic foot prevention and management (Lung et al., 2020). Smart insole systems, a prominent technology in this domain (Majumder and Deen, 2019; Macdonald et al., 2021), monitor plantar pressure or temperature and transmit data to the user’s smartphone or watch via Bluetooth. Users receive alerts to reduce pressure by adjusting posture or gait, offering a convenient self-monitoring and prevention method (Lee et al., 2017; Macdonald et al., 2021).
Several studies have proposed smart insoles for monitoring dynamic plantar foot forces in diabetic patients to aid in preventing diabetic foot complications. Ha Van et al. (2017) introduced a wireless pressure-sensitive insole, FeetMe One, for plantar pressure monitoring, calculating plantar pressure maps and multiple gait patterns. Wang et al. (2022) proposed the portable Insole (SLIPS) system, equipped with 64 tri-axial force sensors, for continuous monitoring of plantar pressure and shear stress. In addition to pressure, temperature monitoring has garnered interest due to its association with an increased risk of ulceration in diabetic patients (Hernandez-Contreras et al., 2016; Martín-Vaquero et al., 2019; Gordon et al., 2020). Khandakar et al. (2022) presented an intelligent insole system that measured both plantar pressure and temperature (Figure 7A). This system enabled real-time monitoring of foot pressure and temperature, aiding in the early detection of foot problems. Yavuz et al. (2020) designed Temperature and Pressure Monitoring and Regulating Insoles (TAPMARI), emphasizing the combined benefits of temperature monitoring and plantar pressure offloading in preventing Diabetic Foot Ulcers (DFU). Addressing the high recurrence rate of DFUs with standard nursing methods, smart insole systems like SurroSense Rx (Orpyx Medical Technologies Inc., Calgary, Canada) have been employed (Figure 7B) (Najafi et al., 2017; Abbott et al., 2019). Abbott et al. (2019) demonstrated the efficacy of the SurroSense Rx system in reducing DFU recurrence by providing continuous feedback on plantar pressure and alerting users to adjust pressure throughout daily life via a smartwatch. Moreover, the integration of an intelligent biofeedback system and smart insoles contributes to the development of a telemedicine service system, efficiently diagnosing, managing, and treating patients based on various gait characteristics (Figure 7C). A compilation of research on intelligent monitoring of diabetic foot insoles is presented in Table 4.
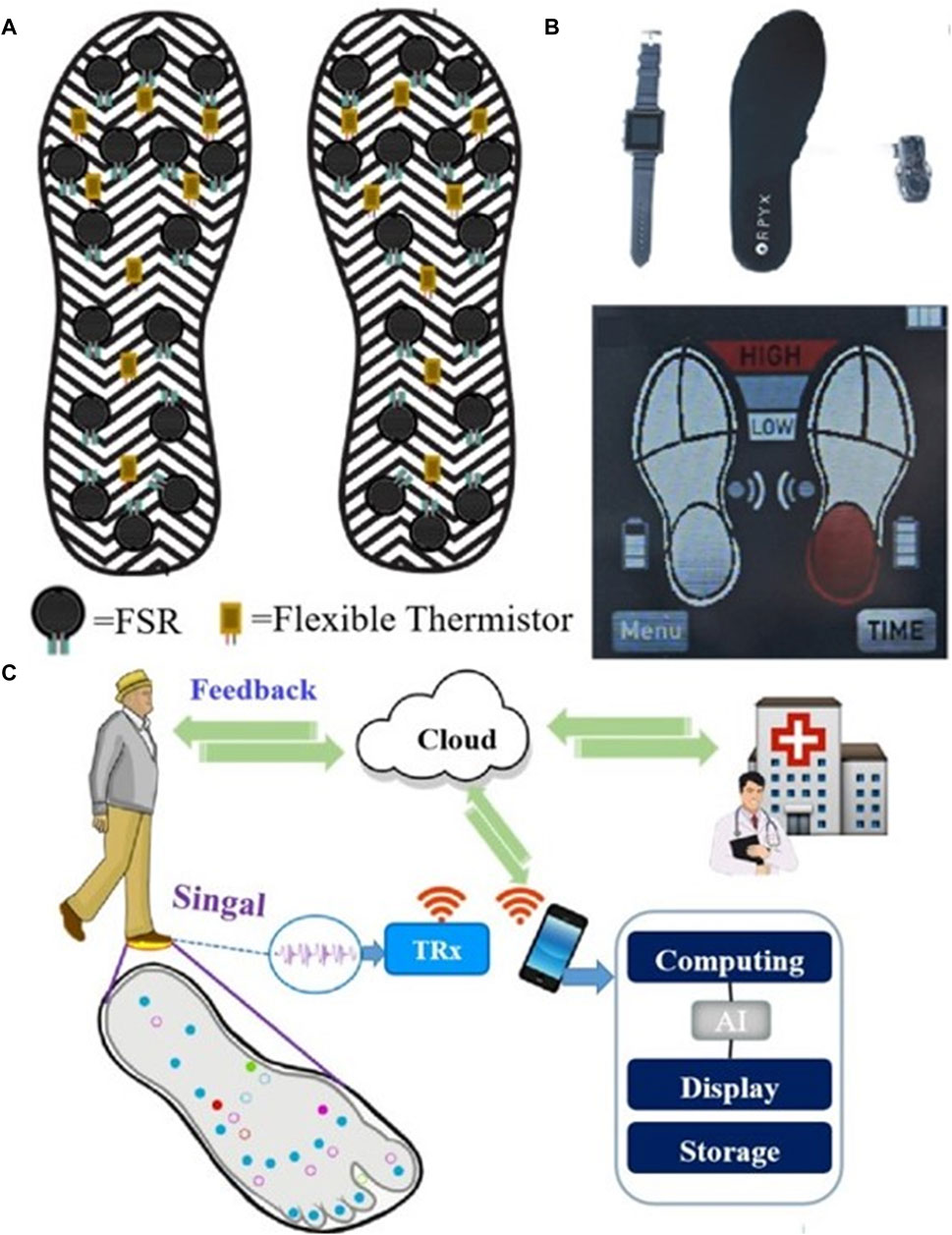
Figure 7. Smart monitoring insole. (A) The smart insole for monitoring plantar pressure and temperature with Force Sensitive Resistor (FSR) and Flexible Thermistor (Khandakar et al., 2022), (B) The sursense Rx smart insole system for monitoring plantar pressure (Abbott et al., 2019), and (C) An intelligent biofeedback system for older adults (Subramaniam et al., 2022).
Beyond monitoring pressure and temperature, it is crucial to consider the impact of humidity and acidity in diabetic foot care. Diabetic patients exhibit a diminished capacity to manage elevated heat and humidity compared to non-diabetic counterparts. The escalation of foot humidity during exercise or in higher ambient temperatures amplifies the risk of diabetic foot complications (Kularathne et al., 2019). Moreover, bacterial proliferation in ulcers can lead to increased wound acidity, contributing to the development of foot ulcers (Salvo et al., 2017b). Addressing these concerns, Kularathne et al. (2019) innovatively designed a device that seamlessly connects to any diabetic shoe, monitoring patients’ steps, weight, foot temperature, and humidity. In a parallel effort, Salvo et al. (2017a; Salvo et al., 2017b) developed a wearable sensor capable of tracking temperature and acidity changes in foot ulcers of diabetes patients over a week. Nevertheless, the existing literature lacks sufficient reporting on insoles designed explicitly for monitoring and mitigating foot humidity and acidity, underscoring the need for further research and evidence in this critical domain.
Despite the proven efficacy of intelligent monitoring in preventing and treating diabetic foot conditions, ensuring patient compliance is paramount. Prolonged wearing and continuous connection requirements for data uploading underscore the necessity for maintaining compliance to ensure accurate data collection and preserve the effectiveness of diagnosis and treatment.
4 Additive manufacturing of diabetic foot insoles
Traditional customized foot orthotics have historically been crafted through subtractive manufacturing processes, involving the carving or milling of materials to achieve the desired orthotic shape (Newman et al., 2015). This method, however, tends to be time-consuming, labor-intensive, and generates waste through excess material removal (Paris et al., 2016). In contrast, additively manufactured foot orthoses offer advantages such as high precision, customization, rapid production, and environmental sustainability. These attributes contribute to enhanced biomechanical control, comfort, risk reduction for pressure points, and shortened delivery times, ultimately expanding patient access to customized orthotics (Jin et al., 2015; Chen et al., 2016; Paris et al., 2016).
As a burgeoning technology, additive manufacturing (AM) is gradually finding application in the production of diabetic foot insoles (Srivastava and Gaur, 2020). AM constructs three-dimensional objects by incrementally adding thin layers of materials guided by digital models (Wong and Hernandez, 2012). Its adoption streamlines production processes with short development cycles, and it is extensively utilized in the biomedical field (DebRoy et al., 2018). There are various additive manufacturing (AM) technologies, encompassing Selective Laser Sintering (SLS), Selective Laser Melting (SLM), Stereolithography (SLA), Fused Deposition Modeling (FDM), and 3D Printing (3DP) (Chen et al., 2016; Abdulhameed et al., 2019). Notably, FDM and SLS predominantly manufacture customized insoles for diabetic foot patients, as depicted in Figures 8A, B. FDM is the most commonly used technology for crafting customized insoles for diabetic feet, creating three-dimensional structures by depositing thermoplastic material onto a substrate in layers through a temperature-controlled printhead (Winarso et al., 2022). Research by Davia-Aracil et al. (2018) comparing traditional subtractive techniques to FDM for manufacturing anatomical insoles found FDM to be 43% more cost-efficient and time-effective. Ma et al. (2019) employed material extrusion techniques, including FDM and fused filament fabrication (FFF), to print adjustable modulus porous structures for diabetic foot insoles, demonstrating their mechanical effectiveness. Peker et al. (2020) implemented an upgraded FDM system to manufacture diabetic foot insoles with reduced production time.
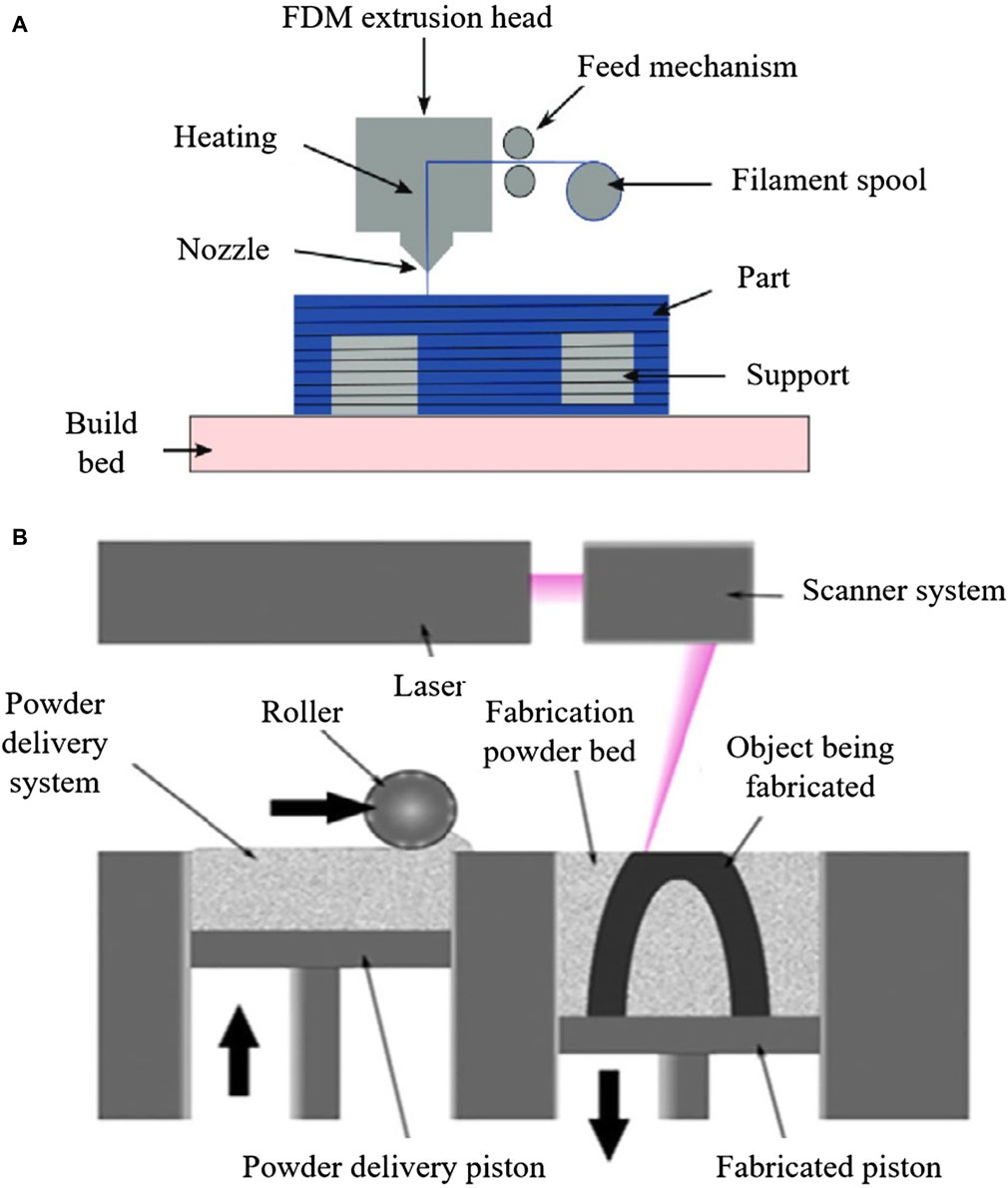
Figure 8. AM process for manufacturing diabetic foot insoles. (A) Illustration of the FDM process (Alafaghani et al., 2017). (B) Illustration of SLS Process (Abdulhameed et al., 2019).
While FDM is the most economical technique for creating entirely geometric customized insoles (Vyavahare et al., 2019), it has limitations. Interlayer bonding characteristics in FDM result in printed parts with highly anisotropic mechanical properties, potentially leading to early failure (Rankouhi et al., 2016; Lim et al., 2021). Additionally, materials used in FDM may exhibit suboptimal durability and mechanical properties (Hudak et al., 2022).
Another AM technology used for customized diabetic foot insoles is SLS, an industrial 3D printing method utilizing a powder bed to construct 3D objects (Fina et al., 2017). SLS involves selectively melting a thin layer of powdered material on a build platform according to a 3D model using a laser. This process is repeated layer by layer until the final object is formed (Mazzoli, 2013), allowing the production of complex structures with superior dimensional accuracy and strength (Awad et al., 2020). Li et al. (2022) highlighted SLS’s ability to successfully fabricate the unit array design beneath the insole’s lower surface. In comparison to FDM, SLS exhibits greater potential for manufacturing insoles with intricate structures. Table 5 provides a comparative overview of AM methods for diabetic foot insoles.
5 Performance evaluation of diabetic foot insoles
The evaluation of diabetic foot insoles encompasses both objective and subjective assessments. Objectively, evaluations focus on plantar pressure distribution analysis, gait analysis, and electromyography, primarily examining the pressure offloading performance of diabetic foot insoles (Bellomo et al., 2022). Prior to clinical application, a crucial step involves the performance evaluation of customized insoles for diabetic feet, ensuring accurate and efficient feedback to enhance the design (Anderson et al., 2020; Song et al., 2022).
The in silico method, also known as the numerical method, employs computational models to numerically evaluate a product’s performance (Anderson et al., 2021). Once developed and validated, it efficiently assesses the pressure-offloading performance of customized insoles (Peng et al., 2021). Unlike conventional experiments that involve human participants, in silico approaches, such as FE modeling, offer a more precise alternative by isolating the effects of specific insole design variables without the need for physical experiments on human subjects or models (Behforootan et al., 2017). However, the current challenge lies in developing valid computational models. Musculoskeletal models of the foot and foot-insole FE models have become common computational tools for evaluating diabetic foot insoles (Keenan et al., 2022). The musculoskeletal model is crafted to emulate human movement by integrating rigid bones, muscle fibers, tendons, ligaments, and other connective tissues crucial for understanding and predicting human activities (Falisse et al., 2019). Utilizing these models allows for an assessment of how insole design parameters impact everyday gait. Chen et al. (2015) introduced a 3D FE musculoskeletal model of the foot, providing a tool to evaluate the pressure-offloading performance of insoles made from different materials and thicknesses. Similarly, Jafarzadeh et al. (2021) employed a foot-insole FE model to scrutinize the influence of insole stiffness on shape optimization outcomes. Despite their utility, developing musculoskeletal models remains challenging in the current stage of research. These models are simplified representations, and as such, the boundary conditions derived from them may lack accuracy and appropriateness. The complexity of accurately capturing the intricacies of human biomechanics poses ongoing challenges in refining musculoskeletal models for robust and reliable evaluations of insole designs.
The in vitro evaluation method employs laboratory testing to assess the performance of diabetic foot insoles (Zhang et al., 2019). This testing utilizes a pressure measurement system to gauge the plantar pressure of subjects during both gait and standing (Burns et al., 2019; Anzai et al., 2022). The experimental results are then compared with FE simulation results to evaluate the pressure-offloading performance of the insoles. In its early stages, the F-Scan system served as the primary pressure measurement system; however, it encountered issues of poor accuracy and repeatability (Lorkowski et al., 2021). Subsequently, with advancements in science and technology, the Pedar system, renowned for its superior performance, has become widely adopted for pressure measurement (Yamamoto et al., 2020). Numerous researchers have conducted pressure tests on diabetic foot insoles using the Pedar system. Nouman et al. (2021), for instance, utilized the Pedar system to measure peak plantar pressure in diabetic foot patients while maintaining balance on level ground. The experimental average peak plantar pressure was then compared with the peak contact pressure obtained from FEA for validation. Tang et al. (2019) employed the Pedar-x insole pressure measurement system (Novel Pedar System, Germany) to measure contact pressure between feet and insoles during static standing with arms akimbo, assessing the insoles’ performance. D’Amico et al. (2021) utilized the Pedar-X system to measure in-shoe dynamic plantar pressure during walking in multiple trials along a 15 m walkway in a laboratory setting. Shi et al. (2022) obtained pressure values using the Pedar insole measurement system (Novel GmbH, Munich, Germany) to evaluate four different insoles during walking. Participants self-selected walking speeds to exhibit their typical gait characteristics on a 10 m straight sidewalk while barefoot and wearing insoles. A summary of in vitro performance evaluations of diabetic foot insoles is presented in Table 6.
Subjective evaluation primarily involves collecting patient feedback on comfort, compliance, and overall satisfaction, typically obtained through randomized trials and questionnaires. Lavery et al. (2012) conducted a randomized trial with 299 patients, assessing standard care versus reduced insoles over 18 months. Patient questionnaires revealed insights into compliance and durability, impacting the effectiveness of shear-reducing insoles in preventing diabetic foot ulcers. In another study, Ming et al. (2019) conducted a 24-month trial evaluating sensor-equipped insoles for measuring foot temperature in 300 participants with diabetes and severe peripheral neuropathy. While objective measurements offer quantitative data, they may require specialized equipment or expertise. In contrast, subjective measurements provide qualitative data and may be more manageable. Combining both approaches could yield a more comprehensive assessment of diabetic insole effectiveness. A comparison of the advantages and disadvantages of each evaluation method is detailed in Table 7.
6 Challenges and future perspectives
In summary, customized insoles for diabetic individuals exhibit significant potential in effectively offloading plantar pressure, contributing substantially to early prevention, pain reduction, and economic burden alleviation. The continuous evolution of smart insoles for diabetic foot care is paving the way for an improved remote digital healthcare system (Ahmed et al., 2020). The ongoing advancement of smart insoles for diabetic foot care is paving the path toward an enhanced remote digital healthcare system. Nonetheless, despite the relatively comprehensive research on diabetic foot insoles, numerous limitations still require further exploration. Consequently, existing research encounters several challenges, and the following issues are underscored, along with corresponding suggestions for the design and implementation of diabetic foot insoles.
(1) Biomechanical Correspondence: Future studies must comprehensively consider the biomechanical correspondence between various tissues in the human foot, including bones, muscles, and fascia (Allan et al., 2016; Asghar and Naaz, 2022). Evaluating joint angles, muscle activity, and skeletal stress and strain is crucial for effective studies on diabetic foot, offering insights into foot motion, stability, muscle coordination, functionality, and predicting disease risks.
(2) Personalization in Design: Designing diabetic foot insoles should prioritize personalization in future studies (Lorkowski et al., 2021). While current research often bases designs on typical foot structures, the efficiency of insoles can be highly individualized due to differences in foot structure, arch type, or disease characteristics. Comprehensive consideration of the foot characteristics and conditions of diabetic patients is essential in the design and manufacturing process (Chen et al., 2016; Chhikara et al., 2022; Jin et al., 2015).
(3) Expanded Mechanical Indicators: In addition to peak pressure and shear force, future evaluations of diabetic foot should incorporate various mechanical indicators, including peak pressure gradient, pressure-time integral, pressure mean, pressure fluctuation index, foot skeletal stress, and contact area (Lung et al., 2016). These indicators offer a more comprehensive analysis, guiding the design of diabetic foot insoles effectively.
(4) Diversified Gait Simulation: To enhance the relevance of findings, future studies should expand the diversity of gait simulations. While many studies focus on neutral stationary stances and normal walking gaits, daily life involves varied and changing gaits (Nouman et al., 2019). Accounting for this diversity will reduce errors in pressure analysis results, aligning them more closely with actual plantar pressure distribution.
(5) Improved Patient Adherence: Enhancing patient adherence is crucial for the effectiveness of pressure-offloading insoles (Park et al., 2014). Adequate training on innovative insole technology is necessary to achieve the expected pressure-offloading effect of intelligent insoles, emphasizing the need for comprehensive patient education and support.
Based on the challenges highlighted earlier, several future research directions for the advancement of diabetic foot insoles can be outlined. These include:
(1) Reverse Design with Machine Learning: Utilizing machine learning for reverse design of insoles tailored to different levels of Diabetic Foot Ulcers (DFU). This involves exploring data characteristics, model training for parameter optimization, and selecting optimal insole designs, significantly reducing the overall design cycle.
(2) Topology Optimization with 3D Printing: Combining topology optimization technology with 3D printing to create lightweight insoles with guaranteed mechanical properties (Davia-Aracil et al., 2018; Awad et al., 2020). This approach involves designing personalized insoles with a dot matrix structure to optimize pressure distribution under the foot.
(3) Integration of Flexible Sensors: Integrating flexible sensors into smart insoles, utilizing sensors made of flexible materials that offer good flexibility, elasticity, and bendability (Cheung and Zhang, 2008; Chatwin et al., 2020). Flexible resistive pressure sensors can capture real-time information on plantar pressure changes, contributing to the development of intelligent and comfortable diabetic foot insoles.
(4) Monitoring Foot Humidity: Considering the monitoring of foot humidity for diabetic patients. This is particularly relevant given the reduced ability of individuals with diabetes to cope with high heat and humidity. Monitoring foot humidity can provide valuable information for reducing the risk of diabetic foot development (Ning et al., 2022). Despite this importance, there is a notable gap in devices reported in existing literature for addressing foot humidity concerns.
7 Conclusion
This review has provided a comprehensive overview of the design, manufacturing, and performance evaluation of diabetic foot insoles. The design phase often employs iterative optimization methods rooted in biomechanics and FEA, allowing for the prediction of design parameters associated with insoles that offer superior pressure offloading effects. The discourse also delves into the evolving landscape of smart insoles designed specifically for diabetic foot care. In the realm of manufacturing, while Fused Deposition Modeling (FDM) stands out for its cost-effectiveness and efficiency, its limitations in printing complex structures necessitate the use of Selective Laser Sintering (SLS) for intricate insole designs. Insoles featuring total contact customization, softer and absorbent materials, elliptical porous structures, or TPMS structures are deemed more fitting for diabetic foot prevention, taking into account both pressure offloading performance and comfort. Prior to clinical application, a thorough evaluation of insole performance through in silico and in vitro testing approaches is crucial. Despite the strides made in exploring various facets of diabetic foot insoles, there are avenues for further research. Future investigations could explore inverse design strategies leveraging machine learning, use topology optimization techniques for lightweight insole development, integrate flexible sensors for enhanced functionality, and delve into the development of novel skin-like materials tailored specifically for diabetic foot insoles. These advancements hold promise for refining the efficacy, personalization, and innovation of diabetic foot care technologies.
Author contributions
YR: Funding acquisition, Resources, Writing–original draft. HW: Conceptualization, Writing–original draft. XS: Conceptualization, Writing–original draft. YW: Resources, Writing–review and editing. YL: Conceptualization, Funding acquisition, Writing–review and editing. WZ: Conceptualization, Resources, Writing–review and editing.
Funding
The author(s) declare that financial support was received for the research, authorship, and/or publication of this article. This work was supported in part by the National Key R&D Program of China (Key Special Project for Marine Environmental Security and Sustainable Development of Coral Reefs 2022-2.1), Dalian Key Laboratory of Digital Medicine for Critical Diseases, the Fundamental Research Funds for the Central Universities (No: DUT23YG217) and the Dalian University of Technology and Affiliated Central Hospital joint research fund (No: 2023ZXYG15) (YR, HW, XS, YW, YL).
Conflict of interest
The authors declare that the research was conducted in the absence of any commercial or financial relationships that could be construed as a potential conflict of interest.
Publisher’s note
All claims expressed in this article are solely those of the authors and do not necessarily represent those of their affiliated organizations, or those of the publisher, the editors and the reviewers. Any product that may be evaluated in this article, or claim that may be made by its manufacturer, is not guaranteed or endorsed by the publisher.
References
Abbott, C. A., Chatwin, K. E., Foden, P., Hasan, A. N., Sange, C., Rajbhandari, S. M., et al. (2019). Innovative intelligent insole system reduces diabetic foot ulcer recurrence at plantar sites: a prospective, randomised, proof-of-concept study. Lancet Digital Health 1, e308–e318. doi:10.1016/S2589-7500(19)30128-1
Abdulhameed, O., Al-Ahmari, A., Ameen, W., and Mian, S. H. (2019). Additive manufacturing: challenges, trends, and applications. Adv. Mech. Eng. 11, 168781401882288. doi:10.1177/1687814018822880
Ahmed, S., Barwick, A., Butterworth, P., and Nancarrow, S. (2020). Footwear and insole design features that reduce neuropathic plantar forefoot ulcer risk in people with diabetes: a systematic literature review. J. Foot Ankle Res. 13, 30. doi:10.1186/s13047-020-00400-4
Alafaghani, A., Qattawi, A., Alrawi, B., and Guzman, A. (2017). Experimental optimization of fused deposition modelling processing parameters: a design-for-manufacturing approach. Procedia Manuf. 10, 791–803. doi:10.1016/j.promfg.2017.07.079
Aliberti, S., de, S. X., Costa, M., de Campos Passaro, A., Arnone, A. C., Hirata, R., et al. (2011). Influence of patellofemoral pain syndrome on plantar pressure in the foot rollover process during gait. Clinics 66, 367–372. doi:10.1590/S1807-59322011000300001
Allan, J., Munro, W., and Figgins, E. (2016). Foot deformities within the diabetic foot and their influence on biomechanics: a review of the literature. Prosthetics Orthot. Int. 40, 182–192. doi:10.1177/0309364615592705
Anderson, J., Williams, A. E., and Nester, C. (2020). Development and evaluation of a dual density insole for people standing for long periods of time at work. J. Foot Ankle Res. 13, 42. doi:10.1186/s13047-020-00402-2
Anderson, R., Andrej, J., Barker, A., Bramwell, J., Camier, J.-S., Cerveny, J., et al. (2021). MFEM: a modular finite element methods library. Comput. Math. Appl. 81, 42–74. doi:10.1016/j.camwa.2020.06.009
Anzai, E., Ren, D., Cazenille, L., Aubert-Kato, N., Tripette, J., and Ohta, Y. (2022). Random forest algorithms to classify frailty and falling history in seniors using plantar pressure measurement insoles: a large-scale feasibility study. BMC Geriatr. 22, 746. doi:10.1186/s12877-022-03425-5
Apelqvist, J. (2012). Diagnostics and treatment of the diabetic foot. Endocrine 41, 384–397. doi:10.1007/s12020-012-9619-x
Armstrong, D. G., Swerdlow, M. A., Armstrong, A. A., Conte, M. S., Padula, W. V., and Bus, S. A. (2020). Five year mortality and direct costs of care for people with diabetic foot complications are comparable to cancer. J. Foot Ankle Res. 13, 16. doi:10.1186/s13047-020-00383-2
Asghar, A., and Naaz, S. (2022). The transverse arch in the human feet: a narrative review of its evolution, anatomy, biomechanics and clinical implications. Morphologie 106, 225–234. doi:10.1016/j.morpho.2021.07.005
Awad, A., Fina, F., Goyanes, A., Gaisford, S., and Basit, A. W. (2020). 3D printing: principles and pharmaceutical applications of selective laser sintering. Int. J. Pharm. 586, 119594. doi:10.1016/j.ijpharm.2020.119594
Behforootan, S., Chatzistergos, P., Naemi, R., and Chockalingam, N. (2017). Finite element modelling of the foot for clinical application: a systematic review. Med. Eng. Phys. 39, 1–11. doi:10.1016/j.medengphy.2016.10.011
Bellomo, T. R., Lee, S., McCarthy, M., Tong, K. P. S., Ferreira, S. S., Cheung, T. P., et al. (2022). Management of the diabetic foot. Seminars Vasc. Surg. 35, 219–227. doi:10.1053/j.semvascsurg.2022.04.002
Burns, G. T., Deneweth Zendler, J., and Zernicke, R. F. (2019). Validation of a wireless shoe insole for ground reaction force measurement. J. Sports Sci. 37, 1129–1138. doi:10.1080/02640414.2018.1545515
Bus, S. A., Akkerman, E. M., and Maas, M. (2021). Changes in sub-calcaneal fat pad composition and their association with dynamic plantar foot pressure in people with diabetic neuropathy. Clin. Biomech. 88, 105441. doi:10.1016/j.clinbiomech.2021.105441
Chanda, A., and Unnikrishnan, V. (2018). Novel insole design for diabetic foot ulcer management. Proc. Institution Mech. Eng. Part H J. Eng. Med. 232, 1182–1195. doi:10.1177/0954411918808330
Chatwin, K. E., Abbott, C. A., Boulton, A. J. M., Bowling, F. L., and Reeves, N. D. (2020). The role of foot pressure measurement in the prediction and prevention of diabetic foot ulceration—a comprehensive review. Diabetes/Metabolism Res. Rev. 36, e3258. doi:10.1002/dmrr.3258
Chen, R. K., Jin, Y., Wensman, J., and Shih, A. (2016). Additive manufacturing of custom orthoses and prostheses—a review. Addit. Manuf. 12, 77–89. doi:10.1016/j.addma.2016.04.002
Chen, T., Tian, M., and Wang, X. (2021). A novel porous structural design of the orthotic insole for diabetic foot. Int. Conf. Comput. Control Robotics, 188–192. doi:10.1109/ICCCR49711.2021.9349389
Chen, W.-M., Lee, S.-J., and Lee, P. V. S. (2015). Plantar pressure relief under the metatarsal heads – therapeutic insole design using three-dimensional finite element model of the foot. J. Biomechanics 48, 659–665. doi:10.1016/j.jbiomech.2014.12.043
Cheung, J. T.-M., and Zhang, M. (2008). Parametric design of pressure-relieving foot orthosis using statistics-based finite element method. Med. Eng. Phys. 30, 269–277. doi:10.1016/j.medengphy.2007.05.002
Chhikara, K., Singh, G., Gupta, S., and Chanda, A. (2022). Progress of additive manufacturing in fabrication of foot orthoses for diabetic patients: a review. Ann. 3D Print. Med. 8, 100085. doi:10.1016/j.stlm.2022.100085
Claybrook, F., Mohammed, M., and Southee, D. (2022). Investigation of additive manufactured Split P TPMS elastomeric structures for diabetic foot insoles. Trans. Addit. Manuf. Meets Med., 664. doi:10.18416/AMMM.2022.2209664
Collings, R., Freeman, J., Latour, J. M., and Paton, J. (2021). Footwear and insole design features for offloading the diabetic at risk foot—a systematic review and meta-analyses. Endocrinol. Diab Metab. 4, e00132. doi:10.1002/edm2.132
D’Amico, M., Kinel, E., Roncoletta, P., Gnaldi, A., Ceppitelli, C., Belli, F., et al. (2021). Data-driven CAD-CAM vs traditional total contact custom insoles: a novel quantitative-statistical framework for the evaluation of insoles offloading performance in diabetic foot. PLoS One 16, e0247915. doi:10.1371/journal.pone.0247915
Davia-Aracil, M., Hinojo-Pérez, J. J., Jimeno-Morenilla, A., and Mora-Mora, H. (2018). 3D printing of functional anatomical insoles. Comput. Industry 95, 38–53. doi:10.1016/j.compind.2017.12.001
DebRoy, T., Wei, H. L., Zuback, J. S., Mukherjee, T., Elmer, J. W., Milewski, J. O., et al. (2018). Additive manufacturing of metallic components – process, structure and properties. Prog. Mater. Sci. 92, 112–224. doi:10.1016/j.pmatsci.2017.10.001
Deschamps, K., Matricali, G. A., Roosen, P., Desloovere, K., Bruyninckx, H., Spaepen, P., et al. (2013). Classification of forefoot plantar pressure distribution in persons with diabetes: a novel perspective for the mechanical management of diabetic foot? PLoS One 8, e79924. doi:10.1371/journal.pone.0079924
Edmonds, M., Manu, C., and Vas, P. (2021). The current burden of diabetic foot disease. J. Clin. Orthop. Trauma 17, 88–93. doi:10.1016/j.jcot.2021.01.017
El-Hilaly, R., Elshazly, O., and Amer, A. (2013). The role of a total contact insole in diminishing foot pressures following partial first ray amputation in diabetic patients. Foot 23, 6–10. doi:10.1016/j.foot.2012.10.002
Erdemir, A., Guess, T. M., Halloran, J., Tadepalli, S. C., and Morrison, T. M. (2012). Considerations for reporting finite element analysis studies in biomechanics. J. Biomechanics 45, 625–633. doi:10.1016/j.jbiomech.2011.11.038
Falisse, A., Serrancolí, G., Dembia, C. L., Gillis, J., Jonkers, I., and De Groote, F. (2019). Rapid predictive simulations with complex musculoskeletal models suggest that diverse healthy and pathological human gaits can emerge from similar control strategies. J. R. Soc. Interface 16, 20190402. doi:10.1098/rsif.2019.0402
Fernando, M. E., Crowther, R. G., Lazzarini, P. A., Sangla, K. S., Wearing, S., Buttner, P., et al. (2016). Plantar pressures are higher in cases with diabetic foot ulcers compared to controls despite a longer stance phase duration. BMC Endocr. Disord. 16, 51. doi:10.1186/s12902-016-0131-9
Fina, F., Goyanes, A., Gaisford, S., and Basit, A. W. (2017). Selective laser sintering (SLS) 3D printing of medicines. Int. J. Pharm. 529, 285–293. doi:10.1016/j.ijpharm.2017.06.082
Ghassemi, A., Mossayebi, A. R., Jamshidi, N., Naemi, R., and Karimi, M. T. (2015). Manufacturing and finite element assessment of a novel pressure reducing insole for Diabetic Neuropathic patients. Australas. Phys. Eng. Sci. Med. 38, 63–70. doi:10.1007/s13246-014-0319-0
Gordon, I. L., Rothenberg, G. M., Lepow, B. D., Petersen, B. J., Linders, D. R., Bloom, J. D., et al. (2020). Accuracy of a foot temperature monitoring mat for predicting diabetic foot ulcers in patients with recent wounds or partial foot amputation. Diabetes Res. Clin. Pract. 161, 108074. doi:10.1016/j.diabres.2020.108074
Haris, F., Liau, B.-Y., Jan, Y.-K., Akbari, V. B. H., Primanda, Y., Lin, K.-H., et al. (2021). A review of the plantar pressure distribution effects from insole materials and at different walking speeds. Appl. Sci. 11, 11851. doi:10.3390/app112411851
Ha Van, G., Haddad, J., Bensimon, Y., Dillard, L., and Jacobs, D. (2017). Biofeedback strategy and reduction of foot pressure in diabetic patient with neuropathy: benefit of connected insoles FEETME: a preliminary study. Ann. Phys. Rehabilitation Med. 60, e40. doi:10.1016/j.rehab.2017.07.178
Hernandez-Contreras, D., Peregrina-Barreto, H., Rangel-Magdaleno, J., and Gonzalez-Bernal, J. (2016). Narrative review: diabetic foot and infrared thermography. Infrared Phys. Technol. 78, 105–117. doi:10.1016/j.infrared.2016.07.013
Hudak, Y. F., Li, J.-S., Cullum, S., Strzelecki, B. M., Richburg, C., Kaufman, G. E., et al. (2022). A novel workflow to fabricate a patient-specific 3D printed accommodative foot orthosis with personalized latticed metamaterial. Med. Eng. Phys. 104, 103802. doi:10.1016/j.medengphy.2022.103802
Jafarzadeh, E., Soheilifard, R., and Ehsani-Seresht, A. (2021). Design optimization procedure for an orthopedic insole having a continuously variable stiffness/shape to reduce the plantar pressure in the foot of a diabetic patient. Med. Eng. Phys. 98, 44–49. doi:10.1016/j.medengphy.2021.10.008
Jin, Y., Plott, J., Chen, R., Wensman, J., and Shih, A. (2015). Additive manufacturing of custom orthoses and prostheses – a review. Procedia CIRP 36, 199–204. doi:10.1016/j.procir.2015.02.125
Jodheea-Jutton, A., Hindocha, S., and Bhaw-Luximon, A. (2022). Health economics of diabetic foot ulcer and recent trends to accelerate treatment. Foot 52, 101909. doi:10.1016/j.foot.2022.101909
Jones, A. D., De Siqueira, J., Nixon, J. E., Siddle, H. J., Culmer, P. R., and Russell, D. A. (2022). Plantar shear stress in the diabetic foot: a systematic review and meta-analysis. Diabet. Med. 39, e14661. doi:10.1111/dme.14661
Keenan, B. E., Evans, S. L., and Oomens, C. W. J. (2022). A review of foot finite element modelling for pressure ulcer prevention in bedrest: current perspectives and future recommendations. J. Tissue Viability 31, 73–83. doi:10.1016/j.jtv.2021.06.004
Kerr, M., Barron, E., Chadwick, P., Evans, T., Kong, W. M., Rayman, G., et al. (2019). The cost of diabetic foot ulcers and amputations to the National Health Service in England. Diabet. Med. 36, 995–1002. doi:10.1111/dme.13973
Khandakar, A., Mahmud, S., Chowdhury, M. E. H., Reaz, M. B. I., Kiranyaz, S., Mahbub, Z. B., et al. (2022). Design and implementation of a smart insole system to measure plantar pressure and temperature. Sensors 22, 7599. doi:10.3390/s22197599
Korada, H., Maiya, A., Rao, S. K., and Hande, M. (2020). Effectiveness of customized insoles on maximum plantar pressure in diabetic foot syndrome: a systematic review. Diabetes & Metabolic Syndrome Clin. Res. Rev. 14, 1093–1099. doi:10.1016/j.dsx.2020.06.041
Kularathne, N., Wijayathilaka, U., Kottawaththa, N., Hewakoralage, S., and Thelijjagoda, S. (2019). “Dia-shoe: a smart diabetic shoe to monitor and prevent diabetic foot ulcers,” in 2019 International Conference on Advancements in Computing, USA, 5-7 Dec. 2019 (IEEE), 410–415. doi:10.1109/ICAC49085.2019.9103408
Kumar, K. R., Vinothkumar, P., and Soms, N. (2022). Investigation on the development of custom foot insole using soft polylactic acid by fused deposition modelling technique. J. Mater. Eng. Perform. 32, 1790–1796. doi:10.1007/s11665-022-07208-2
Lavery, L. A., LaFontaine, J., Higgins, K. R., Lanctot, D. R., and Constantinides, G. (2012). Shear-reducing insoles to prevent foot ulceration in high-risk diabetic patients. Adv. Skin Wound Care 25, 519–524. doi:10.1097/01.ASW.0000422625.17407.93
Lee, S.-H., Lee, H.-J., Chang, W. H., Choi, B.-O., Lee, J., Kim, J., et al. (2017). Gait performance and foot pressure distribution during wearable robot-assisted gait in elderly adults. J. NeuroEngineering Rehabilitation 14, 123. doi:10.1186/s12984-017-0333-z
Li, J., Shu, W., Yang, Y., and Yan, R. (2020). Parametric modeling and performance analysis of a personalized insole based on 3D scanning and selective laser sintering. Int. J. Comput. Integr. Manuf. 33, 936–945. doi:10.1080/0951192X.2020.1815849
Li, J., Wei, Y., Zhao, Z., and Yao, D. (2022). Improvement of mechanical properties of personalized insole manufactured with selective laser sintering based on process parameter optimization and cell structure design. 3D Print. Addit. Manuf. 10, 992–1002. doi:10.1089/3dp.2022.0080
Lim, J. Z. M., Ng, N. S. L., and Thomas, C. (2017). Prevention and treatment of diabetic foot ulcers. J. R. Soc. Med. 110, 104–109. doi:10.1177/0141076816688346
Lim, T., Cheng, H., Song, W., Lee, J., Kim, S., and Jung, W. (2021). Simulated and experimental investigation of mechanical properties for improving isotropic fracture strength of 3D-printed capsules. Materials 14, 4677. doi:10.3390/ma14164677
Lo, W. T., Yick, K. L., Ng, S. P., and Yip, J. (2014). New methods for evaluating physical and thermal comfort properties of orthotic materials used in insoles for patients with diabetes. J. Rehabilitation Res. Dev. 51, 311–324. doi:10.1682/jrrd.2013.01.0012
Lorkowski, J., Gawronska, K., and Pokorski, M. (2021). Pedobarography: a review on methods and practical use in foot disorders. Appl. Sci. 11, 11020. doi:10.3390/app112211020
Lung, C.-W., Hsiao-Wecksler, E. T., Burns, S., Lin, F., and Jan, Y.-K. (2016). Quantifying dynamic changes in plantar pressure gradient in diabetics with peripheral neuropathy. Front. Bioeng. Biotechnol. 4, 54. doi:10.3389/fbioe.2016.00054
Lung, C.-W., Wu, F.-L., Liao, F., Pu, F., Fan, Y., and Jan, Y.-K. (2020). Emerging technologies for the prevention and management of diabetic foot ulcers. J. Tissue Viability 29, 61–68. doi:10.1016/j.jtv.2020.03.003
Ma, Z., Lin, J., Xu, X., Ma, Z., Tang, L., Sun, C., et al. (2019). Design and 3D printing of adjustable modulus porous structures for customized diabetic foot insoles. Int. J. Lightweight Mater. Manuf. 2, 57–63. doi:10.1016/j.ijlmm.2018.10.003
Macdonald, E. M., Perrin, B. M., Cleeland, L., and Kingsley, M. I. C. (2021). Podiatrist-delivered health coaching to facilitate the use of a smart insole to support foot health monitoring in people with diabetes-related peripheral neuropathy. Sensors 21, 3984. doi:10.3390/s21123984
Majumder, S., and Deen, M. J. (2019). Smartphone sensors for health monitoring and diagnosis. Sensors 19, 2164. doi:10.3390/s19092164
Martinez-Santos, A., Preece, S., and Nester, C. J. (2019). Evaluation of orthotic insoles for people with diabetes who are at-risk of first ulceration. J. Foot Ankle Res. 12, 35. doi:10.1186/s13047-019-0344-z
Martín-Vaquero, J., Hernández Encinas, A., Queiruga-Dios, A., José Bullón, J., Martínez-Nova, A., Torreblanca González, J., et al. (2019). Review on wearables to monitor foot temperature in diabetic patients. Sensors 19, 776. doi:10.3390/s19040776
Mazzoli, A. (2013). Selective laser sintering in biomedical engineering. Med. Biol. Eng. Comput. 51, 245–256. doi:10.1007/s11517-012-1001-x
Ming, A., Walter, I., Alhajjar, A., Leuckert, M., and Mertens, P. R. (2019). Study protocol for a randomized controlled trial to test for preventive effects of diabetic foot ulceration by telemedicine that includes sensor-equipped insoles combined with photo documentation. Trials 20, 521. doi:10.1186/s13063-019-3623-x
Moayedi, M., Arshi, A. R., Salehi, M., Akrami, M., and Naemi, R. (2021). Associations between changes in loading pattern, deformity, and internal stresses at the foot with hammer toe during walking; a finite element approach. Comput. Biol. Med. 135, 104598. doi:10.1016/j.compbiomed.2021.104598
Mondal, S., Katzschmann, R., and Clemens, F. (2023). Magnetorheological behavior of thermoplastic elastomeric honeycomb structures fabricated by additive manufacturing. Compos. Part B Eng. 252, 110498. doi:10.1016/j.compositesb.2023.110498
Najafi, B., Ron, E., Enriquez, A., Marin, I., Razjouyan, J., and Armstrong, D. G. (2017). Smarter sole survival: will neuropathic patients at high risk for ulceration use a smart insole-based foot protection system? J. Diabetes Sci. Technol. 11, 702–713. doi:10.1177/1932296816689105
Newman, S. T., Zhu, Z., Dhokia, V., and Shokrani, A. (2015). Process planning for additive and subtractive manufacturing technologies. CIRP Ann. 64, 467–470. doi:10.1016/j.cirp.2015.04.109
Ning, K., Yick, K.-L., Yu, A., and Yip, J. (2022). Effects of textile-fabricated insole on foot skin temperature and humidity for enhancing footwear thermal comfort. Appl. Ergon. 104, 103803. doi:10.1016/j.apergo.2022.103803
Niu, J., Liu, J., Zheng, Y., Ran, L., and Chang, Z. (2020). Are arch-conforming insoles a good fit for diabetic foot? Insole customized design by using finite element analysis. Hum. Factors Ergonomics Manuf. Serv. Industries 30, 303–310. doi:10.1002/hfm.20841
Nouman, M., Chong, D. Y. R., Srewaradachpisal, S., and Chatpun, S. (2023). The effect of customized insole pads on plantar pressure distribution in a diabetic foot with neuropathy: material and design study using finite element analysis approach. Appl. Sci. 13, 399. doi:10.3390/app13010399
Nouman, M., Dissaneewate, T., Chong, D. Y. R., and Chatpun, S. (2021). Effects of custom-made insole materials on frictional stress and contact pressure in diabetic foot with neuropathy: results from a finite element analysis. Appl. Sci. 11, 3412. doi:10.3390/app11083412
Nouman, M., Dissaneewate, T., Leelasamran, W., and Chatpun, S. (2019). The insole materials influence the plantar pressure distributions in diabetic foot with neuropathy during different walking activities. Gait Posture 74, 154–161. doi:10.1016/j.gaitpost.2019.08.023
Panda, B., Leite, M., Biswal, B. B., Niu, X., and Garg, A. (2018). Experimental and numerical modelling of mechanical properties of 3D printed honeycomb structures. Measurement 116, 495–506. doi:10.1016/j.measurement.2017.11.037
Paris, H., Mokhtarian, H., Coatanéa, E., Museau, M., and Ituarte, I. F. (2016). Comparative environmental impacts of additive and subtractive manufacturing technologies. CIRP Ann. 65, 29–32. doi:10.1016/j.cirp.2016.04.036
Park, S.-B., Lee, K.-D., Kim, D.-W., Yoo, J.-H., and Kim, K.-H. (2014). An analysis of functional insole on foot pressure distribution of shape memory material combinations. J. Foot Ankle Res. 7, A120. doi:10.1186/1757-1146-7-S1-A120
Patwa, R., Saha, N., and Sáha, P. (2020). Magnetic hydrogel based shoe insoles for prevention of diabetic foot. J. Magnetism Magnetic Mater. 514, 167153. doi:10.1016/j.jmmm.2020.167153
Peker, A., Aydin, L., Kucuk, S., Ozkoc, G., Cetinarslan, B., Canturk, Z., et al. (2020). Additive manufacturing and biomechanical validation of a patient-specific diabetic insole. Polym. Adv. Technol. 31, 988–996. doi:10.1002/pat.4832
Peng, Y., Wong, D. W.-C., Chen, T. L.-W., Wang, Y., Zhang, G., Yan, F., et al. (2021). Influence of arch support heights on the internal foot mechanics of flatfoot during walking: a muscle-driven finite element analysis. Comput. Biol. Med. 132, 104355. doi:10.1016/j.compbiomed.2021.104355
Perren, S., Formosa, C., Camilleri, L., Chockalingam, N., and Gatt, A. (2021). The thermo-pressure concept: a new model in diabetic foot risk stratification. Appl. Sci. 11, 7473. doi:10.3390/app11167473
Rachůnková, I., Spielauer, A., Staněk, S., and Weinmüller, E. B. (2013). The structure of a set of positive solutions to Dirichlet BVPs with time and space singularities. Georgian Math. J. 20, 95–127. doi:10.1515/gmj-2013-0002
Rajan, T. P., Souza, L. D., Ramakrishnan, G., and Zakriya, G. M. (2016). Comfort properties of functional warp-knitted polyester spacer fabrics for shoe insole applications. J. Industrial Text. 45, 1239–1251. doi:10.1177/1528083714557056
Rankouhi, B., Javadpour, S., Delfanian, F., and Letcher, T. (2016). Failure analysis and mechanical characterization of 3D printed ABS with respect to layer thickness and orientation. J. Fail. Analysis Prev. 16, 467–481. doi:10.1007/s11668-016-0113-2
Salles, A. S., and Gyi, D. E. (2012). The specification of personalised insoles using additive manufacturing. Work 41, 1771–1774. doi:10.3233/WOR-2012-0383-1771
Salvo, P., Calisi, N., Melai, B., Cortigiani, B., Mannini, M., Caneschi, A., et al. (2017a). Temperature and pH sensors based on graphenic materials. Biosens. Bioelectron. 91, 870–877. doi:10.1016/j.bios.2017.01.062
Salvo, P., Calisi, N., Melai, B., Dini, V., Paoletti, C., Lomonaco, T., et al. (2017b). Temperature- and pH-sensitive wearable materials for monitoring foot ulcers. Int. J. Nanomedicine 12, 949–954. doi:10.2147/IJN.S121726
Schaper, N. C., van Netten, J. J., Apelqvist, J., Bus, S. A., Hinchliffe, R. J., Lipsky, B. A., et al. (2020). Practical guidelines on the prevention and management of diabetic foot disease (IWGDF 2019 update). Diabetes/Metabolism Res. Rev. 36, e3266. doi:10.1002/dmrr.3266
Senneville, E. M., Lipsky, B. A., van Asten, S. A. V., and Peters, E. J. (2020). Diagnosing diabetic foot osteomyelitis. Diabetes/Metabolism Res. Rev. 36, e3250. doi:10.1002/dmrr.3250
Shalamberidze, M., Sokhadze, Z., and Tatvidze, M. (2021). The design of individual orthopedic insoles for the patients with diabetic foot using integral curves to describe the plantar over-pressure areas. Comput. Math. Methods Med. 2021, 1–11. doi:10.1155/2021/9061241
Sharma, S., Singh, J., Kumar, H., Sharma, A., Aggarwal, V., Gill, A. S., et al. (2020). Utilization of rapid prototyping technology for the fabrication of an orthopedic shoe inserts for foot pain reprieve using thermo-softening viscoelastic polymers: a novel experimental approach. Meas. Control 53, 519–530. doi:10.1177/0020294019887194
Shi, C., Lu, N., Qin, Y., Liu, M., Li, H., and Li, H. (2021). Study on mechanical properties and permeability of elliptical porous scaffold based on the SLM manufactured medical Ti6Al4V. PLoS One 16, e0247764. doi:10.1371/journal.pone.0247764
Shi, Q. Q., Li, P. L., Yick, K.-L., Li, N.-W., and Jiao, J. (2022). Effects of contoured insoles with different materials on plantar pressure offloading in diabetic elderly during gait. Sci. Rep. 12, 15395. doi:10.1038/s41598-022-19814-0
Shim, D. W., Sung, S.-Y., Chung, W.-Y., Kang, K.-Y., Park, S.-J., Lee, J. W., et al. (2021). Superior pedal function recovery of newly designed three spike insole over total contact insole in refractory plantar fasciitis: a randomized, double-blinded, non-inferiority study. PLoS One 16, e0255064. doi:10.1371/journal.pone.0255064
Singh, G., Gupta, S., and Chanda, A. (2021). Biomechanical modelling of diabetic foot ulcers: a computational study. J. Biomechanics 127, 110699. doi:10.1016/j.jbiomech.2021.110699
Song, Y., Shao, E., Bíró, I., Baker, J. S., and Gu, Y. (2022). Finite element modelling for footwear design and evaluation: a systematic scoping review. Heliyon 8, e10940. doi:10.1016/j.heliyon.2022.e10940
Srivastava, V., and Gaur, H. (2020). Revolutionary development in orthopedic insole by additive manufacturing. J. Crit. Rev. 7, 1943–1947.
Subramaniam, S., Majumder, S., Faisal, A. I., and Deen, M. J. (2022). Insole-based systems for health monitoring: current solutions and research challenges. Sensors 22, 438. doi:10.3390/s22020438
Tang, L., Wang, L., Bao, W., Zhu, S., Li, D., Zhao, N., et al. (2019). Functional gradient structural design of customized diabetic insoles. J. Mech. Behav. Biomed. Mater. 94, 279–287. doi:10.1016/j.jmbbm.2019.03.003
Telfer, S., Erdemir, A., Woodburn, J., and Cavanagh, P. R. (2014). What has finite element analysis taught us about diabetic foot disease and its management? A systematic review. PLoS One 9, e109994. doi:10.1371/journal.pone.0109994
van Netten, J. J., Bus, S. A., Apelqvist, J., Lipsky, B. A., Hinchliffe, R. J., Game, F., et al. (2020). Definitions and criteria for diabetic foot disease. Diabetes/Metabolism Res. Rev. 36, e3268. doi:10.1002/dmrr.3268
Vyavahare, S., Teraiya, S., Panghal, D., and Kumar, S. (2019). Fused deposition modelling: a review. Rapid Prototyp. J. 26, 176–201. doi:10.1108/RPJ-04-2019-0106
Wang, L., Jones, D., Jones, A., Chapman, G. J., Siddle, H. J., Russell, D., et al. (2022). A portable insole system to simultaneously measure plantar pressure and shear stress. IEEE Sensors J. 22, 9104–9113. doi:10.1109/JSEN.2022.3162713
Winarso, R., Anggoro, P. W., Ismail, R., Jamari, J., and Bayuseno, A. P. (2022). Application of fused deposition modeling (FDM) on bone scaffold manufacturing process: a review. Heliyon 8, e11701. doi:10.1016/j.heliyon.2022.e11701
Wong, K. V., and Hernandez, A. (2012). A review of additive manufacturing. ISRN Mech. Eng. 2012, 1–10. doi:10.5402/2012/208760
Yamamoto, T., Hoshino, Y., Kanzaki, N., Nukuto, K., Yamashita, T., Ibaraki, K., et al. (2020). Plantar pressure sensors indicate women to have a significantly higher peak pressure on the hallux, toes, forefoot, and medial of the foot compared to men. J. Foot Ankle Res. 13, 40. doi:10.1186/s13047-020-00410-2
Yavuz, M., Ersen, A., Monga, A., Lavery, L. A., Garrett, A. G., Salem, Y., et al. (2020). Temperature- and pressure-regulating insoles for prevention of diabetic foot ulcers. J. Foot Ankle Surg. 59, 685–688. doi:10.1053/j.jfas.2019.05.009
Yu, Q., Qiao, G., Wang, M., Yu, L., Sun, Y., Shi, H., et al. (2022). Stem cell-based therapy for diabetic foot ulcers. Front. Cell Dev. Biol. 10, 812262. doi:10.3389/fcell.2022.812262
Yufeng, Z., and Yanwu, L. I. U. (2021). Multi-slice spiral CT angiography in the diagnosis of diabetic foot lower extremity artery disease. Imaging Sci. Photochem. 39, 611. doi:10.7517/issn.1674-0475.201203
Zeng, W., Mukherjee, S., Caudillo, A., Forman, J., and Panzer, M. B. (2021). Evaluation and validation of thorax model responses: a hierarchical approach to achieve high biofidelity for thoracic musculoskeletal system. Front. Bioeng. Biotechnol. 9, 712656. doi:10.3389/fbioe.2021.712656
Zhang, P., Lu, J., Jing, Y., Tang, S., Zhu, D., and Bi, Y. (2017). Global epidemiology of diabetic foot ulceration: a systematic review and meta-analysis. Ann. Med. 49, 106–116. doi:10.1080/07853890.2016.1231932
Keywords: diabetic foot, customized insoles, finite element analysis, pressure offloading, additive manufacturing, smart insoles
Citation: Ren Y, Wang H, Song X, Wu Y, Lyu Y and Zeng W (2024) Advancements in diabetic foot insoles: a comprehensive review of design, manufacturing, and performance evaluation. Front. Bioeng. Biotechnol. 12:1394758. doi: 10.3389/fbioe.2024.1394758
Received: 02 March 2024; Accepted: 24 May 2024;
Published: 15 July 2024.
Edited by:
Rezaul Begg, Victoria University, AustraliaReviewed by:
Rajshree Hillstrom, Biomed Consulting, Inc., United StatesGarrett Brian McGuinness, Dublin City University, Ireland
Copyright © 2024 Ren, Wang, Song, Wu, Lyu and Zeng. This is an open-access article distributed under the terms of the Creative Commons Attribution License (CC BY). The use, distribution or reproduction in other forums is permitted, provided the original author(s) and the copyright owner(s) are credited and that the original publication in this journal is cited, in accordance with accepted academic practice. No use, distribution or reproduction is permitted which does not comply with these terms.
*Correspondence: Yongtao Lyu, eW9uZ3Rhb2x1QGRsdXQuZWR1LmNu; Wei Zeng, emVuZy53b3JrQGdtYWlsLmNvbQ==, d3plbmcwM0BueWl0LmVkdQ==
†These authors have contributed equally to this work