- 1Department of Chemistry, Kohat University of Science and Technology, Kohat, Pakistan
- 2Department of Dental Materials, Institute of Basic Medical Sciences Khyber Medical University, Peshawar, Pakistan
- 3Department of Biochemistry, Bahauddin Zakariya University, Multan, Pakistan
- 4Department of Pharmacognosy, College of Pharmacy, King Saud University, Riyadh, Saudi Arabia
- 5Department of Pharmaceutical Chemistry, College of Pharmacy, King Saud University Riyadh, Riyadh, Saudi Arabia
- 6Department of Infectious Diseases, The Affiliated Hospital of Southwest Medical University, Luzhou, China
Hydrogen peroxide (H2O2) is one of the main byproducts of most enzymatic reactions, and its detection is very important in disease conditions. Due to its essential role in healthcare, the food industry, and environmental research, accurate H2O2 determination is a prerequisite. In the present work, Morus nigra sawdust deposited zinc oxide (ZnO) nanoparticles (NPs) were synthesized by the use of Trigonella foenum extract via a hydrothermal process. The synthesized platform was characterized by various techniques, including UV-Vis, FTIR, XRD, SEM, EDX, etc. FTIR confirmed the presence of a Zn‒O characteristic peak, and XRD showed the hexagonal phase of ZnO NPs with a 35 nm particle size. The EDX analysis confirmed the presence of Zn and O. SEM images showed that the as-prepared nanoparticles are distributed uniformly on the surface of sawdust. The proposed platform (acetic acid-capped ZnO NPs deposited sawdust) functions as a mimic enzyme for the detection of H2O2 in the presence of 3,3′,5,5′-tetramethylbenzidine (TMB) colorimetrically. To get the best results, many key parameters, such as the amount of sawdust-deposited nanoparticles, TMB concentration, pH, and incubation time were optimized. With a linear range of 0.001–0.360 μM and an R2 value of 0.999, the proposed biosensor’s 0.81 nM limit of quantification (LOQ) and 0.24 nM limit of detection (LOD) were predicted, respectively. The best response for the proposed biosensor was observed at pH 7, room temperature, and 5 min of incubation time. The acetic acid-capped sawdust deposited ZnO NPs biosensor was also used to detect H2O2 in blood serum samples of diabetic patients and suggest a suitable candidate for in vitro diagnostics and commercial purposes.
1 Introduction
Hydrogen peroxide (H2O2) monitoring in diverse matrices has crucial roles in cell metabolism and has diverse applications in industrial processes (Zhang et al., 2018). H2O2 is used in medical diagnostics, clinical research and industrial sectors including textiles, paper, pharmaceuticals, food processing, cleaning, disinfection, etc (Patel et al., 2020). Additionally, in the biosystem, it regulates metabolic activity, cell apoptosis, immune cell activation, and different physiological processes (Miller et al., 2010). It serves as an oxidative agent, a stress marker, and a cell defensive agent. Similarly, it is an important biomarker for a variety of diseases and disorders, including cardiovascular, Alzheimer’s, Parkinson’s, diabetes, and neurodegenerative disorders (Rao and Balachandran, 2002). Furthermore, H2O2 is a byproduct of lactate, alcohol, glucose, glutamate, and cholesterol oxidases. In the past, different detection methods for H2O2 quantifications were applied. These include chemiluminescence (Irani-nezhad et al., 2019), chromatography (Nakashima et al., 1994), electrochemistry (Lee et al., 2016), fluorescence (Senthamizhan et al., 2016), electrochemical methods, etc (Chen et al., 2014). However, most of these approaches are toxic to living cells, thus making them ineffective for in situ H2O2 detection in biological materials. Besides, some of these techniques are time-consuming, expensive, and complex, restricting their application in laboratories with limited resources (Nishan et al., 2021a). Conversely, in comparison to other complex approaches, colorimetric methods for detecting H2O2 have been getting key attention nowadays due to their easy handling and low cost. The progress of colorimetric reactions can be monitored with the naked eye (Khaliq et al., 2023).
Cellulose is the main constituent of sawdust. It is one of the most abundant, natural, renewable, biocompatible, and environmentally friendly macromolecules (Park et al., 2019). Cellulosic materials have adaptable surface characteristics, low cost, better mechanical properties, a higher aspect ratio, a lower density, a higher surface area, and a lower density. Cellulose-based sawdust has been utilized as a sacrificial porous template because it is non-edible, cheap, renewable, and readily available biomass (Khaliq et al., 2023).
Various nanomaterials, including positively charged gold nanoparticles (Jv et al., 2010), CuS nanoparticles (Dutta et al., 2013), graphene oxide (Song et al., 2010), ceria nanoparticles (Ornatska et al., 2011), cupric oxide nanoparticles (Chen et al., 2011), and CoFe2O4 NPs (Shi et al., 2011), have been found to exhibit peroxidase-like activity and are employed to detect H2O2 visually. Additionally, acetic acid-capped ZnO NPs are recyclable, highly stable, and efficient, have good sensing and catalytic capabilities, and have a tremendous potential to replace expensive noble metal NPs in biosensing. Because ZnO NPs have a large band gap (3.3 eV), they can be employed for UV luminescence at room temperature (Khranovskyy et al., 2012). Furthermore, ZnO NPs have a high isoelectric point (pI) of 9.5, allowing effective immobilization of enzymes with a low pI, i.e., ≤5 (Wei et al., 2010). ZnO NPs are also biocompatible, have the largest family of nanostructures, are crystalline, and have a high surface-to-volume ratio (Abou Chaaya et al., 2014).
In the present study, the hydrothermal method was used for the synthesis of Morus nigra-deposited ZnO NPs with the use of Trigonella foenum extract as a reductant. To further improve their sensing abilities, the synthesized NPs were capped with acetic acid. The oxidation of chromogenic substrate, i.e., TMB, by H2O2 in the presence of acetic acid-capped sawdust deposited@ZnO is being reported for the first time. The proposed platform is a new, simple, quick, highly sensitive, and selective approach for H2O2 detection. The amount of capped NPs, pH, TMB concentration, and incubation time were among the various reaction parameters that were adjusted to achieve the best performance out of the suggested sensor. The sensitivity and selectivity were also investigated under the aforementioned optimum conditions. Finally, H2O2 levels were also measured in blood serum samples to testify to the fabricated platform.
2 Experimental procedure
2.1 Materials and reagents
In the entire experimental procedure, all chemicals used were of analytical grade, and no further purification was performed. Double-distilled water was used in the preparation of solutions. NaOH (97%), HCl (37%), acetic acid (97%), ascorbic acid (97%), urea (99.5%), and 3,3′,5,5′-tetramethylbenzidine (TMB) were procured from Daejung, South Korea. KGaA and H2O2 (35%) were purchased from Merck. The collection of blood serum was performed at a local lab close to the divisional teaching hospital in Kohat, KP, from three diabetic individuals. The serum was twice diluted with a PBS solution to decrease the complexity of the matrix, according to the earlier reports (Singh et al., 2022).
2.2 Instrumentation
Fourier transform infrared spectroscopy (FTIR, Nicolet 6,700, US) was used to find the characteristic peaks of the synthesized platform in the range of 4,000–500 cm-1. The morphology of the synthesized NPs was confirmed using a scanning electron microscope (SEM) with INCAx-act Oxford Instruments (TESCAN VEGA (LMU)). X-ray diffraction was used to identify the phases of the produced ZnO NPs (JCPDS, file No. 04-0783). The absorption spectra were taken with a Shimadzu UV-Vis spectrophotometer (1,800, Japan).
2.3 Synthesis of Sawdust-deposited@ZnO NPs
The green leaves of T. foenum were collected, washed with distilled water, and dried in sunlight for 4 days. The leaves were ground into a fine powder with the help of a blender. Extract was prepared in distilled water by suspending 5 g of leaf powder in 200 mL of distilled water on a hot plate with a magnetic stirrer (1,000 rpm) for 1 h at 65°C. The mixture was filtered, and the extract was poured into a beaker. One Gram of zinc acetate was dissolved in 50 mL of distilled water and placed on a hot plate. Subsequently, 50 mL of extract solution was added dropwise to the zinc acetate solution, and 1 g of sawdust from M. nigra was gradually added while stirring at 65°C for 4 h. The synthesized sawdust-deposited@ZnO NPs solution was centrifuged for 15 min at 4,500 rpm to obtain solid material.
2.4 Capping of sawdust-deposited@ZnO NPs with acetic acid
The Sawdust-deposited@ZnO NPs were capped with an acetic acid solution such that 0.12 g of the mimic enzyme was mixed with 2 mL of acetic acid for 30 min through a mortar and pestle thoroughly. It resulted in the formation of a brown mixture that was placed in an Eppendorf tube for further use (Asad et al., 2022; Nishan et al., 2022).
2.5 Colorimetric sensing of H2O2
Capped sawdust-deposited@ZnO NPs (25 μL) were suspended in 500 μL phosphate buffer (pH 7), followed by the addition of 150 μL TMB solution (18 mM). Add 90 μL of H2O2 (0.360 μM) to the reaction mixture and incubate at room temperature for the colorimetric reaction. The absorption spectrum of the resultant solution was recorded using a UV-Vis spectrophotometer. Some experimental parameters, such as response time, pH, the amount of capped NPs, and the concentration of TMB solution, have been tuned up to achieve the best results of the proposed platform.
3 Results and discussion
3.1 Characterization of the sawdust-deposited@ZnO NPs
3.1.1 UV-vis spectroscopy
To investigate the optical characteristics of the sawdust-deposited@ZnO NPs, a UV-Visible spectrophotometer was used. The UV-Vis absorption spectrum of the synthesized sawdust-deposited@ZnO NPs with a peculiar absorption band at 320 nm is shown in Figure 1A. The fact that ZnO has a considerable, sharp absorption implies that the nanoparticles distribution is monodispersed (Talam et al., 2012).
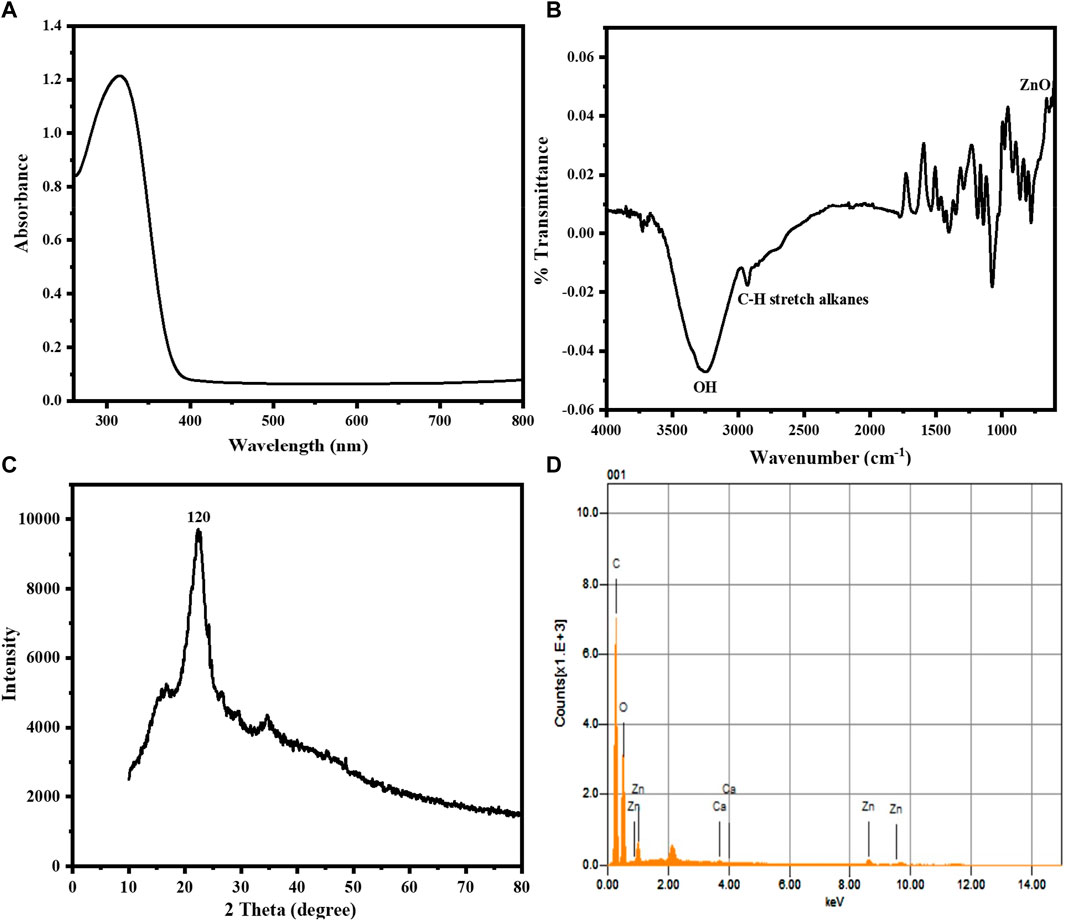
FIGURE 1. (A) UV-Vis absorption spectrum of the sawdust-deposited@ZnO NPs showing its characteristic surface Plasmon resonance peak at 320 nm. (B) FTIR spectrum of the synthesized sawdust-deposited@ZnO NPs showing the presence of a ZnO band. (C) XRD pattern of the synthesized sawdust-deposited@ZnO NPs, indicating the orthorhombic phase of ZnO. (D) EDX spectral analysis of the synthesized sawdust-deposited@ZnO showing the presence of C, Zn, and O.
3.1.2 FTIR analysis of the sawdust-deposited@ZnO NPs
FTIR analysis in the range of 4,000–500 cm-1, was used to determine the different functional groups found on the surface of the sawdust-deposited@ZnO NPs. The broad absorption band at 3,310 cm-1 indicates the presence of an OH group from the plant source on the surface of the synthesized platform. The peak around 2,950 cm-1 shows the C-H stretching vibration of the alkyl group present in the mimic enzyme. The most important characteristic peak around 580 cm-1 represents the presence of Zn‒O bond present in our synthesized platform, indicating that the ZnO nanoparticles present in the sawdust-deposited@ZnO NPs are as shown in Figure 1B. A similar pattern of peaks has already been reported for ZnO in the literature (Xiong et al., 2006).
3.1.3 XRD analysis
The X-ray diffraction pattern of the synthesized sawdust-deposited@ZnO NPs is shown in Figure 1C. The XRD results of the prepared platform centered at 2θ = 21 reveal a diffraction peak with miller indices of 120. When compared to standard data, it was found that the peak matched the hexagonal phase of ZnO NPs standard data (JCPDS card no. 36–1451) (Srivastava et al., 2013). The average crystal size of orthorhombic-phase ZnO NPs was calculated to be about 35 nm using Scherer equation.
3.1.4 EDX analysis
The chemical composition of the sawdust-deposited@ZnO NPs was examined using EDX analysis, as shown in Figure 1D and Table 1. The results showed the presence of Zn and O in the sawdust-deposited@ZnO sample. In addition to Zn and O, some other elements like Ca and C are also present. The percent contents of Zn, O, C, and Ca are 3.64, 46.95, 49.90, and 0.17, respectively, by weight as shown in the table.
3.1.5 SEM analysis
To investigate the surface morphology of the synthesized sawdust-deposited@ZnO NPs, SEM images of different resolutions were taken, as shown in Figure 2A−D. SEM images confirmed that the prepared ZnO NPs are distributed uniformly over the surface of sawdust. This uniform distribution of the nanoparticles is highly desirable and helpful in terms of the surface area of the nanoparticles for their catalytic activity.
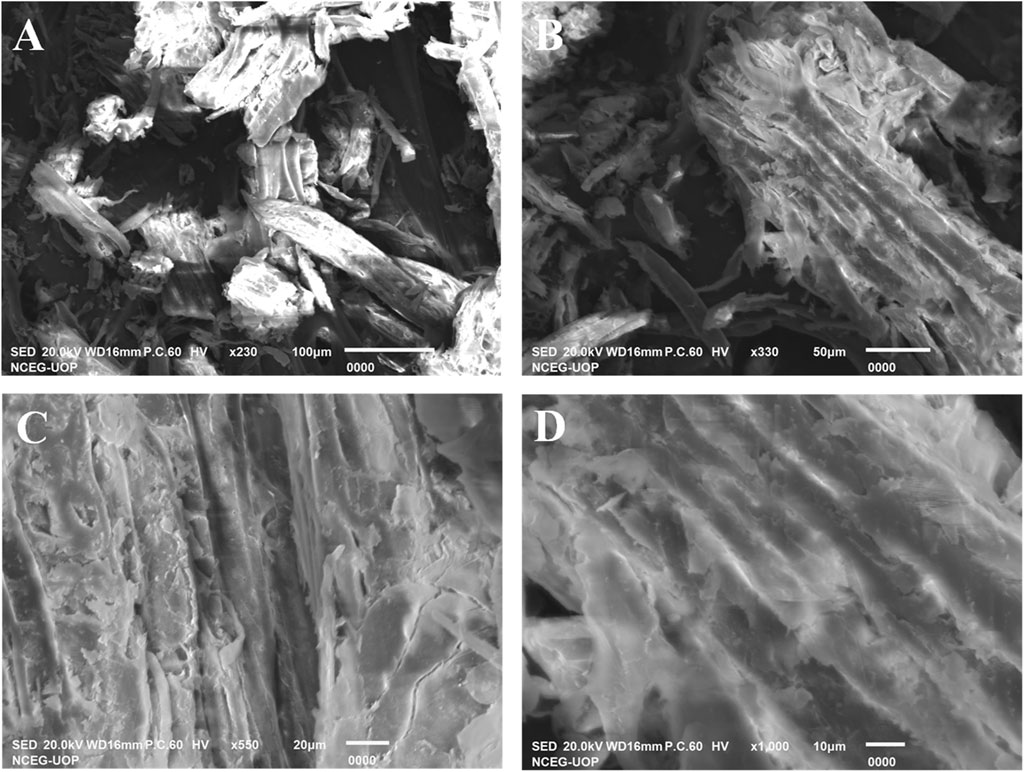
FIGURE 2. SEM images (A‒D) were taken at different magnifications. The results depict that the nanoparticles are distributed uniformly over the surface of sawdust to enhance its catalytic activity.
3.2 Colorimetric detection of H2O2
H2O2 sensing by the proposed sawdust-deposited@ZnO NPs was done using a very simple and highly selective colorimetric approach. The optical sensing and UV-Vis absorption spectra are shown in Figure 3. When H2O2 is introduced to the sensor system, it produces a blue-green color from the colorless TMB. Mechanistically, adsorption of H2O2 on the surface of NPs produces OH radicals, which oxidize the colorless TMB substrate into a blue-green product, as can be seen with the naked eye, as shown in Figure. The colorimetric change was confirmed by a UV-Vis spectrophotometer. To confirm that the colorimetric change was due to the synthesized sawdust-deposited@ZnO NPs, we used M. nigra sawdust without ZnO NPs as a negative control. When H2O2 was added, no color change was detected; indicating that the color change was caused only by the capped sawdust-deposited@ZnO NPs. UV-Vis spectroscopic investigation validated the negative control experiment, as indicated in Figure.
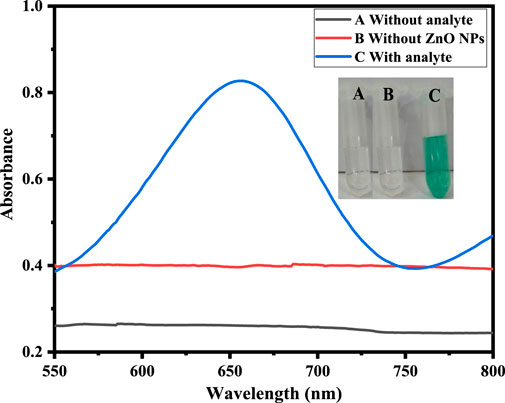
FIGURE 3. Shows sawdust-deposited@ZnO NPs-based H2O2 biosensing. It shows UV-Vis spectra of the solution containing capped sawdust-deposited@ZnO NPs (20 μL), PBS pH 7 (500 μL), TMB (18 mM: 150 µL), and H2O2 (0.360 μM: 100 µL). Spectra were obtained without H2O2 as well as in the presence of H2O2. The Figure shows the UV-Vis spectra of a peculiar solution. Curve A represents the reaction system without analyte, B represents the reaction in which sawdust was used without ZnO NPs. Curve C represents the colorimetric change and peak at 652 nm that occurred when H2O2 was introduced to the sawdust-deposited@ZnO NPs.
3.3 Proposed mechanism of the reaction
In the current work, mimic enzyme (acetic acid-capped ZnO NPs deposited sawdust) receive electrons from TMB. It results in an increase in the conductivity of electrons in the mimic enzyme, which provides an active site for the proposed chemical reaction. The mobility of electrons results in the transfer of electrons to H2O2. It results in the generation of hydroxyl free radicals. The generated hydroxyl free radicals oxidize the TMB, resulting in the formation of a blue-green complex. This colorimetric change is visible to the naked eye and was also confirmed with a UV-Vis spectrophotometer. The maximum absorption was found to be at 652 nm. The detailed proposed reaction can be in seen in Scheme 1.
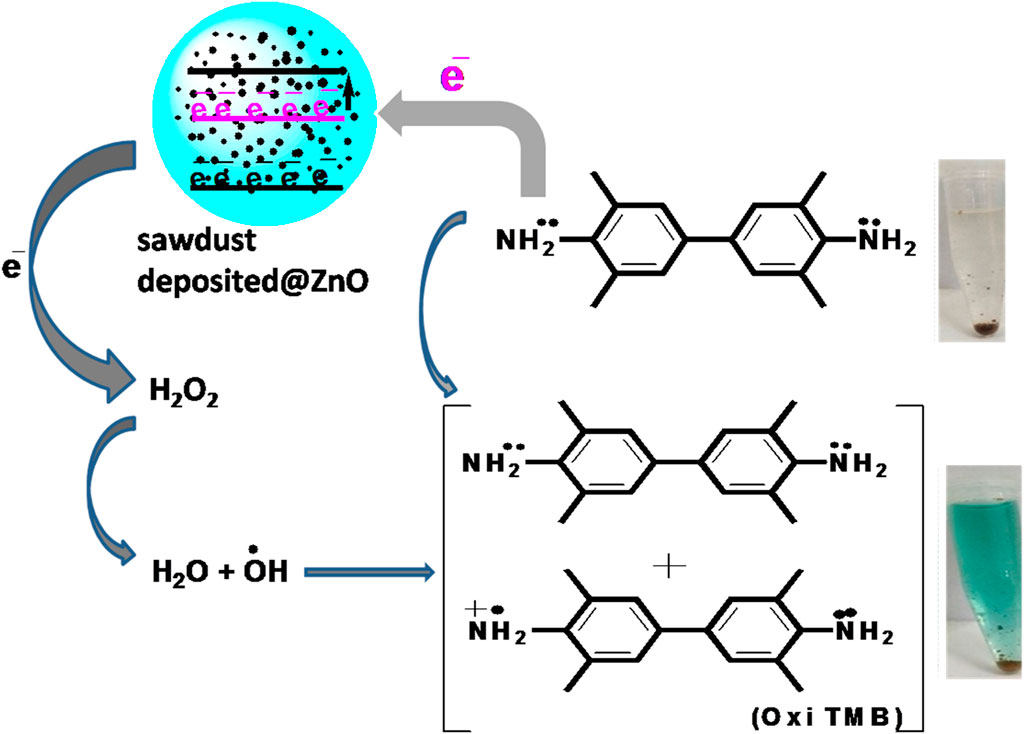
SCHEME 1. Showing the proposed reaction for the colorimetric sensing of hydrogen peroxide based on the fabricated mimic enzyme.
3.4 Optimization of parameters
3.4.1 Amount of capped ZnO NPs
In order to get the best colorimetric response, we first optimized the amount of capped sawdust-deposited@ZnO NPs. Briefly, different amounts (10–70 µL) of the capped sawdust-deposited@ZnO NPs were tested, and the best colorimetric response was obtained at a 40 µL concentration, as shown in Figure 4A. No significant colorimetric response was obtained below 40 μL, so the 40 µL amount was taken as the optimum amount for further experiments. Previously, we reported about 25 μL of capped TiO2 NPs as an optimum concentration for the colorimetric sensing of H2O2 (Nishan et al., 2021a). Under the given conditions, an increase in the concentration of the mimic enzyme from 40 µL up to 70 µL results in a lower response. This can possibly be explained by the fact that unreacted mimic enzyme interferes with the already oxidized TMB, resulting in much lower absorption.
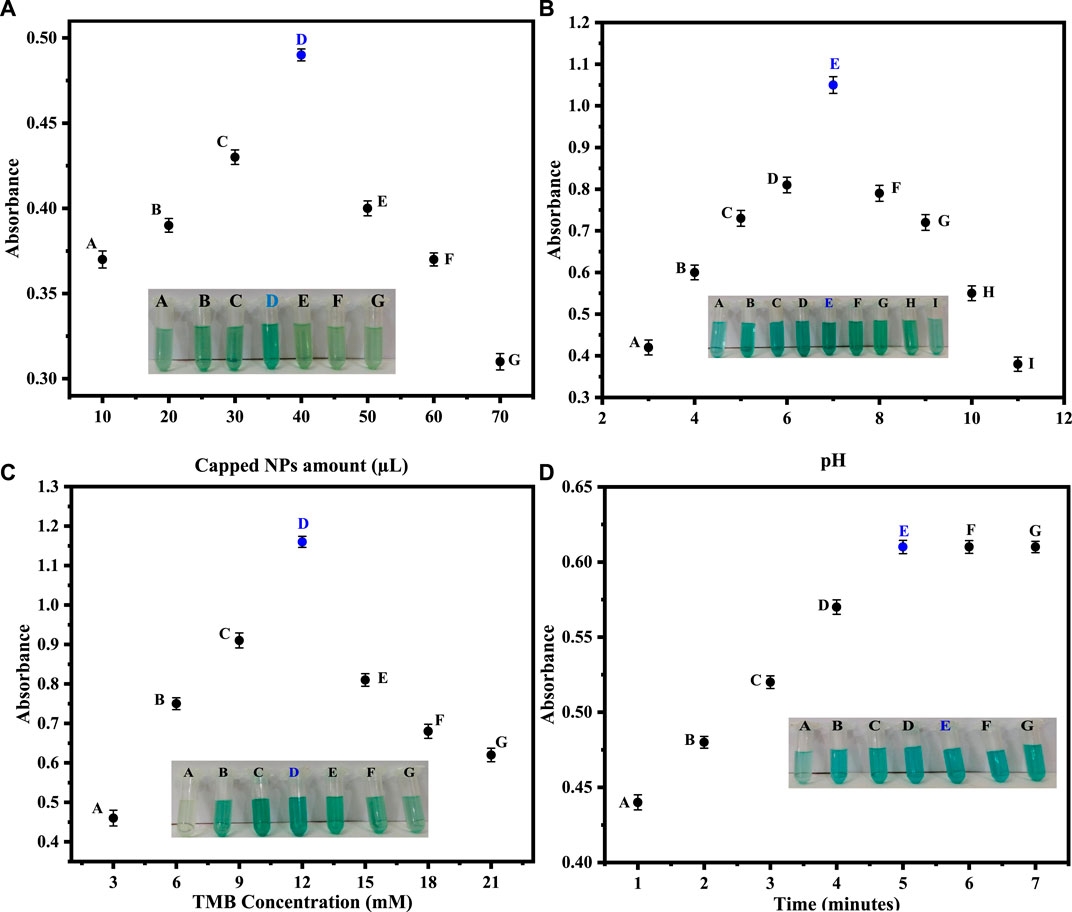
FIGURE 4. (A) Optimization of the capped sawdust-deposited@ZnO NPs [Reaction cond. PBS 500 µL (pH 7), TMB 150 µL (18 mM), H2O2 100 µL (0.360 μM). (B) Different pH optimizations for the proposed capped sawdust-deposited@ZnO NPs show the best colorimetric response at pH 7. [Reaction cond. Capping 20 μL, TMB 150 µL (18 mM), H2O2 100 µL (0.360 μM)]. (C) The optimization of TMB concentration of the capped sawdust-deposited@ZnO NPs [Reaction cond. Capping 40 μL, PBS 500 µL (pH 7), H2O2 100 µL (0.360 μM)]. (D) Reaction time optimization for the suggested capped sawdust-deposited@ZnO NPs [reaction cond. Capping 40 μL, PBS 500 µL (pH 7), TMB 150 µL (12 mM), H2O2 100 µL (0.360 μM)].
3.4.2 pH optimization
Different pH optimizations were done to get the maximum colorimetric response. Briefly, different pH solutions of PBS were made, and their respective pH values were adjusted using sodium hydroxide and hydrochloric acid solutions. The best colorimetric response shown by the capped sawdust-deposited@ZnO NPs was recorded on pH 7, as shown in Figure 4B. No significant colorimetric response was noticed above or below this optimum pH of 7, therefore pH 7 was selected as the optimum pH for further experiments. At a lower pH, the concentration of hydrogen ions increases, which results in the protonation of the amino group of the chromogenic substrate TMB. This protonation of TMB makes it less susceptible to oxidation, resulting in a lower colorimetric change. The increase in pH above 7 results in an increase in hydroxyl ion concentration. As a result, the oxidation of TMB reduces, and hence less colorimetric change can be observed. Similarly an earlier study reported pH 7.5 to be optimum for the colorimetric sensing of hydrogen peroxide (Nishan et al., 2021b).
3.4.3 Optimization of TMB concentration
TMB solutions of different concentrations ranging from 3 to 21 mM were prepared. Initially, the colorimetric response increased up to 12 mM and then decreased as the concentration of TMB increased from 13 mM to 21 mM. The best colorimetric response was noticed at 12 mM, as shown in Figure 4C. Recently, for the detection of hydrogen peroxide, the 8 mM optimum TMB concentration was reported by our groups for another nanostructure (Nishan et al., 2023). This could possibly be explained by the fact that in the reported work, a pristine form of nanomaterial functionalized with ionic liquid was used as a mimic enzyme. In the current work, sawdust was used as a matrix material, hence the higher concentration of TMB.
3.4.4 Optimization of time
In colorimetric detection of hydrogen peroxide, the reaction incubation time was also optimized. A colorimetric response was noticed at various time intervals (1–7 min) after adding hydrogen peroxide. The reaction time at various intervals was recorded by UV-Vis spectroscopy. After 5 min, no further change in color or absorbance was noticed, indicating that 5 min is the optimal time for a complete reaction, as shown in Figure 4D. According to the literature, the optimum time for the detection of hydrogen peroxide was 10 min, as reported by (Zarif et al., 2020), which is much higher than our present work.
3.5 Optimization of hydrogen peroxide concentration
H2O2 was detected using a quick and easy colorimetric method based on capped sawdust-deposited@ZnO NPs under ideal experimental circumstances. As seen in Figure 5, the developed biosensor’s sensitivity for H2O2 detection was tested using a range of H2O2 concentrations. At lower H2O2 concentrations, the sensor response and peak intensity were negligible, but as the concentration rose, they grew linearly. H2O2 detection with an R2 value of 0.999 and a linear range of 0.001–0.360 μM was made possible by this method. It was determined that the limits of quantification (LOQ) and detection (LOD) were, respectively, 0.24 nM and 0.81 nM. The suggested colorimetric approach had the advantages of a low detection limit, low cost, and naked eye observation over other previously published detection methods. Based on the linear range and limit of detection, we compared this work for H2O2 detection with previously reported colorimetric approaches, as shown in Table 2. It is clear from the results that the fabricated sensor showed an exceptional limit of detection and a comparable wide linear range with previous works from our group.
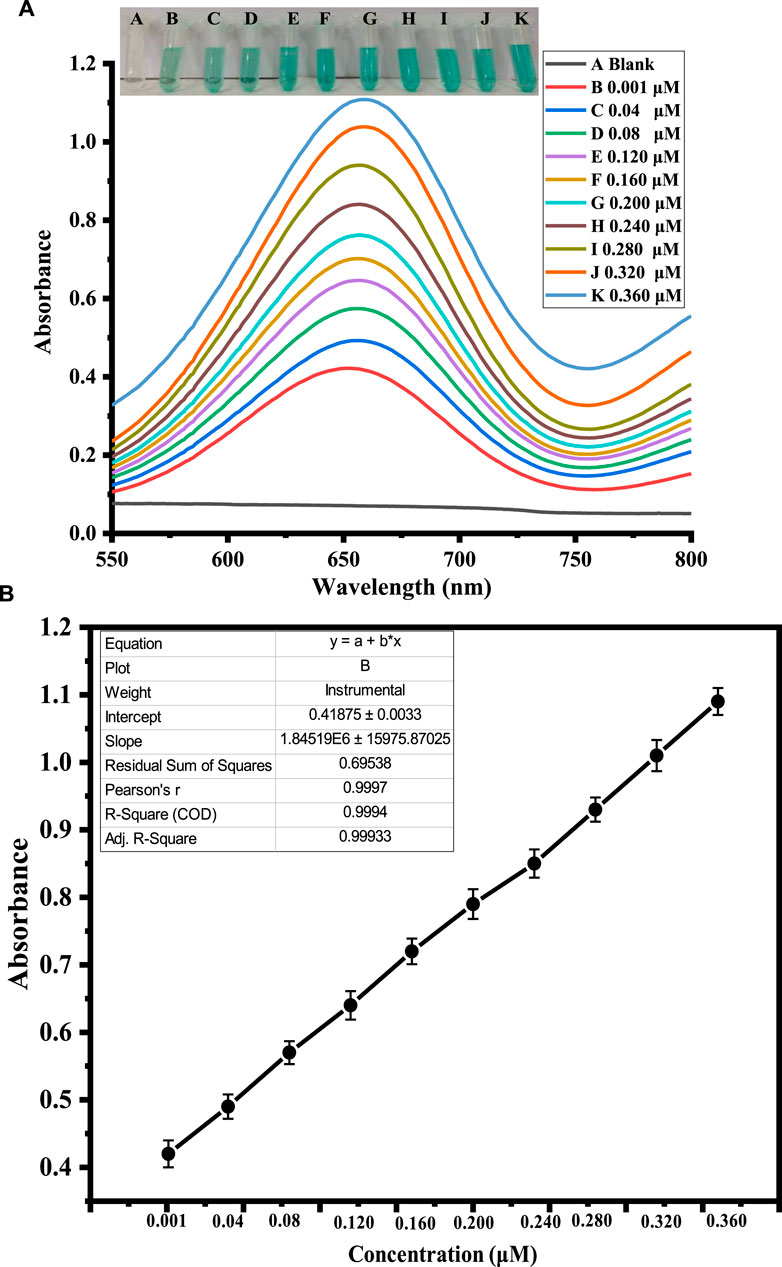
FIGURE 5. Shows the optimization of H2O2 concentration. Figure (A) shows the UV-Vis response recorded at different concentrations of H2O2. The inset figure shows varying color changes with the addition of different concentrations of H2O2. Figure (B) shows the corresponding calibration curve of the absorbance at different H2O2 concentrations.
3.6 Selectivity analysis the proposed sensor
The potential interfering chemicals, including ascorbic acid, lead, uric acid, glucose, and nitrite, were used to test the selectivity of the proposed sensor. All these interfering chemicals had substantially lower absorbance than H2O2, as shown in Figure 6. The recorded absorbance was highest when H2O2 was added, and no significant absorbance change was seen when a co-existing material was added. In the presence of higher amounts of ascorbic acid, lead, uric acid, glucose, and nitrite ions, the suggested sensor has a substantially stronger selectivity for H2O2. All the experiments were performed in the presence of 0.360 μM H2O2 and a double concentration of other interfering substances.
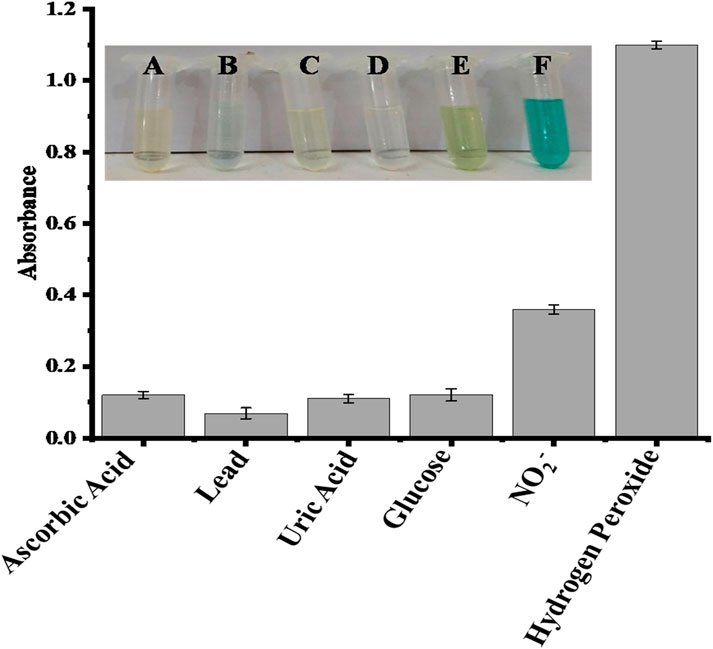
FIGURE 6. Comparative interference study of the proposed capped sawdust-deposited@ZnO NPs sensor for the detection of hydrogen peroxide with other analytes, indicating high selectivity of the proposed platform for the sensing of H2O2. In the inset Figure, the letters A, B, C, D, E, and F represent ascorbic acid, lead, uric acid, glucose, nitrite, and hydrogen peroxide, respectively.
3.7 Real sample analysis
To assess the practical application of the proposed sensor to detect H2O2 content, the measurement of H2O2 in the blood serum sample of a diabetes patient was carried out as shown in Table 3. The present amount of H2O2 was calculated from the already calibrated graph by using the spiking method. Different concentrations of H2O2 solution, such as 0.017, 0.120, and 0.206 μM, were spiked into the blood serum sample of a diabetes patient and analyzed, as shown in Figure 7. The results demonstrated that the H2O2 concentrations in the real samples determined by the current assay are in good agreement with the spiked H2O2 concentrations.
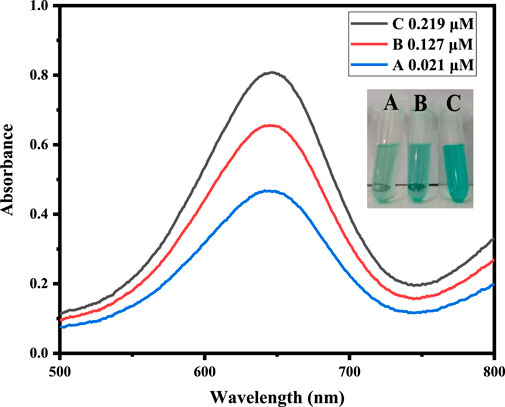
FIGURE 7. Real sample analysis of a blood serum sample of a diabetes patient at optimized conditions by the addition of different concentrations of hydrogen peroxide, such as 0.017, 0.120, and 0.206 μM.
4 Conclusion
Morus nigra-deposited ZnO@NPs were successfully synthesized from T. foenum extract. The synthesized platform was characterized with various standard analytical techniques, including FTIR, SEM, XRD, and EDX. The synthesized sawdust-deposited@ZnO NPs were capped with acetic acid and successfully used for the colorimetric sensing of H2O2. Our current finding demonstrates that acetic acid-capped sawdust-deposited@ZnO NPs show enhanced intrinsic peroxidase-like activity. The proposed platform showed good sensitivity and selectivity in the presence of a double amount of potential interfering species. The fabricated platform shows a number of advantages over natural enzymes, including easy preparation, low cost, quick reaction times, and high stability. These advantages make it a suitable candidate peroxidase-mimic for future applications in biotechnology, medical diagnostics, and hydrogen peroxide monitoring.
Data availability statement
The raw data supporting the conclusion of this article will be made available by the authors, without undue reservation.
Author contributions
UN: Conceptualization, Formal Analysis, Investigation, Project administration, Supervision, Writing–original draft, Writing–review and editing. TZ: Data curation, Investigation, Methodology, Writing–original draft. AB: Investigation, Methodology, Project administration, Supervision, Visualization, Writing–review and editing. NM: Conceptualization, Formal Analysis, Methodology, Visualization, Writing–review and editing. SA: Data curation, Formal Analysis, Investigation, Validation, Writing–review and editing. MS: Data curation, Investigation, Software, Writing–original draft. NK: Formal Analysis, Methodology, Validation, Visualization, Writing–review and editing. MA: Data curation, Formal Analysis, Investigation, Methodology, Software, Writing–original draft. RU: Funding acquisition, Investigation, Project administration, Resources, Software, Visualization, Writing–review and editing. EA: Formal Analysis, Funding acquisition, Methodology, Project administration, Resources, Visualization, Writing–original draft. KC: Conceptualization, Funding acquisition, Investigation, Methodology, Project administration, Resources, Supervision, Writing–original draft, Writing–review and editing.
Funding
The author(s) declare financial support was received for the research, authorship, and/or publication of this article. This research work is supported by the Doctoral research fund of the Affiliated Hospital of Southwest Medical University, Luzhou 646000, China, awarded to KC. The authors thank researchers supporting Project number (RSP2024R110) King Saud University, Riyadh, Saudi Arabia.
Acknowledgments
KC acknowledges the doctoral research fund of the Affiliated Hospital of Southwest Medical University, Luzhou 646000, China. Authors wish to thanks Researchers Supporting Project Number (RSP2024R110) at King Saud University Riyadh Saudi Arabia for financial support.
Conflict of interest
The authors declare that the research was conducted in the absence of any commercial or financial relationships that could be construed as a potential conflict of interest.
Publisher’s note
All claims expressed in this article are solely those of the authors and do not necessarily represent those of their affiliated organizations, or those of the publisher, the editors and the reviewers. Any product that may be evaluated in this article, or claim that may be made by its manufacturer, is not guaranteed or endorsed by the publisher.
References
Abou Chaaya, A., Bechelany, M., Balme, S., and Miele, P. (2014). ZnO 1D nanostructures designed by combining atomic layer deposition and electrospinning for UV sensor applications. J. Mater. Chem. A 2, 20650–20658. doi:10.1039/c4ta05239k
Asad, M., Muhammad, N., Khan, N., Shah, M., Khan, M., Khan, M., et al. (2022). Colorimetric acetone sensor based on ionic liquid functionalized drug-mediated silver nanostructures. J. Pharm. Biomed. Analysis 221, 115043. doi:10.1016/j.jpba.2022.115043
Bandi, R., Alle, M., Park, C.-W., Han, S.-Y., Kwon, G.-J., Kim, N.-H., et al. (2021). Cellulose nanofibrils/carbon dots composite nanopapers for the smartphone-based colorimetric detection of hydrogen peroxide and glucose. Sensors Actuators B Chem. 330, 129330. doi:10.1016/j.snb.2020.129330
Chen, W., Chen, J., Liu, A. L., Wang, L. M., Li, G. W., and Lin, X. H. (2011). Peroxidase-like activity of cupric oxide nanoparticle. ChemCatChem 3, 1151–1154. doi:10.1002/cctc.201100064
Chen, X., Wu, G., Cai, Z., Oyama, M., and Chen, X. (2014). Advances in enzyme-free electrochemical sensors for hydrogen peroxide, glucose, and uric acid. Microchim. Acta 181, 689–705. doi:10.1007/s00604-013-1098-0
Choleva, T. G., Gatselou, V. A., Tsogas, G. Z., and Giokas, D. L. (2018). Intrinsic peroxidase-like activity of rhodium nanoparticles, and their application to the colorimetric determination of hydrogen peroxide and glucose. Microchim. Acta 185, 22–29. doi:10.1007/s00604-017-2582-8
Dutta, A. K., Das, S., Samanta, S., Samanta, P. K., Adhikary, B., and Biswas, P. (2013). CuS nanoparticles as a mimic peroxidase for colorimetric estimation of human blood glucose level. Talanta 107, 361–367. doi:10.1016/j.talanta.2013.01.032
Guan, J., Peng, J., and Jin, X. (2015). Synthesis of copper sulfide nanorods as peroxidase mimics for the colorimetric detection of hydrogen peroxide. Anal. Methods 7, 5454–5461. doi:10.1039/c5ay00895f
Irani-nezhad, M. H., Khataee, A., Hassanzadeh, J., and Orooji, Y. (2019). A chemiluminescent method for the detection of H2O2 and glucose based on intrinsic peroxidase-like activity of WS2 quantum dots. Molecules 24, 689. doi:10.3390/molecules24040689
Jv, Y., Li, B., and Cao, R. (2010). Positively-charged gold nanoparticles as peroxidiase mimic and their application in hydrogen peroxide and glucose detection. Chem. Commun. 46, 8017–8019. doi:10.1039/c0cc02698k
Khaliq, A., Nazir, R., Khan, M., Rahim, A., Asad, M., Shah, M., et al. (2023). Co-doped CeO2/activated C nanocomposite functionalized with ionic liquid for colorimetric biosensing of H2O2 via peroxidase mimicking. Molecules 28, 3325. doi:10.3390/molecules28083325
Khranovskyy, V., Lazorenko, V., Lashkarev, G., and Yakimova, R. (2012). Luminescence anisotropy of ZnO microrods. J. Luminescence 132, 2643–2647. doi:10.1016/j.jlumin.2012.04.048
Lee, J. H., Huynh-Nguyen, B.-C., Ko, E., Kim, J. H., and Seong, G. H. (2016). Fabrication of flexible, transparent silver nanowire electrodes for amperometric detection of hydrogen peroxide. Sensors Actuators B Chem. 224, 789–797. doi:10.1016/j.snb.2015.11.006
Liu, H., Zhu, L., Ma, H., Wen, J., Xu, H., Qiu, Y., et al. (2019). Copper (II)-coated Fe3O4 nanoparticles as an efficient enzyme mimic for colorimetric detection of hydrogen peroxide. Microchim. Acta 186, 518–519. doi:10.1007/s00604-019-3599-y
Miller, E. W., Dickinson, B. C., and Chang, C. J. (2010). Aquaporin-3 mediates hydrogen peroxide uptake to regulate downstream intracellular signaling. Proc. Natl. Acad. Sci. 107, 15681–15686. doi:10.1073/pnas.1005776107
Nakashima, K., Wada, M., Kuroda, N., Akiyama, S., and Imai, K. (1994). High-performance liquid chromatographic determination of hydrogen peroxide with peroxyoxalate chemiluminescence detection. J. Liq. Chromatogr. Relat. Technol. 17, 2111–2126. doi:10.1080/10826079408013535
Nishan, U., Haq, S. U., Rahim, A., Asad, M., Badshah, A., Ali Shah, A.-u.-H., et al. (2021a). Ionic-liquid-stabilized TiO2 nanostructures: a platform for detection of hydrogen peroxide. ACS omega 6, 32754–32762. doi:10.1021/acsomega.1c04548
Nishan, U., Khan, H. U., Rahim, A., Asad, M., Qayum, M., Khan, N., et al. (2022). Non-enzymatic colorimetric sensing of nitrite in fortified meat using functionalized drug mediated manganese dioxide. Mater. Chem. Phys. 278, 125729. doi:10.1016/j.matchemphys.2022.125729
Nishan, U., Niaz, A., Muhammad, N., Asad, M., Khan, N., Khan, M., et al. (2021b). Non-enzymatic colorimetric biosensor for hydrogen peroxide using lignin-based silver nanoparticles tuned with ionic liquid as a peroxidase mimic. Arabian J. Chem. 14, 103164. doi:10.1016/j.arabjc.2021.103164
Nishan, U., Ullah, I., Muhammad, N., Afridi, S., Asad, M., Haq, S. U., et al. (2023). Investigation of silver-doped iron oxide nanostructures functionalized with ionic liquid for colorimetric sensing of hydrogen peroxide. Arabian J. Sci. Eng. 48, 7703–7712. doi:10.1007/s13369-023-07791-z
Ornatska, M., Sharpe, E., Andreescu, D., and Andreescu, S. (2011). Paper bioassay based on ceria nanoparticles as colorimetric probes. Anal. Chem. 83, 4273–4280. doi:10.1021/ac200697y
Park, N.-M., Choi, S., Oh, J. E., and Hwang, D. Y. (2019). Facile extraction of cellulose nanocrystals. Carbohydr. Polym. 223, 115114. doi:10.1016/j.carbpol.2019.115114
Patel, V., Kruse, P., and Selvaganapathy, P. R. (2020). Solid state sensors for hydrogen peroxide detection. Biosensors 11 (9), 9. doi:10.3390/bios11010009
Rao, A., and Balachandran, B. (2002). Role of oxidative stress and antioxidants in neurodegenerative diseases. Nutr. Neurosci. 5, 291–309. doi:10.1080/1028415021000033767
Rauf, S., Ali, N., Tayyab, Z., Shah, M. Y., Yang, C. P., Hu, J., et al. (2020). Ionic liquid coated zerovalent manganese nanoparticles with stabilized and enhanced peroxidase-like catalytic activity for colorimetric detection of hydrogen peroxide. Mater. Res. Express 7, 035018. doi:10.1088/2053-1591/ab7f10
Senthamizhan, A., Balusamy, B., Aytac, Z., and Uyar, T. (2016). Ultrasensitive electrospun fluorescent nanofibrous membrane for rapid visual colorimetric detection of H 2 O 2. Anal. Bioanal. Chem. 408, 1347–1355. doi:10.1007/s00216-015-9149-5
Shi, W., Zhang, X., He, S., and Huang, Y. (2011). CoFe 2 O 4 magnetic nanoparticles as a peroxidase mimic mediated chemiluminescence for hydrogen peroxide and glucose. Chem. Commun. 47, 10785–10787. doi:10.1039/c1cc14300j
Singh, J., Singh, R., Singh, S., Mitra, K., Mondal, S., Vishwakarma, S., et al. (2022). Colorimetric detection of hydrogen peroxide and cholesterol using Fe3O4-brominated graphene nanocomposite. Anal. Bioanal. Chem. 414, 2131–2145. doi:10.1007/s00216-021-03848-w
Song, Y., Qu, K., Zhao, C., Ren, J., and Qu, X. (2010). Graphene oxide: intrinsic peroxidase catalytic activity and its application to glucose detection. Adv. Mater. 22, 2206–2210. doi:10.1002/adma.200903783
Srivastava, V., Gusain, D., and Sharma, Y. C. (2013). Synthesis, characterization and application of zinc oxide nanoparticles (n-ZnO). Ceram. Int. 39, 9803–9808. doi:10.1016/j.ceramint.2013.04.110
Talam, S., Karumuri, S. R., and Gunnam, N. (2012). Synthesis, characterization, and spectroscopic properties of ZnO nanoparticles. Int. Sch. Res. Notices 2012, 1–6. doi:10.5402/2012/372505
Teodoro, K. B., Migliorini, F. L., Christinelli, W. A., and Correa, D. S. (2019). Detection of hydrogen peroxide (H2O2) using a colorimetric sensor based on cellulose nanowhiskers and silver nanoparticles. Carbohydr. Polym. 212, 235–241. doi:10.1016/j.carbpol.2019.02.053
Wei, Y., Li, Y., Liu, X., Xian, Y., Shi, G., and Jin, L. (2010). ZnO nanorods/Au hybrid nanocomposites for glucose biosensor. Biosens. Bioelectron. 26, 275–278. doi:10.1016/j.bios.2010.06.006
Xiong, G., Pal, U., Serrano, J., Ucer, K., and Williams, R. (2006). Photoluminesence and FTIR study of ZnO nanoparticles: the impurity and defect perspective. Phys. status solidi C. 3, 3577–3581. doi:10.1002/pssc.200672164
Zarif, F., Rauf, S., Khurshid, S., Muhammad, N., Hayat, A., Rahim, A., et al. (2020). Effect of pyridinium based ionic liquid on the sensing property of Ni0 nanoparticle for the colorimetric detection of hydrogen peroxide. J. Mol. Struct. 1219, 128620. doi:10.1016/j.molstruc.2020.128620
Zhang, L., Hai, X., Xia, C., Chen, X.-W., and Wang, J.-H. (2017). Growth of CuO nanoneedles on graphene quantum dots as peroxidase mimics for sensitive colorimetric detection of hydrogen peroxide and glucose. Sensors Actuators B Chem. 248, 374–384. doi:10.1016/j.snb.2017.04.011
Zhang, W., Ma, D., and Du, J. (2014). Prussian blue nanoparticles as peroxidase mimetics for sensitive colorimetric detection of hydrogen peroxide and glucose. Talanta 120, 362–367. doi:10.1016/j.talanta.2013.12.028
Zhang, W., Niu, X., Li, X., He, Y., Song, H., Peng, Y., et al. (2018). A smartphone-integrated ready-to-use paper-based sensor with mesoporous carbon-dispersed Pd nanoparticles as a highly active peroxidase mimic for H2O2 detection. Sensors Actuators B Chem. 265, 412–420. doi:10.1016/j.snb.2018.03.082
Keywords: diabetes, cancer, TMB, hydrothermal process, colorimetric biosensing
Citation: Nishan U, Zahra T, Badshah A, Muhammad N, Afridi S, Shah M, Khan N, Asad M, Ullah R, Ali EA and Chen K (2024) Colorimetric sensing of hydrogen peroxide using capped Morus nigra-sawdust deposited zinc oxide nanoparticles via Trigonella foenum extract. Front. Bioeng. Biotechnol. 12:1338920. doi: 10.3389/fbioe.2024.1338920
Received: 15 November 2023; Accepted: 29 January 2024;
Published: 08 February 2024.
Edited by:
Kang Cui, University of Jinan, ChinaReviewed by:
Forough Ghasemi, Agricultural Biotechnology Research Institute of Iran, IranJaveed Mahmood, King Abdullah University of Science and Technology, Saudi Arabia
Copyright © 2024 Nishan, Zahra, Badshah, Muhammad, Afridi, Shah, Khan, Asad, Ullah, Ali and Chen. This is an open-access article distributed under the terms of the Creative Commons Attribution License (CC BY). The use, distribution or reproduction in other forums is permitted, provided the original author(s) and the copyright owner(s) are credited and that the original publication in this journal is cited, in accordance with accepted academic practice. No use, distribution or reproduction is permitted which does not comply with these terms.
*Correspondence: Umar Nishan, dW1hcm5pc2hhbjg1QGdtYWlsLmNvbQ==; Ke Chen, Y2hlbl9rZUBzd211LmVkdS5jbg==