- 1Oral Diagnosis and Medicine, Faculty of Dental Medicine, Hokkaido University, Sapporo, Japan
- 2Department of Biomaterials and Bioengineering, Faculty of Dental Medicine, Hokkaido University, Sapporo, Japan
- 3BIOMAT, Department of Oral Sciences, KU Leuven, Leuven, Belgium
- 4Department of Dental and Biomedical Materials Science, Graduate School of Biomedical Sciences, Nagasaki University, Nagasaki, Japan
- 5Health and Medical Research Institute, National Institute of Advanced Industrial Science and Technology, Takamatsu, Japan
- 6School of Clinical Psychology, Kyushu University of Medical Science, Miyazaki, Japan
- 7Section for Dental Innovation, Faculty of Dental Medicine, Hokkaido University, Sapporo, Japan
- 8Department of Biomedical Engineering, Iwate Medical University, Shiwa, Japan
- 9Department of Oral Biochemistry and Molecular Biology, Graduate School of Dental Medicine, Hokkaido University, Sapporo, Japan
Various implant surface treatment methods have been developed to achieve good osseointegration in implant treatment. However, some cases remain impossible to treat with implants because osseointegration is not obtained after implantation, and the implants fail. Thus, this study focused on phosphorylated pullulan because of its adhesiveness to titanium (Ti) and bone, high biocompatibility, and early replacement with bone. In this study, the response of bone-related cells to phosphorylated pullulan was evaluated to develop a new surface treatment method. Saos-2 (human osteosarcoma-derived osteoblast-like cells), MC3T3-E1 (mouse calvaria-derived osteoblast-like cells), and RAW264.7 (mouse macrophage-like cells) were used. In evaluating cellular responses, phosphorylated pullulan was added to the culture medium, and cell proliferation and calcification induction tests were performed. The proliferation and calcification of cells on the surface of Ti disks coated with phosphorylated pullulan were also evaluated. In addition, bone morphogenetic protein-2 (BMP-2), an osteogenic factor, was used to evaluate the role of phosphorylated pullulan as a drug carrier in inducing calcification on Ti disks. Phosphorylated pullulan tended to promote the proliferation of osteoblast-like cells and the formation of calcification on Ti disks coated with phosphorylated pullulan. Ti disks coated with phosphorylated pullulan loaded with BMP-2 enhanced calcification. Phosphorylated pullulan inhibited osteoclast-like cell formation. These results are due to the properties of phosphorylated pullulan, such as adhesiveness to titanium and drug-loading function. Therefore, phosphorylated pullulan effectively promotes bone regeneration when coated on titanium implants and is useful for developing a new surface treatment method.
1 Introduction
Since the report of Brånemark regarding osseointegration between bone and titanium (Ti) (Block, 2018), various improvements have been made to achieve good osseointegration (Bosshardt et al., 2017). Surface treatment of implants is one of them. Surface treatments include anodizing, acid etching, sandblasting, TiOblasting (Collaert et al., 2008), titanium plasma-spraying (Becker et al., 2000), hydroxyapatite coating (Smeets et al., 2016), and others. These treatment processes have been clinically applied as products. However, there are still many cases wherein sufficient osseointegration cannot be obtained, and implants drop out prematurely. In addition, hydroxyapatite coating results in loosening of the coating, which causes peri-implantitis, and bone resorption that occur after implantation (Albrektsson, 1998). Furthermore, risk factors in implant treatment include diabetes, radiation therapy, smoking, periodontal disease, and bruxism, which can make treatment more difficult (Albrektsson, 1998; Kullar and Miller, 2019). Therefore, improvement of implant surface properties, which achieve sufficient osseointegration acquisition, an affinity for titanium and bone, high biocompatibility, and early bone replacement properties, requires new coating materials (Choi et al., 2013; Abraham, 2014; Smeets et al., 2016; Chouirfa et al., 2019; Wegener et al., 2020).
Pullulan is polysaccharide consisting of α-(1,6) linked maltotriose composed of three molecules of glucose bonded α-(1,4). It is produced from sucrose or starch by Aureobasidium pullulans. The substance has excellent biosafety (Aydogdu et al., 2016) and adhesive properties and is used in various fields, such as food and pharmaceuticals. Furthermore, phosphorylated pullulan has excellent biocompatibility, and it can promote bone regeneration and serve as a drug carrier (Yonehiro et al., 2013). Phosphorylated pullulan also promoted good osseointegration when implants were coated with phosphorylated pullulan and implanted in the parietal bone of a miniature pig (Cardoso et al., 2017). Bone formation was similarly enhanced when phosphorylated pullulan-coated Ti disks were implanted in rabbit tibia (Cardoso et al., 2014). However, few reports have been found on the effects of phosphorylated pullulan on bone-related cells in cell experiments.
In this study, phosphorylated pullulan was added to the culture medium or adsorbed to Ti disks, and then bone-related cells were cultured by using them for evaluation of cell proliferation and differentiation induction. Differentiation induction was also evaluated to investigate the drug carrier ability of phosphorylated pullulan when phosphorylated pullulan was loaded with a bone formation factor.
2 Materials and methods
2.1 Preparation of the phosphorylated pullulan
Pullulan (average molecular weight of 322,916; Hayashibara, Inc., Okayama, Japan) was dissolved in sodium hydroxide aqueous solution and stirred at 20°C overnight; after cooling to 0°C, phosphoryl chloride was added, and the solution was stirred at 0°C for 6 h. The solution was dialyzed to remove sodium phosphate and sodium chloride by-products. After concentration, the solution was lyophilized to produce phosphorylated pullulan (average molecular weight of 617,515; Figure 1).
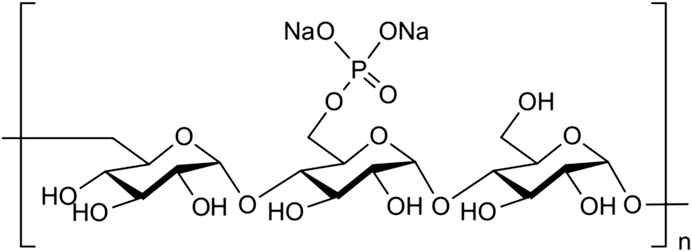
Figure 1. Structure of phosphorylated pullulan. Phosphate groups were attached to the carbon at position six of the glycosidic-bonded glucan at α1-4 and α1-6 to form pullulan.
2.2 Phosphorylated pullulan treatment on Ti disks (coating of phosphorylated pullulan on Ti disks)
2.2.1 Observation of Ti disks
Ti disks (JIS class 2 pure titanium, GC Corporation, Tokyo, Japan) were randomly ground with a #400 waterproof abrasive paper in 5-cm squares for 4 min. The same method was used for the following grinding. The ground Ti disks were observed using a scanning electron microscope (SEM, S4800, Hitachi High-Tech Corporation, Tokyo, Japan) and a shape-measuring laser microscope (VK-X200, Keyence Corporation, Osaka, Japan).
2.2.2 Confirmation of the phosphorylated pullulan adhesion on Ti disks
The Ti disks were ground using the same method. The ground Ti disks were immersed in 99.5% acetone for 1 h and then in 99% ethanol for 30 min. Immersion was repeated three times. The obtained Ti disks were immersed in 1% phosphorylated pullulan solution using MilliQ as a solvent for 24 h. Then, the disks were ultrasonically washed in deionized water for 10 min, and this process was repeated three times. The controls were immersed in MilliQ for 24 h and washed in the same manner.
In confirming the adhesion of phosphorylated pullulan to Ti disks, the disks were analyzed by X-ray photoelectron spectroscopy (XPS, Axis-Ultra, Shimadzu Corporation, Kyoto, Japan).
2.2.3 Observation of cells on Ti disks coated with phosphorylated pullulan
The Ti disks were soaked in 99.5% acetone, 99% ethanol, and 0.5% hydrochloric acid and sonicated for 10 min. Afterward, the disks were sonicated two times with deionized water for 10 min. The disks were sterilized in an autoclave. After immersion in 1% phosphorylated pullulan solution using MilliQ as a solvent for 24 h, the disks were washed three times with deionized water. The control disks were immersed in MilliQ instead of 1% phosphorylated pullulan solution for 24 h and washed in the same manner. Ti disks were placed on 24-well plates and seeded with Saos-2 at a concentration of 7.5 × 104 cells/well. The medium was Dulbecco’s Modified Eagle Medium (DMEM) with high glucose (Sigma-Aldrich Co. LLC., St. Louis, United States of America) containing 10% FBS and 1% penicillin–streptomycin amphotericin B. The cells were incubated for 30 min at 37°C under 5% CO2.
After 30 min, the disks were washed and immersed in 1% glutaraldehyde to fix the cells. The cells were then dehydrated using an alcohol series (50%, 60%, 70%, 80%, 90%, 95%, 99.5%, and 100%), and critical point drying was performed. The samples were pretreated with Pt–Pd conductors using an ion sputtering system (E1030, Hitachi High-Tech Corporation, Tokyo, Japan). The samples were observed using a SEM at a pressurized voltage of 10 kV.
2.3 Cell proliferation test
2.3.1 Cell culture using a culture medium, including phosphorylated pullulan
MEM-α (FUJIFILM Wako Pure Chemical Corporation, Osaka, Japan) containing 10% FBS and 1% penicillin–streptomycin–amphotericin B was prepared as the culture medium. MC3T3-E1 cells were seeded at a concentration of 1 × 103 cells/well in 96-well plates and cultured for 1 day at 37°C under 5% CO2. The culture medium was aspirated and then replaced with a new medium, including phosphorylated pullulan at concentrations of 0% (control), 0.01%, 0.1%, 0.5%, 1%, and 3%, and incubated for 2 days. Using the Cell Counting Kit-8 (WST-8, Dojindo Laboratories, Kumamoto, Japan), the number of cells was evaluated indirectly. Absorbance was measured using a microscope reader (Spectra Max Paradigm, Thermo Fisher Scientific, Waltham, United States of America) at 450 nm. Dunnett’s t-test was used for statistical processing between control and other conditions, and the statistically significant difference was set to p < 0.05.
2.3.2 Cell culture using Ti disks coated with phosphorylated pullulan
The ground Ti disks were immersed in 99.5% acetone for 1 h and then in 99% ethanol for 30 min. These processes were repeated three times. Then, the disks were ultrasonically cleaned three times in deionized water for 10 min and immersed in 1% phosphorylated pullulan solution using MilliQ as a solvent for 24 h. The disks were sonicated in deionized water for 10 min and repeated three times. The control disks were immersed in MilliQ for 24 h and washed in the same manner. The disks were then sterilized in an autoclave. Phosphorylated pullulan-coated Ti disks and control Ti disks were placed on 24-well plates and seeded with MC3T3-E1 at a concentration of 1 × 104 cells/well. The cells were cultured for 1 or 2 days. The culture medium was MEM-α containing 10% FBS and 1% penicillin–streptomycin–amphotericin B. The cells were cultured at 37°C under 5% CO2. The Ti disks were washed once with PBS, avoiding the stream from hitting the Ti disks directly. The Ti disks were transferred to another plate filled with a fresh medium, and 50 µL of WST8 solution was added to each well. The cells were then incubated. The number of cells was evaluated by measuring the absorbance at 450 nm using a microplate reader. Student’s t-test was used for statistical processing between the two conditions, and the statistically significant difference was set to p < 0.05.
2.4 Effect of phosphorylated pullulan on the induction of calcification
2.4.1 Cell culture using a culture medium, including phosphorylated pullulan
Saos-2 was seeded into 24-well plates at a concentration of 2.3 × 104 cells/well and cultured for 5 days under the same conditions as described in Section 2.2.3. Culturing was continued for another week under three conditions using three different media, namely, calcification induction medium, calcification induction medium mixed with phosphorylated pullulan at a concentration of 1%, and DMEM medium as a control. The calcification induction medium was DMEM with supplements. The final concentrations of the supplements were 10 nM dexamethasone, 50 μg/mL ascorbic acid, and 5 mM β-glycerophosphate. The culture was performed at 37°C under 5% CO2 in the air phase, and the medium was changed after 3 or 4 days. After incubation, each well was washed with PBS. Cells were fixed with methanol and washed again. Then, the cells were stained with an alizarin red solution and washed several times with deionized water.
2.4.2 Cell culture using Ti disks coated with phosphorylated pullulan
The ground Ti disks were soaked in 99.5% acetone, 99% ethanol, and 0.5% hydrochloric acid and sonicated for 10 min. Afterward, the disks were sonicated two times with deionized water for 10 min. The disks were sterilized in an autoclave. After immersion in 1% phosphorylated pullulan solution using MilliQ as a solvent for 24 h. The control Ti disks were immersed in MilliQ for 24 h instead of 1% phosphorylated pullulan. Phosphorylated pullulan-coated Ti disks and control Ti disks were placed on 24-well plates and seeded with Saos-2 at 6.48 × 104 cells/well for 3 days under the same conditions described in Section 2.2.3. The Ti disks were transferred to new 24-well plates filled with a calcification induction medium and incubated for 2 weeks. The Ti disks were washed; the cells were fixed, and calcification formation was stained using the same methods as described in Section 2.4.1.
2.5 BMP-2 loading effect using phosphorylated pullulan on Ti disks
The ground Ti disks were washed and sterilized using the same process described in Section 2.4.2. The Ti disks were immersed in 0.2 μg/mL BMP-2 (Proteintech Group, Inc., Rosemont, United States of America) + 1% phosphorylated pullulan solution or 0.2 μg/mL BMP-2 solution for 1 day. The disks were washed three times, transferred to a new plate, seeded with Saos-2 at a concentration of 1 × 105 cells/well, and incubated for 3 days under the same conditions as described in Section 2.2.3. The disks were transferred to a new plate and replaced with a calcification induction medium, and the concentration of β-glycerophosphate was adjusted to 10 mM. The concentrations of the other supplements such as dexamethasone and ascorbic acid were not changed from Section 2.4. The cells were cultured for 4 days for calcification induction in the same way as described in Section 2.4.1. The cells were washed, fixed, and stained using the same methods as described in Section 2.4.1.
2.6 Effect of phosphorylated pullulan on osteoclast-like cell formation
RAW264.7 were seeded at a density of 5,000 cells/cm2 in 24-well plates and grown for several days. MEM-α containing 10% FBS supplemented with 1% penicillin–streptomycin–amphotericin B was used as the medium. The cells were cultured at 37°C under 5% CO2 atmosphere. Next, 50 ng/mL of receptor activator of nuclear factor-kappa B ligand (RANKL, sRANKL, Oriental Yeast, Tokyo, Japan) and phosphorylated pullulan were added at 0% (control), 0.1%, 1%, and 3%, and the cells were incubated at 37°C for 6 days under 5% CO2 atmosphere. The medium was changed every 2 days. After culture, cells were washed with PBS, fixed with 4% paraformaldehyde (FUJIFILM Wako Pure Chemical Corporation, Osaka, Japan), washed with distilled water, and stained for osteoclast-like cells using the TRAP staining kit (Cosmo Bio Company, Inc., Tokyo, Japan). Osteoclast-like cells were defined as TRAP-positive cells with three or more nuclei and were observed and measured. Osteoclast-like cells were randomly counted in 20 fields of view. Dunnett’s t-test was used for statistical processing between control and other conditions, and the statistically significant difference was set to p < 0.05.
3 Result
3.1 Phosphorylated pullulan treatment of Ti disks
3.1.1 SEM
The surfaces of Ti disks ground in random directions with #400 waterproof abrasive paper of 5-cm square were observed by SEM, and the entire surface was uniformly ground without any large scratches (Figure 2).
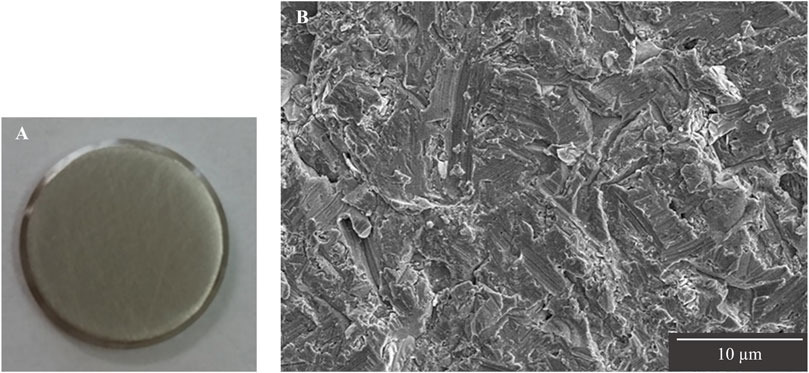
Figure 2. Observation of Ti disk surfaces after grinding. (A) Image of Ti disk ground with #400 waterproof abrasive paper. (B) SEM image of Ti disk surface ground up to #400.
3.1.2 Shape measurement laser microscope
The surface roughness of the disk ground with #400 waterproof paper was examined using a laser microscope. The Ra of the disk ground with #400 waterproof paper was 0.47 ± 0.04 µm. The 3D images showed that the top surface of the disk ground with #400 waterproof paper had a height difference of 7.4 µm (Figure 3).
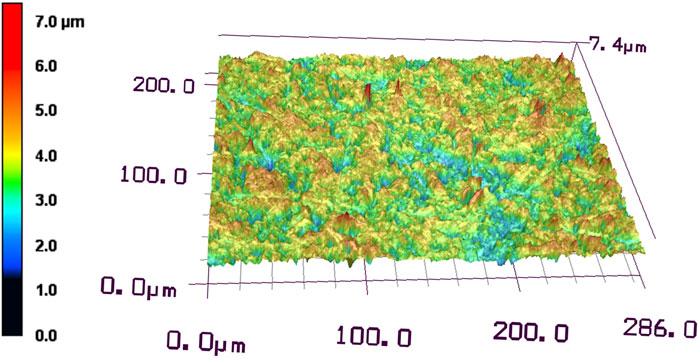
Figure 3. Laser microscopic observation of Ti disk surface after grinding with #400 waterproof paper.
3.1.3 XPS
XPS analysis of the Ti disk surface treated with phosphorylated pullulan showed a peak at approximately 134 eV. This signal was derived from phosphorus (P2p), and the presence of phosphate groups derived from phosphorylated pullulan on the Ti disk surface was confirmed. The composition of phosphorus on the Ti disk surface treated with phosphorylated pullulan was 0.5–0.9 atomic% of the total percentage of elements detected. This result indicates that the phosphorylated pullulan adsorbed to the Ti disk surface (Figure 4).
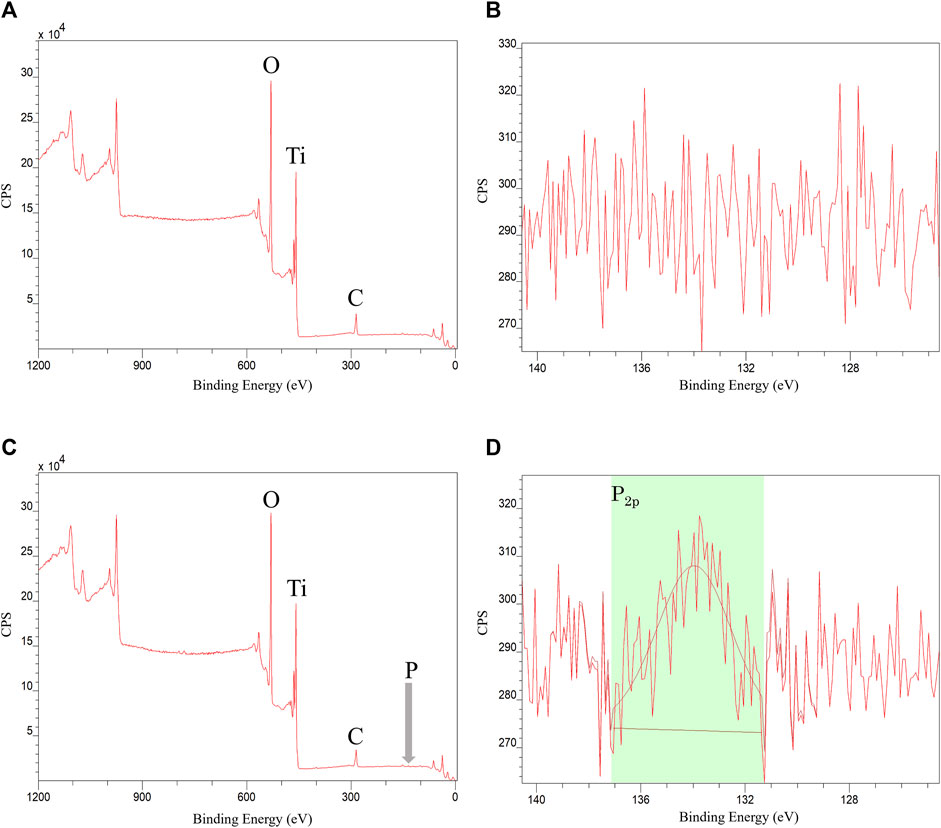
Figure 4. XPS measurement of Ti disk. (A,B) Control Ti disk. (C,D) Phosphorylated pullulan-coated Ti disk.
3.2 Observation of cells on the phosphorylated pullulan-treated Ti disk surface
Saos-2 cells cultured on the Ti disks for 30 min were observed to adhere to the surface of the Ti disks by SEM. Lamellipodia and filopodia were observed on the cell on each Ti disks, which are involved in cell body migration and probing the surrounding environment (Mattila and Lappalainen, 2008). Cells cultured on phosphorylated pullulan-treated Ti disks seem to have more filopodia than control Ti disks (Figure 5).
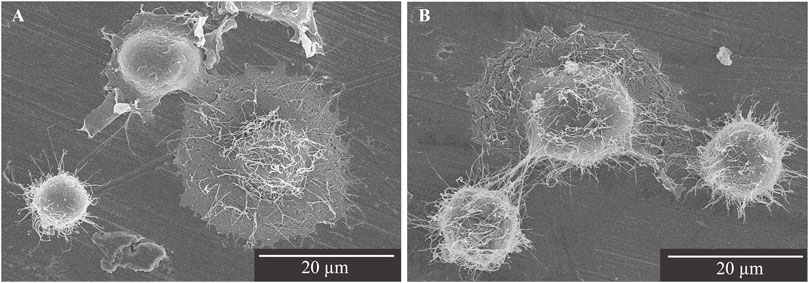
Figure 5. SEM image of Saos-2 on the Ti disks at ×2,000 magnification. Scale bar = 20 μm. (A) Control Ti disk. (B) Phosphorylated pullulan-coated Ti disk.
3.3 Cell proliferation test
3.3.1 Cell culture using a culture medium, including phosphorylated pullulan
The direct addition of phosphorylated pullulan to the culture medium at concentrations of 1% and 3% significantly inhibited the cell proliferation of MC3T3-E1 compared with the control (Figure 6).
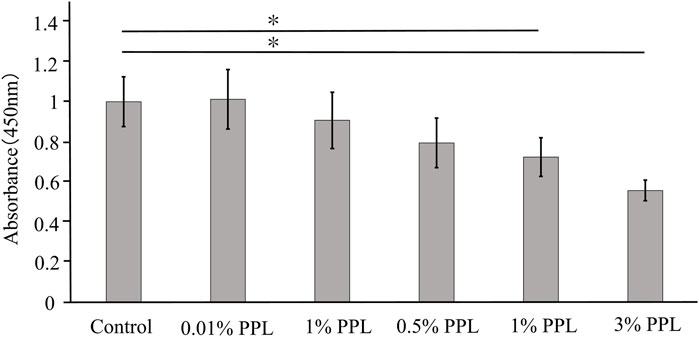
Figure 6. Cell proliferation test using medium supplemented with phosphorylated pullulan. The cells were MC3T3-E1. Control in the graph showed the medium without phosphorylated pullulan. PPL in the graph showed the medium supplemented with phosphorylated pullulan. Significance difference test: *p < 0.05.
3.3.2 Cell culture using phosphorylated pullulan-coated Ti disks
On day 1, the number of MC3T3-E1 cells did not change, but on day 2, the number of MC3T3-E1 cells tended to increase on the phosphorylated pullulan-coated Ti disks compared with the uncoated Ti disks, although the difference was not statistically significant (Figure 7).
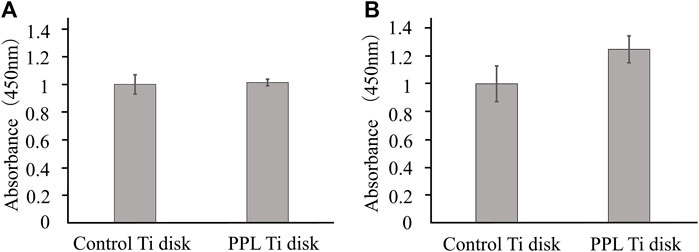
Figure 7. Cell proliferation test on Ti disk surface. The cells were MC3T3-E1. (A) Day 1. (B) Day 2.
3.4 Effect of osteoblast-like cells on the induction of calcification
3.4.1 Cell culture using a culture medium, including phosphorylated pullulan
Saos-2 produced calcification products in the calcification induction medium group, whereas no calcification occurred in the DMEM and calcification-inducing medium groups containing 1% phosphorylated pullulan (Figure 8).
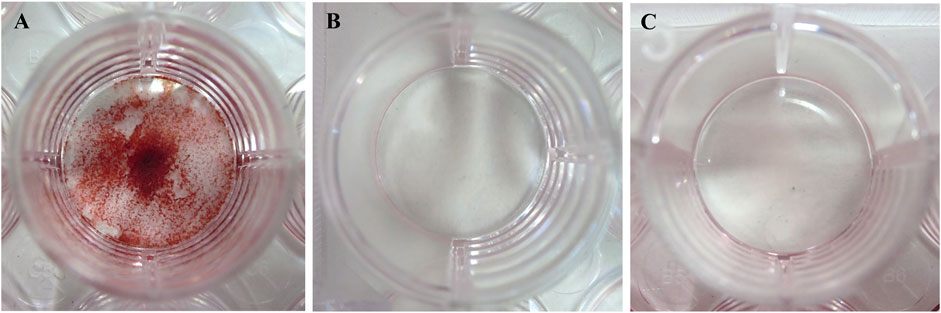
Figure 8. Induction of calcification. The cells were Saos-2. (A) Calcification induction medium. (B) Calcification induction medium supplemented with 1% phosphorylated pullulan. (C) DMEM medium.
3.4.2 Cell culture using phosphorylated pullulan-coated Ti disks
Compared with the uncoated Ti disks, dense calcification occurred on the surface of the phosphorylated pullulan-coated Ti disks (Figure 9).
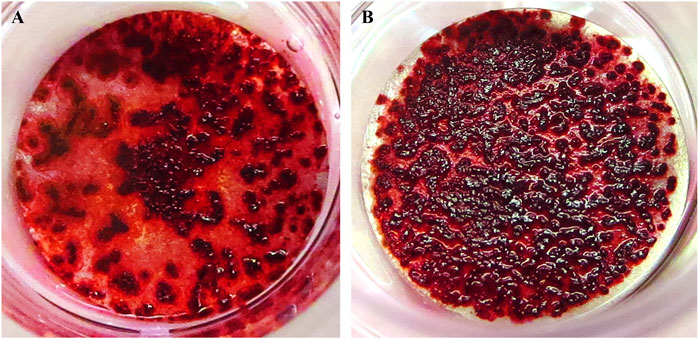
Figure 9. Calcification induction test on the Ti disk surface. The cells were Saos-2. (A) Uncoated Ti disk (control). (B) Ti disk coated with 1% phosphorylated pullulan.
3.5 Effects of phosphorylated pullulan carrying osteogenic factors on Ti disks
Compared with Ti disks immersed in 0.2 μg/mL BMP-2 solution, the surface of Ti disks immersed in 1% phosphorylated pullulan solution with 0.2 μg/mL BMP-2 showed more calcification and extensive deposition (Figure 10).
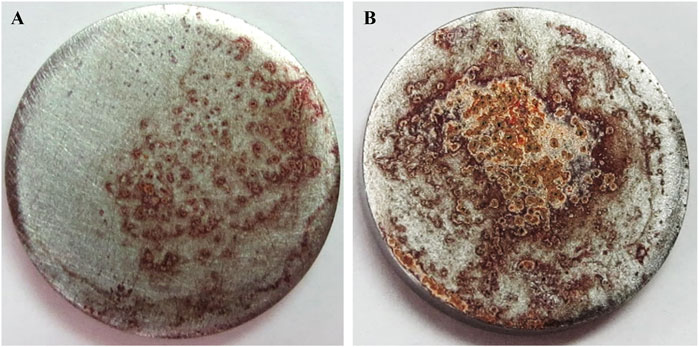
Figure 10. Effects of phosphorylated pullulan carrying osteogenic factor on the Ti disk. The cells were Saos-2. (A) Ti disk immersed in 0.2 μg/mL BMP-2 solution. (B) Ti disk immersed in 0.2 μg/mL BMP-2 + 1% phosphorylated pullulan solution.
3.6 Effects on osteoclast-like cell formation
The control group (without phosphorylated pullulan) showed a large number of TRAP-positive cells with three or more nuclei, which has a large round morphology. As the concentration of phosphorylated pullulan in the culture medium increased, the number of nuclei decreased, and the multinucleated giant cells showed a thin and small morphology.
The number of osteoclast-like cells was significantly decreased in a concentration-dependent manner in the medium containing 0.1%, 1%, and 3% phosphorylated pullulan compared with the control group (Figure 11).
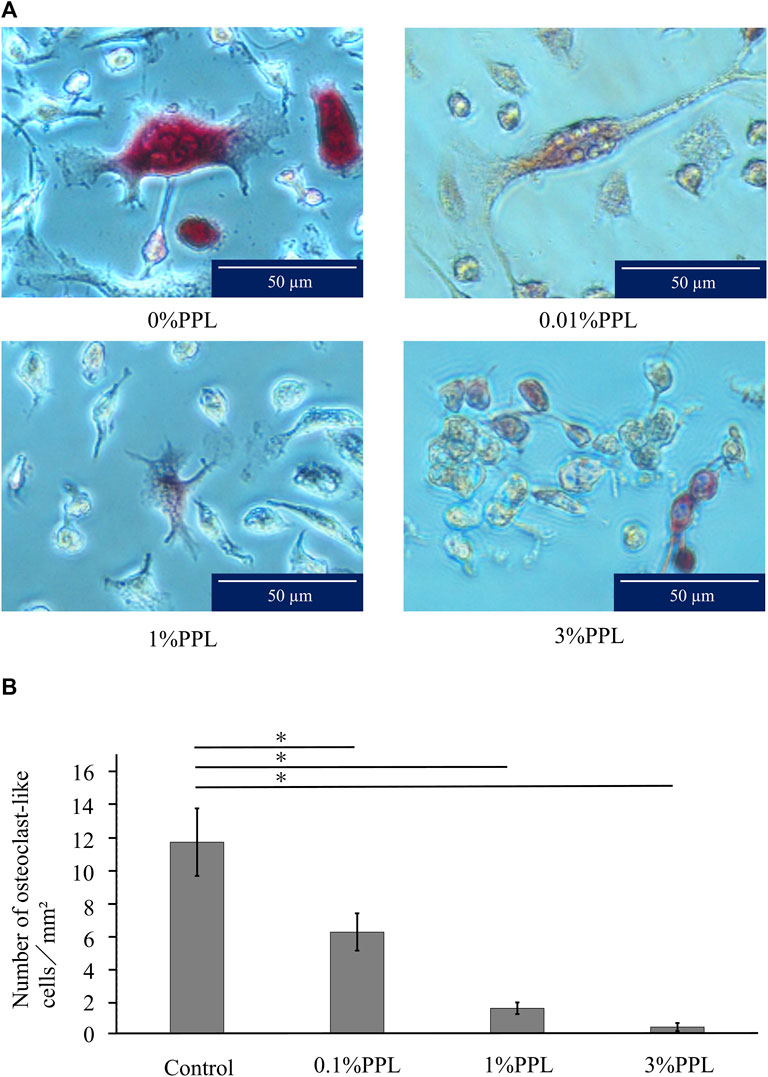
Figure 11. Effect of phosphorylated pullulan on osteoclast-like cell formation. The cells were RAW264.7. (A) Observation with an optical microscope. The percentage of phosphorylated pullulan in the culture medium is indicated. (B) The measurement of osteoclast-like cells. Significant difference test: *p < 0.05.
4 Discussion
When culturing cells on a Ti disk surface, examining the surface texture is necessary because the roughness of the Ti disk surface affects cell dynamics (Zizzari et al., 2015; Saruta et al., 2019; Hasegawa et al., 2020). In this study, # 400 waterproof abrasive paper was used as an intermediate roughness with no significant effect on cell adhesion, proliferation, or differentiation. Titanium has also been reported to be a difficult metal to grind (Shimakura et al., 2000). Therefore, in grinding Ti disks, we searched and selected a method that does not cause large scratches on the surface of the disk when the disk is observed by SEM and that allows uniform grinding of the entire surface.
Furthermore, the results of XPS measurements (Figure 4) indicated that once the phosphorylated pullulan was adsorbed to the titanium surface, it adsorbed firmly to the titanium surface without desorbing even under a strong physical cleaning force by ultrasound. In addition to XPS, FT-IR measurement, 31PNMR measurement, and the phosphorus–molybdenum method can be used to detect the presence of phosphorus elements or phosphate groups, but the detection sensitivity of these methods is weak on the topmost surface. XPS is an effective method of analyzing phosphorylated pullulan that is adsorbed to the Ti surface. Titanium combines with oxygen in the atmosphere and easily changes to titanium oxide to form a passivation film (Hanawa, 2022). Consequently, titanium becomes resistant to corrosion, making it an excellent implant material. Based on previous reports, titanium oxide hydrate and phosphate ions are adsorbed and affected by pH (Healyt and Ducheyne, 1992; Kang et al., 2011). Another report indicates that the phosphate group formed a strong chemical bond with titanium oxide, such as a chelate bond, following pH (Iravani et al., 2015). Thus, it is predicted an electrical or some other type of attraction occurred between titanium oxide and the phosphate group of phosphorylated pullulan. In addition, a detailed investigation of pH and other conditions may enable thicker phosphorylated pullulan films to adsorb Ti disk surfaces with a strong chemical bond. Further analysis of the adhesion mechanism between titanium dioxide and phosphate groups is required in the future.
In the cell proliferation test, the addition of phosphorylated pullulan to the culture medium slightly decreased cell proliferation. On the one hand, when phosphorylated pullulan was coated onto the Ti disk surface, the number of cells increased compared with the control. This trend may be due to the phosphorylated pullulan adsorption of molecules and ions. Phosphorylated pullulan on the Ti disk surface chemically or physically could adsorb carbohydrates, lipids, amino acids, vitamins, and various factors that act on cells in a culture medium (Warner, 2019). Thus, phosphorylated pullulan adsorbing to the Ti disk surface continuously may supply high-concentration functional molecules to the cells adhering to the Ti disk surface. On the other hand, when phosphorylated pullulan was added directly to the culture medium, the supply of molecules that act on the cells could be reduced for the cells adhering to the bottom of the culture dish. Molecules that act on cells could be adsorbed by phosphorylated pullulan, which is suspended in the culture medium. Consequently, cell proliferation decreased gradually as the concentration of phosphorylated pullulan increased.
A similar mechanism is suggested for the calcification induction test and the formation of osteoclast-like cells. The phosphorylated pullulan in the culture medium might adsorb dexamethasone, ascorbic acid, β-glycerophosphate, which are necessary for calcification, and RANKL, which is necessary for osteoclast-like cell formation. Therefore, these substances adsorbed by the phosphorylated pullulan in the culture medium could not reach the cells attached to the bottom of the culture dish. In addition, calcification induction and the formation of osteoclast-like cells did not occur sufficiently. This phenomenon may result in the suppression of calcification and a decrease in the number of osteoclast-like cells. On the contrary, in the case of phosphorylated pullulan on the Ti disk, the continuous and high-concentration supply of dexamethasone, ascorbic acid, and β-glycerophosphate adsorbed by phosphorylated pullulan to cells could promote calcification. However, it is unlikely that the phosphorylated pullulan-treated Ti disk was stained because of the adsorption of alizarin red to the phosphorylated pullulan. Considering that the structure of alizarin red consists of three benzene rings and two functional hydroxyl groups, the hydroxyl groups could be negatively charged in an aqueous solution. Therefore, it has the same charge as the phosphorylated pullulan. Thus, they could repel each other.
Consequently, cells can directly and continually receive molecules that act on cells in the culture medium and substances necessary for calcification from phosphorylated pullulan by selectively adhering phosphorylated pullulan to a target site such as a Ti disk.
The three elements involved in bone regeneration are scaffolding, cells, and growth factors. (Pilipchuk, 2015; Thrivikraman et al., 2017). In addition to surface modification of implants, which is related to scaffolding, this study also investigated growth factors. Furthermore, the use of growth factors can be an approach to a good improvement method for successful implant treatment. One bone growth factor is BMP-2. However, BMP-2 alone is not easily maintained on the Ti disk surface; therefore, a carrier with biodegradability, sustained release, and easy moldability is necessary when BMP-2 is applied to a living body (Bialy et al., 2017; Kowalczewski and Saul, 2018). Thus, we focused on the adsorb property of pullulan itself and the phosphate groups of phosphorylated pullulan. BMP-2 has a positive charge under a neutral solution (Guzman et al., 2015). If phosphate groups of phosphorylated pullulan ionize, it might electrically attract BMP-2. We thought that BMP-2 could be chemically or physically loaded on phosphorylated pullulan using these two properties. In fact, calcification was enhanced on Ti disks treated with a mixed solution of phosphorylated pullulan and BMP-2 compared with Ti disks treated only with BMP-2.
When phosphorylated pullulan bound to BMP-2 adsorbed to titanium, Saos-2 on the disk surface directly, continuously, and at high concentrations could receive BMP-2, dexamethasone, ascorbic acid, and β-glycerophosphate. Therefore, the formation of calcification on the Ti disks could be promoted. These results indicate that BMP-2 could be loaded onto phosphorylated pullulan without losing its function. However, excessive amounts of BMP-2 have a negative effect on calcification (Wikesjö et al., 2008; Chaudhar et al., 2012). Thus, the amount of BMP-2 used should be noted.
In current hydroxyapatite coatings, hydroxyapatite is not absorbed in the tissue when it is detached from the surface, so it remains for a long time and it can easily become a source of infection. However, phosphorylated pullulan treatment could chemically bond to titanium, and it has high adhesion compared with hydroxyapatite coating. In addition, phosphorylated pullulan treatment does not become a source of infection because phosphorylated pullulan is gradually replaced by bone when implanted in bone (Morimoto et al., 2023). It is relatively quickly absorbed, although it is detached. Infection is harmful to implant treatment, and phosphorylated pullulan treatment is considered to be a useful treatment method.
5 Conclusion
Based on the properties of phosphorylated pullulan, the effects of phosphorylated pullulan on cells and its function as a drug carrier in cell culture were examined.
The results showed that phosphorylated pullulan, when adsorbed to Ti disks, promotes the proliferation of osteoblast-like cells and induces calcification on the disks.
The drug carrier function of phosphorylated pullulan with BMP-2 was also shown to induce calcification on Ti disks.
The addition of phosphorylated pullulan to the culture medium affected the formation of osteoclast-like cells.
Therefore, phosphorylated pullulan affects the proliferation and differentiation of bone-associated cells depending on the application conditions. Moreover, the coating of phosphorylated pullulan on titanium implants may contribute to the improvement of osseointegration and the development of surface treatment methods for implants.
Data availability statement
The original contributions presented in the study are included in the article/Supplementary Material, further inquiries can be directed to the corresponding author.
Ethics statement
Ethical approval was not required for the studies on humans in accordance with the local legislation and institutional requirements because only commercially available established cell lines were used. Ethical approval was not required for the studies on animals in accordance with the local legislation and institutional requirements because only commercially available established cell lines were used.
Author contributions
KaN: Data curation, Formal Analysis, Investigation, Methodology, Visualization, Writing–original draft. KoN: Conceptualization, Formal Analysis, Investigation, Methodology, Visualization, Writing–original draft, Writing–review and editing. TA: Formal Analysis, Funding acquisition, Investigation, Methodology, Visualization, Writing–review and editing. SA: Investigation, Methodology, Writing–review and editing. KY: Investigation, Methodology, Writing–review and editing. MN: Funding acquisition, Writing–review and editing. HH: Funding acquisition, Writing–review and editing. ST: Formal Analysis, Investigation, Writing–review and editing. MT: Methodology, Project administration, Supervision, Writing–review and editing. YK: Project administration, Supervision, Writing–review and editing. BM: Methodology, Project administration, Supervision, Writing–review and editing. YY: Conceptualization, Project administration, Resources, Supervision, Writing–review and editing.
Funding
The author(s) declare financial support was received for the research, authorship, and/or publication of this article. This research was supported by the JSPS KAKENHI (Grant Numbers 19H04461 TA, 20K09950 MN, 23K11842 HH) and AMED under Grant Number JP22hk0102087.
Conflict of interest
The authors declare that the research was conducted in the absence of any commercial or financial relationships that could be construed as a potential conflict of interest.
Publisher’s note
All claims expressed in this article are solely those of the authors and do not necessarily represent those of their affiliated organizations, or those of the publisher, the editors and the reviewers. Any product that may be evaluated in this article, or claim that may be made by its manufacturer, is not guaranteed or endorsed by the publisher.
References
Abraham, C. M. (2014). A brief historical perspective on dental implants, their surface coatings and treatments. Open Dent. J. 8, 50–55. doi:10.2174/1874210601408010050
Albrektsson, T. (1998). Hydroxyapatite-coated implants: a case against their use. Oral Maxillofac. Surg. 56, 1312–1326. doi:10.1016/S0278-2391(98)90616-4
Aydogdu, H., Keskin, D., Baran, E. T., and Tezcaner, A. (2016). Pullulan microcarriers for bone tissue regeneration. Mater. Sci. Eng. 63, 439–449. doi:10.1016/j.msec.2016.03.002
Becker, W., Becker, B. E., Ricci, A., Bahat, O., Rosenberg, E., Rose, L. F., et al. (2000). A prospective multicenter clinical trial comparing one- and two-stage titanium screw-shaped fixtures with one-stage plasma-sprayed solid-screw fixtures. Clin. Implant Dent. Relat. Res. 2, 159–165. doi:10.1111/j.1708-8208.2000.tb00007.x
Bialy, I. E., Jiskoot, W., and Nejadnik, M. R. (2017). Formulation, delivery and stability of bone morphogenetic proteins for effective bone regeneration. Springer 34, 1152–1170. doi:10.1007/s11095-017-2147-x
Block, M. S. (2018). Dental implants: the last 100 years. J. Oral Maxillofac. Surg. 76, 11–26. doi:10.1016/j.joms.2017.08.045
Bosshardt, D. D., Chappuis, V., and Buser, D. (2017). Osseointegration of titanium, Titanium alloy and zirconia dental implants: current knowledge and open questions. Periodontology 73, 22–40. doi:10.1111/prd.12179
Cardoso, M. V., Chaudhari, A., Yoshida, Y., Meerbeek, B. V., Naert, I., and Duyck, J. (2014). Bone tissue response to implant surfaces functionalized with phosphate-containing polymers. Clin. Oral Implants Res. 25, 91–100. doi:10.1111/clr.12053
Cardoso, M. V., Rycker, J. D., Chaudhari, A., Coutinho, E., Yoshida, Y., Meerbeek, B. V., et al. (2017). Titanium implant functionalization with phosphate-containing polymers may favour in vivo osseointegration. J. Clin. Periodontology 44, 950–960. doi:10.1111/jcpe.12736
Chaudhari, A., Cardoso, M. V., Martens, J., Vandamme, K., Naert, I., and Duyck, J. (2012). Bone tissue response to BMP-2 adsorbed on amorphous microporous silica implants. J. Clin. Periodontology 39, 1206–1213. doi:10.1111/jcpe.12005
Choi, A. H., Ben-Nissan, B., Matinlinna, J. P., and Conway, R. C. (2013). Current perspectives: calcium phosphate nanocoatings and nanocomposite coatings in dentistry. J. Dent. Res. 92 (10), 853–859. doi:10.1177/0022034513497754
Chouirfa, H., Bouloussa, H., Migonney, V., and Falentin-Daudré, C. (2019). Review of titanium surface modification techniques and coatings for antibacterial applications. Acta Biomater. 83, 37–54. doi:10.1016/j.actbio.2018.10.036
Collaert, B., and De Bruyn, H. (2008). Immediate functional loading of TiOblast dental implants in full-arch edentulous maxillae: a 3-year prospective study. Clin. Oral Implants Res. 19, 1254–1260. doi:10.1111/j.1600-0501.2008.01586.x
Guzman, R. C. D., Tsuda, S. M., Ton, M.-T. N., Zhang, X., Esker, A. R., and Dyke, M. E. V. (2015). Binding interactions of keratin-based hair fiber extract to gold, keratin, and BMP-2. Plos One 10, e0137233. doi:10.1371/journal.pone.0137233
Hanawa, T. (2022). Biocompatibility of titanium from the viewpoint of its surface. Sci. Technol. Adv. Mater. 23, 457–472. doi:10.1080/14686996.2022.2106156
Hasegawa, M., Saruta, J., Hirota, M., Taniyama, T., Sugita, Y., Kubo, K., et al. (2020). A newly created meso-micro-and nano-scale rough titanium surface promotes bone-implant integration. Int. J. Mol. Sci. 21, 783. doi:10.3390/ijms21030783
Healy, K. E., and Ducheyne, P. (1992). Hydration and preferential molecular adsorption on titanium in vitro. Biomaterials 13, 553–561. doi:10.1016/0142-9612(92)90108-z
Iravani, E., Allahyari, S. A., Shojaeib, Z., and Torab-Mostaedia, M. (2015). Surface modification and spectroscopic characterization of TiO2 nanoparticles with 2-aminoethyl dihydrogen phosphate. J. Braz. Chem. Soc. 26 (8), 1608–1616. doi:10.5935/0103-5053.20150131
Kang, S. A., Li, W., Lee, H. E., Phillips, B. L., and Lee, Y. J. (2011). Phosphate uptake by TiO2: batch studies and NMR spectroscopic evidence for multisite adsorption. J. Colloid Interface Sci. 364, 455–461. doi:10.1016/j.jcis.2011.07.088
Kowalczewski, C. J., and Saul, J. M. (2018). Biomaterials for the delivery of growth factors and other therapeutic agents in tissue engineering approaches to bone regeneration. Front. Pharmacol. 9, 513. doi:10.3389/fphar.2018.00513
Kullar, A. S., and Miller, C. S. (2019). Are there contraindications for placing dental implants? Dent. Clin. N. Am. 63, 345–362. doi:10.1016/j.cden.2019.02.004
Liddelow, G., and Klineberg, I. (2011). Patient-related risk factors for implant therapy. A critique of pertinent literature. Aust. Dent. J. 56, 417–426. doi:10.1111/j.18347819.2011.01367.x
Mattila, P. K., and Lappalainen, P. (2008). Filopodia: molecular architecture and cellular functions. Nat. Rev. Mol. Cell Biol. 9, 446–454. doi:10.1038/nrm2406
Morimoto, Y., Hasegawa, T., Hongo, H., Yamamoto, T., Maruoka, H., Haraguchi-Kitakamae, M., et al. (2023). Phosphorylated pullulan promotes calcification during bone regeneration in the bone defects of rat tibiae. Front. Bioeng. Biotechnol. 11, 1243951. doi:10.3389/fbioe.2023.1243951
Pilipchuk, S. P., Plonka, A. B., Monje, A., Taut, A. D., Lanis, A., Kang, B., et al. (2015). Tissue engineering for bone regeneration and osseointegration in the oral cavity. Dent. Mater. 31, 317–338. doi:10.1016/j.dental.2015.01.006
Saruta, J., Sato, N., Ishijima, M., Okubo, T., Hirota, M., and Ogawa, T. (2019). Disproportionate effect of sub-micron topography on osteoconductive capability of titanium. Int. J. Mol. Sci. 20, 4027. doi:10.3390/ijms20164027
Shimakura, M., Yamamoto, M., Nakajima, K., and Yoshida, N. (2000). Application of a centrifugal shooting type polishing system to polish pure titanium. Dent. Mater. J. 19, 405–412. doi:10.4012/dmj.19.405
Smeets, R., Stadlinger, B., Schwarz, F., Beck-Broichsitter, B., Jung, O., Precht, C., et al. (2016). Impact of dental implant surface modifications on osseointegration. BioMed Res. Int. 2016, 1–16. doi:10.1155/2016/6285620
Thrivikraman, G., Athirasala, A., Twohig, C., Boda, S. K., and Bertassoni, L. E. (2017). Biomaterials for craniofacial bone regeneration. Dent. Clin. N. Am. 61, 835–856. doi:10.1016/j.cden.2017.06.003
Warner, R. D. (2019). Review: analysis of the process and drivers for cellular meat production. Animal 13 (12), 3041–3058. doi:10.1017/S1751731119001897
Wegener, B., Sichler, A., Milz, S., Sprecher, C., Pieper, K., Hermanns, W., et al. (2020). Development of a novel biodegradable porous iron-based implant for bone replacement. Sci. Rep. 10, 9141. doi:10.1038/s41598-020-66289-y
Wikesjö, U. M. E., Qahash, M., Polimeni, G., Susin, C., Shanaman, R. H., Rohrer, M. D., et al. (2008). Alveolar ridge augmentation using implants coated with recombinant human bone morphogenetic protein-2: histologic observations. J. Clin. Periodontology 35, 1001–1010. doi:10.1111/j.1600-051X.2008.01321.x
Yonehiro, J., Yoshida, Y., Yamashita, A., Yoshizawa, S., Ohta, K., Kamata, N., et al. (2013). Flavonol-containing phosphorylated pullulan may attenuate pulp inflammation. Int. Endod. J. 46, 119–127. doi:10.1111/j.1365-2591.2012.02095.x
Keywords: phosphorylated pullulan, dental implants, surface modification, cellular response, titanium
Citation: Nagamoto K, Nakanishi K, Akasaka T, Abe S, Yoshihara K, Nakamura M, Hayashi H, Takemoto S, Tamura M, Kitagawa Y, Van Meerbeek B and Yoshida Y (2024) Investigation of a new implant surface modification using phosphorylated pullulan. Front. Bioeng. Biotechnol. 12:1378039. doi: 10.3389/fbioe.2024.1378039
Received: 29 January 2024; Accepted: 01 May 2024;
Published: 22 May 2024.
Edited by:
Alejandro Dario Sosnik, Technion Israel Institute of Technology, IsraelReviewed by:
Silvia Spriano, Polytechnic University of Turin, ItalyPeng Chen, Tohoku University, Japan
Masahiro Okada, Okayama University, Japan
Copyright © 2024 Nagamoto, Nakanishi, Akasaka, Abe, Yoshihara, Nakamura, Hayashi, Takemoto, Tamura, Kitagawa, Van Meerbeek and Yoshida. This is an open-access article distributed under the terms of the Creative Commons Attribution License (CC BY). The use, distribution or reproduction in other forums is permitted, provided the original author(s) and the copyright owner(s) are credited and that the original publication in this journal is cited, in accordance with accepted academic practice. No use, distribution or reproduction is permitted which does not comply with these terms.
*Correspondence: Ko Nakanishi, bmFrYW5pc2hpLWtvQGRlbi5ob2t1ZGFpLmFjLmpw