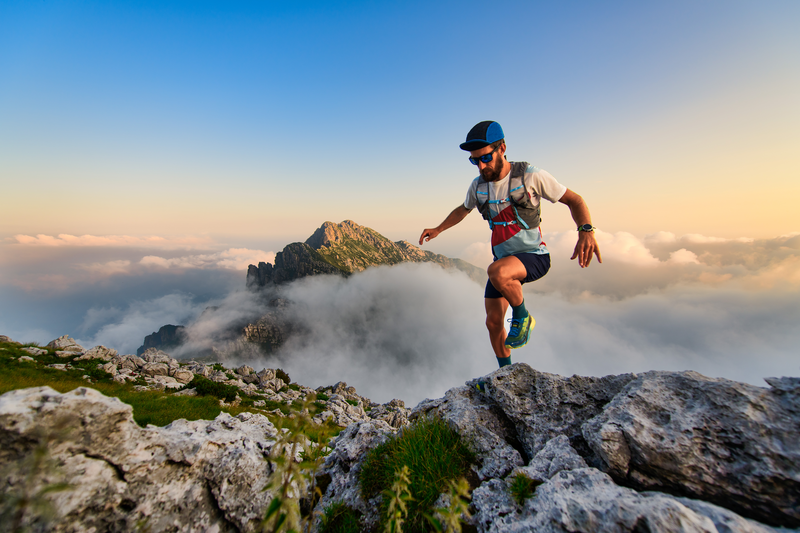
94% of researchers rate our articles as excellent or good
Learn more about the work of our research integrity team to safeguard the quality of each article we publish.
Find out more
ORIGINAL RESEARCH article
Front. Bioeng. Biotechnol. , 21 June 2024
Sec. Biomechanics
Volume 12 - 2024 | https://doi.org/10.3389/fbioe.2024.1364095
Objective: The purpose of this study is to analyze the inherent relationship between the score values and the biomechanical characteristics of the forward kicking motion, we aim to identify the fundamental variables influencing the score values of the forward kicking motion and establish the key biomechanical factors that effectively trigger scoring in the forward kicking motion.
Methods: The DaeDo electronic scoring system was used with the Vicon optical motion capture system and the Kistler 3D force platform to obtain kinematic and kinetic variables of the front roundhouse kick motion. Linear bivariate correlation analysis and principal component analysis were used to analyze the associations between kinematic, kinetic variables, and scoring values, and summarize key biomechanical factors for effectively scoring.
Results: The peak ankle plantar flexion angle and knee extension torque of the kicking leg showed a significant negative correlation with scoring values (r < 0, p < 0.05), while other variables showed no statistical significance. The peak knee flexion angle and hip extension angular velocity of the supporting leg showed a significant positive correlation with scoring values (r > 0, p < 0.01), while the peak ankle plantar flexion torque showed a significant negative correlation with scoring values (r < 0, p < 0.05), and other variables showed no statistically significant correlation. The absolute values of eigenvectors of the first and second principal components, which included hip angular velocity, ankle angle, knee torque, and hip torque, were relatively large, indicating their strong influence on effective scoring triggering.
Conclusion: Maintaining ankle dorsiflexion and a larger knee flexion angle in the kicking leg is favorable for triggering scoring. Higher knee flexion angle and hip extension angular velocity in the supporting leg are also advantageous for triggering scoring. “Body posture” and “Strength” are key factors that effectively trigger scoring.
Competitive Taekwondo is a combative sport characterized by direct physical contact and intense confrontation (Pieter et al., 2012). Its action traits include fast technical movements, short contact time, and frequent changes in techniques and tactics (Lin et al., 2023). In past competitions, referees had to subjectively judge the effectiveness of athletes’ strikes (Zhi et al., 2010). Because there is a blind spot in the field of vision when athletes come into contact with each other, judging results were prone to unfairness, partiality, and delays (Fan et al., 2010; Qing et al., 2014). To address these issues, significant revisions were made to the Taekwondo competition regulation after the Rio Olympics, leading the sport into the era of electronic protective gear (Lin and Gao, 2020). As the official certified brand of the World Taekwondo Federation, DaeDo electronic protective gear have been widely used in major events such as the Olympics and World Championships (Nan and Jian, 2017).
In competitive Taekwondo, the effectiveness of strikes is not only influenced by strength but also closely related to the strike precision, angle, and hit rate on the target area (Razman and Chong, 2019). The features of competitive Taekwondo techniques are powerful strike to the opponent’s trunk or head with the lower limbs (Geßlein et al., 2020). Therefore, athletes need to focus on enhancing the explosiveness of muscles and the coordination of movements during their training to improve strike power and speed. Additionally, by designing appropriate technical actions, conducting professional physical training, and enhancing body flexibility in postural control, the strength and accuracy of strikes can be further improved.
Taekwondo have high extremely requirements for flexibility and specialized strength from athletes (Bridge et al., 2014). It involves frequent use of kicking and striking techniques in order to eliminate opponents through scoring. The scoring areas of electronic protective gear mainly include the opponent’s trunk and head. The characteristic of front roundhouse kick involves significant limb movements in a short amount of time, requiring athletes to possess high levels of flexibility and coordination. Additionally, athletes need to have excellent specialized strength, including muscle strength, explosive power, and postural control (Nan et al., 2017). Through training and techniques accumulation, athletes can swiftly use front roundhouse kick actions for both offensive and defensive purposes, making it difficult for opponents to predict and respond. Moreover, the feature of this action minimizes the vulnerabilities exposed by athletes, effectively enhancing their defensive capabilities (Son et al., 2020).
The current literature on the front roundhouse kick mainly involves technical and tactical adjustments under the new rules (He and Pang, 2019), biomechanical characteristics of specific techniques (Aloui et al., 2022; Liu et al., 2023), and the impact of training on kicking techniques (Ojeda-et al., 2021; Sant’ana et al., 2021). However, in studies related to the use of DaeDo electronic body protectors to trigger scoring in Taekwondo, there is a lack of research on key biomechanical factors related to the scoring values of the front roundhouse kick. In other sports, research on key biomechanical factors has been able to determine the primary factors that determine competition results (Bullock et al., 2009; Crossland et al., 2011; Tomasevicz et al., 2020). It is hypothesized that there is relatively little attention given to the relationship between the value of effective scoring and the kinematic and kinetic characteristics of the kicking motion when using electronic protectors to trigger valid scoring.
Therefore, this study aims to investigate the correlation between the kicking speed, posture control in movements (Liu et al., 2023), and the scoring values by electronic protective gear of the front roundhouse kick, using high-speed camera systems and a three-dimensional force platform. By analyzing the intrinsic relationship between scoring values and the biomechanical characteristics of the front roundhouse kick, this study aims to reveal the underlying variables that influence scoring values and summary key biomechanical factors for effectively triggering score with the front roundhouse kick. This will provide Taekwondo coaches and athletes with variables to assess the effectiveness of the front roundhouse kick in triggering the electronic protective gear, thus offering theoretical support for improving the training effectiveness of the front roundhouse kick technique in Taekwondo and triggering scores effectively during competitions.
The novelty of this study lies in the investigation of the correlation between the biomechanical characteristics of the lower extremities during the front roundhouse kick in Taekwondo and the effective scores of electronic protectors. This study aims to analyze the intrinsic relationship between scoring values and biomechanical factors, providing insights into the variables that influence scoring values and identifying key biomechanical factors for effectively triggering scores with the front roundhouse kick. This research fills the gap in the existing literature by focusing on the biomechanical factors related to scoring values in Taekwondo using electronic protective gear.
In this study, we used G Power 3.1.9.2 to calculate the required sample size with an effect size of 0.7. Under the conditions of α = 0.05 and a statistical power of 95%, 13 participants were needed. Based on the weight class set for men’s Taekwondo competitions in the Olympic Games, Finally, 15 excellent athletes from Wuhan Sports University Taekwondo sports training team, (with a weight range of 54–87 kg), were recruited as the participants for this experiment (18.00 ± 2.20 years, 182.15 ± 8.62 cm, 70.00 ± 14.82 kg). All participants had experience participating in regional-level or higher-level competitions and were in a healthy physical condition that would not influence their kicking performance. None of the participants engaged in intense exercise within 24 h prior to the experiment, and they had no significant injuries or abnormalities in their anatomical structure or function within the past 6 months. All participants exhibited good overall physical condition and athletic abilities.
Based on the characteristics of the front roundhouse kick motion, relevant studies (Jeong et al., 2021; Jian et al., 2020; Qiu et al., 2021), and interviews with coaches and athletes, this study divided the front roundhouse kick movement into four events and two phases, as shown in Figure 1.
Preparation Moment (E1): The moment when the striking leg leaves the ground and the moment when the attacking foot rise from the force platform with no ground reaction force. Lift Knee Moment (E2): The moment of minimal flexion of the striking leg knee joint and the moment of maximum angle of the striking leg knee joint. Kick Moment (E3): The moment of maximum extension of the striking leg knee joint and the moment of minimum angle of the striking leg knee joint. Recovery Moment (E4): The moment the striking leg lands and the moment the attacking foot touches the force platform with ground reaction force emerge. Lift Knee Phase (P1): From the preparation moment to the lift knee moment, E1-E2. Striking Phase (P2): From the lift knee moment to the Kick moment, E2-E3. Recovery Phase (P3): From the kick moment to the recovery moment, E3-E4. In this study, the striking leg is defined as the lower limb of one side that hit the dummy, and the lower limb in contact with the ground is defined as the supporting leg.
The experiment utilized 9 infrared high-speed cameras (T40, Vicon, United Kingdom) with a sampling frequency of 200 Hz and Marker balls with a diameter of 14 mm to collect kinematic data. The Kistler 3D force platform (9260AA6, Switzerland) with 4 independent force plates of size 60 × 40 cm and a sampling frequency of 1,500 Hz was used to collect dynamic data. Synchronization between the Vicon system and the Kistler system was achieved through a digital signal converter. The scoring values were recorded using the Korean DaeDo electronic protective gear and scoring system (specifically designed for the Olympic Games), which included torso protectors, electronic helmets, and electronic foot guards. During the experiment, the dummy was in an upright position, and the electronic protective gear was worn by the dummy. The electronic protective gear was wirelessly connected to the computer through a signal receiver.
This study has been reviewed and approved by the ethics review of Wuhan Sports University (NO. 2022048) and participants were instructed on the testing procedures prior to the test. The test procedures are illustrated in Figure 2. Preparations before the test involved initializing and calibrating the Vicon 3D motion capture system, preheating and calibrating the Kistler 3D force platform, as well as testing and calibrating the Daedo electronic protective gear. Synchronization of data between the Vicon and Kistler systems was confirmed. Participants underwent a health assessment and provided informed consent form, being informed about the experimental procedures and precautions. Participants engaged in a 15-min warm-up activity, followed by attaching reflective Marker balls. Once the attachment was completed, participants were instructed to rapidly strike the dummy equipped with the electronic protective gear according to the predefined global coordinate direction and the position of the target dummy. The height of the dummy was adjusted to match the habitual striking height of the participants. After the athletes fully adapted, the experimenters checked the signals of the electronic protective gear and set the electronic scoring system to the corresponding weight class of the participant’s match. Data collection commenced thereafter.
In taekwondo competitions, in order to complete movements with higher quality and achieve the goal of scoring, the collected actions in this study aimed to simulate realistic combat situations as closely as possible. The participants performed a combination of step-in and front roundhouse kick, as shown in Figure 1. After each movement of the subject is completed, the same coach determined whether the test action met the technical standards for a front roundhouse kick, and five successful actions were selected as test samples. The dominant kicking limb of all subjects was the right leg.
Definition of Joint Coordinate System and Joint Angles according to the setup in Visual 3D. The joint coordinate system is defined with three axes in reference to the participant’s body. The axes include the coronal axis (X-axis, flexion/extension), the sagittal axis (Y-axis, abduction/adduction), and the vertical axis (Z-axis, internal/external rotation). The following joint angles are defined: Ankle Joint Angle: The angle between the foot and the line extending from the leg. Knee Joint Angle: The angle between the lower leg and the line extending from the thigh. Hip Joint Angle: The angle between the thigh and the line extending from the trunk.
During the front roundhouse kick, the joint movement sequence of the striking leg involves pelvic rotation, followed by simultaneous flexion of the knee joint, hip abduction/adduction, and trunk rotation until contact with the target is made (Chun et al., 2020). Studies on front roundhouse kick in taekwondo typically analyze the movement process using variables such as maximum joint angles, peak joint angular velocities, peak joint moments, and vertical ground reaction forces (Moreira and Paula, 2017; Sant’ana et al., 2017; Chang et al., 2021; Jeong et al., 2021; Liu et al., 2021; Pei and Jian, 2021; Zhao and Jia, 2022; Zhi et al., 2022). The selected variables for this study are as follows:
1) Range of Motion of Hip, Knee, and Ankle Joints: The maximum flexion/extension angles (°) of the hip, knee, and ankle joints for both the striking leg and the supporting leg. Flexion of the hip, knee, and dorsiflexion of the ankle are defined as positive directions.
2) Velocity of Motion of Hip, Knee, and Ankle Joints: The peak angular velocities (rad/s) of the hip, knee, and ankle joints for both the striking leg and the supporting leg are measured. The direction of joint angular velocities follows the same definition as the joint angles.
3) Kinematics: The peak knee joint moment (N·m), ankle joint moment (N·m), hip joint moment (N·m) for both the kicking leg and the supporting leg are measured. Additionally, the vertical ground reaction force (vGRF), after being normalized to body weight (BW), is also measured.
4) Score Value: The score value (Unitless) corresponding to the effective front roundhouse kick action is measured.
Select the three optimal movements from each participant’s data, and export them as C3D files after marker labeling and gap filling in Vicon software. Then import the C3D files into Visual 3D software. The lower limb model is used for static modeling, and relevant variables are computed. The kinematic and kinetic data are filtered with cut-off frequencies of 10 Hz and 25 Hz, respectively (Ibrahim et al., 2014; Moreira and Paula, 2017; Yi et al., 2018). The kinetic data are normalized to each participant’s body weight multiplied by their height. The kinematic, kinetic, and score values of the three selected data sets are averaged.
In this study, statistical analysis of the data was performed using SPSS 19.0 software. Linear bivariate correlation analysis was conducted to examine the relationships between kinematics, kinetics, muscle activity, and score values (Winter et al., 2001; Keylock et al., 2022). The significance level was set at p < 0.05 to determine statistical significance. One asterisks (*) denote p < 0.05, while double asterisks (**) denote p < 0.01.
Based on the research variables, we chose ten key variables that may influence the success or failure of athletes in triggering scores, as shown in Figure 3. These variables include hip, knee, ankle joint angles, angular velocities, joint moments, and vertical ground reaction forces. All variables were computed using Visual 3D software. Principal Component Analysis (PCA) was performed using the “factoextra” package in R software (Warmenhoven et al., 2021; Jiang et al., 2023). The sample correlation matrix was calculated based on the sample data matrix, and the eigenvalues and eigenvectors of the correlation matrix were obtained. The principal components and their contributions were determined based on these eigenvalues and eigenvectors. Finally, key variables to trigger scores in the front roundhouse kick movements were identified through PCA.
As shown in Figure 4A, for striking leg, significant negative correlations were observed between the peak dorsiflexion angle of the ankle joint and the peak extension moment of the knee joint (r = −0.349, p = 0.020; r = −0.306, p = 0.043), as well as no statistically significant relationships between the peak flexion angle of the knee joint, peak extension angle of the hip joint, peak extension angular velocity of the knee joint, peak plantar flexion angular velocity of the ankle joint, peak extension angular velocity of the hip joint, peak plantar flexion moment of the ankle joint, peak extension moment of the hip joint and the score values (p > 0.05).
Figure 4. (A) kinematics and kinetic characteristics of the striking leg; (B) kinematics and kinetic characteristics of the supporting leg (*) significant discrepancy p < 0.05 (**) very significant discrepancy, p < 0.01).
Furthermore, as shown in Figure 4B, for supporting leg, there were significant positive correlations between the peak flexion angle of the knee joint, peak extension angular velocity of the hip joint, and peak dorsiflexion moment of the ankle joint with the score values (r = 0.573, p = 0.000; r = 0.321, p = 0.034; r = 0.368, p = 0.014). Similarly, no statistically significant relationships were found between the peak dorsiflexion angle of the ankle joint, peak extension angle of the hip joint, peak extension angular velocity of the knee joint, peak dorsiflexion angular velocity of the ankle joint, peak extension moment of the knee joint, peak flexion moment of the hip joint, peak vertical ground reaction force and the score values (p > 0.05).
An assessment was conducted on the suitability of the research data for principal component analysis. From Table 1, it can be observed that the Kaiser-Meyer-Olkin (KMO) measure is 0.608, surpassing the threshold of 0.6, thereby satisfying the prerequisite requirement for conducting principal component analysis. This indicates that the data can be utilized for principal component analysis. Furthermore, the data successfully passed the Bartlett’s sphericity test (p < 0.05), affirming the appropriateness of employing principal component analysis on the research data.
Table 2 presents an analysis of the principal component extraction and the amount of information extracted. From the table, it is evident that a total of 6 principal components were extracted in the principal component analysis, with eigenvalues all greater than 1. The variance explained by these 6 principal components are 29.560%, 13.605%, 10.518%, 9.725%, 7.802%, and 5.394% respectively, with a cumulative variance explained of 76.603%. Furthermore, the analysis yielded 6 principal components, with their corresponding weighted variance explained as follows: 29.560/76.603 = 38.59%; 13.605/76.603 = 17.76%; 10.518/76.603 = 13.73%; 9.725/76.603 = 12.70%; 7.802/76.603 = 10.18%; 5.394/76.603 = 7.04%.
Table 3 illustrates the information extraction of the principal components for the research items and their corresponding relationships. From the table, it can be observed that all research items have communalities values higher than 0.4, indicating a strong correlation between the research items and the principal components, enabling effective information extraction. Therefore, this study extracted relevant information from the loading coefficient table of each principal component. PC1, PC2, PC5, and PC6 primarily contain joint angles and angular velocities of the offensive leg, joint torques, angular velocities, and vertical ground reaction force of the support leg. These indicators reflect the posture of the offensive leg and the muscle recruitment ability of the support leg. Hence, PC1 is defined as the body posture and muscular strength factor. PC3 mainly encompasses joint angles and angular velocities of both the offensive and support legs, thus referred to as the body posture factor. PC4 primarily involves joint torques of the offensive leg and is defined as the muscular strength factor. The 6 principal components mainly reflect the body posture and muscular strength of athletes performing sideways kicking movements.
The research findings indicate a strong correlation between the peak joint angles and peak joint angular velocities of the selected offensive leg and support leg with the effective triggering of scores. It is speculated that this is because the movement between joints in the front roundhouse kick action proceeds in a proximal-to-distal sequence, where the peak velocities in the proximal segments are smaller than those in the distal segments. Joints accelerate sequentially from the proximal segments to the distal segments, enabling the foot to reach maximum linear velocity (Estevan et al., 2015). In the accelerating phase of the striking leg, If the knee joint moment remains high, it hampers the transmission of speed from the proximal to the distal segments, thus failing to accelerate the foot to the conditions for effective triggering of scoring. On the other hand, a larger knee extension moment may be disadvantageous for knee flexion. According to Quinzi et al. (2013), a lower knee flexion angle leads to a smaller rotation area of the striking leg, and Ying et al. (2011) also indicated that insufficient knee flexion significantly affects the rotational speed of the striking leg with the hip joint as the center, thereby affecting the transmission of inertia torque to the striking phase (Crossland et al., 2011). Similarly, Liu et al. (2021) suggests that the amplitude of knee flexion directly affects the speed of the striking phase. A greater internal/external rotation angular velocity of the knee joint during the knee lifting phase is more conducive to increasing the knee flexion and extension velocity, and the mass distribution of the lower extremity is closer to the axis of rotation. According to the results of principal component analysis, peak hip joint moment and peak knee joint moment are the key variables affecting effective triggering score of the roundhouse kick action. Hoelbling et al. (2020) have already shown that for the front roundhouse kick, the hip flexors must counteract the hip extension moment, which is caused by the knee joint’s inertial torque in the direction of extension during the angular acceleration process. When the knee joint extends, the angular velocity of the hip joint is relatively lower, producing a greater hip flexion moment, which increases the foot’s linear velocity during the transmission to the distal segment, thus more conducive to triggering scoring.
The present study found that the peak joint torque values of the offensive leg and support leg are also crucial factors in triggering effective score values. One study (Machado et al., 2010) suggests that the striking techniques requires contact between the back of the foot and the electronic protective gear worn by the dummy, and the contact area between the foot and the electronic protective gear directly affects whether the final score can be triggered. During the kick moment, the inversion/eversion of the ankle joint affects the vertical component of contact between the foot and the opponent, leading to a decrease in the contact area between the foot and the opponent, thus affecting the score. The results of this study indicate that larger dorsiflexion angle at the ankle joint adversely affects trigger scoring. This is because during the kick moment, the contact between the dorsum of the foot and the electronic protective gear is not completely perpendicular, the angles and moments at the ankle joint generate components in the sagittal and vertical axes that are not sufficient to trigger scoring. Therefore, in matches where electronic protective gear is used, apart from factors like speed and strength, the angle of the ankle joint is also a crucial factor influencing scoring. Athletes should pay attention to reducing inversion/eversion of the ankle joint in their daily training.
We also found a significant positive correlation between the hip joint extension angular velocity of the supporting leg, the knee joint flexion angle and the scoring value. There was also a significant negative correlation between the ankle joint plantar flexion moment and the scoring value. In order to trigger scoring, the goal of striking is to generate sufficient speed at the distal end of the striking leg, requiring close coordination between the lower limbs and the trunk (Ervilha et al., 2020). During the knee lifting phase, the trunk undergoes significant rotation around its axis to obtain a larger moment of inertia, thus driving the lower limbs to complete the striking action (Shi et al., 2012). For the supporting leg, its stability is a key factor in ensuring trigger scores (Giroux et al., 2016). Excessive knee joint flexion/extension moment hinders the transfer of moment of inertia from the trunk to the striking leg, lowering the quality of subsequent actions. Previous studies have shown a significant correlation between the extension moment generated at the hip joint and the flexion angular velocity of knee joint (Fong and Tsang, 2012). The sagittal axis ground reaction force can provide moment for hip joint rotation, accelerating body rotation to drive the striking leg to complete the striking action. At the same time, the forward kinetic energy of the body is converted into elastic potential energy stored in the lower limb muscles, which is then released during take-off, generating a larger vertical ground reaction force (Yu and Ji, 2020). This feedbacks into the knee lifting phase, increasing the height of knee-lifting for athletes. The striking leg generates significant impact force at the moment of striking, but the supporting leg prevents the body from moving backward after the impact, thus making attack of the subsequent kicking more powerful (Flanagan and Salem, 2008).
The peak ankle dorsiflexion angle, peak knee extension moment of the kicking leg, peak knee flexion angle of the supporting leg, peak hip extension angular velocity, and peak ankle dorsiflexion moment were identified as key biomechanical indicators influencing effective scoring in the forward kicking motion. There was a strong correlation between the joint angle peaks, joint angular velocity peaks, joint moment peaks, and vertical ground reaction force of the supporting leg with effective scoring. The factors of “body posture” and “muscle strength” were identified as crucial factors for executing the forward kicking motion and triggering effective scoring.
In summary, the key factors for effectively triggering scoring in electronic protective gear are body posture and strength. Taekwondo coaches and athletes can focus on developing specialized training sessions for body posture and strength training, thereby improving effective scoring ratios in competitive competitions. Due to the limitations of this study, the definition of the principal component “muscle strength” factor was characterized solely by joint torque. Future research needs to confirm the effect of lower limb joint muscle strength on effectively triggering scores. Furthermore, this study only examined the overall correlation between peak values of relevant variables and score values. Future research should explicitly identify the key biomechanical factors for effectively triggering scores at different moments (preparation, knee-lift, striking, recovery).
The raw data supporting the conclusion of this article will be made available by the authors, without undue reservation.
The studies involving humans were approved by the ethical review of Wuhan Sports University. The studies were conducted in accordance with the local legislation and institutional requirements. Written informed consent for participation in this study was provided by the participants’ legal guardians/next of kin.
MJ: Conceptualization, Data curation, Formal Analysis, Investigation, Methodology, Software, Supervision, Visualization, Writing–original draft, Writing–review and editing, Project administration, Validation. YM: Funding acquisition, Project administration, Resources, Supervision, Writing–review and editing. RH: Investigation, Validation, Writing–review and editing. LL: Validation, Writing–review and editing. ZW: Data curation, Investigation, Validation, Writing–review and editing. SL: Funding acquisition, Resources, Supervision, Writing–review and editing. QP: Data curation, Formal Analysis, Supervision, Writing–review and editing. JX: Investigation, Project administration, Resources, Writing–review and editing. WZ: Conceptualization, Methodology, Writing–review and editing.
The author(s) declare that financial support was received for the research, authorship, and/or publication of this article. This study was supported by the funding of the East Lake Scholars Sponsorship Program of Wuhan Sports University in China, Hubei Provincial Department of Education of China [grant number D20194101], Natural Science Basic Research Program of Shangxi Province [grant number 2022JQ-051], Science and Technology Team Foundation of Wuhan Sports University [grant number 21KT02], the 14th Five-Year-Plan Advantageous and Characteristic Disciplines (Groups) of Colleges and Universities in Hubei Province [grant number 2021-05].
The authors declare that the research was conducted in the absence of any commercial or financial relationships that could be construed as a potential conflict of interest.
All claims expressed in this article are solely those of the authors and do not necessarily represent those of their affiliated organizations, or those of the publisher, the editors and the reviewers. Any product that may be evaluated in this article, or claim that may be made by its manufacturer, is not guaranteed or endorsed by the publisher.
Aloui, A., Tayech, A., Mejri, M. A., Makhlouf, I., Clark, C. C., Granacher, U., et al. (2022). Reliability and validity of a new taekwondo-specific change-of-direction speed test with striking techniques in elite taekwondo athletes: a pilot study. Front. Physiology 13, 774546. doi:10.3389/fphys.2022.774546
Bridge, C. A., Ferreira Da Silva Santos, J., Chaabene, H., Pieter, W., and Franchini, E. (2014). Physical and physiological profiles of taekwondo athletes. Sports Med. 44, 713–733. doi:10.1007/s40279-014-0159-9
Bullock, N., Gulbin, J. P., Martin, D. T., Ross, A., Holland, T., and Marino, F. (2009). Talent identification and deliberate programming in skeleton: ice novice to Winter Olympian in 14 months. J. Sports Sci. 27 (4), 397–404. doi:10.1080/02640410802549751
Chang, W. G., Lin, K. Y., Chu, M. Y., and Chow, T. H. (2021). Differences in pivot leg kinematics and electromyography activation in various round house kicking heights. J. Sports Sci. Med. 20 (3), 457–465. doi:10.52082/jssm.2021.457
Chun, J. X., Wei, J. L., DE, H. L., and Hai, L. Y. (2020). Effect of hitting speed of four tactical movements of taekwondo back roundhouse kick and its enlightenment for sports training. J. Chengdu Sport Univ. 46 (1), 114–120. doi:10.15942/j.jcsu.2020.01.018
Crossland, B. W., Hartman, J. E., Kilgore, J. L., Hartman, M. J., and Kaus, J. M. (2011). Upper-body anthropometric and strength measures and their relationship to start time in elite luge athletes. J. Strength & Cond. Res. 25 (10), 2639–2644. doi:10.1519/JSC.0b013e318207ed7a
Ervilha, U. F., Fernandes, F. D. M., Souza, C. C. D., and Hamill, J. (2020). Reaction time and muscle activation patterns in elite and novice athletes performing a taekwondo kick. Sports Biomech. 19 (5), 665–677. doi:10.1080/14763141.2018.1515244
Estevan, I., Falco, C., Silvernail, J. F., and Jandacka, D. (2015). Comparison of lower limb segments kinematics in a Taekwondo kick. An approach to the proximal to distal motion. J. Hum. Kinet. 47 (1), 41–49. doi:10.1515/hukin-2015-0060
Fan, L., Zhi, H. G., and Liang, Z. L. (2010). First time application of electronic taekwondo protective gear in China. J. Wuhan Inst. Phys. Educ. 44 (10), 73–77+96. doi:10.3969/j.issn.1000-520X.2010.10.016
Flanagan, S. P., and Salem, G. J. (2008). Lower extremity joint kinetic responses to external resistance variations. J. Appl. Biomechanics 24 (1), 58–68. doi:10.1123/jab.24.1.58
Fong, S. S., and Tsang, W. W. (2012). Relationship between the duration of taekwondo training and lower limb muscle strength in adolescents. Hong Kong Physiother. J. 30 (1), 25–28. doi:10.1016/j.hkpj.2011.11.004
GeßLEIN, M., Rüther, J., Bail, H. J., Schuster, P., Krutsch, W., and Wolpert, A. K. (2020). Injury incidence rates and profiles in elite taekwondo during competition and training. Int. J. Sports Med. 41 (1), 54–58. doi:10.1055/a-1021-1776
Giroux, C., Rabita, G., Chollet, D., and Guilhem, G. (2016). Optimal balance between force and velocity differs among world-class athletes. J. Appl. Biomechanics 32 (1), 59–68. doi:10.1123/jab.2015-0070
He, Y., and Pang, J. P. (2019). Development trend of men’s taekwondo techniques and tactics under new rules: taking world champion lee dae-hoon as an example. J. Wuhan Sports Univ. 53 (12), 82–87. doi:10.15930/j.cnki.wtxb.2019.12.012
Hoelbling, D., Baca, A., and Dabnichki, P. (2020). Sequential action, power generation and balance characteristics of a martial arts kick combination. Int. J. Perform. Analysis Sport 20 (5), 766–781. doi:10.1080/24748668.2020.1774730
Ibrahim Haridy, A. M., and Ibrahim Atta, I. (2014). Analytical study of some biomechanical variables and their effect on achievement level in the Pole vault. J. Appl. Sports Sci. 4 (1), 32–39. doi:10.55384/2790-4237.1043
Jeong, H. S., O'Sullivan, D. M., Jeong, D. H., and Lee, S. Y. (2021). Sports injuries and illnesses after implementation of the web-based surveillance system in world Taekwondo. J. Athl. Train. 56 (11), 1232–1238. doi:10.4085/330-19
Jian, J. S., Ying, H. Z., Yu, L., Zhi, H. W., Chao, H. F., and Yuan, L. (2020). The covariate analysis of kinematic characteristics of taekwondo athletes’ ankle joints in turning kick with different fatigues. Chin. J. Sports Med. 39 (12), 924–931. doi:10.3969/j.issn.1000-6710.2020.12.002
Jiang, X., Xu, D., Fang, Y., Bíró, I., Baker, J. S., and Gu, Y. (2023). PCA of running biomechanics after 5 km between novice and experienced runners. Bioengineering 10 (7), 876. doi:10.3390/bioengineering10070876
Keylock, L., Felton, P., Alway, P., Brooke-Wavell, K., Peirce, N., and King, M. (2022). Lumbar bone mineral adaptation: the effect of fast bowling technique in adolescent cricketers. Med. Sci. Sports Exerc. 54 (3), 438–446. doi:10.1249/mss.0000000000002820
Lin, D. S., and Gao, Z. H. (2020). The characteristics of the use of turning kick skills and training countermeasures in taekwondo competition under new rules. J. Beijing Sport Univ. 43 (10), 114–123. doi:10.19582/j.cnki.11-3785/g8.2020.10.011
Lin, L., Yong, M., Shi, J. L., Qian, P., Ling, L. D., Jun, X., et al. (2023). Biomechanics research on laterality effect between dominant and non-dominant during front cross kick in taekwondo. J. Wuhan Sports Univ. 57 (1), 73–81. doi:10.3969/j.issn.1000-520X.2023.01.010
Liu, T. T., Lin, Y. C., Tang, W. T., Hamill, J., and Chang, J. S. (2021). Lower-limb kinematic characteristics of Taekwondo kicks at different attack angles. Int. J. Perform. Analysis Sport 21 (4), 519–531. doi:10.1080/24748668.2021.1924526
Liu, L., Jia, M. Y., Ma, Y., Lin, S. J., Peng, Q., Xiong, J., et al. (2023). Biomechanics research on laterality effect between dominant and non-dominant during double roundhouse kick in the competitive taekwondo. Heliyon 9 (10), e20843. doi:10.1016/j.heliyon.2023.e20843
Machado, S. M., Osório, R. A. L., Silva, N. S., and Magini, M. (2010). Biomechanical analysis of the muscular power of martial arts athletes. Med. Biol. Eng. Ccomputing 48, 573–577. doi:10.1007/s11517-010-0608-z
Moreira, P., and Paula, L. (2017). Kinesiologic description of the round house kick: a brief review. J. Athl. Enhanc. 6 (1), 1–6. doi:10.4172/2324-9080.1000250
Nan, Z., and Jian, M. G. (2017). Technical and tactical characteristics of taekwondo men's 58kg champion zhao shuai in 2016 Rio Olympics. J. Beijing Sport Univ. 40 (2), 95–99. doi:10.19582/j.cnki.11-3785/g8.2017.02.015
Nan, Z., Wei, J. L., Jian, B. Z., and Hai, L. Y. (2017). The competition pattern and development trend of taekwondo in the last three Olympic Games. J. Shandong Sport Univ. 33 (4), 92–96. doi:10.14104/j.cnki.1006-2076.2017.04.017
Ojeda-Aravena, A., Herrera-Valenzuela, T., Valdés-Badilla, P., Cancino-López, J., Zapata-Bastias, J., and García-García, J. M. (2021). Inter-Individual variability of a high-intensity interval training with specific techniques vs. repeated sprints program in sport-related fitness of taekwondo athletes. Front. Physiology 12, 766153. doi:10.3389/fphys.2021.766153
Pei, F. C., and Jian, J. S. (2021). Kinematic feature analysis of the risk of acute injury of the lateral collateral ligament caused by whip leg. J. Xi'an Phys. Educ. Univ. 38 (2), 219–225. doi:10.16063/j.cnki.issn1001-747x.2021.02.014
Pieter, W., Fife, G. P., and O'Sullivan, D. M. (2012). Competition injuries in taekwondo: a literature review and suggestions for prevention and surveillance. Br. J. Sports Med. 46 (7), 485–491. doi:10.1136/bjsports-2012-091011
Qing, C. Z., Zhi, H. G., Shan, L., and Yu, H. C. (2014). Scoring characters of referees in world qualifying trials for 2012 London Olympics. China Sport Sci. Technol. 50 (3), 65–68. doi:10.3969/j.issn.1002-9826.2014.03.011
Qiu, S. Z., Ben, Y. F., and Yu, Y. Z. (2021). On the correlations among scoring factors in world competitive trampoline. J. Xi'an Phys. Educ. Univ. 38 (3), 379–384. doi:10.16063/j.cnki.issn1001-747x.2021.03.018
Quinzi, F., Camomilla, V., Felici, F., DI Mario, A., and Sbriccoli, P. (2013). Differences in neuromuscular control between impact and no impact roundhouse kick in athletes of different skill levels. J. Electromyogr. Kinesiol. 23 (1), 140–150. doi:10.1016/j.jelekin.2012.09.006
Razman, R., and Chong, R. W. L. (2019). Reliability and validity of a taekwondo electronic body protector. J. Sports Eng. Technol. 233 (2), 202–209. doi:10.1177/1754337118815
Sant'Ana, J., Franchini, E., Da Silva, V., and Diefenthaeler, F. (2017). Effect of fatigue on reaction time, response time, performance time, and kick impact in taekwondo roundhouse kick. Sports Biomech. 16 (2), 201–209. doi:10.1080/14763141.2016.1217347
Sant'Ana, J., Sakugawa, R. L., and Diefenthaeler, F. (2021). The effect of a pace training session on internal load and neuromuscular parameters in taekwondo athletes. Front. Physiology 12, 710627. doi:10.3389/fphys.2021.710627
Shi, M. L., Yi, F. B., and Yu, P. Q. (2012). Biomechanical analysis on principles of lower limb whiplash movement. China Sport Sci. Technol. 48 (4), 101–107+136. doi:10.3969/j.issn.1002-9826.2012.04.015
Son, B., Cho, Y. J., Jeong, H. S., and Lee, S. Y. (2020). Injuries in Korean elite taekwondo athletes: a prospective study. Int. J. Environ. Res. Public Health 17 (14), 5143. doi:10.3390/ijerph17145143
Tomasevicz, C. L., Ransone, J. W., and Bach, C. W. (2020). Predicting bobsled pushing ability from various combine testing events. J. Strength & Cond. Res. 34 (9), 2618–2626. doi:10.1519/JSC.0000000000002489
Warmenhoven, J., Bargary, N., Liebl, D., Harrison, A., Robinson, M. A., Gunning, E., et al. (2021). PCA of waveforms and functional PCA: a primer for biomechanics. J. Biomechanics 116, 110106. doi:10.1016/j.jbiomech.2020.110106
Winter, E. M., Eston, R. G., and Lamb, K. L. (2001). Statistical analyses in the physiology of exercise and kinanthropometry. J. Sports Sci. 19 (10), 761–775. doi:10.1080/026404101317015429
Yi, J., Zhi, L., and Dian, K. C. (2018). Influence of different sole thickness on biomechanical parameters of human lower extremity. J. Xi'an Phys. Educ. Univ. 35 (6), 731–741. doi:10.16063/j.cnki.issn1001-747x.2018.06.015
Ying, H., Hai, J. D., and Zhong, Q. J. (2011). Biomechanics research on the offensive back turning kick in long distance of elite tae kwon do players. J. Beijing Sport Univ. 34 (4), 64–67. doi:10.19582/j.cnki.11-3785/g8.2011.04.017
Yu, L., and Ji, H. Z. (2020). Kinematics analysis on different positions' serve skills in world elite male tennis players. China Sport Sci. 40 (8), 58–64. doi:10.16469/j.css.202008006
Zhao, L. L., and Jia, Y. Z. (2022). Characteristics of extensor group of lower limbs in drop jumping. J. Xi'an Phys. Educ. Univ. 39 (2), 224–231. doi:10.16063/j.cnki.issn1001-747x.2022.02.012
Zhi, H. G., Ju, T. F., Wen, G. R., and Zhi, M. Q. (2010). The effects of new rule and electronic protective gear application on taekwondo technique. China Sport Sci. Technol. 46 (4), 86–89+98. doi:10.3969/j.issn.1002-9826.2010.04.014
Keywords: Taekwondo, electronic protective gear, front roundhouse kick, biomechanics, effective scores
Citation: Jia M, Ma Y, Huang R, Liu L, Wang Z, Lin S, Peng Q, Xiong J and Zheng W (2024) Correlation analysis between biomechanical characteristics of lower extremities during front roundhouse kick in Taekwondo and effective scores of electronic protectors. Front. Bioeng. Biotechnol. 12:1364095. doi: 10.3389/fbioe.2024.1364095
Received: 01 January 2024; Accepted: 06 June 2024;
Published: 21 June 2024.
Edited by:
Michael Döllinger, University Hospital Erlangen, GermanyReviewed by:
Fajar Awang Irawan, State University of Semarang, IndonesiaCopyright © 2024 Jia, Ma, Huang, Liu, Wang, Lin, Peng, Xiong and Zheng. This is an open-access article distributed under the terms of the Creative Commons Attribution License (CC BY). The use, distribution or reproduction in other forums is permitted, provided the original author(s) and the copyright owner(s) are credited and that the original publication in this journal is cited, in accordance with accepted academic practice. No use, distribution or reproduction is permitted which does not comply with these terms.
*Correspondence: Yong Ma, bWF5b25nQHdoc3UuZWR1LmNu
Disclaimer: All claims expressed in this article are solely those of the authors and do not necessarily represent those of their affiliated organizations, or those of the publisher, the editors and the reviewers. Any product that may be evaluated in this article or claim that may be made by its manufacturer is not guaranteed or endorsed by the publisher.
Research integrity at Frontiers
Learn more about the work of our research integrity team to safeguard the quality of each article we publish.