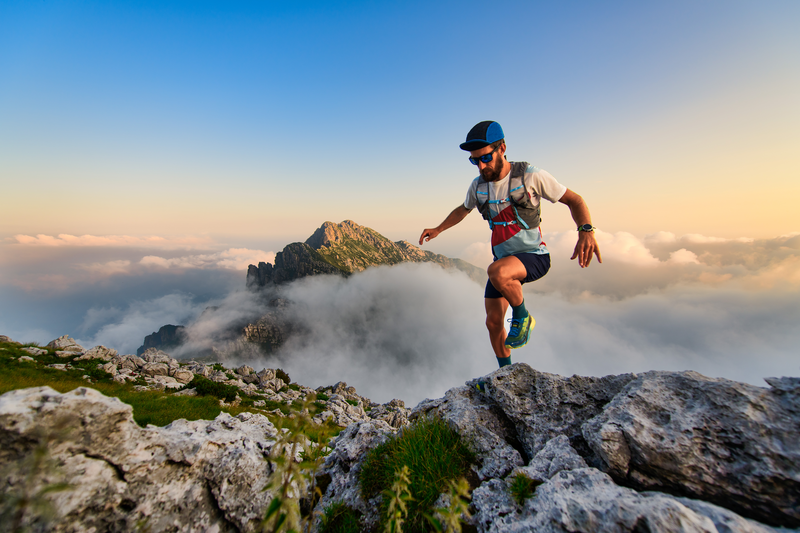
94% of researchers rate our articles as excellent or good
Learn more about the work of our research integrity team to safeguard the quality of each article we publish.
Find out more
MINI REVIEW article
Front. Bioeng. Biotechnol. , 23 January 2024
Sec. Biomaterials
Volume 12 - 2024 | https://doi.org/10.3389/fbioe.2024.1348856
This article is part of the Research Topic Biomedical Biodegradable Matrices and Composites View all 5 articles
Chronic wound management is an intractable medical and social problem, affecting the health of millions worldwide. Decellularized extracellular matrix (dECM)-based materials possess remarkable biological properties for tissue regeneration, which have been used as commercial products for skin regeneration in clinics. However, the complex external environment and the longer chronic wound-healing process hinder the application of pure dECM materials. dECM-based composite materials are constructed to promote the healing process of different wounds, showing noteworthy functions, such as anti-microbial activity and suitable degradability. Moreover, fabrication technologies for designing wound dressings with various forms have expanded the application of dECM-based composite materials. This review provides a summary of the recent fabrication technologies for building dECM-based composite materials, highlighting advances in dECM-based molded hydrogels, electrospun fibers, and bio-printed scaffolds in managing wounds. The associated challenges and prospects in the clinical application of dECM-based composite materials for wound healing are finally discussed.
Skin is the largest organ that acts as a protective barrier from the complex external environment (Li et al., 2022a). The health of the skin is often affected by various thermal, mechanical, and chemical hazards. Accordingly, wound management is a problem that humankind must face (He et al., 2023). Although the body possesses wound-healing ability by self-regulation with multiple cells, the wound-healing process is significantly affected by numerous factors (Cui et al., 2022). Bacterial infection, inflammation, and insufficient wound management may induce chronic wound infection, which frequently occurs in diabetes, cardiovascular disorders, and elderly patients (Las Heras et al., 2020; Xu et al., 2022b; Duan et al., 2023). Besides the loss of skin functionality in massive trauma, the scar formation also brought psychological and social challenges to patients (Fernandes et al., 2022).
Allogenic/xenogeneic tissue or organs have been used in clinical organ transplantation and skin tissue regeneration. However, the adverse immune rejection response induced by antigens of tissue or organs significantly increased the possibility of failure in organ transplantation (Wong et al., 2021). Fortunately, researchers have developed various decellularization methods to obtained decellularized extracellular matrix (dECM), including physical methods (freeze-thaw cycling and pressurization), chemical methods (bases and acids, detergents), and enzymes mediated biological methods (Golebiowska et al., 2024). During the decellularization process, the immunogenic cellular components of native tissue are removed, whereas the extracellular biomacromolecules and other tissue-specific bioactive molecules in extracellular matrix (ECM) are mostly preserved (Chen et al., 2023a; Xu et al., 2023). dECM could accelerate the wound healing process and enhance tissue repair by multiple cellular processes, including in motivating cell growth and proliferation, promoting re-epithelization and angiogenesis, and inhibiting inflammation (Wang et al., 2021). Decellularized extracellular matrix (dECM) based biomedical products (such as AlloDerm® and Oasis®) have been used in the clinic as a promising substitute for regular wound dressings (Mostow et al., 2005; Callcut et al., 2006).
Although dECM tended to form hydrogels under 37°C to mimic tissue microenvironment, the unsuitable physicochemical properties and fast degradation rate of dECM still hinder the application (Chen et al., 2023b). The combination of natural and synthetic polymers with dECM could improve the mechanical properties, which has been used for promoting tissue regeneration (Zhang et al., 2022; Shen et al., 2023; Shi et al., 2023). Due to the risk of infections, developing antibacterial and effective dECM-based biomaterials for promoting wound healing is urgently needed. dECM hybrid scaffolds such as nanofiber films, sponges, hydrogels, and three-dimensional (3D) meshes with outstanding performance have been continuously constructed for tissue regeneration. The multiple approaches, including electrospinning, molding, and 3D printing, significantly expand the application of dECM-composite biomaterials.
In this review, we focused on the combination of dECM-derived biomaterials with drugs or other biomaterials for skin tissue engineering. More specifically, we discussed the fabrication techniques for constructing dECM-composite scaffolds that are suitable for wound healing. Finally, we highlight the contemporary challenges and prospects of dECM-composite biomaterials in skin regeneration applications (Figure 1). This review aims to provide the comprehension of dECM-based composite biomaterials, guiding the researchers to choose suitable fabrication technologies and ultimately promoting the clinical translation of biomaterial in skin tissue engineering.
As mentioned, dECM-based composites have exhibited numerous outstanding outcomes in promoting skin regeneration. Therefore, it is crucial to build composite materials with appropriate morphologic forms, such as nanofiber, films, hydrogel, and 3D meshes, to manage different types of wounds. In this section, we have categorized recent literature based on fabrication technologies (including molding, electrospinning, and 3D printing) to build dECM-based composite wound dressing.
After decellularization, solubilized dECM powder could be manipulated to form a hydrogel using molding process after adjusting the suitable pH and physiological temperature, which may be based on the thermal cross-linking of collagen-induced self-assembly (Pati et al., 2014). Due to their excellent bioactivity, injectable feasibility, and thermal-sensitive properties, dECM-based hydrogels have shown attractive advantages in tissue regeneration (Zhang et al., 2021b). However, their rapid degradation and weak mechanical properties still pose a major challenge. Designing a dECM-based hydrogel system with suitable physical and chemical properties is required for expanding its application in the tissue regeneration field. For example, Xu et al. mixed gelatin, chitosan, and dECM to form composite hydrogel (dECM/Gel/CS), showing suitable compressive elastic modulus (≥482.17 kPa), excellent hydrophilicity, and appropriate degradability (Xu et al., 2021). The dECM/Gel/CS composite showed exceptional biocompatibility and stable antibacterial activity on Escherichia coli and Staphylococcus aureus. In another work, Zhang et al. successfully fabricated a photo-cross-linkable hydrogel based on dECM-methacrylate (dECM-MA) and gelatin methacryloyl (GelMA) as skin scaffolds (Zhang et al., 2021a). The mechanical strength and water absorption of dECM-MA/GelMA scaffolds were significantly improved compared to the GelMA and dECM groups. Moreover, immunohistochemical results of the wound area showed that the dECM-MA/GelMA group facilitated collagen deposition and promoted angiogenesis and re-epithelialization.
To avoid the secondary injuries of changing wound dressing, researchers have designed dECM-based hydrogel with appropriate adhesion for wound treatment. Wang et al. designed a composite hydrogel system that combines with o-nitrobenzene-modified gelatin-coated dECM to enhance the tissue adhesive property (Wang et al., 2023a). The composite scaffolds significantly accelerated the epidermal regeneration in the wound model by promoting angiogenesis and collagen fiber reformation. Likely, Bo et al. fabricated adipose-derived stem cells loaded hydrogel system made from a combination of dECM and o-nitrobenzene-modified hyaluronic acid (Bo et al., 2020). The photo-cross-linkable composite hydrogel exhibited good skin adhesive activity, which could apply to different kinds of skin defects with irregular forms. Moreover, the cells-loaded hybrid system significantly accelerated the wound closure rate, which was achieved by enhancing collagen deposition, promoting re-epithelialization, and promoting angiogenesis.
Besides improving the mechanical properties of dECM-based hydrogel wound dressing, numerous functional molecular or bioactive substances were combined with dECM to satisfy different wound microenvironments. For instance, Liu et al. developed a bioactive composite hydrogel by mixing dECM, asiaticoside-loaded polydopamine nanoparticles, and GelMA for wound healing (Liu et al., 2023). Compared with other groups, composite hydrogels exhibited higher regenerated hair follicle numbers, revealing the ability to promote scarless wound healing. Tang et al. combined dECM hydrogel with platelet-rich plasma derived from the human body to promote wound healing (Tang et al., 2022). The migration and tube formation assays on human umbilical vein endothelial cells (HUVECs) showed that the hydrogel could promote angiogenesis, and the immunofluorescence staining and polymerase chain reaction (PCR) analysis of RAW 264.7 cells indicated the capability of M2 macrophage polarization promotion. Moreover, the wound repair experiment of nude mice and porcine showed that hydrogel could significantly promote skin regeneration. Recently, Song et al. prepared adipose-derived mesenchymal stem cell-derived exosomes encapsulated dECM hydrogels (ECM@exo) for skin regeneration (Song et al., 2023). Culturing with human immortalized keratinocyte cells (HaCaT) and HUVECs, ECM@exo significantly enhanced cell proliferation, migration, and angiogenesis. In vivo evaluations investigated in normal and diabetic wound models showed that the ECM@exo could reduce the inflammatory factor (tumor necrosis factor-α (TNF-α) and interleukin-6 (IL-6)) expression. To controlling the releasement of bioactive factors, Xiao et al. proposed a dECM-MA hydrogel for the spatiotemporal delivery of macrophage-associated cytokine (Xiao et al., 2023). In detail, the dECM-MA hydrogel encapsulated pro-healing cytokines acted as the core, whereas the pro-inflammatory cytokines-loaded dECM-MA hydrogel was the sheath portion. Compared with single cytokines therapy, the spatiotemporal dual cytokines release hydrogel remarkably improved cell proliferation, increased the cell migratory rate, and induced higher collagen III/I expression in fibroblasts. Moreover, the hydrogel exhibited higher angiogenic effects in vitro and also accelerated skin reconstruction with high quality.
Nanofibers or microfibers that are produced by electrospinning have emerged as potential biomedical materials for wound management (Memic et al., 2019). This electrospinning fibers-based wound dressing with large specific surfaces could fit different kinds of skin, providing a suitable environment for cell adhesion and growth (Wang et al., 2023b). The dECM-based electrospinning fibers have shown potential in skin repair due to the penetrating network structure that could mimic the ECM structure of natural skin. In contrast, dECM materials provide biochemical for tissue regeneration. By controlling the electrospun solution properties and process parameters, electrospun fibers combined with polymers and dECM have been utilized in tissue engineering applications (Krishtul et al., 2020; Li et al., 2022b).
Typically, dECM and polymers were dissolved in a suitable solution to fabricate composite electrospun fibers. For instance, Kim et al. prepared nanofibers of poly-D,L-lactide-co-glycolide (PLGA) and dECM with different concentrations (0.25%, 0.5% and 1%) (Kim et al., 2017). The increase in the dECM ratio significantly enhanced the mechanical strength of electrospun fibers, promoting the proliferation of rat granulation fibroblasts. Similarly, Kim et al. prepared nanofibrous electrospun hybrid scaffolds using heart dECM and poly (l-lactide-co-caprolactone) (PLCL) (Kim et al., 2018) as wound dressing materials. Compared with the gelatin-PLCL group, the prepared nanofibers effectively promoted angiogenesis and vessel maturation in vitro, accelerating wound healing by minimizing inflammation, promoting angiogenesis, and reducing scar in vivo. In addition, Gao et al. combined electrospinning with gas foaming techniques to fabricate 3D nanofiber scaffolds based on polycaprolactone (PCL) and dECM and loaded with ε-polylysine (Gao et al., 2023). The 3D nanofiber scaffolds with layer-like structures exhibited excellent thermal stability, hydrophilicity, and outstanding antibacterial activity. Moreover, in vivo results demonstrated that the nanofibers were suitable to accelerate wound healing by promoting CD31 expression and decreasing TNF-α expression.
Bioactive molecules, such as antibacterial drugs or particles, were also used in dECM-based nanofiber systems. For example, Chandika et al. fabricated usnic acid-enriched dECM-PCL nanofibrous scaffold for promoting wound healing (Chandika et al., 2022). Furthermore, the nanofiber scaffolds showed desirable anti-microbial properties and biofilm inhibition activities activity, indicating long-term usnic acid releasement for 14 days. In addition, Zhan et al. designed a dECM/PLCL multifunctional nanofibers platform loaded with copper and coated with chitosan (CTS@PLCL/DWJM@Cu) via a coaxial electrospinning approach (Zhan et al., 2022). The core-shell nanofiber exhibited initial releasement of chitosan for exerting early antibacterial activity, presenting sustained release copper to promote neovascularization. The core-shell nanofiber showed satisfactory mechanical properties, suitable hydrophilicity and protein absorption, and excellent inhibition efficacy of both S. aureus and E. coli. Overall, CTS@PLCL/DWJM@Cu significantly inhibited bacterial growth, promoted neovascularization, and created a microenvironment for collagen deposition, further promoting the wound healing of diabetic wounds.
Besides mixing dECM with polymer as an electrospinning solution, dECM composite materials with different electrospinning forms have been developed. For example, Tang et al. prepared PLGA nanofiber that cultured human adipose-derived stem cells (hADSCs) and then decellularized them to obtain dECM-PLGA meshes, promoting the wound healing process in vitro (Tang et al., 2019). In another investigation, Lee et al. developed human dermal fibroblasts loaded with electrospun PCL fibers to obtain a 3D multi-layered fibrous scaffold (Lee et al., 2016). In detail, the cell was cultured on PCL fiber for 4 days. After decellularization, the freeze-milling dECM-PCL powder was used to fabricate a fibrous scaffold by the electrohydrodynamic jet process. The SEM images showed that all scaffolds with micro-fibrous bundles dECM-PCL based scaffolds showed higher tensile modulus and faster water absorption rate than pure PCL-based scaffolds. Gholipourmalekabadi et al. fabricated bi-layer membranes by using human amniotic membrane dECM and silk fibroin nanofiber as 3D artificial skin (Gholipourmalekabadi et al., 2018). After being cultured with ADSCs for 7 days, the composite membranes significantly increased the expression of pro-angiogenesis factors compared with pure dECM.
3D printing has gained increasing attention in tissue regeneration due to the ability to prepare scaffolds with suitable 3D morphologies and structures by using computer-aided design (Wang et al., 2022b). 3D printing is a viable option to create suitable structures for matching the inhomogeneous wound site, which also mimics native skin tissue by combining biomaterials with cells (Tanfani et al., 2023). Due to the printability of dECM, researchers constructed different types of bioengineered 3D structures based on dECM, showing translational potential for promoting tissue repair (Hwang et al., 2022). However, the low viscosity and long gelation time may result in the poor shape fidelity of dECM hydrogel-based 3D structure (Yeleswarapu et al., 2023).
The addition of polymers in dECM-based bio-ink or mixing the dECM with other kinds of bio-ink has been employed to enhance the mechanical properties of the printed structure. Jorgensen et al. provided a hybrid bioink by combining fibrinogen and dECM for extrusion-based bioprinting (Jorgensen et al., 2020). Compared with the dECM hydrogel, the combination of fibrinogen demonstrated higher storage modulus and viscosity at low temperatures, which showed shear thinning properties and longer structural stability. Bashiri et al. prepared a bioink based on alginate/gelatin that loaded different human placental dECM concentrations (Bashiri et al., 2023). The addition of dECM could significantly improve the compressive strength and tensile strength of the 3D printing scaffold. Compared with the alginate/gelatin scaffold, the 3D printed scaffolds with 5% dECM significantly enhanced wound healing and pro-angiogenic gene expression. Fu et al. exploited a 3D-printed dECM-GelMA-HAMA scaffold that incorporated hADSCs as a skin substitute (Fu et al., 2023). The integrated 3D printed scaffold significantly promoted wound healing in vivo, relying on promoting angiogenesis, re-epithelialization, and collagen deposition, and inhibiting inflammatory response.
Additionally, there is an urgent need to design dECM-based biomaterial that inhibits bacterial infection for wound healing. Likewise, Xu et al. prepared a hybrid 3D printed tissue engineering scaffold composed of gelatin, quaternized chitosan, and dECM assembled with poly (ionic liquid)s (Xu et al., 2022a). By assembling poly (ionic liquid)s into the scaffold, the composite platform displayed a strong Gram-negative and Gram-positive bacteria inhibition rate, effectively preventing bacterial infection for a long time. Moreover, the composite scaffold demonstrated excellent hemostatic effect and hemocompatibility, thus showing an essential potential in skin engineering. Hu et al. reported a multifunctional dECM-MA-based 3D printing hydrogel scaffold embedded with copper epigallocatechin gallate nano-capsules for diabetic wound management (Hu et al., 2023). The cellular experiments indicated that the multifunctional 3D platform showed favorable anti-inflammatory capacity and good biocompatibility, which could accelerate vascularization and promote cell migration and invasion. In vivo experiments of the diabetic split-thickness skin graft model demonstrated that compared with commercial dECM groups, 3D-printed scaffolds significantly decreased the size of wound area with no obvious contracture. Moreover, 3D-printed scaffolds showed a similar ratio of collagen I/III in dermis and skin thickness to native tissue, indicating the potential tissue regeneration ability with non-scar in diabetic wound. In another work, Lin et al. prepared sodium alginate and dECM-MA as bio-ink to load with the curcumin and basic fibroblast growth factor (bFGF) for a 3D scaffold construction (Lin et al., 2023). The bFGF in scaffolds could significantly promote neovascularization, and the curcumin could inhibit bacterial growth, indicating a promising way to promote the bacterial infection wound for potential clinic applications. Nanoparticles with unique properties have been used in 3D structure construction, which may reinforce the mechanical and physicochemical properties of hydrogels or introduce the new biological functions of bio-ink (Chakraborty et al., 2021). Hu et al. fabricated 3D scaffolds by mixing dECM with mesoporous bioactive glass and exosomes as bioink to through extrusion-based printing technology (Hu et al., 2021). The composite hydrogel scaffolds showed a sustained release of exosomes for 14 days, and the addition of bioactive glass significantly promoted the cell proliferation, adhesion, angiogenesis. Moreover, the 3D wound dressing not only showed rapid hemostatic ability but also shortened wound closure rate and enhanced angiogenesis in diabetic wounds.
In nature, skin consists of epidermis, dermis, and hypodermis in order; relative cells were interspersed in ECM that supported the mechanical properties and resilience of skin (Daikuara et al., 2022). To simulate natural full-thickness skin with the epidermal and dermal compartment, Ahn et al. used a 3D bioprinting technology by sacrificial gelatin-assisted to build a full-thickness skin model, which was constructed by dECM-loaded human dermal fibroblast as dermis and sacrificial gelatin-loaded human epidermal keratinocyte as epidermal layer (Ahn et al., 2023). In another work, Jin et al. designed an artificial skin with a 3D structure, which was constructed by 20% GelMA as an epidermal layer, 1.5% porcine skin dECM as a dermis layer, and 10% GelMA as a vascular network (Jin et al., 2021). The in vitro results showed that the 3D-printing skin model provided a suitable microenvironment for cell proliferation and also supported epidermis reconstruction. In addition, the full-thickness wound test revealed that the 3D-printing skin substitute significantly enhanced the wound-healing process and promoted the synthesis of collagen III while inhibiting the excessive proliferation of collagen I.
Wound management remains a serious challenge burden on healthcare systems nowadays. Due to the limitations of dECM-based biomaterials alone in the wound healing process, the strategy of combining polymers or drug agents with dECM significantly enhanced the physicochemical property, showing great potential in skin regeneration. Moreover, the emergence of fabrication techniques and the breakthrough of apparatuses may provide a great way for dECM-based composite wound dressing in successful clinic translation. In this review, we have summarized the recent developments of dECM-based composite materials in wound healing, highlighting the significance of the fabrication method in the design and bio-function of those biomaterials.
As a promising field, some challenges remain in developing dECM-based composite wound dressing for clinic application. Various types of functional components with different mechanisms have been united with dECM-based biomaterials for promoting wound healing. However, due to the dynamic changes of the wound healing process, it is difficult to meet the requirements of the entire skin regeneration process. Moreover, the wound microenvironment is rather complicated and involves immune relation cells, pH, oxygen, enzymes, and microbiology, among others. Therefore, developing dECM-based wound dressing could respond to the wound microenvironment, and precise delivery of the bioactive agents on demand is a further direction.
Although dECM-based composite biomaterials with different forms are produced by various fabrication technologies as alternatives to skin grafts, skin is a complex organ that is composed of epidermal, dermal, and hypodermal, which contain different cell types and density, ECM composition, and microstructure. Fabrication of tissue-engineered skin substitutes with 3D micro-structure and the combination of relative cells are still challenging. Therefore, the integration of various fabrication technologies in building skin substitutes is promising. The cooperation of clinicians, scientists, and mechanical engineers may move the dECM-based biomaterial forward in the future.
PX: Writing–original draft. JC: Writing–original draft. YD: Writing–original draft. RK: Writing–review and editing. AC: Writing–review and editing.
The author(s) declare financial support was received for the research, authorship, and/or publication of this article. The authors thank the financial support from the National Natural Science Foundation of China (NSFC 32271410, 32071323, and 81971734), the Science and Technology Projects in Fujian Province (2022FX1, 2023Y4008), Scientific Research Funds of Huaqiao University (21BS113), and the Open Research Fund of Academy of Advanced Carbon Conversion Technology, Huaqiao University (AACCT0004).
The authors declare that the research was conducted in the absence of any commercial or financial relationships that could be construed as a potential conflict of interest.
All claims expressed in this article are solely those of the authors and do not necessarily represent those of their affiliated organizations, or those of the publisher, the editors and the reviewers. Any product that may be evaluated in this article, or claim that may be made by its manufacturer, is not guaranteed or endorsed by the publisher.
Ahn, M., Cho, W.-W., Lee, H., Park, W., Lee, S.-H., Back, J. W., et al. (2023). Engineering of uniform epidermal layers via sacrificial gelatin bioink-assisted 3D extrusion bioprinting of skin. Adv. Healthc. Mater 12 (27), 2301015. doi:10.1002/adhm.202301015
Bashiri, Z., Rajabi Fomeshi, M., Ghasemi Hamidabadi, H., Jafari, D., Alizadeh, S., Nazm Bojnordi, M., et al. (2023). 3D-printed placental-derived bioinks for skin tissue regeneration with improved angiogenesis and wound healing properties. Mater. Today Bio. 20, 100666. doi:10.1016/j.mtbio.2023.100666
Bo, Q., Yan, L., Li, H., Jia, Z., Zhan, A., Chen, J., et al. (2020). Decellularized dermal matrix-based photo-crosslinking hydrogels as a platform for delivery of adipose derived stem cells to accelerate cutaneous wound healing. Mater. Des. 196, 109152. doi:10.1016/j.matdes.2020.109152
Callcut, R. A., Schurr, M. J., Sloan, M., and Faucher, L. D. (2006). Clinical experience with Alloderm: a one-staged composite dermal/epidermal replacement utilizing processed cadaver dermis and thin autografts. Burns 32 (5), 583–588. doi:10.1016/j.burns.2005.12.002
Chakraborty, A., Roy, A., Ravi, S. P., and Paul, A. (2021). Exploiting the role of nanoparticles for use in hydrogel-based bioprinting applications: concept, design, and recent advances. Biomater. Sci. 9 (19), 6337–6354. doi:10.1039/d1bm00605c
Chandika, P., Khan, F., Heo, S.-Y., Kim, Y.-M., Yi, M., and Jung, W.-K. (2022). Enhanced wound-healing capability with inherent antimicrobial activities of usnic acid incorporated poly(ε-caprolactone)/decellularized extracellular matrix nanofibrous scaffold. Biomater. Adv. 140, 213046. doi:10.1016/j.bioadv.2022.213046
Chen, Y., Chen, L. F., Wang, Y., Luo, S. C., Zhang, J. T., Kankala, R. K., et al. (2023a). Modeling dECM-based inflammatory cartilage microtissues in vitro for drug screening. Compos. Part B. 250, 110437. doi:10.1016/j.compositesb.2022.110437
Chen, Y. W., Lin, Y. H., Lin, T. L., Lee, K. X. A., Yu, M. H., and Shie, M. Y. (2023b). 3D-biofabricated chondrocyte-laden decellularized extracellular matrix-contained gelatin methacrylate auxetic scaffolds under cyclic tensile stimulation for cartilage regeneration. Biofabrication 15 (4), 045007. doi:10.1088/1758-5090/ace5e1
Cui, T., Yu, J., Wang, C.-F., Chen, S., Li, Q., Guo, K., et al. (2022). Micro-gel ensembles for accelerated healing of chronic w'ound via pH regulation. Adv. Sci. 9 (22), 2201254. doi:10.1002/advs.202201254
Daikuara, L. Y., Chen, X. F., Yue, Z. L., Skropeta, D., Wood, F. M., Fear, M. W., et al. (2022). 3D bioprinting constructs to facilitate skin regeneration. Adv. Funct. Mater 32 (3), 2105080. doi:10.1002/adfm.202105080
Duan, Y. Y., Xu, P. Y., Ge, P. Y., Chen, L. F., Chen, Y., Kankala, R. K., et al. (2023). NIR-responsive carrier-free nanoparticles based on berberine hydrochloride and indocyanine green for synergistic antibacterial therapy and promoting infected wound healing. Regen. Biomater. 10, rbad076. doi:10.1093/rb/rbad076
Fernandes, M. G., Silva, L. P. D., Cerqueira, M. T., Ibanez, R., Murphy, C. M., Reis, R. L., et al. (2022). Mechanomodulatory biomaterials prospects in scar prevention and treatment. Acta Biomater. 150, 22–33. doi:10.1016/j.actbio.2022.07.042
Fu, H. J., Zhang, D. Q., Zeng, J. S., Fu, Q., Chen, Z. Y., Sun, X. R., et al. (2023). Application of 3D-printed tissue-engineered skin substitute using innovative biomaterial loaded with human adipose-derived stem cells in wound healing. Int. J. Bioprint. 9 (2), 674–406. doi:10.18063/ijb.v9i2.674
Gao, X. F., Jiang, G. H., Ruan, L. M., Sun, Y. F., Yunusov, K. E., Jing, Y. T., et al. (2023). Electrospun porcine acellular dermal matrix and polycaprolactone composite nanofibrous scaffolds for accelerating wound healing. Fibers Polym. 24 (2), 589–601. doi:10.1007/s12221-023-00077-z
Gholipourmalekabadi, M., Samadikuchaksaraei, A., Seifalian, A. M., Urbanska, A. M., Ghanbarian, H., Hardy, J. G., et al. (2018). Silk fibroin/amniotic membrane 3D bi-layered artificial skin. Biomed. Mater. 13 (3), 035003. doi:10.1088/1748-605X/aa999b
Golebiowska, A. A., Intravaia, J. T., Sathe, V. M., Kumbar, S. G., and Nukavarapu, S. P. (2024). Decellularized extracellular matrix biomaterials for regenerative therapies: advances, challenges and clinical prospects. Bioact. Mater. 32, 98–123. doi:10.1016/j.bioactmat.2023.09.017
He, L., Di, D., Chu, X., Liu, X., Wang, Z., Lu, J., et al. (2023). Photothermal antibacterial materials to promote wound healing. J. Control. Release 363, 180–200. doi:10.1016/j.jconrel.2023.09.035
Hu, Y., Wu, B., Xiong, Y., Tao, R., Panayi, A. C., Chen, L., et al. (2021). Cryogenic 3D printed hydrogel scaffolds loading exosomes accelerate diabetic wound healing. Chem. Eng. J. 426, 130634. doi:10.1016/j.cej.2021.130634
Hu, Y., Xiong, Y., Zhu, Y., Zhou, F., Liu, X., Chen, S., et al. (2023). Copper-Epigallocatechin gallate enhances therapeutic effects of 3D-printed dermal scaffolds in mitigating diabetic wound scarring. ACS Appl. Mater Interfaces 15 (32), 38230–38246. doi:10.1021/acsami.3c04733
Hwang, D. G., Jo, Y., Kim, M., Yong, U., Cho, S., Choi, Y. M., et al. (2022). A 3D bioprinted hybrid encapsulation system for delivery of human pluripotent stem cell-derived pancreatic islet-like aggregates. Biofabrication 14 (1), 014101. doi:10.1088/1758-5090/ac23ac
Jin, R., Cui, Y., Chen, H., Zhang, Z., Weng, T., Xia, S., et al. (2021). Three-dimensional bioprinting of a full-thickness functional skin model using acellular dermal matrix and gelatin methacrylamide bioink. Acta Biomater. 131, 248–261. doi:10.1016/j.actbio.2021.07.012
Jorgensen, A. M., Chou, Z. S., Gillispie, G., Lee, S. J., Yoo, J. J., Soker, S., et al. (2020). Decellularized skin extracellular matrix (dsECM) improves the physical and biological properties of fibrinogen hydrogel for skin bioprinting applications. Nanomaterials 10 (8), 1484. doi:10.3390/nano10081484
Kim, K., Lee, J. Y., Kim, H., Shin, J., Shin, Y., Yoo, Y. T., et al. (2017). Fabrication of electropsun PLGA and small intestine submucosa-blended nanofibrous membranes and their biocompatibility for wound healing. Fibers Polym. 18 (2), 231–239. doi:10.1007/s12221-017-6936-y
Kim, T. H., Jung, Y., and Kim, S. H. (2018). Nanofibrous electrospun heart decellularized extracellular matrix-based hybrid scaffold as wound dressing for reducing scarring in wound healing. Tissue Eng. Part A 24 (9-10), 830–848. doi:10.1089/ten.tea.2017.0318
Krishtul, S., Baruch, L., and Machluf, M. (2020). Processed tissue-derived extracellular matrices: tailored platforms empowering diverse therapeutic applications. Adv. Funct. Mater 30 (18), 1900386. doi:10.1002/adfm.201900386
Las Heras, K., Igartua, M., Santos-Vizcaino, E., and Hernandez, R. M. (2020). Chronic wounds: current status, available strategies and emerging therapeutic solutions. J. Control. Release 328, 532–550. doi:10.1016/j.jconrel.2020.09.039
Lee, H., Yang, S., Kim, M., and Kim, G. (2016). A scaffold with a bio-mimetically designed micro/nano-fibrous structure using decellularized extracellular matrix. RSC Adv. 6 (35), 29697–29706. doi:10.1039/c5ra27845g
Li, R. T., Liu, K., Huang, X., Li, D., Ding, J. X., Liu, B., et al. (2022a). Bioactive materials promote wound healing through modulation of cell behaviors. Adv. Sci. 9 (10), 2105152. doi:10.1002/advs.202105152
Li, S. Y., Deng, R. L., Zou, X. N., Rong, Q., Shou, J. L., Rao, Z. L., et al. (2022b). Development and fabrication of co-axially electrospun biomimetic periosteum with a decellularized periosteal ECM shell/PCL core structure to promote the repair of critical-sized bone defects. Compos. Part B. 234, 109620. doi:10.1016/j.compositesb.2022.109620
Lin, X., Zhang, H., Zhang, H., Zhang, Z., Chen, G., and Zhao, Y. (2023). Bio-printed hydrogel textiles based on fish skin decellularized extracellular matrix for wound healing. Engineering 25, 120–127. doi:10.1016/j.eng.2022.05.022
Liu, S., Zhao, Y. S., Li, M., Nie, L., Wei, Q. Q., Okoro, O. V., et al. (2023). Bioactive wound dressing based on decellularized tendon and GelMA with incorporation of PDA-loaded asiaticoside nanoparticles for scarless wound healing. Chem. Eng. J. 466, 143016. doi:10.1016/j.cej.2023.143016
Memic, A., Abudula, T., Mohammed, H. S., Navare, K. J., Colombani, T., and Bencherif, S. A. (2019). Latest progress in electrospun nanofibers for wound healing applications. ACS Appl. Bio Mater 2 (3), 952–969. doi:10.1021/acsabm.8b00637
Mostow, E. N., Haraway, G. D., Dalsing, M., Hodde, J. P., and King, D. (2005). Effectiveness of an extracellular matrix graft (OASIS Wound Matrix) in the treatment of chronic leg ulcers: a randomized clinical trial. J. Vasc. Surg. 41 (5), 837–843. doi:10.1016/j.jvs.2005.01.042
Pati, F., Jang, J., Ha, D. H., Kim, S. W., Rhie, J. W., Shim, J. H., et al. (2014). Printing three-dimensional tissue analogues with decellularized extracellular matrix bioink. Nat. Commun. 5, 3935. doi:10.1038/ncomms4935
Shen, X., Li, S., Zhao, X., Han, J., Chen, J., Rao, Z., et al. (2023). Dual-crosslinked regenerative hydrogel for sutureless long-term repair of corneal defect. Bioact. Mater. 20, 434–448. doi:10.1016/j.bioactmat.2022.06.006
Shi, J., Teng, Y., Li, D., He, J., Midgley, A. C., Guo, X., et al. (2023). Biomimetic tri-layered small-diameter vascular grafts with decellularized extracellular matrix promoting vascular regeneration and inhibiting thrombosis with the salidroside. Mater. Today bio. 21, 100709. doi:10.1016/j.mtbio.2023.100709
Song, Y. L., You, Y. C., Xu, X. Y., Lu, J. Y., Huang, X. J., Zhang, J. C., et al. (2023). Adipose-derived mesenchymal stem cell-derived exosomes biopotentiated extracellular matrix hydrogels Accelerate diabetic wound healing and skin regeneration. Adv. Sci. 10, 2304023. doi:10.1002/advs.202304023
Tanfani, J. D., Monpara, J. D., and Jonnalagadda, S. (2023). 3D bioprinting and its role in a wound healing renaissance. Adv. Mater. Technol. 8 (17), 21. doi:10.1002/admt.202300411
Tang, J. Z., Li, H. C., Peng, H., Zhang, Z. X., Liu, C. H., Cheng, Y., et al. (2022). Pre-clinical evaluation of thermosensitive decellularized adipose tissue/platelet-rich plasma interpenetrating polymer network hydrogel for wound healing. Mater. Today bio. 17, 100498. doi:10.1016/j.mtbio.2022.100498
Tang, K. C., Yang, K. C., Lin, C. W., Chen, Y. K., Lu, T. Y., Chen, H. Y., et al. (2019). Human adipose-derived stem cell secreted extracellular matrix incorporated into electrospun Poly(Lactic-co-Glycolic Acid)nanofibrous dressing for enhancing wound healing. Polymers 11 (10), 1609. doi:10.3390/polym11101609
Wang, C., Li, G. Y., Cui, K. G., Chai, Z. H., Huang, Z. Y., Liu, Y., et al. (2021). Sulfated glycosaminoglycans in decellularized placenta matrix as critical regulators for cutaneous wound healing. Acta Biomater. 122, 199–210. doi:10.1016/j.actbio.2020.12.055
Wang, L., Liu, F. L., Zhai, X. R., Dong, W., Wei, W., and Hu, Z. H. (2023a). An adhesive gelatin-coated small intestinal submucosa composite hydrogel dressing aids wound healing. Int. J. Biol. Macromol. 241, 124622. doi:10.1016/j.ijbiomac.2023.124622
Wang, S. Q., Zhao, S., Yu, J. C., Gu, Z., and Zhang, Y. Q. (2022b). Advances in translational 3D printing for cartilage, bone, and osteochondral tissue engineering. Small 18 (36), 2201869. doi:10.1002/smll.202201869
Wang, Z. J., Hu, W. K., Wang, W., Xiao, Y., Chen, Y., and Wang, X. H. (2023b). Antibacterial electrospun nanofibrous materials for wound healing. Adv. Fiber Mater 5 (1), 107–129. doi:10.1007/s42765-022-00223-x
Wong, M. L., and Griffiths, L. G. (2014). Immunogenicity in xenogeneic scaffold generation: antigen removal vs. decellularization. Acta Biomater. 10 (5), 1806–1816. doi:10.1016/j.actbio.2014.01.028
Xiao, H. M., Chen, X., Shan, J. Y., Liu, X. Z., Sun, Y., Shen, J. J., et al. (2023). A spatiotemporal release hydrogel based on an M1-to-M2 immunoenvironment for wound management. J. Mat. Chem. B 11 (18), 3994–4004. doi:10.1039/d3tb00463e
Xu, J., Fang, H., Su, Y., Kang, Y., Xu, D., Cheng, Y. Y., et al. (2022a). A 3D bioprinted decellularized extracellular matrix/gelatin/quaternized chitosan scaffold assembling with poly(ionic liquid)s for skin tissue engineering. Int. J. Biol. Macromol. 220, 1253–1266. doi:10.1016/j.ijbiomac.2022.08.149
Xu, J., Fang, H., Zheng, S. S., Li, L. Y., Jiao, Z. R., Wang, H., et al. (2021). A biological functional hybrid scaffold based on decellularized extracellular matrix/gelatin/chitosan with high biocompatibility and antibacterial activity for skin tissue engineering. Int. J. Biol. Macromol. 187, 840–849. doi:10.1016/j.ijbiomac.2021.07.162
Xu, P. Y., Kankala, R. K., Li, Y. W., Wang, S. B., and Chen, A. Z. (2022b). Synergistic chemo-/photothermal therapy based on supercritical technology-assisted chitosan-indocyanine green/luteolin nanocomposites for wound healing. Regen. Biomater. 9, rbac072. doi:10.1093/rb/rbac072
Xu, P. Y., Kankala, R. K., Wang, S., and Chen, A. Z. (2023). Decellularized extracellular matrix-based composite scaffolds for tissue engineering and regenerative medicine. Regen. Biomater. 11, rbad107. doi:10.1093/rb/rbad107
Yeleswarapu, S., Dash, A., Chameettachal, S., and Pati, F. (2023). 3D bioprinting of tissue constructs employing dual crosslinking of decellularized extracellular matrix hydrogel. Biomater. Adv. 152, 213494. doi:10.1016/j.bioadv.2023.213494
Zhan, A., Chen, L., Sun, W., Tang, Y., Chen, J., Yu, D., et al. (2022). Enhancement of diabetic wound healing using a core-shell nanofiber platform with sequential antibacterial, angiogenic, and collagen deposition activities. Mater. Des. 218, 110660. doi:10.1016/j.matdes.2022.110660
Zhang, C. Y., Fu, C. P., Li, X. Y., Lu, X. C., Hu, L. G., Kankala, R. K., et al. (2022). Three-dimensional bioprinting of decellularized extracellular matrix-based bioinks for tissue engineering. Molecules 27 (11), 3442. doi:10.3390/molecules27113442
Zhang, Q., Chang, C. W., Qian, C. Y., Xiao, W. S., Zhu, H. J., Guo, J., et al. (2021a). Photo-crosslinkable amniotic membrane hydrogel for skin defect healing. Acta Biomater. 125, 197–207. doi:10.1016/j.actbio.2021.02.043
Keywords: dECM, skin, wound healing, composite materials, tissue regeneration
Citation: Xu P, Cao J, Duan Y, Kankala RK and Chen A (2024) Recent advances in fabrication of dECM-based composite materials for skin tissue engineering. Front. Bioeng. Biotechnol. 12:1348856. doi: 10.3389/fbioe.2024.1348856
Received: 03 December 2023; Accepted: 15 January 2024;
Published: 23 January 2024.
Edited by:
Xiumei Wang, Tsinghua University, ChinaReviewed by:
Yaobin Wu, Southern Medical University, ChinaCopyright © 2024 Xu, Cao, Duan, Kankala and Chen. This is an open-access article distributed under the terms of the Creative Commons Attribution License (CC BY). The use, distribution or reproduction in other forums is permitted, provided the original author(s) and the copyright owner(s) are credited and that the original publication in this journal is cited, in accordance with accepted academic practice. No use, distribution or reproduction is permitted which does not comply with these terms.
*Correspondence: Aizheng Chen, YXpjaGVuQGhxdS5lZHUuY24=
Disclaimer: All claims expressed in this article are solely those of the authors and do not necessarily represent those of their affiliated organizations, or those of the publisher, the editors and the reviewers. Any product that may be evaluated in this article or claim that may be made by its manufacturer is not guaranteed or endorsed by the publisher.
Research integrity at Frontiers
Learn more about the work of our research integrity team to safeguard the quality of each article we publish.