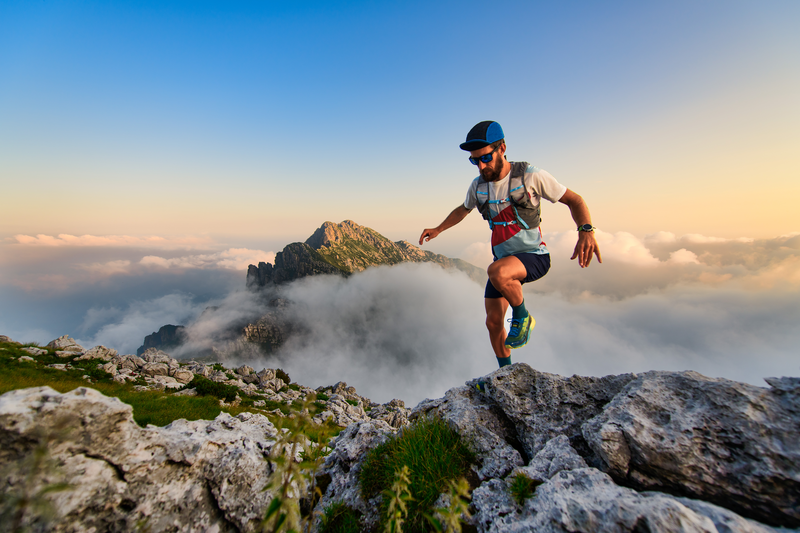
95% of researchers rate our articles as excellent or good
Learn more about the work of our research integrity team to safeguard the quality of each article we publish.
Find out more
BRIEF RESEARCH REPORT article
Front. Bioeng. Biotechnol. , 10 April 2024
Sec. Biomechanics
Volume 12 - 2024 | https://doi.org/10.3389/fbioe.2024.1346365
This article is part of the Research Topic Human Digital Twins for Medical and Product Engineering View all 12 articles
The ‘Timed Up and Go’ test (TUG) is a widely used clinical tool for assessing gait and balance, relying primarily on timing as a measure. However, there are more observable biomechanical compensation strategies within TUG that are indicative of underlying neuromuscular issues and movement priorities. In individuals with unilateral knee osteoarthritis, an increased trunk flexion during TUG is a common phenomenon, often attributed to muscle weakness and/or pain avoidance. Unfortunately, it is difficult to differentiate between these underlying causes using experimental studies alone. This study aimed to distinguish between muscle weakness and pain avoidance as contributing factors, using predictive neuromuscular simulations of the sit-to-walk movement. Muscle weakness was simulated by reducing the maximum isometric force of the vasti muscles (ranging from 20% to 60%), while pain avoidance was integrated as a movement objective, ensuring that peak knee load did not exceed predefined thresholds (2–4 times body weight). The simulations demonstrate that a decrease in muscular capacity led to greater trunk flexion, while pain avoidance led to slower movement speeds and altered muscle recruitments, but not to greater trunk flexion. Our predictive simulations thus indicate that increased trunk flexion is more likely the result of lack of muscular reserve rather than pain avoidance. These findings align with reported differences in kinematics and muscle activations between moderate and severe knee osteoarthritis patients, emphasizing the impact of severe muscle weakness in those with advanced knee osteoarthritis. The simulations offer valuable insights into the mechanisms behind altered movement strategies, potentially guiding more targeted treatment.
Quality of healthcare is at risk due to an increase of age-related health issues and a shortage of healthcare workers in the near future. The primary focus of many governments is to prolong the independence of older adults in their own homes. A crucial daily activity that supports this independence is the act of rising from a seated position (Dall and Kerr, 2010). This seemingly simple task becomes increasingly challenging as individuals age and is closely linked to a higher risk of falls (Fuller, 2000).
In Western societies, a significant percentage of individuals over the age of 65 suffer from symptomatic osteoarthritis (OA), particularly affecting the knee joint (Eysink et al., 2019). The ‘Timed Up and Go’ test (TUG) is a clinical evaluation of gait and balance, serving as a diagnostic tool in these individuals (Bouchouras et al., 2015). It involves a sit-to-walk exercise where individuals rise from a chair, walk a distance of 3 m, turn, walk back to the chair, and sit down. In a clinical setting, the time taken for this exercise is measured, if the exercise takes more than 30 s the patient is indicated with an high risk of falling.
Recent experimental research has demonstrated that beyond timing, biomechanical compensation strategies are indicative of the underlying age-related changes in the neuromuscular system and movement priorities in sit-to-walk (van der Kruk et al., 2022). Individuals with unilateral OA often exhibit asymmetrical movements while standing up, bearing additional weight on the unaffected side by leaning the trunk or by using asymmetric arm movements (Turcot et al., 2012; Naili et al., 2018; Davidson et al., 2013; Eitzen et al., 2014; Lamontagne et al., 2012). Additionally, they display increased maximum trunk flexion (Turcot et al., 2012; Sagawa et al., 2017). Recent systematic reviews suggest that these altered trunk flexion patterns may be related to muscle weakness and/or pain avoidance (van der Kruk and Geijtenbeek, 2023a).
Whereas experimental studies cannot distinguish between these underlying causes (van der Kruk et al., 2021), predictive simulations are a promising tool to determine the interconnectivity and interdependency of the neuromuscular capacity, reinforcement schemes, sensory integration, and adaptation strategies in standing up. We recently published the validation of a planar neuromusculoskeletal model with reflex-based muscle control that simulates the sit-to-walk movement (van der Kruk and Geijtenbeek, 2023a). This communication aims to leverage this predictive neuromuscular framework to determine whether the observed alterations in trunk flexion strategies in individuals with unilateral knee osteoarthritis are more likely attributed to muscle weakness or to pain avoidance.
The design and validation of the musculoskeletal model and controller have been previously published (van der Kruk and Geijtenbeek, 2023a). We employed the H1120 model, featuring 11 degrees of freedom and 20 Hill-type muscles, which was initially developed as an OpenSim3 model and translated into Hyfydy (Figure 1) (van der Kruk and Geijtenbeek, 2023b). Peak isometric forces in this model are based on a lower limb model by (G2392) (Delp et al., 1990). The validation study showed that the simulated joint angles, muscle activation, and joint loading fell within experimental ranges with some limitations. Due to simplified contact geometries of the chair and buttocks, thighs rolling over the chair’s surface is not considered in the simulation which in reality extend the contact period. Ground contact forces for the stance leg’s during the initial step were underestimated due to the simplified foot contact which results in an underestimation of the ankle joint load of the stance leg during this initial step.
Figure 1. The musculoskeletal model (H1120) has 11 degrees of freedom (3 dofs between the pelvis and the ground, a pin joint (1-dof) at the hip, ankle and knee, a 1-dof lumbar joint, between the pelvis and the lumbar, and a 1-dof thoracic joint between the lumbar and the torso) and is actuated using 20 Hill-type muscle-tendon units. The H1120 model is available on (van der Kruk and Geijtenbeek, 2023b).
Contact forces between the feet and the ground and between the buttocks and the chair were modelled with a Hunt Crossley force spheres and box, respectively. The simulation of the sit-to-walk movement involved the utilization of a standing-up controller (van der Kruk and Geijtenbeek, 2023a) followed by a gait controller (Geyer and Herr, 2010). The standing-up controller operates on a reflex control principle, featuring two distinct states, each with its own set of control parameters. The reflex controller is based on monosynaptic and antagonistic proprioceptive feedback from the muscles and vestibular feedback linked to the pelvis tilt. The timing of the transition between the states is part of the optimization problem. To account for neural latencies, we incorporated data from previous studies.
States calculated from the model were muscle length (L), muscle velocity (v), muscle force (F), and pelvis tilt orientation (θ) and velocity (θ˙). The optimization problem encompassed a total of 551 free parameters, comprising controller gains (KC, KL+, KF±, Kp, and Kv), muscle length feedback offsets (lo), proportional feedback of θ (θo), proportional feedback of the lumbar and thoracic joints, transition times between the controllers, and stance load threshold for the gait state controller.
We used the objective measures from the published sit-to-walk controller (van der Kruk and Geijtenbeek, 2023a). These measures encompassed the following criteria: a gait velocity measure with a minimum threshold of 0.8 m/s, range penalties designed to replicate joint limitations, including lumbar extension (−50°–0°), thorax extension (−15°–15°), pelvis tilt (−50°–30°), and ankle angle (−60°–60°), a constraint on knee limit force (500 N/m) that represents the passive knee properties when the knee exceeds the range of 120° flexion or 10° extension, and minimal head acceleration (threshold of 1 m/s^2). The selected speed was considered the minimum required speed to have a gait pattern that represents gait inside the lab and clinic. In daily life, normal gait speeds for older adults typically range around 0.9 m/s for women and 1.0 m/s for men (Kasović et al., 2021). As such, a speed of 0.8 m/s was considered a realistic minimum for measurements conducted in clinical environments.
Additionally, we included an energy estimate aimed at reducing energy consumption during the stand-up phase and minimizing the cost of transport during gait. The measure consisted of metabolic energy expenditure based on (Wang et al., 2012; Bhargava et al., 2004) (
The optimization process was conducted using the open source software SCONE with the Covariance Matrix Adaptation Evolutionary Strategy (CMA-ES) (Geijtenbeek, 2019). Simulations were performed using the Hyfydy simulation engine (Geijtenbeek, 2024). The population size of each generation was 10, and the number of iterations varied depending on the simulation duration. Multiple parallel optimizations were executed with the same initial guesses based on (van der Kruk and Geijtenbeek, 2023a), and the best set was subsequently employed as the starting point for the next set of optimizations, meaning the initial guesses for each condition were based on solution of the previous condition (−30% strength on −20% strength, etc.).
Muscular capacity progressively declines with age, with the largest relative decline in knee flexion-extension. Individuals with knee osteoarthritis also have pronounced decline in quadriceps strength (Slemenda et al., 1997; McAlindon et al., 1993; V Hurley et al., 1997). A recent review indicates that the Rectus Femoris (RF) exhibits the most substantial relative muscle atrophy (Naruse et al., 2023). However, imaging studies and cadaver studies in older (Ward et al., 2009) and younger adults (Handsfield et al., 2014) show that the Vasti (VAS) muscles undergo the greatest decline in absolute PCSA. Specifically, there is an approximately 85 cm2 reduction in the combined vastus lateralis, medialis, and intermedius, compared to an approximate 21 cm2 reduction in the RF.
To therefore simulate reduced muscular capacity in knee extension, we systematically bilaterally decreased the vasti (VAS) maximum isometric force by 20%, 30%, 40%, 50%, and 60%. We chose for a bilateral approach, since the contralateral knee in subjects with unilateral OA also show quadriceps strength deficits, although in a lesser extent (Alnahdi et al., 2012). We initiated the model with the optimized sit-to-walk controller for the standard seat (van der Kruk and Geijtenbeek, 2023a; van der Kruk and Geijtenbeek, 2023b) (neutral condition). The best set of the reduced model was then employed as the starting point for the subsequent optimization, replicating a progressive capacity decline scenario.
To simulate pain avoidance, we incorporated a cost function requiring the simulation to minimize the peak unilateral knee load of the stepping leg to less than 4, 3, and 2 times the body weight (BW). The joint load above the set threshold is integrated over time as measure. Individuals with symptomatic unilateral knee OA prefer to step with the affected leg first. Therefore, we implemented a cost function that becomes zero once the peak knee load on the stepping leg (left leg in simulations) was below the set threshold.
We analysed the trunk flexion, calculated as the angle between the global vertical and the trunk (sum of pelvis tilt, lumbar extension, and thoracic extension). Additionally, we assessed differences in timing, muscle activation, joint loading, and kinematics. The outcomes under various conditions were visualized using the OpenSim software (Delp et al., 2007).
Reducing the maximum isometric strength VAS led to an increased bilateral activation of the Vasti muscle (Figure 2). The simulation compensates with increased hip extensor activity (HAM, GMAX) and increased plantarflexor muscle activity (GAS, SOL) in the stepping leg. This aligns with findings from experimental studies on sit-to-stand movements in older adults (Smith et al., 2020).
Figure 2. (A) Muscle activation for conditions in which VAS strength is reduced. The grey shade represents experimental sEMG data adopted from (van der Kruk and Geijtenbeek, 2023a). Note that since no Maximum Voluntary Contraction was available in this dataset, the excitation was normalized by the maximum excitation measured within the individual complete sit-to-walk trials (stand up-walk 3 m–turn–walk 3 m–sit down). As a result the activation levels indicated by the sEMG might be higher than the actual activation levels. (B) Joint loading for conditions in which VAS strength is reduced.
We found a bilateral rise increase of ankle joint loading, particularly on the stepping side which doubled in the −50% condition compared to the neutral condition. Reduced VAS muscle strength thus resulted in elevated ankle loads. When VAS strength was further reduced to −60%, the model could not find a solution to rise from the seat unaided.
A minor increase in trunk flexion was observed when VAS strength was reduced (Figure 3). Trunk flexion at seat-off reached 42.8° in the −50% condition versus 38.3° in the −20% condition (40.8° in the neutral condition). The timing of the movement (Figure 3C) showed no noticeable pattern with reduced muscle strength. We hypothesize that in the −20% and 30% condition, there remains sufficient physiological reserve to allow for variations in gait velocity. Consequently, standing up can be slower, as gait velocity can compensate to achieve the desired average speed. Evidently, this compensatory strategy appears to be more efficient in terms of our cost assessment. However, as the strength reduction is further decreased to 40% and 50%, patterns of reduced movement velocity begin to emerge.
Figure 3. (A) Simulated sit-to-walk movement adopted from (van der Kruk and Geijtenbeek, 2023a). The stepping leg is the leg that steps first. (B) Trunk flexion from the initiation of the movement to initiation of seat unloading. (C) Conditions for the sit-to-walk simulations shown in color for seat-off, first toe off (when the ground reaction force of the stepping leg becomes zero), and fourth toe off. There is a mild increase in trunk flexion when the VAS strength was reduced. When the knee is unloaded there is a slower movement speed.
In the neutral condition, the peak knee loads (stance leg: 6.8 BW, stepping leg: 5.6 BW) and hip loads (stance leg: 3.6 BW, stepping leg: 3.4 BW) manifest just after seat-off. The peak ankle load in the stepping leg occurs during the rising phase (stepping leg: 1.3 BW), and for stance leg, it arises at the end of the single stance phase (stance leg: 2.4 BW), consistent with experimental data (van der Kruk and Geijtenbeek, 2023a). Unloading of the knee is accomplished by reducing ipsilateral activation of VAS and hamstrings (HAM) (Figure 4). Following Toe-off, there is an increased ipsilateral activation of the tibialis anterior muscle (TA), resulting in increased dorsiflexion, which compensates for reduced foot-ground clearance. Unloading the knee (ranging from −29% to −59%) led to reduced load on the ipsilateral hip (ranging from −23% to −39%) and ankle (ranging from −37% to −40%). However, the ipsilateral ankle did not exhibit proportional unloading, unlike the other joints. Loading on the contralateral knee and hip increased, ranging from +5% to +11% and 0% to +5%, respectively. As for the contralateral ankle, there was a load decrease in the <4BW condition (−4%) and an increase in the other conditions (14% and 29%).
Figure 4. (A) Muscle activation in which the knee joint of the stepping leg is unloaded (pain avoidance). The grey shade represents experimental sEMG data adopted from (van der Kruk and Geijtenbeek, 2023a). Note that since no Maximum Voluntary Contraction was available in this dataset, the excitation was normalized by the maximum excitation measured within the individual complete sit-to-walk trials (stand up-walk 3 m–turn–walk 3 m–sit down). As a result the activation levels indicated by the sEMG might be higher than the actual activation levels. (B) joint loading in which the knee joint of the stepping leg is unloaded.
Interestingly, during knee unloading, the simulation yielded a smaller, rather than a greater, trunk angle at seat-off compared to the neutral condition (Figure 4). There was an increase in movement time observed with increased knee unloading (from initiation to heel strike of the first step: 1.1s in the neutral condition up to 1.3s in the <2x BW knee load condition) (Figure 3D).
Our simulations reveal a minor increase in trunk flexion (2v) in response to a decrease in maximum isometric force in VAS (representing reduced muscular capacity). The data files, simulations, and videos can be found in the repository (van der Kruk and Geijtenbeek, 2024). Experimental studies have reported an approximate increase of nine° in trunk angle during sit-to-stand movements in individuals with osteoarthritis (OA) compared to controls (46.4° versus 37.5°) (Turcot et al., 2012). We observed similar differences in trunk angle when simulating a low seat condition presented in (van der Kruk and Geijtenbeek, 2023a; van der Kruk and Geijtenbeek, 2023b) (51° trunk angle, approximately 10° difference with neutral condition). Low seat height increases the task demand resulting in less muscular reserve. In essence, it reflects a reduced muscular capacity (van der Kruk et al., 2021). Consequently, we suspect that the trunk angle would have exhibited greater flexion had the muscle strength of more muscles been compromised. Pain avoidance, simulated with the objective of reducing knee load, resulted in altered muscle recruitments, characterized by reduced ipsilateral activation of VAS and HAM, and slower movement speeds. However, it did not yield greater trunk flexion strategies. The predictive simulations thus support the idea that increased trunk flexion is more likely a consequence of insufficient muscular reserve than a pain avoidance mechanism.
The increase in time to perform the sit-to-walk movement is consistent with findings in the sit-to-stand (STS) task. Previous studies have reported that individuals with knee OA take significantly more time to complete the STS task and TUG compared to older adults (Turcot et al., 2012; Su et al., 1998; Patsika et al., 2011). Various authors have postulated that this time difference may be attributed to increased forward body bending (Su et al., 1998)or quadriceps muscle weakness (Alghadir et al., 2015). However, the simulations show that reducing movement velocity alone results in decreased peak knee loading, and might therefore be the result of a pain reduction strategy.
Our simulation model contains a number of limitations, which can be addressed in future studies:
• The simulations are conducted with a planar model. In reality, human movements during sit-to-walk involve lateral trunk motions and arm support (van der Kruk et al., 2022). Individuals with unilateral symptomatic knee osteoarthritis can use this to redistribute the load (van der Kruk and Geijtenbeek, 2023a). However, this aspect could not be tested in the current model. Additionally, individuals with knee OA exhibit increased antagonist muscle activation (Davidson et al., 2013; Patsika et al., 2011; Bouchouras et al., 2015; Bouchouras et al., 2020), which is linked to the medial-lateral and internal-external rotational stability of the knee. These complexities were not captured by the simplified planar joint in the model.
• In the simulations we only tested the effect of reduced VAS isometric strength. In reality, OA patients experience muscle weakness in multiple muscle groups, which could contribute to greater trunk flexion. Furthermore, regarding the musculoskeletal model, the maximum isometric forces are based on (Delp et al., 1990), where VAS muscles are relatively weak for a healthy adult, as indicated by the maximum activation of this muscle during chair rising (van der Kruk and Geijtenbeek, 2023a).
• The H1120 model represents a male adult with a height of 1.80 m and a mass of 75 kg. Body height and mass influence movement strategies in sit-to-walk. (van der Kruk and Geijtenbeek, 2023a).To ensure direct comparisons with experimental datasets, it would be beneficial to diversify musculoskeletal models and simulations.
• The feet were modelled using single rigid bodies, and the addition of a separate toe segment might allow for further unloading of the knee. We do not expect this to drastically change the results, because the placement and stiffness of the contact spheres allows for mimicking toe rolls.
While the Timed-Up-and-Go test is a commonly employed clinical assessment, only the timing of the exercise is typically reported in clinical and literature contexts. There is a scarcity of biomechanical data pertaining to OA patients’ performance in this test, particularly regarding which leg they use to step out first. Our choice to reduce the peak load in the stepping leg was informed by clinical observations. Given that in the sit-to-walk movement, individuals must unload one leg to initiate gait, this assumption appears valid from a motor control perspective. We did conduct simulations where the stance leg was unloaded (available online (van der Kruk and Geijtenbeek, 2024)). Those simulations demonstrate that the energy objective costs were higher when the stance leg was unloaded compared to the stepping leg, providing further support for the idea that unloading the stepping leg is a more natural movement.
When examining published experimental data, greater trunk flexion was only observed in individuals with severe knee OA (van der Kruk and Geijtenbeek, 2023a). In contrast, individuals with moderate knee OA exhibited a similar movement trajectory to that of healthy individuals but with altered muscle recruitment patterns (van der Kruk and Geijtenbeek, 2023a). This discrepancy can be attributed to variations in muscle strength. It is plausible that individuals with severe OA, due to reduced daily activity, possess less muscular reserve compared to individuals with moderate OA. Consequently, severe OA patients may resort to increased trunk flexion during the process of standing up as a compensatory mechanism to counteract their strength limitations.
Taking this line of reasoning further, it is conceivable that in individuals with early and moderate symptomatic knee OA, unloading the knee to alleviate pain could potentially contribute to the development of muscular weakness in specific muscle groups, such as the vasti, over time. Humans stand up over 60 times a day and the simulations indicate that unloading the knee during this action is accomplished through reduced activation of the VAS. Since it is reasonable to infer that this compensation mechanism occurs in various daily activities, we can postulate the following hypothesis: individuals with early and moderate OA may unload the knee to mitigate pain, inadvertently leading to the underuse of specific muscle groups, particularly the vasti muscles. Over time, this underuse could result in a decline in vasti strength, resulting in increased trunk flexion, as observed in individuals with severe knee OA. Our interpretation for unilateral OA patients is that reduced activity leads to the bilateral muscle strength deficits while the specific compensation strategies during these activities, like reduced VAS activation, result in the unilateral muscle strength deficit in specific muscle groups in the affected limb (Figure 4). This underscores the significance of monitoring and addressing pain in individuals with early and moderate OA.
The original contributions presented in the study are included in the article/Supplementary material, further inquiries can be directed to the corresponding author.
EV: Conceptualization, Data curation, Funding acquisition, Investigation, Methodology, Resources, Supervision, Validation, Visualization, Writing–original draft, Writing–review and editing. TG: Software, Writing–review and editing.
The author(s) declare financial support was received for the research, authorship, and/or publication of this article. This study was funded by NWO-TTW VENI Grant 18145 (2021). The funders had no role in study design, data collection and analysis, decision to publish, or preparation of the manuscript.
Author TG was employed by Goatstream.
The remaining author declare that the research was conducted in the absence of any commercial or financial relationships that could be construed as a potential conflict of interest.
All claims expressed in this article are solely those of the authors and do not necessarily represent those of their affiliated organizations, or those of the publisher, the editors and the reviewers. Any product that may be evaluated in this article, or claim that may be made by its manufacturer, is not guaranteed or endorsed by the publisher.
Alghadir, A., Anwer, S., and Brismée, J.-M. (2015). The reliability and minimal detectable change of Timed up and Go test in individuals with grade 1–3 knee osteoarthritis. BMC Musculoskelet. Disord. 16, 174–177. doi:10.1186/s12891-015-0637-8
Alnahdi, A. H., Zeni, J. A., and Snyder-Mackler, L. (2012). Muscle impairments in patients with knee osteoarthritis. Sports Health 4 (4), 284–292. doi:10.1177/1941738112445726
Bhargava, L. J., Pandy, M. G., and Anderson, F. C. (2004). A phenomenological model for estimating metabolic energy consumption in muscle contraction. J. Biomech. 37 (1), 81–88. doi:10.1016/s0021-9290(03)00239-2
Bouchouras, G., Patsika, G., Hatzitaki, V., and Kellis, E. (2015). Kinematics and knee muscle activation during sit-to-stand movement in women with knee osteoarthritis. Clin. Biomech. 30 (6), 599–607. doi:10.1016/j.clinbiomech.2015.03.025
Bouchouras, G., Sofianidis, G., Patsika, G., Kellis, E., and Hatzitaki, V. (2020). Women with knee osteoarthritis increase knee muscle co-contraction to perform stand to sit. Aging Clin. Exp. Res. 32, 655–662. doi:10.1007/s40520-019-01245-z
Dall, P. M., and Kerr, A. (2010). Frequency of the sit to stand task: an observational study of free-living adults. Appl. Ergon. 41 (1), 58–61. doi:10.1016/j.apergo.2009.04.005
Davidson, B. S., Judd, D. L., Thomas, A. C., Mizner, R. L., Eckhoff, D. G., and Stevens-Lapsley, J. E. (2013). Muscle activation and coactivation during five-time-sit-to-stand movement in patients undergoing total knee arthroplasty. J. Electromyogr. Kinesiol. 23 (6), 1485–1493. doi:10.1016/j.jelekin.2013.06.008
Delp, S. L., Anderson, F. C., Arnold, A. S., Loan, P., Habib, A., John, C. T., et al. (2007). OpenSim: open-source software to create and analyze dynamic simulations of movement. IEEE Trans. Biomed. Eng. 54 (11), 1940–1950. doi:10.1109/tbme.2007.901024
Delp, S. L., Loan, J. P., Hoy, M. G., Zajac, F. E., Topp, E. L., and Rosen, J. M. (1990). An interactive graphics-based model of the lower extremity to study orthopaedic surgical procedures. IEEE Trans. Biomed. Eng. 37 (8), 757–767. doi:10.1109/10.102791
Eitzen, I., Fernandes, L., Nordsletten, L., Snyder-Mackler, L., and Risberg, M. A. (2014). Weight-bearing asymmetries during Sit-To-Stand in patients with mild-to-moderate hip osteoarthritis. Gait Posture 39 (2), 683–688. doi:10.1016/j.gaitpost.2013.09.010
Eysink, P. E. D., Poos, M., Gijsen, R., Kommer, G. J., and van Gool, C. H. (2019). Epidemiologische data van Ziekten van het botspierstelsel en bindweefsel: achtergrondrapport voor Programma Zinnige Zorg.
Geijtenbeek, T. (2019). Scone: open source software for predictive simulation of biological motion. J. Open Source Softw. 4 (38), 1421. doi:10.21105/joss.01421
Geijtenbeek, T. (2024). The {Hyfydy} simulation software. Available at: https://hyfydy.com.
Geyer, H., and Herr, H. (2010). A muscle-reflex model that encodes principles of legged mechanics produces human walking dynamics and muscle activities. IEEE Trans. neural Syst. rehabilitation Eng. 18 (3), 263–273. doi:10.1109/tnsre.2010.2047592
Handsfield, G. G., Meyer, C. H., Hart, J. M., Abel, M. F., and Blemker, S. S. (2014). Relationships of 35 lower limb muscles to height and body mass quantified using MRI. J. Biomech. 47 (3), 631–638. doi:10.1016/j.jbiomech.2013.12.002
Kasović, M., Štefan, L., and Štefan, A. (2021). Normative data for gait speed and height norm speed in≥ 60-year-old men and women. Clin. Interv. Aging 16, 225–230. doi:10.2147/cia.s290071
Lamontagne, M., Beaulieu, M. L., Varin, D., and Beaulé, P. E. (2012). Lower-limb joint mechanics after total hip arthroplasty during sitting and standing tasks. J. Orthop. Res. 30 (10), 1611–1617. doi:10.1002/jor.22127
McAlindon, T. E., Cooper, C., Kirwan, J. R., and Dieppe, P. A. (1993). Determinants of disability in osteoarthritis of the knee. Ann. Rheum. Dis. 52 (4), 258–262. doi:10.1136/ard.52.4.258
Naili, J. E., Broström, E. W., Gutierrez-Farewik, E. M., and Schwartz, M. H. (2018). The centre of mass trajectory is a sensitive and responsive measure of functional compensations in individuals with knee osteoarthritis performing the five times sit-to-stand test. Gait Posture 62, 140–145. doi:10.1016/j.gaitpost.2018.03.016
Naruse, M., Trappe, S., and Trappe, T. A. (2023). Human skeletal muscle-specific atrophy with aging: a comprehensive review. J. Appl. Physiol. 134 (4), 900–914. doi:10.1152/japplphysiol.00768.2022
Patsika, G., Kellis, E., and Amiridis, I. G. (2011). Neuromuscular efficiency during sit to stand movement in women with knee osteoarthritis. J. Electromyogr. Kinesiol. 21 (5), 689–694. doi:10.1016/j.jelekin.2011.05.006
Sagawa, Y., Bonnefoy-Mazure, A., Armand, S., Hoffmeyer, P., Suva, D., and Turcot, K. (2017). Individuals with knee osteoarthritis exhibit altered movement patterns during the sit-to-stand task. Mov. Sport Sciences-Science Mot. (98), 39–49. doi:10.1051/sm/2017004
Slemenda, C., et al. (1997). Quadriceps weakness and osteoarthritis of the knee. Ann. Intern Med. 127 (2), 97–104. doi:10.7326/0003-4819-127-2-199707150-00001
Smith, S. H. L., Reilly, P., and Bull, A. M. J. (2020). A musculoskeletal modelling approach to explain sit-to-stand difficulties in older people due to changes in muscle recruitment and movement strategies. J. Biomech. 98, 109451. doi:10.1016/j.jbiomech.2019.109451
Su, F. C., Lai, K. A., and Hong, W. H. (1998). Rising from chair after total knee arthroplasty. Clin. Biomech. 13 (3), 176–181. doi:10.1016/s0268-0033(97)00039-9
Turcot, K., Armand, S., Fritschy, D., Hoffmeyer, P., and Suvà, D. (2012). Sit-to-stand alterations in advanced knee osteoarthritis. Gait Posture 36 (1), 68–72. doi:10.1016/j.gaitpost.2012.01.005
van der Kruk, E., and Geijtenbeek, T. (2023a). A planar neuromuscular controller to simulate age-related adaptation strategies in the sit-to-walk movement. bioRxiv, 2011–2023. doi:10.1101/2023.11.24.568552
van der Kruk, E., and Geijtenbeek, T. (2023b). Repository sit-to-walk simulations. Available at: https://github.com/BODIES-Lab-TU-Delft/Sit-to-walk-predictive-simulations.
van der Kruk, E., and Geijtenbeek, T. (2024). Repository case study simulations. Available at: https://github.com/BODIES-Lab-TU-Delft/STW-case-study-unilateral-pain.git.
van der Kruk, E., Silverman, A. K., Koizia, L., Reilly, P., Fertleman, M., and Bull, A. M. J. (2021). Age-related compensation: neuromusculoskeletal capacity, reserve and movement objectives. J. Biomech. 122, 110385. doi:10.1016/j.jbiomech.2021.110385
van der Kruk, E., Strutton, P., Koizia, L. J., Fertleman, M., Reilly, P., and Bull, A. M. J. (2022). Why do older adults stand-up differently to young adults? investigation of compensatory movement strategies in sit-to-walk. npj Aging 8 (1), 13. doi:10.1038/s41514-022-00094-x
V Hurley, M., Scott, D. L., Rees, J., and Newham, D. J. (1997). Sensorimotor changes and functional performance in patients with knee osteoarthritis. Ann. Rheum. Dis. 56 (11), 641–648. doi:10.1136/ard.56.11.641
Wang, J. M., Hamner, S. R., Delp, S. L., and Koltun, V. (2012). Optimizing locomotion controllers using biologically-based actuators and objectives. ACM Trans. Graph. (TOG) 31 (4), 1–11. doi:10.1145/2185520.2335376
Keywords: predictive simulation, neuromuscular model, knee osteoarthritis, ageing, sit-to-walk, timed-up-and-go
Citation: Van Der Kruk E and Geijtenbeek T (2024) Is increased trunk flexion in standing up related to muscle weakness or pain avoidance in individuals with unilateral knee pain; a simulation study. Front. Bioeng. Biotechnol. 12:1346365. doi: 10.3389/fbioe.2024.1346365
Received: 29 November 2023; Accepted: 28 March 2024;
Published: 10 April 2024.
Edited by:
Anne D. Koelewijn, Technische Fakultät, Friedrich-Alexander-Universität Erlangen-Nürnberg, GermanyReviewed by:
Varun Joshi, University of Michigan, United StatesCopyright © 2024 Van Der Kruk and Geijtenbeek. This is an open-access article distributed under the terms of the Creative Commons Attribution License (CC BY). The use, distribution or reproduction in other forums is permitted, provided the original author(s) and the copyright owner(s) are credited and that the original publication in this journal is cited, in accordance with accepted academic practice. No use, distribution or reproduction is permitted which does not comply with these terms.
*Correspondence: Eline Van Der Kruk, ZS52YW5kZXJrcnVrQHR1ZGVsZnQubmw=
Disclaimer: All claims expressed in this article are solely those of the authors and do not necessarily represent those of their affiliated organizations, or those of the publisher, the editors and the reviewers. Any product that may be evaluated in this article or claim that may be made by its manufacturer is not guaranteed or endorsed by the publisher.
Research integrity at Frontiers
Learn more about the work of our research integrity team to safeguard the quality of each article we publish.