- 1Department of Prosthodontics, Peking University School and Hospital of Stomatology and National Center for Stomatology and National Clinical Research Center for Oral Diseases and National Engineering Research Center of Oral Biomaterials and Digital Medical Devices and Beijing Key Laboratory of Digital Stomatology and Research Center of Engineering and Technology for Computerized Dentistry Ministry of Health and NMPA Key Laboratory for Dental Materials, Beijing, China
- 2Stem Cell and Regenerative Medicine Lab, Beijing Institute of Radiation Medicine, Beijing, China
- 3Department of Periodontology, Peking University School and Hospital of Stomatology and National Center for Stomatology and National Clinical Research Center for Oral Diseases and National Engineering Research Center of Oral Biomaterials and Digital Medical Devices and Beijing Key Laboratory of Digital Stomatology and Research Center of Engineering and Technology for Computerized Dentistry Ministry of Health and NMPA Key Laboratory for Dental Materials, Beijing, China
The term “peri-implantitis” (peri-implantitis) refers to an inflammatory lesion of the mucosa surrounding an endosseous implant and a progressive loss of the peri-implant bone that supports the implant. Recently, it has been suggested that the increased sensitivity of implants to infection and the quick elimination of supporting tissue after infection may be caused by a dysregulated peri-implant mucosal immune response. Macrophages are polarized in response to environmental signals and play multiple roles in peri-implantitis. In peri-implantitis lesion samples, recent investigations have discovered a considerable increase in M1 type macrophages, with M1 type macrophages contributing to the pro-inflammatory response brought on by bacteria, whereas M2 type macrophages contribute to inflammation remission and tissue repair. In an effort to better understand the pathogenesis of peri-implantitis and suggest potential immunomodulatory treatments for peri-implantitis in the direction of macrophage polarization patterns, this review summarizes the research findings related to macrophage polarization in peri-implantitis and compares them with periodontitis.
1 Introduction
Since Brånemark first made dental implants available in the 1960s, they have been the norm for those with edentulism and missing teeth (Brånemark et al., 1969; Brånemark et al., 1977; Albrektsson, Brånemark, Hansson and Lindström, 1981). However, peri-implantitis (PI) is an increasingly serious biological complication of oral implantology. Its prevalence increases with the duration of the implant (French, Ofec and Levin, 2021; Obreja et al., 2021). The term “peri-implantitis” (PI) refers to an inflammatory lesion of the mucosa surrounding an endosseous implant and a progressive loss of the peri-implant bone that supports the implant (Renvert, Persson, Pirih and Camargo, 2018). According to reports, it affects between 5% and 37% of implants and between 11% and 53% of patients (Fransson, Lekholm, Jemt and Berglundh, 2005; Roos-Jansåker, Lindahl, Renvert and Renvert, 2006; Renvert, Roos-Jansåker, Lindahl, Renvert and Rutger Persson, 2007; Koldsland, Scheie and Aass, 2010; Rinke, Ohl, Ziebolz, Lange and Eickholz, 2011).
Although the clinical and radiological manifestations of PI and periodontitis share many features, there are key differences in their clinical progression, histological features, and microbial composition, suggesting different pathogenesis (Carcuac and Berglundh, 2014). By using 16S pyrosequencing, Kumar et al. discovered that the peri-implant microbiome differs greatly from the periodontal microbiome with regard to both health and illness. Peri-implantitis is a microbiological heterogeneous infection predominantly brought on by Gram-negative bacteria (i.e., the dominant species are not the same in each individual) and is not as complex as periodontitis (Kumar et al., 2012).
When PI samples were compared to periodontitis samples, the region of inflammatory infiltration was more than twice as large in the PI samples, and there were also considerably more macrophages and plasma cells in the PI samples overall (Carcuac and Berglundh, 2014). In both PI and periodontitis lesions, plasma cells and lymphocytes predominate. However, PMN and macrophages take more percentage in PI than in periodontitis (Esposito et al., 1997; Gualini and Berglundh, 2003; Berglundh, Gislason, Lekholm, Sennerby and Lindhe, 2004; Berglundh, Zitzmann and Donati, 2011; Carcuac and Berglundh, 2014). The periapical tissue goes through a “self-limiting” process when the ligature is removed in which the connective tissue capsule divides the ICT from the bone in periodontitis, whereas in the peri-implant tissue, the ICT extends to the bone crest (Berglundh, Zitzmann and Donati, 2011).
Implants dysregulate the immune response in the peri-implant mucosa (PIM), as shown by the development of a mouse model of dental implants and experimental PI (Pirih et al., 2015; Koutouzis, Eastman, Chukkapalli, Larjava and Kesavalu, 2017; Tzach-Nahman, Mizraji, Shapira, Nussbaum and Wilensky, 2017; Heyman et al., 2018; Heyman et al., 2022). This “dysregulated homeostasis” or inflammatory condition of the PIM may be the cause of the implant’s greater vulnerability to infection and the swift elimination of supporting tissue after infection (Carcuac and Berglundh, 2014).
Notably, Macrophages become polarized while responding to environmental signals, with M1 macrophages playing a role in bacterially-induced pro-inflammatory responses and M2 macrophages in inflammation regression and tissue repair (Yu et al., 2016; Palevski et al., 2017). Studies have shown an increase in polymorphonuclear leukocytes (PMN) and macrophages in PI lesions compared to periodontitis. Additionally, PI lesion samples revealed a notable rise in M1 macrophages (Fretwurst et al., 2020). This kind of macrophage polarization feature could partially explain the faster progression of PI in humans compared to periodontitis. It is consistent with the finding that PI advances more quickly than periodontitis because there is an increased quantity and density of PMN and macrophages (particularly M1) in the peri-implant lesions (Dionigi, Larsson, Carcuac and Berglundh, 2020). Studies on the function of macrophage polarization in the onset of PI and periodontitis have gradually risen in recent years (Figure 1). This review summarizes the research results related to macrophage polarization in PI and compares them with periodontitis in an attempt to deepen the understanding of the pathogenesis of PI and propose possible immunomodulatory therapies for PI in the direction of macrophage polarization patterns. This will improve our knowledge of, capacity to avoid, and manage PI.
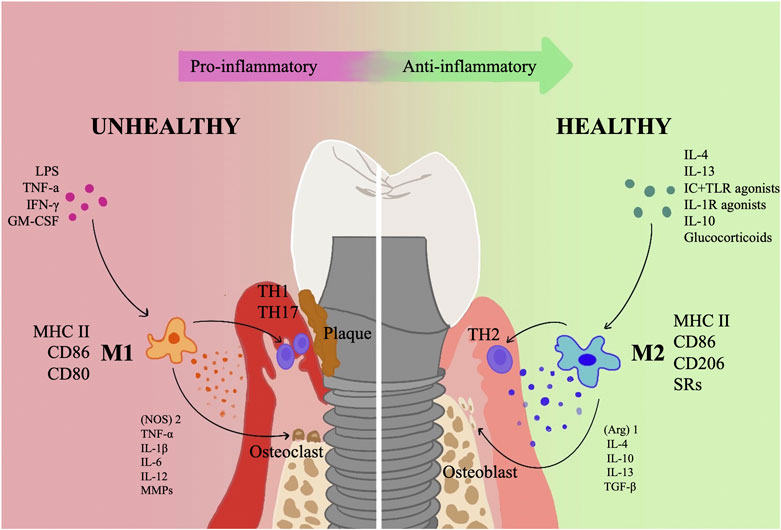
FIGURE 1. An overview of how polarized macrophages contribute to the incidence and growth of PI. The progressive and retreating phases of inflammation are dominated, respectively, by the M1 and M2 phenotypes of macrophages. M1 primarily serves a pro-inflammatory role, releasing a number of pro-inflammatory substances such (NOS)2, TNF-α, IL-1, IL-6, IL-12, and MMPs and collaborating with Th1 and Th17 cells. In addition, M1 type activates osteoclasts and causes resorption of alveolar bone; M2 type primarily functions as an anti-inflammatory, carrying out tissue repair via a variety of anti-inflammatory factors, such as (Arg)1, IL-4, IL-10, IL-13, and TGF-β, mainly synergizes with Th2 cells, and activates osteoblasts to promote bone regeneration.
2 Overview of the polarization of macrophages
Macrophages were first recognized for their phagocytic abilities. They also contributed to host-microbe equilibrium, antigen presentation, mobilization of immune defense mechanisms, and resistance to bacterial infection (Sun X. et al., 2021). Although several attempts have been made to classify macrophages, the most widely accepted classification has been the mononuclear phagocytic system (MPS). There are also other functional classifications of macrophages, for example, binary classification of inflammatory states classifies macrophages into activated macrophages and alternatively activated macrophages (AAM) (Gordon and Martinez, 2010; Sica and Mantovani, 2012; Wynn, Chawla and Pollard, 2013).
Macrophages can adjust to a variety of activation states that fall under the M1/M2 phenotypes of macrophage polarization in order to support immune activity and maintain tissue homeostasis (Martinez, Sica, Mantovani and Locati, 2008). The pro-inflammatory cytokines TNF-γ, interleukins IL-1, IL-6, and IL-12, as well as a high volume of reactive nitrogen and oxygen intermediates, are all produced by M1 macrophages after being primed by the interferon IFN-γ. These responses encourage Th1 responses with potent bactericidal and antitumor activity. IL-4 or IL-13 can prime M2 macrophages, which then express high levels of a metabolic marker called arginase (Arg) 1, the differentiation cluster CD206, and the anti-inflammatory cytokine IL-10, thereby dampening the inflammatory response to preserve tissue homeostasis, thereby attenuating the inflammatory response to maintain tissue homeostasis. The repression of parasites, stimulation of tissue remodeling, advancement of tumors, and immunomodulatory actions are all facilitated by M2 macrophages. Table 1 summaries the polarization types, characteristics and basic functions of macrophages. In summary, M1 macrophages have a role in bacterial killing and inflammation, whereas M2 macrophages are primarily involved in tissue homeostasis, suppression, inflammatory regression, and tissue healing (Morris, Singer and Lumeng, 2011; Sica and Mantovani, 2012).
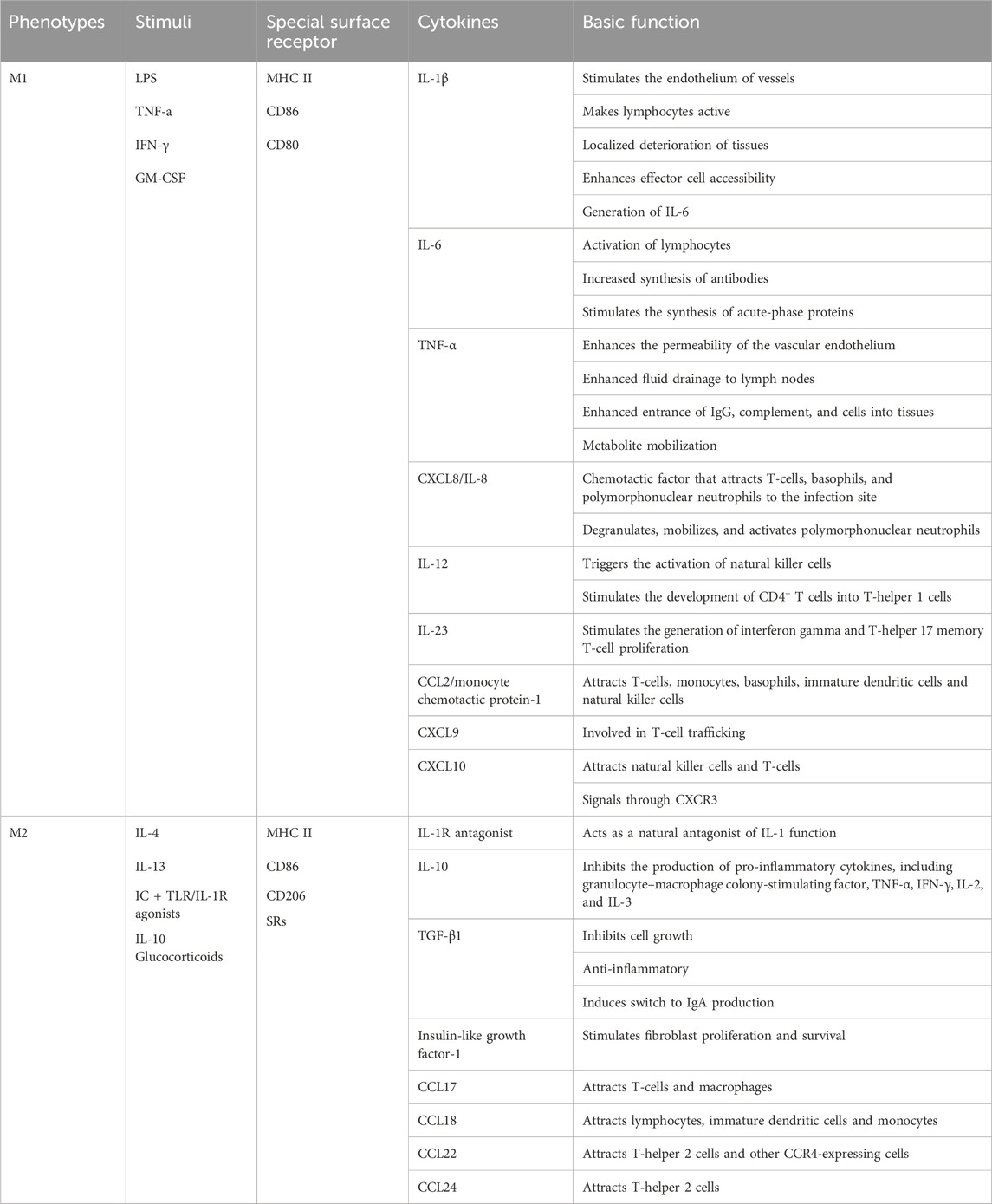
TABLE 1. The polarization types, characteristics and basic functions of macrophages (Sima and Glogauer, 2013; Sun X. et al., 2021).
The development of numerous inflammatory disorders, including infections, obesity, and cancer, is characterized by an imbalanced M1/M2 ratio (Wynn et al., 2013). Obesity, atherosclerosis, diabetes, allergies and asthma, autoimmunity, and cancer are a few examples of chronic diseases that are linked to specific macrophage polarization profiles (Sima and Glogauer, 2013). It has been proven that macrophages can become functionally polarized in vivo, both in healthy and unhealthy circumstances. Pregnancy, embryogenesis, and the preservation of normal conditions in particular tissues (such as the testis and fat tissue) are all included in the former. Included in the latter are cancer, vascular disease, infection, chronic inflammation, tissue healing, and metabolism (Sica and Mantovani, 2012).
3 Polarization of macrophages in periodontitis
As the sixth most common disease in the world, periodontitis is a common condition that affects many people. In its extreme stages, 10% of adult population are affected (Larsson et al., 2022). It is a chronic infectious illness characterized by microbial-related and host-mediated inflammation, which is brought on by the persistent breakdown of supportive periodontal tissues, which is started by plaque biofilm (Tonetti, Greenwell and Kornman, 2018). A considerable number of animal experiments and human studies have shown increased polarization of M1 macrophages in periodontitis (Table 2). In mice infected with Porphyromonas gingivalis (Pg), an animal investigation found that M1 macrophages dramatically expanded in the gingival tissue (Lam et al., 2014). M1 macrophages increased higher than M2 in the periodontitis group compared to the healthy control group, according to research by Yu T et al. on animals. Additionally, periodontal tissue affected by periodontitis showed an increase in the M1 inflammatory factors TNF-α and IL-1β as well as the M2 inflammatory factor IL-10 (Yu et al., 2016). Another human study showed that M1 macrophages increased in periodontitis compared to healthy controls (Higuchi, Sm, Yamashita, Ozaki and Yoshimura, 2020). However, when periodontitis worsens, the macrophage phenotype may alter. M1 is enhanced during the inflammatory phase while macrophage phenotype polarizes towards the M2 type during the recovery stage (Gonzalez et al., 2015; Viniegra et al., 2018; Zhou et al., 2019; Wu X. et al., 2020).
3.1 M1 macrophage polarization in periodontitis
Numerous M1 macrophages are present at the sites of bone degradation in chronic osteolytic disorders, such as various types of arthritis and periodontitis. These macrophages contribute significantly to disease-induced bone resorption by producing inflammatory cytokines including IL-1β and TNF-α and activating osteoclasts (Arend and Dayer, 1990; Stashenko, Jandinski, Fujiyoshi, Rynar and Socransky, 1991; Metzger, 2000; Górska et al., 2003; Andrukhov et al., 2011; Shaddox et al., 2011). Clinical outcomes may be enhanced by antagonist therapy that lowers TNF-α and IL-1β levels (Zwerina, Redlich, Schett and Smolen, 2005; McInnes and Schett, 2007). By employing IL-1β and TNF-α antagonists or knocking down the IL-1 receptor and TNF receptor, alveolar bone resorption in mice with experimental periodontitis was also decreased (Assuma, Oates, Cochran, Amar and Graves, 1998; Graves and Cochran, 2003). Additionally, gingival crevicular fluid IL-1 levels were found to be lower, IL-10 levels were higher, and bone resorption activity was lower when periodontal treatment was effective (Holmlund, Hänström and Lerner, 2004; de Lima Oliveira et al., 2012).
Matrix metalloproteinases (MMPs), which are involved in the breakdown of the extracellular matrix, are just one of the significant proteases that M1 macrophages release in addition to cytokines in the advancement of periodontal disorders (Franco, Patricia, Timo, Claudia and Marcela, 2017). MMPs are produced as a result of the inflammatory cytokines TNF-α, IL-1, and IL-6, all of which are highly expressed in diseased periodontal tissue (Stashenko et al., 1991; Irwin and Myrillas, 1998; Irwin, Myrillas, Traynor, Leadbetter and Cawston, 2002), some of these MMPs are also associated with increased M1/M2 ratios during disease (J. Yang et al., 2018).
3.2 M2 macrophage polarization in periodontitis
Widespread expression of the M2 macrophage’s IL-10 in inflamed periodontal tissue is linked to tissue healing, a reduction in periodontitis severity, and a reduction to inflammation (Lappin, MacLeod, Kerr, Mitchell and Kinane, 2001; Garlet, Martins, Fonseca, Ferreira and Silva, 2004; Garlet, 2010). In IL-10 deficient animals, which were more vulnerable to Pg-induced alveolar bone loss, its protective effect was also demonstrated (Sasaki et al., 2004). Additionally, TGF-β is regarded as one of the most significant cytokines involved in the upkeep of the M2 phenotype, which suppresses the synthesis of endogenous NO (Vodovotz, Bogdan, Paik, Xie and Nathan, 1993), and is crucial for the recruitment of bone marrow mesenchymal stem cells (MSCs) during tissue regeneration (Fu et al., 2019). By releasing IL-4, IL-10, IL-13, and TGF-β throughout the inflammatory process, M2 macrophages counteract the M1 type macrophage response, control inflammation, and aid in tissue repair and wound healing (Mosser and Edwards, 2008; Wynn and Vannella, 2016).
3.3 Potential pathways for macrophage polarization in the etiology of periodontitis
M1 macrophages and Th1/Th17 lymphocytes are more prevalent than M2 macrophages and Th2/Treg lymphocyte subsets in active periodontal diseases compared to both of these cell types (Cavalla and Hernandez, 2022).
Through interactions with other immune cells, it has been demonstrated that macrophage polarization plays a role in the etiology of periodontitis: (1) macrophage-PMN-monocyte crosstalk: during inflammation, M1 macrophages locally recruit PMN to clear pathogens. Monocytes emerge after PMN recruitment and are activated as M2 macrophages to remove apoptotic PMN and other debris; (2) macrophage-lymphocyte crosstalk: M1 type macrophages activated by LPS, TNF-α, and IFN-γ produce IL-23, which stimulates Th17 cell infiltration. An inflammatory amplification loop is created when a Th17 cell releases IL-17 (a pro-inflammatory cytokine that promotes PMN recruitment and activation), IL-1, IL-6, TNF-α, MMPs, and RANKL. The decoy receptor osteoprotegerin and RANKL, a significant pro-osteoclastic mediator, are necessary for the coupling of bone resorption and creation (Sima and Glogauer, 2013).
4 Relationship between implants and dysregulated immune responses in the peri-implant mucosa (PIM)
Animal experiments based on a murine implant model have shown that the titanium implant itself promoted peri-implant inflammation and dysregulated mucosal homeostasis. Langerhans cells, the primary antigen-presenting cells of the oral epithelium, were hampered in their ability to mature, which was a result of the implant’s release of titanium ions. Titanium dental implants disrupted the immunological control of the PIM by impairing the growth of oral Langerhans cells (Heyman et al., 2018).
In peri-implant tissue biopsies, a reduction in inflammatory cell density was seen as healing time increased, so it is thought that the onset and regression of inflammation is a characteristic of PIM healing (Tomasi et al., 2016). This occurrence might be the PIM’s transitional immunological state before it returns to a homeostatic level resembling healthy gingival tissue. However, inflammatory infiltration of the PIM had been reported in implants that did not show clinical signs related to inflammation even 6 months after implant insertion, as found in animal studies (Pongnarisorn et al., 2007). Determining whether the PIM reaches a “normal” steady state, as it does in the gingiva, is therefore uncertain, suggesting the possibility that the PIM develops an alternative immune homeostasis. Given that the peri-implant tissue is more “inflamed” than the normal gingiva based on Th17/Treg homeostasis, this theory could explain why the implant is more susceptible to infection (Heyman et al., 2022). As mentioned previously, M1 macrophages and Th1/Th17 lymphocyte subsets are more prevalent than M2 macrophages and Th2/Treg lymphocyte subsets in active periodontal diseases (Cavalla and Hernandez, 2022). Thus, although the role played by macrophage polarization in the immune dysregulation of peri-implant tissues has not been fully investigated, it can be hypothesized that its role should not be underestimated.
Additionally, utilizing a mouse dental implant model, Heyman et al. discovered that dental implants were able to promote dysbiosis of the oral microbiota and increase inflammation and bone loss in the remote teeth in addition to locally raising inflammation and bone loss. It was not entirely clear which mechanisms induced the promotion of bone loss at the remote site. The Th1 immune response, represented by IFN-γ, may yet be implicated in this process, according to findings of cytokine production and lymphocyte infiltration in the gingiva (Heyman et al., 2020). The possibility of M1 macrophages contributing is also raised by this.
5 Polarization of macrophages in peri-implantitis
Currently, there are only several studies investigating macrophage polarization in PI. No consensus has been reached.
It was reported earlier that the number of M1 macrophages present was similar between the periodontitis and PI groups, although higher than that of healthy controls (Karatas et al., 2020). M1 and M2 expression in PI samples did not show any statistically significant differences (Galarraga-Vinueza, Obreja, Khoury, et al., 2021a).
However, research from the previous 2 years revealed that PI had much more M1 macrophages than periodontitis did. In comparison to periodontitis samples, it was discovered that PI samples showed a much greater degree of inflammatory cell infiltration and a significantly higher number of M1 macrophages (Dionigi et al., 2020; Fretwurst et al., 2020). M2 macrophage counts, however, did not significantly differ between the two illnesses (Fretwurst et al., 2020). M1 macrophage levels were also noticeably greater in advanced PI cases (i.e., radiographic marginal bone loss >50% of implant length, PI severity classification (Monje et al., 2019)), and a significant association between higher M1 macrophage expression and deeper probing depth was found (Galarraga-Vinueza, Obreja, Ramanauskaite, et al., 2021b). Table 3 summarizes the literature related to macrophage polarization in human PI in recent years.
5.1 M1/M2 polarization in regulating osteoclast and osteoblast functions
It is now believed that the large number of macrophages and elevated M1 macrophages observed in PI lesions indicate a strong immune system response to local factors that increase tissue destruction. The histological data in the literature are consistent with the progression of PI disease observed in the clinic (Derks et al., 2016; Fretwurst et al., 2020). The higher expression of M1 macrophages may be associated with a “destructive” inflammatory response and significant peri-implant osteolysis in advanced PI cases (Garlet and Giannobile, 2018; Zhuang et al., 2019; Fretwurst et al., 2020).
Through the secretion of cytokines that activate osteoclast precursors and encourage Th1 responses, M1 contributes to the activation of osteoclasts. Concurrently, M1 contributes to the generation of cytokines that are thought to be important for bone resorption, including PGE2, IL-1β, TNF-α, IL-6, and IL-12. PGE2 is the most potent inducer of periodontal bone resorption among them. It also facilitates a number of detrimental processes in the alveolar bone, including reducing osteoblast viability and mineralization and promoting the development of osteoclasts (Oka et al., 2007; Ruiz-Heiland, Yong, von Bremen and Ruf, 2021). LPS stimulates M1’s expression of IL-1β, and TNF-α and IL-1β together stimulate M1’s synthesis of IL-1β to support osteoclast activation and differentiation (Ruiz-Heiland et al., 2021); TNF-α also causes T cells and B cells to produce RANKL (Becerra-Ruiz, Guerrero-Velázquez, Martínez-Esquivias, Martínez-Pérez and Guzmán-Flores, 2022). Furthermore, IL-6 causes osteoclasts to break down the extracellular matrix and create MMPs, which eventually results in alveolar bone resorption (Figueiredo et al., 2020).
As was previously noted, TGF-β is regarded as one of the key cytokines in the preservation of the M2 phenotype and is crucial for bone marrow MSC recruitment during tissue healing (Fu et al., 2019). M2 also expresses high levels of IL-10 (Zhou et al., 2019), which helps to partially explain its role in the formation of new bone. The excessive effects of IL-10 and IL-4 on the healing process appear to be related to the downregulation of proinflammatory cytokines and MMP as well as the stimulation of osteoblasts. M2 secretes BMP-2, which speeds up osteogenesis (Liang, Wang, Wu and Wang, 2021). To sum up, M2 secretes anti-inflammatory and repair mediators, including TGF-β, IL-4, IL-10, and vascular endothelial growth factor, which in turn suppress proinflammatory cytokines and encourage tissue regeneration and homeostasis restoration. The M2-induced local microenvironment promotes osseointegration and angiogenesis (Park, Silvin, Ginhoux and Merad, 2022).
5.2 Titanium particles and foreign body reactions in peri-implant tissues
The presence of foreign bodies is thought to be strongly associated with PI, and they cause a dysregulated immune response in the peri-implant tissues. These foreign bodies are mainly titanium and dental adhesives (Wilson et al., 2015). Successive studies have reported cases of post-implant titanium allergy or peri-implant mucosal reactive lesions, and metallic-like particles and cells suggestive of allergic reactions, such as eosinophils and PMN, have been observed histologically. Available data suggested that titanium particles were present in more than 90% of PI lesions (Shafizadeh, Amid, Mahmoum and Kadkhodazadeh, 2021). Several in vitro studies have confirmed that microns or nanoparticles of titanium implant alloys may be cytotoxic and enhance pro-inflammatory responses (Okuda-Shimazaki, Takaku, Kanehira, Sonezaki and Taniguchi, 2010; Cai et al., 2011; Irshad et al., 2013; Pettersson et al., 2017). A significant inflammatory reaction was seen in soft tissue biopsies near implants when titanium particles were present (Schlegel, Eppeneder and Wiltfang, 2002; Olmedo et al., 2012; Wilson et al., 2015). There was considerable evidence that debris, titanium ions, and particle shedding could lead to sterile peri-implant inflammation and implant failure (Revell, 2008).
Histological biopsies of human PI samples revealed that M1 macrophages accumulated in areas of increased titanium and iron concentrations (Fretwurst et al., 2016). It has been discovered that titanium particles cause macrophages to react similarly to LPS, and the resulting inflammatory response fuels osteoclast-mediated bone tissue destruction. In vitro and in vivo gene expression, secretome profiling, fluorescence activated cell sorting (FACS), and other analyses on macrophages revealed that M1 polarization occurs in response to titanium particles. However, all of their assays were performed during the early inflammatory phase. Inflammation regression was observed in some tissues in vivo after 6–8 weeks, indicating that M1 and M2 macrophages may be distributed more dynamically and intricately over time (Eger et al., 2018).
To study the impact of various titanium particle sources on macrophage polarization, Eger et al. used a mouse calvarial model (Eger, Sterer, Liron, Kohavi and Gabet, 2017). The findings demonstrated that there was no noticeable difference in M2 macrophage numbers between the experimental and control groups. However, mice exposed to titanium particles produced by machined (M) or sandblasted and acid-etched (SLA) processes had considerably more M1 macrophages (Eger et al., 2018).
In vitro macrophage cultures revealed similar results. TNF-α, IL-1β, and IL-6 mRNA expression in macrophages increased (up to a 3.5-fold rise) when TiO2 particles were added to the culture medium (Ramenzoni, Fluckiger, Attin and Schmidlin, 2021). Titanium ions in physiological solutions induced the release of IL-1β via activating inflammatory vesicles in human macrophages (Pettersson et al., 2017), and all these cytokine profiles were characteristic of M1 polarization.
In conclusion, immune dysregulation can be found in PI. The most common phenomenon is the polarization of macrophages, but related studies are still lacking. The difficulty of creating an animal model is a significant factor in the paucity of data regarding the etiology of PI. PI lesion tissue is not easily available may also be responsible for it. In addition, Regarding the indicators of M1/M2 polarization, there is currently no definite agreement in the macrophage polarization literature (Fretwurst et al., 2020). Research is still needed in the area of choosing more precise molecular markers to distinguish M1/M2 macrophages (Galarraga-Vinueza, Obreja, Ramanauskaite, et al., 2021a).
6 Immunoregulatory therapy for peri-implantitis linked to polarized macrophages
One should not undervalue the role that macrophage polarization plays in the clinical management of periodontitis and PI. It is currently thought that the major goal of macrophage polarization therapy is to get macrophages to polarize toward the M2 macrophages in order to reduce inflammation, encourage tissue repair, and produce anti-inflammatory benefits (Sun et al., 2021; Whitaker, Hernaez-Estrada, Hernandez, Santos-Vizcaino and Spiller, 2021). Promoting macrophage polarization from M1-type to M2-type by immunomodulatory therapy to promote bone regeneration has been successfully attempted in diabetic fracture healing models, and in bone-related diseases including osteoarthritis (OA), osteoporosis (OP), and bone defects (Whitaker et al., 2021; Wang et al., 2023). Macrophage polarization immunomodulatory therapy for periodontitis is currently a hot topic, but those therapies regarding PI are currently rare.
The current literature on regulating macrophage polarization as a therapeutic target for periodontal disease can be summarized as follows: (1) anti-cytokine therapy: when anti-TNF-α therapy was used in combination with mechanical debridement, periodontal parameters showed a tendency of improvement (Pers, Saraux, Pierre and Youinou, 2008; Mayer, Balbir-Gurman and Machtei, 2009; Ortiz et al., 2009). Therapeutic blocking of IL-1 receptors dramatically reduced local inflammatory cell infiltration, osteoclast activation, and bone resorption in an animal model of periodontitis (Assuma et al., 1998; Delima et al., 2001); (2) pharmacological treatment: when used systemically, rosiglitazone inhibited bone resorption during inflammation, increased bone regeneration during the repair of periodontitis, and polarized macrophages toward the M2 macrophages (Di Paola et al., 2006; Hassumi et al., 2009; Viniegra et al., 2018). Other drugs that affect macrophage polarization include PPARγ agonists (thiazolidinediones) (Charo, 2007; Stienstra et al., 2008; Lu et al., 2011), zoledronic acid, statins (Fujita et al., 2010), trabectedin (Germano et al., 2010); (3) cell therapy: isolated polarized M2 macrophages had the potential to initiate the regression of periodontal disease inflammation (Sima and Glogauer, 2013); (4) gene knockout: It has been shown that local injection of AKT inhibitors decreased the M1/M2 ratio and reduced alveolar bone resorption in mice with periodontitis, and that in vitro knockdown of Akt2 hindered M1 polarization and enhanced M2 polarization (Zhuang et al., 2019; Wu et al., 2020). The polarization of M1 macrophages was also decreased by TET1 knockdown because it prevented the NF-κB signaling pathway from being activated (Huang, Tian, Li and Xu, 2019).
Surface modification of titanium may influence macrophage polarization (Figure 2). Successfully synthesising IL-23R non-competitive antagonist nanocoatings on titanium surfaces, Pizarek et al. discovered that the coatings inhibited the IL-23/17A pathway, which is a source of inflammation, and polarized macrophages to the M2 phenotype in vitro cellular studies (Pizarek, Fischer and Aparicio, 2023). In a recent study, it was discovered that by modifying macrophage polarization, a surface modification technique using peptide coatings might reduce chronic inflammation and further increase osseointegration around the implant material (Bai et al., 2020). By interfering with integrin-α2β1 and integrin-αvβ3, peptide-modified titanium implants might successfully reduce peri-implant inflammation in wear particle models and induce macrophage polarization to a pro-healing M2 phenotype. With the use of tetravalent 3,4-dihydroxy-L-phenylalanine (DOPA) and Arg-Gly-Asp (RGD) sequences, this catecholic peptide with mussel-inspired structure was created. The mussel adhesion mechanism allowed for the easy apposition of this peptide to the surface of medical titanium materials, enhancing osteoblast adherence and fostering osteogenesis of titanium implants even under inflammatory circumstances (Guo et al., 2022).
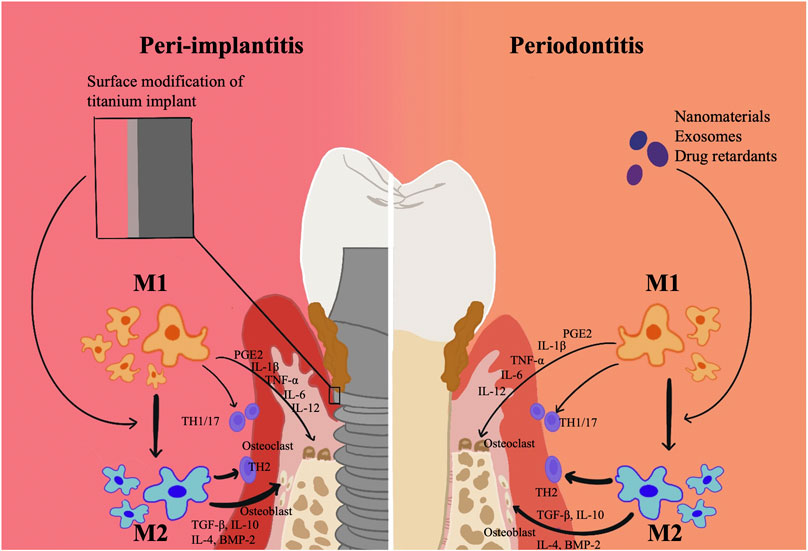
FIGURE 2. Surface modification technique of titanium related to Macrophage polarization in periodontitis and peri-implantitis. Surface modification of titanium may influence macrophage polarization. By modifying macrophage polarization, surface modification technique might reduce chronic inflammation and further increase osseointegration around the implant material.
In addition, MSC therapy has great potential in preventing and treating peri-implantitis. Li et al. used a hydrogel loaded with gingival-derived MSCs and injected it into the peri-implant area of a rat model of early implant placement and found that it was effective in improving epithelial closure around the implant and promoting M2 macrophage polarization. This would aid in preventing the growth of peri-implantitis (Li et al., 2023). However, the precise molecular processes and signaling pathways of interactions between epithelial cells and macrophages have not yet been clarified.
Nevertheless, the majority of inflammatory disease treatments, including those for periodontitis, are palliative and only offer temporary relief. The idea of immunomodulatory nanosystems (IMNs) may be able to solve this issue (Ahamad et al., 2021). The main IMNs for macrophage polarization-associated periodontitis include nanomaterials, exosomes, and periodontal drug retardants (Sun et al., 2021). Table 4 summarizes the immunomodulatory treatment strategies for macrophage polarization-associated periodontitis in recent years. Possible therapeutic strategies related to macrophage polarization in PI are less studied, but the current research in the periodontal field may provide some new directions for future research.
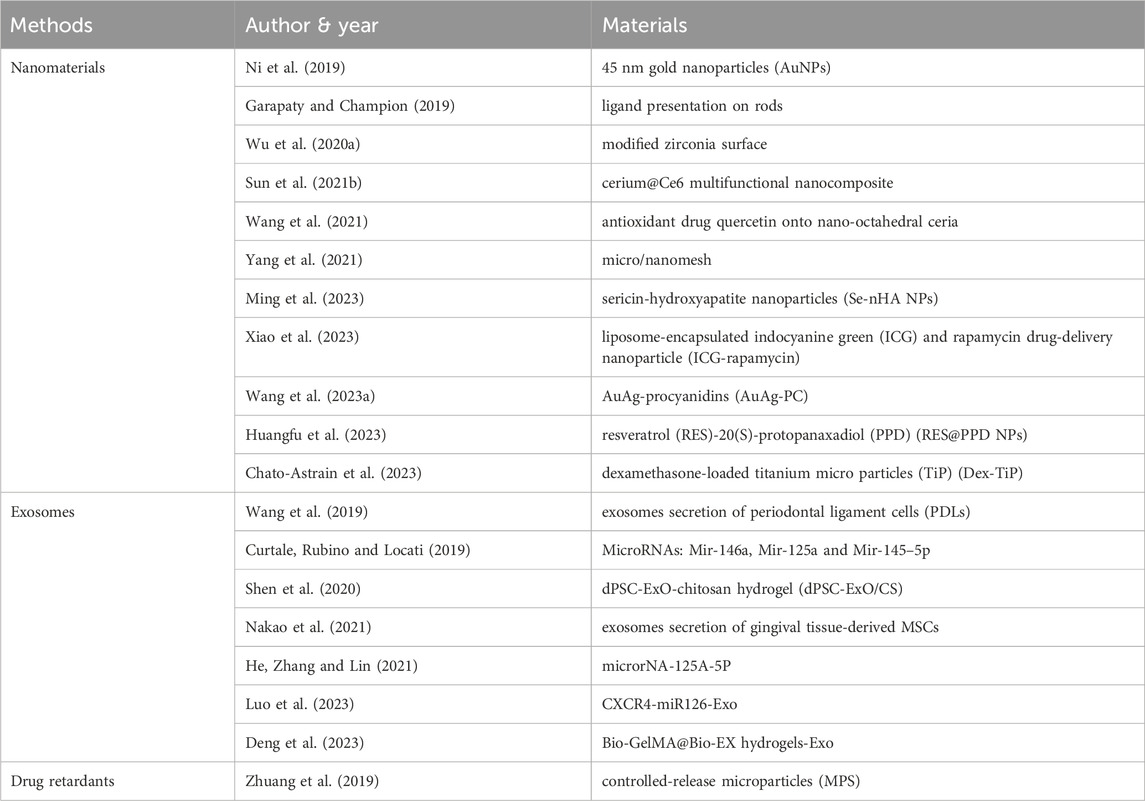
TABLE 4. Materials used in Immunomodulatory therapies related to Macrophage polarization in periodontitis.
7 Conclusion
Animal experiments based on a murine implant model have shown that the titanium implant itself promoted peri-implant inflammation and dysregulated mucosal homeostasis. Titanium ions that were released from the implant acted as a mediator in this process. It is currently thought that the onset and resolution of inflammation is a characteristic of PIM healing, but it is unclear whether the PIM achieves a “normal” stable state as in the gingiva, suggesting the possibility that the PIM develops alternative immune homeostasis. The available data indicate that macrophage polarization plays a significant role in the dysregulation of peri-implant immunity, despite the fact that the mechanisms behind this dysregulation are not fully understood.
Macrophage polarization has a complex and extensive variety of roles, with M2 macrophages primarily involved in tissue homeostasis, suppression and regression of inflammation, and tissue repair, and M1 macrophages promoting bacterial death and increasing inflammation. Although studies related to macrophage polarization in PI are not sufficiently thorough, the available literature suggests that the higher expression of M1 macrophages in PI compared to periodontitis may be associated with a “destructive” inflammatory response and significant peri-implant osteolysis in patients diagnosed with advanced PI. Furthermore, macrophage polarization toward the M1 phenotype may be caused by micron- or nano-sized particles of typical titanium implant alloys.
The ability to control immune homeostasis has been tentatively shown in some studies to be a promising therapeutic strategy. This is accomplished by carefully examining the mechanisms of action of various cytokines and mediators that regulate macrophage polarization and by controlling the ratio of macrophages with different polarization phenotypes to achieve a good balance between immune defense and tissue homeostasis.
However, the need for an experimental model and an unambiguous agreement on the markers to distinguish M1 from M2 polarization, which permits careful examination of this crucial issue, still exists. For further research, more PI lesion tissue needs to be gathered. The treatment options for PI macrophage polarization are few and will likely require more research in the future.
Author contributions
YL: Methodology, Writing–original draft. XL: Methodology, Writing–original draft. DG: Writing–review and editing. LM: Writing–review and editing. XF: Writing–review and editing. YZ: Writing–review and editing. SP: Conceptualization, Funding acquisition, Methodology, Writing–original draft, Writing–review and editing.
Funding
The author(s) declare financial support was received for the research, authorship, and/or publication of this article. This work was supported by the National Key R&D Program of China (2020YFC2009000, Project No. 2020YFC2009005), and supported by Beijing Natural Science Foundation (No. 7222228), and by the National Program for Multidisciplinary Cooperative Treatment on Major Diseases (PKUSSNMP-202004).
Conflict of interest
The authors declare that the research was conducted in the absence of any commercial or financial relationships that could be construed as a potential conflict of interest.
Publisher’s note
All claims expressed in this article are solely those of the authors and do not necessarily represent those of their affiliated organizations, or those of the publisher, the editors and the reviewers. Any product that may be evaluated in this article, or claim that may be made by its manufacturer, is not guaranteed or endorsed by the publisher.
References
Ahamad, N., Kar, A., Mehta, S., Dewani, M., Ravichandran, V., Bhardwaj, P., et al. (2021). Immunomodulatory nanosystems for treating inflammatory diseases. Biomaterials 274, 120875. doi:10.1016/j.biomaterials.2021.120875
Ahmad, I., Naqvi, R. A., Valverde, A., and Naqvi, A. R. (2023). LncRNA MALAT1/microRNA-30b axis regulates macrophage polarization and function. Front. Immunol. 14, 1214810. doi:10.3389/fimmu.2023.1214810
Albrektsson, T., Brånemark, P. I., Hansson, H. A., and Lindström, J. (1981). Osseointegrated titanium implants. Requirements for ensuring a long-lasting, direct bone-to-implant anchorage in man. Acta Orthop. Scand. 52 (2), 155–170. doi:10.3109/17453678108991776
Andrukhov, O., Ulm, C., Reischl, H., Nguyen, P. Q., Matejka, M., and Rausch-Fan, X. (2011). Serum cytokine levels in periodontitis patients in relation to the bacterial load. J. Periodontol. 82 (6), 885–892. doi:10.1902/jop.2010.100425
Arend, W. P., and Dayer, J. M. (1990). Cytokines and cytokine inhibitors or antagonists in rheumatoid arthritis. Arthritis Rheum. 33 (3), 305–315. doi:10.1002/art.1780330302
Assuma, R., Oates, T., Cochran, D., Amar, S., and Graves, D. T. (1998). IL-1 and TNF antagonists inhibit the inflammatory response and bone loss in experimental periodontitis. J. Immunol. 160 (1), 403–409. doi:10.4049/jimmunol.160.1.403
Bai, J., Wang, H., Chen, H., Ge, G., Wang, M., Gao, A., et al. (2020). Biomimetic osteogenic peptide with mussel adhesion and osteoimmunomodulatory functions to ameliorate interfacial osseointegration under chronic inflammation. Biomaterials 255, 120197. doi:10.1016/j.biomaterials.2020.120197
Becerra-Ruiz, J. S., Guerrero-Velázquez, C., Martínez-Esquivias, F., Martínez-Pérez, L. A., and Guzmán-Flores, J. M. (2022). Innate and adaptive immunity of periodontal disease. From etiology to alveolar bone loss. Oral Dis. 28 (6), 1441–1447. doi:10.1111/odi.13884
Benoit, M., Desnues, B., and Mege, J. L. (2008). Macrophage polarization in bacterial infections. J. Immunol. 181 (6), 3733–3739. doi:10.4049/jimmunol.181.6.3733
Berglundh, T., Armitage, G., Araujo, M. G., Avila-Ortiz, G., Blanco, J., Camargo, P. M., et al. (2018). Peri-implant diseases and conditions: consensus report of workgroup 4 of the 2017 world Workshop on the classification of periodontal and peri-implant diseases and conditions. J. Periodontol. 89 (1), S313–S318. doi:10.1002/JPER.17-0739
Berglundh, T., Gislason, O., Lekholm, U., Sennerby, L., and Lindhe, J. (2004). Histopathological observations of human periimplantitis lesions. J. Clin. Periodontol. 31 (5), 341–347. doi:10.1111/j.1600-051X.2004.00486.x
Berglundh, T., Zitzmann, N. U., and Donati, M. (2011). Are peri-implantitis lesions different from periodontitis lesions? J. Clin. Periodontol. 38 (11), 188–202. doi:10.1111/j.1600-051X.2010.01672.x
Brånemark, P. I., Adell, R., Breine, U., Hansson, B. O., Lindström, J., and Ohlsson, A. (1969). Intra-osseous anchorage of dental prostheses. I. Experimental studies. Scand. J. Plast. Reconstr. Surg. 3 (2), 81–100. doi:10.3109/02844316909036699
Brånemark, P. I., Hansson, B. O., Adell, R., Breine, U., Lindström, J., Hallén, O., et al. (1977). Osseointegrated implants in the treatment of the edentulous jaw. Experience from a 10-year period. Scand. J. Plast. Reconstr. Surg. Suppl. 16, 1–132.
Cai, K., Hou, Y., Hu, Y., Zhao, L., Luo, Z., Shi, Y., et al. (2011). Correlation of the cytotoxicity of TiO2 nanoparticles with different particle sizes on a sub-200-nm scale. Small 7 (21), 3026–3031. doi:10.1002/smll.201101170
Carcuac, O., and Berglundh, T. (2014). Composition of human peri-implantitis and periodontitis lesions. J. Dent. Res. 93 (11), 1083–1088. doi:10.1177/0022034514551754
Cavalla, F., and Hernandez, M. (2022). Polarization profiles of T lymphocytes and macrophages responses in periodontitis. Adv. Exp. Med. Biol. 1373, 195–208. doi:10.1007/978-3-030-96881-6_10
Charo, I. F. (2007). Macrophage polarization and insulin resistance: PPARγ in control. Cell Metab. 6 (2), 96–98. doi:10.1016/j.cmet.2007.07.006
Chato-Astrain, J., Toledano-Osorio, M., Alaminos, M., Toledano, M., Sanz, M., and Osorio, R. (2023). Effect of functionalized titanium particles with dexamethasone-loaded nanospheres on macrophage polarization and activity. Dent. Mater 40, 66–79. doi:10.1016/j.dental.2023.10.023
Curtale, G., Rubino, M., and Locati, M. (2019). MicroRNAs as molecular switches in macrophage activation. Front. Immunol. 10, 799. doi:10.3389/fimmu.2019.00799
Delima, A. J., Oates, T., Assuma, R., Schwartz, Z., Cochran, D., Amar, S., et al. (2001). Soluble antagonists to interleukin-1 (IL-1) and tumor necrosis factor (TNF) inhibits loss of tissue attachment in experimental periodontitis. J. Clin. Periodontol. 28 (3), 233–240. doi:10.1034/j.1600-051x.2001.028003233.x
de Lima Oliveira, A. P., de Faveri, M., Gursky, L. C., Mestnik, M. J., Feres, M., Haffajee, A. D., et al. (2012). Effects of periodontal therapy on GCF cytokines in generalized aggressive periodontitis subjects. J. Clin. Periodontol. 39 (3), 295–302. doi:10.1111/j.1600-051X.2011.01817.x
Deng, D., Li, X., Zhang, J. J., Yin, Y., Tian, Y., Gan, D., et al. (2023). Biotin-avidin system-based delivery enhances the therapeutic performance of MSC-derived exosomes. ACS Nano 17 (9), 8530–8550. doi:10.1021/acsnano.3c00839
Derks, J., Schaller, D., Håkansson, J., Wennström, J. L., Tomasi, C., and Berglundh, T. (2016). Peri-implantitis - onset and pattern of progression. J. Clin. Periodontol. 43 (4), 383–388. doi:10.1111/jcpe.12535
Dionigi, C., Larsson, L., Carcuac, O., and Berglundh, T. (2020). Cellular expression of DNA damage/repair and reactive oxygen/nitrogen species in human periodontitis and peri-implantitis lesions. J. Clin. Periodontol. 47 (12), 1466–1475. doi:10.1111/jcpe.13370
Di Paola, R., Mazzon, E., Maiere, D., Zito, D., Britti, D., De Majo, M., et al. (2006). Rosiglitazone reduces the evolution of experimental periodontitis in the rat. J. Dent. Res. 85 (2), 156–161. doi:10.1177/154405910608500208
Eger, M., Hiram-Bab, S., Liron, T., Sterer, N., Carmi, Y., Kohavi, D., et al. (2018). Mechanism and prevention of titanium particle-induced inflammation and osteolysis. Front. Immunol. 9, 2963. doi:10.3389/fimmu.2018.02963
Eger, M., Sterer, N., Liron, T., Kohavi, D., and Gabet, Y. (2017). Scaling of titanium implants entrains inflammation-induced osteolysis. Sci. Rep. 7, 39612. doi:10.1038/srep39612
Esposito, M., Thomsen, P., Mölne, J., Gretzer, C., Ericson, L. E., and Lekholm, U. (1997). Immunohistochemistry of soft tissues surrounding late failures of Brånemark implants. Clin. Oral Implants Res. 8 (5), 352–366. doi:10.1034/j.1600-0501.1997.080502.x
Figueiredo, L. C., Bueno-Silva, B., Nogueira, C. F. P., Valadares, L. C., Garcia, K. M. M., Filho, G., et al. (2020). Levels of gene expression of immunological biomarkers in peri-implant and periodontal tissues. Int. J. Environ. Res. Public Health 17 (23), 9100. doi:10.3390/ijerph17239100
Franco, C., Patricia, H. R., Timo, S., Claudia, B., and Marcela, H. (2017). Matrix metalloproteinases as regulators of periodontal inflammation. Int. J. Mol. Sci. 18 (2), 440. doi:10.3390/ijms18020440
Fransson, C., Lekholm, U., Jemt, T., and Berglundh, T. (2005). Prevalence of subjects with progressive bone loss at implants. Clin. Oral Implants Res. 16 (4), 440–446. doi:10.1111/j.1600-0501.2005.01137.x
French, D., Ofec, R., and Levin, L. (2021). Long term clinical performance of 10 871 dental implants with up to 22 years of follow-up: a cohort study in 4247 patients. Clin. Implant Dent. Relat. Res. 23 (3), 289–297. doi:10.1111/cid.12994
Fretwurst, T., Buzanich, G., Nahles, S., Woelber, J. P., Riesemeier, H., and Nelson, K. (2016). Metal elements in tissue with dental peri-implantitis: a pilot study. Clin. Oral Implants Res. 27 (9), 1178–1186. doi:10.1111/clr.12718
Fretwurst, T., Garaicoa-Pazmino, C., Nelson, K., Giannobile, W. V., Squarize, C. H., Larsson, L., et al. (2020). Characterization of macrophages infiltrating peri-implantitis lesions. Clin. Oral Implants Res. 31 (3), 274–281. doi:10.1111/clr.13568
Fu, X., Liu, G., Halim, A., Ju, Y., Luo, Q., and Song, A. G. (2019). Mesenchymal stem cell migration and tissue repair. Cells 8 (8), 784. doi:10.3390/cells8080784
Fujita, E., Shimizu, A., Masuda, Y., Kuwahara, N., Arai, T., Nagasaka, S., et al. (2010). Statin attenuates experimental anti-glomerular basement membrane glomerulonephritis together with the augmentation of alternatively activated macrophages. Am. J. Pathol. 177 (3), 1143–1154. doi:10.2353/ajpath.2010.090608
Galarraga-Vinueza, M. E., Obreja, K., Khoury, C., Begic, A., Ramanauskaite, A., Sculean, A., et al. (2021a). Influence of macrophage polarization on the effectiveness of surgical therapy of peri-implantitis. Int. J. Implant Dent. 7 (1), 110. doi:10.1186/s40729-021-00391-2
Galarraga-Vinueza, M. E., Obreja, K., Ramanauskaite, A., Magini, R., Begic, A., Sader, R., et al. (2021b). Macrophage polarization in peri-implantitis lesions. Clin. Oral Investig. 25 (4), 2335–2344. doi:10.1007/s00784-020-03556-2
Garapaty, A., and Champion, J. A. (2019). Shape of ligand immobilized particles dominates and amplifies the macrophage cytokine response to ligands. PLoS One 14 (5), e0217022. doi:10.1371/journal.pone.0217022
Garlet, G. P. (2010). Destructive and protective roles of cytokines in periodontitis: a re-appraisal from host defense and tissue destruction viewpoints. J. Dent. Res. 89 (12), 1349–1363. doi:10.1177/0022034510376402
Garlet, G. P., and Giannobile, W. V. (2018). Macrophages: the bridge between inflammation resolution and tissue repair? J. Dent. Res. 97 (10), 1079–1081. doi:10.1177/0022034518785857
Garlet, G. P., Martins, W., Fonseca, B. A., Ferreira, B. R., and Silva, J. S. (2004). Matrix metalloproteinases, their physiological inhibitors and osteoclast factors are differentially regulated by the cytokine profile in human periodontal disease. J. Clin. Periodontol. 31 (8), 671–679. doi:10.1111/j.1600-051X.2004.00545.x
Germano, G., Frapolli, R., Simone, M., Tavecchio, M., Erba, E., Pesce, S., et al. (2010). Antitumor and anti-inflammatory effects of trabectedin on human myxoid liposarcoma cells. Cancer Res. 70 (6), 2235–2244. doi:10.1158/0008-5472.Can-09-2335
Gonzalez, O. A., Novak, M. J., Kirakodu, S., Stromberg, A., Nagarajan, R., Huang, C. B., et al. (2015). Differential gene expression profiles reflecting macrophage polarization in aging and periodontitis gingival tissues. Immunol. Invest. 44 (7), 643–664. doi:10.3109/08820139.2015.1070269
Gordon, S., and Martinez, F. O. (2010). Alternative activation of macrophages: mechanism and functions. Immunity 32 (5), 593–604. doi:10.1016/j.immuni.2010.05.007
Górska, R., Gregorek, H., Kowalski, J., Laskus-Perendyk, A., Syczewska, M., and Madaliński, K. (2003). Relationship between clinical parameters and cytokine profiles in inflamed gingival tissue and serum samples from patients with chronic periodontitis. J. Clin. Periodontol. 30 (12), 1046–1052. doi:10.1046/j.0303-6979.2003.00425.x
Graves, D. T., and Cochran, D. (2003). The contribution of interleukin-1 and tumor necrosis factor to periodontal tissue destruction. J. Periodontol. 74 (3), 391–401. doi:10.1902/jop.2003.74.3.391
Gualini, F., and Berglundh, T. (2003). Immunohistochemical characteristics of inflammatory lesions at implants. J. Clin. Periodontol. 30 (1), 14–18. doi:10.1034/j.1600-051x.2003.300103.x
Guo, X., Bai, J., Ge, G., Wang, Z., Wang, Q., Zheng, K., et al. (2022). Bioinspired peptide adhesion on Ti implants alleviates wear particle-induced inflammation and improves interfacial osteogenesis. J. Colloid Interface Sci. 605, 410–424. doi:10.1016/j.jcis.2021.07.079
Hassumi, M. Y., Silva-Filho, V. J., Campos-Júnior, J. C., Vieira, S. M., Cunha, F. Q., Alves, P. M., et al. (2009). PPAR-gamma agonist rosiglitazone prevents inflammatory periodontal bone loss by inhibiting osteoclastogenesis. Int. Immunopharmacol. 9 (10), 1150–1158. doi:10.1016/j.intimp.2009.06.002
He, W., Zhang, N., and Lin, Z. (2021). MicroRNA-125a-5p modulates macrophage polarization by targeting E26 transformation-specific variant 6 gene during orthodontic tooth movement. Arch. Oral Biol. 124, 105060. doi:10.1016/j.archoralbio.2021.105060
Heyman, O., Horev, Y., Koren, N., Barel, O., Aizenbud, I., Aizenbud, Y., et al. (2020). Niche specific microbiota-dependent and independent bone loss around dental implants and teeth. J. Dent. Res. 99 (9), 1092–1101. doi:10.1177/0022034520920577
Heyman, O., Horev, Y., Mizraji, G., Haviv, Y., Shapira, L., and Wilensky, A. (2022). Excessive inflammatory response to infection in experimental peri-implantitis: resolution by Resolvin D2. J. Clin. Periodontol. 49, 1217–1228. doi:10.1111/jcpe.13631
Heyman, O., Koren, N., Mizraji, G., Capucha, T., Wald, S., Nassar, M., et al. (2018). Impaired differentiation of Langerhans cells in the murine oral epithelium adjacent to titanium dental implants. Front. Immunol. 9, 1712. doi:10.3389/fimmu.2018.01712
Higuchi, K., Sm, Z., Yamashita, Y., Ozaki, Y., and Yoshimura, A. (2020). Initial periodontal treatment affects nucleotide-binding domain leucine-rich repeat-containing protein 3 inflammasome priming in peripheral blood mononuclear cells. Arch. Oral Biol. 110, 104625. doi:10.1016/j.archoralbio.2019.104625
Holmlund, A., Hänström, L., and Lerner, U. H. (2004). Bone resorbing activity and cytokine levels in gingival crevicular fluid before and after treatment of periodontal disease. J. Clin. Periodontol. 31 (6), 475–482. doi:10.1111/j.1600-051X.2004.00504.x
Huang, Y., Tian, C., Li, Q., and Xu, Q. (2019). TET1 knockdown inhibits Porphyromonas gingivalis LPS/IFN-γ-Induced M1 macrophage polarization through the NF-κB pathway in THP-1 cells. Int. J. Mol. Sci. 20 (8), 2023. doi:10.3390/ijms20082023
Huangfu, H., Du, S., Zhang, H., Wang, H., Zhang, Y., Yang, Z., et al. (2023). Facile engineering of resveratrol nanoparticles loaded with 20(S)-protopanaxadiol for the treatment of periodontitis by regulating the macrophage phenotype. Nanoscale 15 (17), 7894–7908. doi:10.1039/d2nr06452a
Irshad, M., Scheres, N., Crielaard, W., Loos, B. G., Wismeijer, D., and Laine, M. L. (2013). Influence of titanium on in vitro fibroblast-Porphyromonas gingivalis interaction in peri-implantitis. J. Clin. Periodontol. 40 (9), 841–849. doi:10.1111/jcpe.12136
Irwin, C. R., and Myrillas, T. T. (1998). The role of IL-6 in the pathogenesis of periodontal disease. Oral Dis. 4 (1), 43–47. doi:10.1111/j.1601-0825.1998.tb00255.x
Irwin, C. R., Myrillas, T. T., Traynor, P., Leadbetter, N., and Cawston, T. E. (2002). The role of soluble interleukin (IL)-6 receptor in mediating the effects of IL-6 on matrix metalloproteinase-1 and tissue inhibitor of metalloproteinase-1 expression by gingival fibroblasts. J. Periodontol. 73 (7), 741–747. doi:10.1902/jop.2002.73.7.741
Karatas, O., Balci Yuce, H., Taskan, M. M., Gevrek, F., Lafci, E., and Kasap, H. (2020). Histological evaluation of peri-implant mucosal and gingival tissues in peri-implantitis, peri-implant mucositis and periodontitis patients: a cross-sectional clinical study. Acta Odontol. Scand. 78 (4), 241–249. doi:10.1080/00016357.2019.1691256
Koldsland, O. C., Scheie, A. A., and Aass, A. M. (2010). Prevalence of peri-implantitis related to severity of the disease with different degrees of bone loss. J. Periodontol. 81 (2), 231–238. doi:10.1902/jop.2009.090269
Koutouzis, T., Eastman, C., Chukkapalli, S., Larjava, H., and Kesavalu, L. (2017). A novel rat model of polymicrobial peri-implantitis: a preliminary study. J. Periodontol. 88 (2), e32–e41. doi:10.1902/jop.2016.160273
Kumar, P. S., Mason, M. R., Brooker, M. R., and O’Brien, K. (2012). Pyrosequencing reveals unique microbial signatures associated with healthy and failing dental implants. J. Clin. Periodontol. 39 (5), 425–433. doi:10.1111/j.1600-051X.2012.01856.x
Lam, R. S., O'Brien-Simpson, N. M., Lenzo, J. C., Holden, J. A., Brammar, G. C., Walsh, K. A., et al. (2014). Macrophage depletion abates Porphyromonas gingivalis-induced alveolar bone resorption in mice. J. Immunol. 193 (5), 2349–2362. doi:10.4049/jimmunol.1400853
Lappin, D. F., MacLeod, C. P., Kerr, A., Mitchell, T., and Kinane, D. F. (2001). Anti-inflammatory cytokine IL-10 and T cell cytokine profile in periodontitis granulation tissue. Clin. Exp. Immunol. 123 (2), 294–300. doi:10.1046/j.1365-2249.2001.01448.x
Larsson, L., Kavanagh, N. M., Nguyen, T. V. N., Castilho, R. M., Berglundh, T., and Giannobile, W. V. (2022). Influence of epigenetics on periodontitis and peri-implantitis pathogenesis. Periodontol 90, 125–137. doi:10.1111/prd.12453
Li, J., Liu, Y., Lai, W., Song, L., Deng, J., Li, C., et al. (2023a). MicroRNA-126 regulates macrophage polarization to prevent the resorption of alveolar bone in diabetic periodontitis. Arch. Oral Biol. 150, 105686. doi:10.1016/j.archoralbio.2023.105686
Li, Y., Zhang, J., Wang, C., Jiang, Z., Lai, K., Wang, Y., et al. (2023b). Porous composite hydrogels with improved MSC survival for robust epithelial sealing around implants and M2 macrophage polarization. Acta Biomater. 157, 108–123. doi:10.1016/j.actbio.2022.11.029
Liang, B., Wang, H., Wu, D., and Wang, Z. (2021). Macrophage M1/M2 polarization dynamically adapts to changes in microenvironment and modulates alveolar bone remodeling after dental implantation. J. Leukoc. Biol. 110 (3), 433–447. doi:10.1002/jlb.1ma0121-001r
Lu, M., Sarruf, D. A., Talukdar, S., Sharma, S., Li, P., Bandyopadhyay, G., et al. (2011). Brain PPAR-γ promotes obesity and is required for the insulin-sensitizing effect of thiazolidinediones. Nat. Med. 17 (5), 618–622. doi:10.1038/nm.2332
Luo, H., Chen, D., Li, R., Li, R., Teng, Y., Cao, Y., et al. (2023). Genetically engineered CXCR4-modified exosomes for delivery of miR-126 mimics to macrophages alleviate periodontitis. J. Nanobiotechnology 21 (1), 116. doi:10.1186/s12951-023-01863-w
Martinez, F. O., Sica, A., Mantovani, A., and Locati, M. (2008). Macrophage activation and polarization. Front. Biosci. 13, 453–461. doi:10.2741/2692
Mayer, Y., Balbir-Gurman, A., and Machtei, E. E. (2009). Anti-tumor necrosis factor-alpha therapy and periodontal parameters in patients with rheumatoid arthritis. J. Periodontol. 80 (9), 1414–1420. doi:10.1902/jop.2009.090015
McInnes, I. B., and Schett, G. (2007). Cytokines in the pathogenesis of rheumatoid arthritis. Nat. Rev. Immunol. 7 (6), 429–442. doi:10.1038/nri2094
Metzger, Z. (2000). Macrophages in periapical lesions. Endod. Dent. Traumatol. 16 (1), 1–8. doi:10.1034/j.1600-9657.2000.016001001.x
Ming, P., Liu, Y., Yu, P., Jiang, X., Yuan, L., Cai, S., et al. (2023). A biomimetic Se-nHA/PC composite microsphere with synergistic immunomodulatory and osteogenic ability to activate bone regeneration in periodontitis. Small, e2305490. doi:10.1002/smll.202305490
Monje, A., Pons, R., Insua, A., Nart, J., Wang, H. L., and Schwarz, F. (2019). Morphology and severity of peri-implantitis bone defects. Clin. Implant Dent. Relat. Res. 21 (4), 635–643. doi:10.1111/cid.12791
Morris, D. L., Singer, K., and Lumeng, C. N. (2011). Adipose tissue macrophages: phenotypic plasticity and diversity in lean and obese states. Curr. Opin. Clin. Nutr. Metab. Care 14 (4), 341–346. doi:10.1097/MCO.0b013e328347970b
Mosser, D. M., and Edwards, J. P. (2008). Exploring the full spectrum of macrophage activation. Nat. Rev. Immunol. 8 (12), 958–969. doi:10.1038/nri2448
Nakao, Y., Fukuda, T., Zhang, Q., Sanui, T., Shinjo, T., Kou, X., et al. (2021). Exosomes from TNF-α-treated human gingiva-derived MSCs enhance M2 macrophage polarization and inhibit periodontal bone loss. Acta Biomater. 122, 306–324. doi:10.1016/j.actbio.2020.12.046
Ni, C., Zhou, J., Kong, N., Bian, T., Zhang, Y., Huang, X., et al. (2019). Gold nanoparticles modulate the crosstalk between macrophages and periodontal ligament cells for periodontitis treatment. Biomaterials 206, 115–132. doi:10.1016/j.biomaterials.2019.03.039
Obreja, K., Ramanauskaite, A., Begic, A., Galarraga-Vinueza, M. E., Parvini, P., Sader, R., et al. (2021). The prevalence of peri-implant diseases around subcrestally placed implants: a cross-sectional study. Clin. Oral Implants Res. 32 (6), 702–710. doi:10.1111/clr.13739
Oka, H., Miyauchi, M., Sakamoto, K., Moriwaki, S., Niida, S., Noguchi, K., et al. (2007). PGE2 activates cementoclastogenesis by cementoblasts via EP4. J. Dent. Res. 86 (10), 974–979. doi:10.1177/154405910708601011
Okuda-Shimazaki, J., Takaku, S., Kanehira, K., Sonezaki, S., and Taniguchi, A. (2010). Effects of titanium dioxide nanoparticle aggregate size on gene expression. Int. J. Mol. Sci. 11 (6), 2383–2392. doi:10.3390/ijms11062383
Olmedo, D. G., Paparella, M. L., Spielberg, M., Brandizzi, D., Guglielmotti, M. B., and Cabrini, R. L. (2012). Oral mucosa tissue response to titanium cover screws. J. Periodontol. 83 (8), 973–980. doi:10.1902/jop.2011.110392
Ortiz, P., Bissada, N. F., Palomo, L., Han, Y. W., Al-Zahrani, M. S., Panneerselvam, A., et al. (2009). Periodontal therapy reduces the severity of active rheumatoid arthritis in patients treated with or without tumor necrosis factor inhibitors. J. Periodontol. 80 (4), 535–540. doi:10.1902/jop.2009.080447
Palevski, D., Levin-Kotler, L. P., Kain, D., Naftali-Shani, N., Landa, N., Ben-Mordechai, T., et al. (2017). Loss of macrophage wnt secretion improves remodeling and function after myocardial infarction in mice. J. Am. Heart Assoc. 6 (1), e004387. doi:10.1161/jaha.116.004387
Park, M. D., Silvin, A., Ginhoux, F., and Merad, M. (2022). Macrophages in health and disease. Cell 185 (23), 4259–4279. doi:10.1016/j.cell.2022.10.007
Pers, J. O., Saraux, A., Pierre, R., and Youinou, P. (2008). Anti–TNF-α immunotherapy is associated with increased gingival inflammation without clinical attachment loss in subjects with rheumatoid arthritis. J. Periodontol. 79 (9), 1645–1651. doi:10.1902/jop.2008.070616
Pettersson, M., Kelk, P., Belibasakis, G. N., Bylund, D., Molin Thorén, M., and Johansson, A. (2017). Titanium ions form particles that activate and execute interleukin-1β release from lipopolysaccharide-primed macrophages. J. Periodontal Res. 52 (1), 21–32. doi:10.1111/jre.12364
Pirih, F. Q., Hiyari, S., Leung, H. Y., Barroso, A. D., Jorge, A. C., Perussolo, J., et al. (2015). A murine model of lipopolysaccharide-induced peri-implant mucositis and peri-implantitis. J. Oral Implantol. 41 (5), e158–e164. doi:10.1563/aaid-joi-D-14-00068
Pizarek, J. A., Fischer, N. G., and Aparicio, C. (2023). Immunomodulatory IL-23 receptor antagonist peptide nanocoatings for implant soft tissue healing. Dent. Mater 39, 204–216. doi:10.1016/j.dental.2023.01.001
Pongnarisorn, N. J., Gemmell, E., Tan, A. E., Henry, P. J., Marshall, R. I., and Seymour, G. J. (2007). Inflammation associated with implants with different surface types. Clin. Oral Implants Res. 18 (1), 114–125. doi:10.1111/j.1600-0501.2006.01304.x
Ramenzoni, L. L., Fluckiger, L. B., Attin, T., and Schmidlin, P. R. (2021). Effect of titanium and zirconium oxide microparticles on pro-inflammatory response in human macrophages under induced sterile inflammation: an in vitro study. Mater. (Basel) 14 (15), 4166. doi:10.3390/ma14154166
Renvert, S., Persson, G. R., Pirih, F. Q., and Camargo, P. M. (2018). Peri-implant health, peri-implant mucositis, and peri-implantitis: case definitions and diagnostic considerations. J. Periodontol. 89 (1), S304–S312. doi:10.1002/JPER.17-0588
Renvert, S., Roos-Jansåker, A. M., Lindahl, C., Renvert, H., and Rutger Persson, G. (2007). Infection at titanium implants with or without a clinical diagnosis of inflammation. Clin. Oral Implants Res. 18 (4), 509–516. doi:10.1111/j.1600-0501.2007.01378.x
Revell, P. A. (2008). The combined role of wear particles, macrophages and lymphocytes in the loosening of total joint prostheses. J. R. Soc. Interface 5 (28), 1263–1278. doi:10.1098/rsif.2008.0142
Rinke, S., Ohl, S., Ziebolz, D., Lange, K., and Eickholz, P. (2011). Prevalence of periimplant disease in partially edentulous patients: a practice-based cross-sectional study. Clin. Oral Implants Res. 22 (8), 826–833. doi:10.1111/j.1600-0501.2010.02061.x
Roos-Jansåker, A. M., Lindahl, C., Renvert, H., and Renvert, S. (2006). Nine-to fourteen-year follow-up of implant treatment. Part II: presence of peri-implant lesions. J. Clin. Periodontol. 33 (4), 290–295. doi:10.1111/j.1600-051X.2006.00906.x
Ruiz-Heiland, G., Yong, J. W., von Bremen, J., and Ruf, S. (2021). Leptin reduces in vitro cementoblast mineralization and survival as well as induces PGE2 release by ERK1/2 commitment. Clin. Oral Investig. 25 (4), 1933–1944. doi:10.1007/s00784-020-03501-3
Sasaki, H., Okamatsu, Y., Kawai, T., Kent, R., Taubman, M., and Stashenko, P. (2004). The interleukin-10 knockout mouse is highly susceptible to Porphyromonas gingivalis-induced alveolar bone loss. J. Periodontal Res. 39 (6), 432–441. doi:10.1111/j.1600-0765.2004.00760.x
Schlegel, K. A., Eppeneder, S., and Wiltfang, J. (2002). Soft tissue findings above submerged titanium implants--a histological and spectroscopic study. Biomaterials 23 (14), 2939–2944. doi:10.1016/s0142-9612(01)00423-9
Shaddox, L. M., Wiedey, J., Calderon, N. L., Magnusson, I., Bimstein, E., Bidwell, J. A., et al. (2011). Local inflammatory markers and systemic endotoxin in aggressive periodontitis. J. Dent. Res. 90 (9), 1140–1144. doi:10.1177/0022034511413928
Shafizadeh, M., Amid, R., Mahmoum, M., and Kadkhodazadeh, M. (2021). Histopathological characterization of peri-implant diseases: a systematic review and meta-analysis. Arch. Oral Biol. 132, 105288. doi:10.1016/j.archoralbio.2021.105288
Shen, Z., Kuang, S., Zhang, Y., Yang, M., Qin, W., Shi, X., et al. (2020). Chitosan hydrogel incorporated with dental pulp stem cell-derived exosomes alleviates periodontitis in mice via a macrophage-dependent mechanism. Bioact. Mater 5 (4), 1113–1126. doi:10.1016/j.bioactmat.2020.07.002
Sica, A., and Mantovani, A. (2012). Macrophage plasticity and polarization: in vivo veritas. J. Clin. Invest. 122 (3), 787–795. doi:10.1172/JCI59643
Sima, C., and Glogauer, M. (2013). Macrophage subsets and osteoimmunology: tuning of the immunological recognition and effector systems that maintain alveolar bone. Periodontol 63 (1), 80–101. doi:10.1111/prd.12032
Stashenko, P., Jandinski, J. J., Fujiyoshi, P., Rynar, J., and Socransky, S. S. (1991). Tissue levels of bone resorptive cytokines in periodontal disease. J. Periodontol. 62 (8), 504–509. doi:10.1902/jop.1991.62.8.504
Stienstra, R., Duval, C., Keshtkar, S., van der Laak, J., Kersten, S., and Müller, M. (2008). Peroxisome proliferator-activated receptor gamma activation promotes infiltration of alternatively activated macrophages into adipose tissue. J. Biol. Chem. 283 (33), 22620–22627. doi:10.1074/jbc.M710314200
Sun, X., Gao, J., Meng, X., Lu, X., Zhang, L., and Chen, R. (2021a). Polarized macrophages in periodontitis: characteristics, function, and molecular signaling. Front. Immunol. 12, 763334. doi:10.3389/fimmu.2021.763334
Sun, Y., Sun, X., Li, X., Li, W., Li, C., Zhou, Y., et al. (2021b). A versatile nanocomposite based on nanoceria for antibacterial enhancement and protection from aPDT-aggravated inflammation via modulation of macrophage polarization. Biomaterials 268, 120614. doi:10.1016/j.biomaterials.2020.120614
Tomasi, C., Tessarolo, F., Caola, I., Piccoli, F., Wennstrom, J. L., Nollo, G., et al. (2016). Early healing of peri-implant mucosa in man. J. Clin. Periodontol. 43 (10), 816–824. doi:10.1111/jcpe.12591
Tonetti, M. S., Greenwell, H., and Kornman, K. S. (2018). Staging and grading of periodontitis: framework and proposal of a new classification and case definition. J. Clin. Periodontol. 45, S149–s161. doi:10.1111/jcpe.12945
Tzach-Nahman, R., Mizraji, G., Shapira, L., Nussbaum, G., and Wilensky, A. (2017). Oral infection with Porphyromonas gingivalis induces peri-implantitis in a murine model: evaluation of bone loss and the local inflammatory response. J. Clin. Periodontol. 44 (7), 739–748. doi:10.1111/jcpe.12735
Viniegra, A., Goldberg, H., Çil, Ç., Fine, N., Sheikh, Z., Galli, M., et al. (2018). Resolving macrophages counter osteolysis by anabolic actions on bone cells. J. Dent. Res. 97 (10), 1160–1169. doi:10.1177/0022034518777973
Vodovotz, Y., Bogdan, C., Paik, J., Xie, Q. W., and Nathan, C. (1993). Mechanisms of suppression of macrophage nitric oxide release by transforming growth factor beta. J. Exp. Med. 178 (2), 605–613. doi:10.1084/jem.178.2.605
Wang, H., Wang, D., Huangfu, H., Chen, S., Qin, Q., Ren, S., et al. (2023a). Highly efficient photothermal branched Au-Ag nanoparticles containing procyanidins for synergistic antibacterial and anti-inflammatory immunotherapy. Biomater. Sci. 11 (4), 1335–1349. doi:10.1039/d2bm01212j
Wang, Y., Li, C., Wan, Y., Qi, M., Chen, Q., Sun, Y., et al. (2021). Quercetin-loaded ceria nanocomposite potentiate dual-directional immunoregulation via macrophage polarization against periodontal inflammation. Small 17 (41), e2101505. doi:10.1002/smll.202101505
Wang, Y., Lin, Q., Zhang, H., Wang, S., Cui, J., Hu, Y., et al. (2023b). M2 macrophage-derived exosomes promote diabetic fracture healing by acting as an immunomodulator. Bioact. Mater 28, 273–283. doi:10.1016/j.bioactmat.2023.05.018
Wang, Z., Maruyama, K., Sakisaka, Y., Suzuki, S., Tada, H., Suto, M., et al. (2019). Cyclic stretch force induces periodontal ligament cells to secrete exosomes that suppress IL-1β production through the inhibition of the NF-κB signaling pathway in macrophages. Front. Immunol. 10, 1310. doi:10.3389/fimmu.2019.01310
Whitaker, R., Hernaez-Estrada, B., Hernandez, R. M., Santos-Vizcaino, E., and Spiller, K. L. (2021). Immunomodulatory biomaterials for tissue repair. Chem. Rev. 121 (18), 11305–11335. doi:10.1021/acs.chemrev.0c00895
Wilson, T. G., Valderrama, P., Burbano, M., Blansett, J., Levine, R., Kessler, H., et al. (2015). Foreign bodies associated with peri-implantitis human biopsies. J. Periodontol. 86 (1), 9–15. doi:10.1902/jop.2014.140363
Wu, J., Yu, P., Lv, H., Yang, S., and Wu, Z. (2020a). Nanostructured zirconia surfaces regulate human gingival fibroblasts behavior through differential modulation of macrophage polarization. Front. Bioeng. Biotechnol. 8, 611684. doi:10.3389/fbioe.2020.611684
Wu, X., Chen, H., Wang, Y., and Gu, Y. (2020b). Akt2 affects periodontal inflammation via altering the M1/M2 ratio. J. Dent. Res. 99 (5), 577–587. doi:10.1177/0022034520910127
Wu, X., Wang, Y., Chen, H., Wang, Y., and Gu, Y. (2023). Phosphatase and tensin homologue determine inflammatory status by differentially regulating the expression of Akt1 and Akt2 in macrophage alternative polarization of periodontitis. J. Clin. Periodontol. 50 (2), 220–231. doi:10.1111/jcpe.13730
Wynn, T. A., Chawla, A., and Pollard, J. W. (2013). Macrophage biology in development, homeostasis and disease. Nature 496 (7446), 445–455. doi:10.1038/nature12034
Wynn, T. A., and Vannella, K. M. (2016). Macrophages in tissue repair, regeneration, and fibrosis. Immunity 44 (3), 450–462. doi:10.1016/j.immuni.2016.02.015
Xiao, L., Feng, M., Chen, C., Xiao, Q., Cui, Y., and Zhang, Y. (2023). Microenvironment-regulating drug delivery nanoparticles for treating and preventing typical biofilm-induced oral diseases. Adv. Mater, e2304982. doi:10.1002/adma.202304982
Yang, J., Zhu, Y., Duan, D., Wang, P., Xin, Y., Bai, L., et al. (2018). Enhanced activity of macrophage M1/M2 phenotypes in periodontitis. Arch. Oral Biol. 96, 234–242. doi:10.1016/j.archoralbio.2017.03.006
Yang, L., Tao, W., Xie, C., Chen, Q., Zhao, Y., Zhang, L., et al. (2023). Interleukin-37 ameliorates periodontitis development by inhibiting NLRP3 inflammasome activation and modulating M1/M2 macrophage polarization. J. Periodontal Res. doi:10.1111/jre.13196
Yang, Y., Lin, Y., Zhang, Z., Xu, R., Yu, X., and Deng, F. (2021). Micro/nano-net guides M2-pattern macrophage cytoskeleton distribution via Src-ROCK signalling for enhanced angiogenesis. Biomater. Sci. 9 (9), 3334–3347. doi:10.1039/d1bm00116g
Yu, T., Zhao, L., Huang, X., Ma, C., Wang, Y., Zhang, J., et al. (2016). Enhanced activity of the macrophage M1/M2 phenotypes and phenotypic switch to M1 in periodontal infection. J. Periodontol. 87 (9), 1092–1102. doi:10.1902/jop.2016.160081
Zhou, L. N., Bi, C. S., Gao, L. N., An, Y., Chen, F., and Chen, F. M. (2019). Macrophage polarization in human gingival tissue in response to periodontal disease. Oral Dis. 25 (1), 265–273. doi:10.1111/odi.12983
Zhuang, Z., Yoshizawa-Smith, S., Glowacki, A., Maltos, K., Pacheco, C., Shehabeldin, M., et al. (2019). Induction of M2 macrophages prevents bone loss in murine periodontitis models. J. Dent. Res. 98 (2), 200–208. doi:10.1177/0022034518805984
Keywords: peri-implantitis, macrophages, polarization, immune dysregulation, periodontitis
Citation: Li Y, Li X, Guo D, Meng L, Feng X, Zhang Y and Pan S (2024) Immune dysregulation and macrophage polarization in peri-implantitis. Front. Bioeng. Biotechnol. 12:1291880. doi: 10.3389/fbioe.2024.1291880
Received: 10 September 2023; Accepted: 09 January 2024;
Published: 29 January 2024.
Edited by:
Xianqi Li, Matsumoto Dental University, JapanReviewed by:
Ning Cheng, 4D Molecular Therapeutics, United StatesZhen Geng, Shanghai University, China
Copyright © 2024 Li, Li, Guo, Meng, Feng, Zhang and Pan. This is an open-access article distributed under the terms of the Creative Commons Attribution License (CC BY). The use, distribution or reproduction in other forums is permitted, provided the original author(s) and the copyright owner(s) are credited and that the original publication in this journal is cited, in accordance with accepted academic practice. No use, distribution or reproduction is permitted which does not comply with these terms.
*Correspondence: Shaoxia Pan, panshaoxia@vip.163.com; Yi Zhang, zhangyi612@hotmail.com; Xianghui Feng, susanf0821@163.com
†These authors have contributed equally to this work and share first authorship