- 1Department of Chemistry, University of Kalyani, Kalyani, India
- 2Department of Chemistry, Pennsylvania State University, State College, PA, United States
- 3Department of Chemistry and Applied Biosciences, Zurich, Switzerland
Carbon quantum dots (CQDs) are gaining a lot more attention than traditional semiconductor quantum dots owing to their intrinsic fluorescence property, chemical inertness, biocompatibility, non-toxicity, and simple and inexpensive synthetic route of preparation. These properties allow CQDs to be utilized for a broad range of applications in various fields of scientific research including biomedical sciences, particularly in bioimaging and biomedicines. CQDs are a promising choice for advanced nanomaterials research for bioimaging and biomedicines owing to their unique chemical, physical, and optical properties. CQDs doped with hetero atom, or polymer composite materials are extremely advantageous for biochemical, biological, and biomedical applications since they are easy to prepare, biocompatible, and have beneficial properties. This type of CQD is highly useful in phototherapy, gene therapy, medication delivery, and bioimaging. This review explores the applications of CQDs in bioimaging and biomedicine, highlighting recent advancements and future possibilities to increase interest in their numerous advantages for therapeutic applications.
1 Introduction
An element’s properties are determined by its electronic configuration, but Quantum phenomena emerge when matter is reduced to nanoscale dimensions due to matter size (Cotta, 2020; García de Arquer et al., 2023). The Chemistry Nobel Prize for 2023 recognizes the discovery and evolution of quantum dots (QDs) and is jointly awarded to Aleksey Yekimov (created size-dependent quantum effects in coloured glass), Louis Brus (first to demonstrate size-dependent particle quantum effects), Moungi Bawendi (revolutionized the chemical productions of QD) (NobelPrize. org., 2024). QDs are tiny nanoparticles with properties determined by size. Quantum dots, the nanotechnology components offer significant benefits to humanity, including tiny sensors, flexible electronics, encrypted quantum communication, thinner solar cells, and surgery to guide tissue removal (Gangasani and Student, 2007; Cheki et al., 2013; Knoblauch et al., 2014; Zhao and Zhu, 2016).
Carbon nanomaterials have drawn great interest due to their simple surface functionalization, easy synthesis methods, biodegradability, and no toxicity. Carbon-based quantum dots or Carbon quantum dots (CQDs) are submicron-sized nanomaterials with zero dimensions that were initially produced in 2004 while purifying single-walled carbon nanotubes (SWCNTs) (Xu et al., 2004). Following this, CQDs have been applied to a broad range of applications because of their inexpensive preparation cost and advantageous qualities, which include solubility in aqueous medium, chemical inertness, biocompatibility, and non-toxicity (Wang et al., 2014; Lim et al., 2015; Sousa et al., 2021). CQDs exhibit unique and fascinating properties, with their colours varying based on their size (Mai et al., 2020). CQDs are largely employed in biological applications due to their intrinsic fluorescence, stability, biocompatibility, ease of manufacture, and lack of adverse effects (Lan et al., 2017; Pourmadadi et al., 2023). Carbon nanotubes, graphene, and fullerenes have low aqueous solubility resulting in no fluorescence emission in visible regions and having limited applications (Sahu et al., 2012; Liang et al., 2013; Yang et al., 2013). On the other hand, CQDs are not having such limitations. CQDs, with their unique optical and electronic properties, are increasingly used in electronics, sensors, solar cells, catalysis, supercapacitors, and energy conversion gadgets (Wang and Hu, 2014; Lim et al., 2015; Ahmad et al., 2017; Pal et al., 2019). Most CQD molecules are typically composed of a sp2/sp3 hybridized carbon core with various surface functional groups (Bhunia et al., 2013; Zhao et al., 2015). The water-soluble nature of CQDs is attributed to the presence of functional groups that contain oxygen, namely, carboxyl (-COOH) and hydroxyl (-OH), on their surface (Zhu et al., 2015). In addition to providing fluorescence characteristics, these functional groups on CQDs assist their solubility and enable them to generate durable colloids in water or polar solvents. Their significant quantum yield (QY) of fluorescence and stable red and near-infrared emission properties make them used in various applications in biology and medicine, particularly in bioimaging and biomedicines (Bhunia et al., 2013; Luo et al., 2013; Zuo et al., 2015). CQDs have also been employed to understand the interactions with various proteins, like other biocompatible nanomaterials (Mondal et al., 2015; 2016; Mondal, 2017; Malik et al., 2019). This opens a new avenue for understanding the significance of CQD in nanomedicines with possible interactions and their outcomes with various human proteins. CQDs’ distinct chemistry and optical and physical characteristics render them a potential candidate for advanced nanomaterials research for bioimaging and biomedicines (Nazir et al., 2014; Sasaki et al., 2021; Sangjan et al., 2022; Gedda et al., 2023).
CQDs and polymer composite materials are efficient for biological, biochemical, and biomedical applications due to their ease of preparation, biocompatibility, and economical (Kausar, 2019; Molaei, 2019; Feng et al., 2021; Wang et al., 2022; Pathak et al., 2023). To create photoluminescent materials, careful tuning of their surface-level chemical groups and size is necessary for proper electrical structure tuning (Pal et al., 2019; Abbas et al., 2023; Yang et al., 2023). CQDs can be synthesized from various sources and mixed with polymers to create composites. Developing better CQD/polymer composite materials utilizing ligand exchange, grafting, and capping, leads to commend biocompatibility and optoelectrical features is a growing research topic (Qi and Gao, 2008; Kausar, 2019; Pathak et al., 2023). A variety of in vivo biological obstacles, such as glomerular filtration barriers, blood-brain barriers, and ion channels, can be overcome by them due to their small size (Yang et al., 2009). This type of CQD has great applications in bioimaging, phototherapy, drug delivery, and gene therapy (Ross et al., 2020; Pathak et al., 2023; Soumya et al., 2023).
We present a concise overview of the applications of CQDs in bioimaging and biomedicine. This review will explore recent advances, future possibilities, and various applications of CQDs and CQDs-based composites in the field of bioimaging and biomedicines, aiming to increase interest in numerous advantages of CQDs in these fields.
2 Synthesis of CQDs
Through the purification method of single-walled carbon nanotubes, Scrivens et al. obtained luminous carbon nanoparticles for the first time in 2004. Since then, several synthetic routes have been reported for the synthesis of CQDs varying in size and surface functional groupings. In general, CQDs can be synthesized using either of two primary methods: top-down or bottom-up (Figure 1) (Niu N. et al., 2017; Yadav et al., 2023). Top-down approaches use physical or chemical mechanisms to split up bigger carbon structures into smaller ones. For instance, arc discharge, laser ablation, and electrochemical oxidation can be utilised to cut graphite or carbon nanotubes into CQDs. These techniques have the potential to yield high-quality CQDs with uniform size distribution and good crystallinity, but they also come with a cost and could produce hazardous byproducts. Conversely, using thermal or chemical reactions, CQDs are assembled from smaller carbon precursors in bottom-up approaches. To create CQDs from organic molecules or biomass, for instance, hydrothermal treatment, solvothermal synthesis, and microwave pyrolysis can be applied (Ren R. et al., 2019). These techniques can provide CQDs with different functional groups and doping components, however, they could also lead to poor size control and low yield. To enhance the characteristics and functionality of CQDs, post-treatment procedures such as doping, surface passivation, and purification can be applied to both top-down and bottom-up approaches. The intended uses and properties of CQDs influence the synthesis technique selection.
2.1 Top-down method
The top-down process incorporates arc discharge, laser ablation, and acidic oxidation to break down the larger carbon resources into smaller components. This method generally employs carbon structures having sp2 hybridization that don’t have effective energy gaps or band gaps as precursor materials. The top-down method is very beneficial and effective for microsystem industries, but there are certain restrictions regarding the uniform size and shape distributions of CQDs, impurity, and costs (Table 1).
2.1.1 Laser ablation
This approach uses a high-energy laser pulse to illuminate the surface of the starting material. Consequently, it reaches a thermodynamic state characterized by incredibly high temperatures (>900°C) and pressures (75 KPa), leading to heating up quickly and condensing into a plasma state in which CQDs are formed by vapour crystallization. The first report of a laser ablation approach was made in 2006 by Sun and colleagues (Sun et al., 2006). The pulsed laser ablation method described by Ren X. et al., 2019 was utilized to produce N-doped micro-pore CQDs (NM-CQDs). NM-CQD was used for cellular staining and imaging and showed good internalization in various cells. Dual-beam laser ablation was described by Cui et al., 2020 as a means of producing carbon quantum dots (CQDs) from inexpensive carbon fabrics for utilization in bioimaging. The laser ablation method yields more PL emissions.
2.1.2 Arc discharge
This process utilizes gas plasma generated in a sealed reactor inside an anodic electrode to reorganize carbon molecules as they break into smaller segments from bulk carbon sources. When the electric current is present, the reactor’s temperature can reach −3,700°C, resulting in massively energetic plasma. Carbon vapour accumulates in the cathode to produce CQDs (Su et al., 2014). When Xu and colleagues used the arch discharge approach first to isolate and purify a single-wall carbon nanotube, they unintentionally discovered fluorescent carbon quantum dots (Xu et al., 2004). The CQDs can exhibit blue-green, orange, or yellow fluorescence at a wavelength of 365 nm. Using HNO3, the carboxyl moiety (hydrophilic) was added to the CQD surface.
2.1.3 Acidic oxidation
It was reported in 2014 that hetero-atom-doped CQDs may be synthesized on a wide scale via hydrothermal reduction and acidic oxidation. To begin, carbon nanoparticles were oxidized using a mixture of H2SO4, HNO3, and NaClO3. (Dong et al., 2010). Subsequently, the oxidized CQDs underwent hydrothermal reactions with sources of selenium, sulfur, and nitrogen. Precursors such as sodium hydrosulfide (NaHS), sodium selenide (NaHSe), and dimethyl formamide (DMF) have each been employed. The generated S-CQDs, Se-CQDs, and N-CQDs, have demonstrated tunable extended fluorescence life-span, improved good quantum yield (QY), and PL activity in contrast to pure CQDs (Yan et al., 2014). CQDs are also used to prepare drug delivery applications and electrochemical sensors once they are generated using the acidic oxidation process.
2.1.4 Electrochemical
The electrochemical method, primarily introduced by Zhou and coworkers in 2007, was used to fabricate blue luminescent CQDs deriving out of multiwall carbon nanotubes. This method involves larger carbon precursors shredding into smaller parts through electrochemical oxidation, with a reference electrode. Zhang et al. have developed water-soluble CQDs exhibiting tunable luminescence (Hou et al., 2015). Deng et al., 2014 utilized this method to synthesize CQDs utilising low-molecular-weight alcohol. However, this technique needs surface passivation, and has a tedious purification process, making it the least frequently used technique.
2.1.5 Ultrasonication
The CQDs preparation process is easy and economical with the use of ultrasonic technology. It creates waves of alternating high- and low-pressure causing tiny bubbles in liquid to evolve and burst. To achieve varied properties, researchers can alter the reaction time, ultrasonic power, and ratio of carbon source to solvent. CQDs have been produced using this technique from various carbon materials, such as graphite, MWCNTs, and carbon fibre. CQDs exhibit blue luminous emission and range in diameter from 1 to 5 nm (Park et al., 2014). The ultrasonic method can also be used to create heteroatom-doped CQDs (Huang et al., 2018). CQDs can also be made using other waste materials that contain carbon (Feng and Zhang, 2019). Food waste and ethanol can be combined to create water-soluble CQDs, which have several benefits including low cytotoxicity, strong photoluminescence, and high Photostability for in vitro bioimaging.
2.2 Bottom-up method
The top-down approach for CQDs synthesis utilizes techniques like combustion, hydrothermal/solvothermal, microwave irradiation, template, and pyrolysis (Table 2). The shape and size of CQDs depend on factors like precursor molecular structures, solvent, and reaction conditions. The reaction condition is important as it affects the reactants as well as the incredibly random nucleation and escalation process of the CQDs. This approach has great advantages in material chemistry, is easy to operate, has lower costs, and is easier for large-scale production. Precursors for CQD synthesis can be chemical or biological, with chemical precursors including glucose, citric acid, sucrose, and natural sources like Azadirachta indica leaves, rice husks, and aloe vera.
2.2.1 Combustion
The combustion method gained popularity for its ease of scaling up, accurate precursor molecule design, affordability, and environmentally friendly features to enhance the bottom-up methods for producing CQDs. The method of synthesizing CQDs using combustion was initially published by Liu et al., 2007. This process uses oxidative acid treatments to adjust the fluorescence characteristics, improve water solubility, and aggregate tiny carbon resources into CQDs. Liu and Coworkers clarified that the process of partially burning a candle with aluminium foil and refluxing it in a solution of nitric acid produced candle ashes, followed by dissoluting the candle ashes in a neutral medium, centrifuging the mixture, and using a dialysis technique to obtain Pure CQDs. Researchers have developed combustion methods for producing fluorescent CQDs, such as combusting citric acid, followed by surface functionalization with carboxyl groups through acetic acid moiety conjugation at elevated temperatures (Yang et al., 2023). The combustion approach synthesizes CQDs with poor QY but good fluorescence without doping.
2.2.2 Hydrothermal
The hydrothermal process includes pouring the precursor materials into an aqueous media, then adding the mixture to a stainless-steel autoclave lined with Teflon and heating it to high pressure for many hours. Zhang et al., 2010 initially introduced the use of the hydrothermal method to prepare 2 nm-diameter fluorescent CQDs by employing L-ascorbic acid as a carbon precursor. The hydrothermal process, which has attracted major interest recently owing to its cost-effectiveness and environmental friendliness, produces CQDs from tiny molecules (amino acids, saccharides, sucrose, proteins, glucose, polymers, polyols, discarded peels or juice, etc.) in a single, environment friendly, and cost-effective way. Carbon quantum dots (CQDs) were made sustainably from biowaste obtained from banana peels incorporating a simple hydrothermal process by Lee et al. (Atchudan et al., 2020). When exposed to UV light (365 nm), these CQDs generate a strong blue fluorescence with a 20% quantum yield (QY). NS-CQDs (QY 53.19%) were also produced utilizing this process from L-lysine and thiourea to detect the concentration of picric acid in water. A solvothermal approach is also involved in synthesizing CQDs in which alcohol, ammonia, and other organic and inorganic solvents are employed in place of water (Zuo et al., 2015).
2.2.3 Microwave
CQDs can be produced efficiently and economically via microwave synthesis, which employs electromagnetic radiation with a broad wavelength that ranges from 1 mm to 1 m (Ahmad et al., 2018). This radiation can provide sufficient energy to disrupt the chemical bonds of the reaction’s precursor components. In comparison to other techniques, this process is easy, straightforward, quick, and ecologically friendly, resulting in higher quantum yield for the CQDs formation. Zhu et al., 2009 and co-workers developed fluorescent CQDs having electrochemiluminescence abilities for the very first time by heating a clear aqueous solution of saccharides and PEG 200 in a 500 W microwave oven for 2–10 min utilizing this simple and affordable method.
2.2.4 Pyrolysis
The pyrolysis technique is a thermal decomposition of a precursor, typically over 430°C, to create nanoscale colloidal particles. It offers practicality, repeatability, simplicity, and high quantum yield (QY). In the presence of a strong acid concentration and an alkali as a catalyst, the carbon precursor cleaves into colloidal nanoparticles. However, separating small precursors from raw materials is challenging. In 2009, Liu et al., 2009 developed a method for preparing CQDs using resol (source of Carbon) with surfactant-modified silica spheres. The CQDs were stable in a broad pH range and showed blue fluorescence. Xue et al., 2016 synthesized N-doped carbon quantum dots (CQDs) from peanut shell waste using an economical carbonization method, which shows excitation-dependent fluorescence emission. Praneerad et al., 2019 produced fluorescent CQDs from durian peel biomass waste, which was used for constructing a composite electrode with higher specific capacitance than a Carbon electrode.
2.2.5 Template
Another commonly used approach for producing nano-sized CQDs is the template-assisted technique which provides higher quantum yields and even size distribution. In this method, CQDs are prepared via two steps. The first step involved calcination, which produced CQDs in the suitable silicon sphere or mesoporous template and the second step involved etching, which removed the supporting components. As a beneficial methodology for CQDs, this procedure is simple, accessible, inhibit particle agglomeration, appropriate for surface passivation, and regulates CQD size (Liu et al., 2009). Mesoporous silica spheres were used as nanoreactors by Zhong and colleagues in 2011 to generate carbon dots with exceptional luminescence characteristics. Templates can be made of hard elements like silica, metal-organic framework, layered host matrices, and zeolite, or soft elements like surfactants. Yang et al., 2013 created a novel soft-hard template technique for photoluminescent CQDs synthesized from organic molecules. The soft template in this report is Pluronic P123, while the hard template is OMS (ordered mesoporous silica) SBA-15. The drawback of the template method is that certain templates are challenging to extract from the CQDs, and the fluorescence activity of the CQDs could be impacted when the templates are removed by heating or acid-base etching. Besides all these techniques discussed here, CQDs can also be synthesized from porous organic polymer following a suitable method for various applications (Pan et al., 2019; Das et al., 2022a).
3 Characteristics of CQDs
Depending on whether sp2 carbon is present, the core-shell architectures of carbon quantum dots (CQDs) can have either an amorphous or graphitic crystalline structure. These tiny (2–3 nm) cores vary in size based on the synthesis method, precursors, and additional factors (Pathak et al., 2023; Yadav et al., 2023). Precursors, methods, and other factors are used to classify cores. The core structure of CQDs is ascertained using instrumental methods such as Nuclear magnetic resonance (NMR), Raman spectroscopy, X-ray diffraction (XRD), TEM, and SEM. X-ray photoelectron spectroscopy (XPS), elemental analysis (EA), and Fourier transform infrared (FT-IR) are further methods. NMR can also be employed to understand the interactions and relaxation studies of CQDs with various proteins (Jaipuria et al., 2012; 2022; Mondal et al., 2013; Dubey et al., 2016; Werbeck et al., 2020). Nitrogen sorption analysis is used to determine the surface area of carbon nanoparticles (Verma et al., 2020). Using zeta potential, the existence of functional groups on the outer layer is verified. The PL characteristics of CQD fluorescence emission arising out of the conjugated p domain are determined by the quantum-confinement effect from p-conjugated electrons within the sp2 atomic structure (Zhu et al., 2015). Fluorescence emission is caused by surface imperfections such as sp2 and sp3 hybridized carbon, and the size of CQDs influences the characteristics of PL (Luo et al., 2014; Zuo et al., 2015). Fluorescence emission and peak placement in CQDs can be impacted by surface imperfections and irregularities. CQDs can be created using the entire spectrum of sunlight (Ahmad et al., 2018). Moreover, photoexcited electron transfer is aided by the CQDs-based composites’ capacity to harvest light, which raises photocatalytic efficiency.
Carbon quantum dots, in contrast to semiconductor QDs, can exist in an amorphous form because of their predominant sp2 and sp3 molecular orbitals (Wang et al., 2014). Carbon QDs are often modified by surface passivation and functionalization with a particular organic ligand. As a result, several organic functional groups, including carboxylic, carbonyl, hydroxyl, and hydrocarbon, are capped on the surface (Cui et al., 2021). The carbon core is covalently linked to every member of this functional group. This effect makes CQD more soluble in water and makes preparing it for use in a variety of applications easier.
4 Properties of CQDs
CQDs are characterized by a “core-shell” nanostructure that is made up of surface functional groups and a nanoscale carbon core (Pourmadadi et al., 2023). Their various structural configurations and quantum confinement effect also affect their catalytic, optical, and electronic behaviours. These properties can further be modified utilizing surface passivation or doping the CQD (Yang et al., 2023). The bandgap and electronic structure are modified by doping and the presence of heteroatoms.
CQDs prepared out of various precursors, exhibit varying absorption spectra in different solvents (Wang et al., 2014). They have similar UV-visible absorption, with absorption peaks in the UV region ranging from 260 nm to 320 nm. The absorption peak in the 280–350 nm range is attributed to electronic transitions from C–O or C=O bonds to π* orbitals. The absorption peak in the 350–600 nm range is attributed to surface chemical moieties. Some CQDs display long-wavelength absorption ranging from 600 to 800 nm, originating out of the aromatic ring-containing structures (Cui et al., 2021). Surface modification or passivation can influence absorption properties. Carbon quantum dots (CQDs) can have their optical characteristics modified by surface passivation, functional groups, and heteroatom doping or co-doping (Yadav et al., 2023). The CQDs are shielded from impurity adherence and extra stability by the protective layer that surface passivation creates on their surface. When surface-passivating chemicals are added to CQDs, they exhibit increased quantum yields and fluorescence, making them highly optically active. Longer wavelength absorption can also be enhanced by surface passivation. Covalent bonds between functionalizing agents and CQDs can produce materials with exceptional photo reversibility, low toxicity, high stability, and strong biocompatibility (Gao et al., 2018). By modifying the π-π* energy level, the dopant modifies the bandgap, electrical structure, and optical characteristics of CQDs. Various processes (as mentioned in Section 2) can be utilized to create such heteroatom-doped CQDs, which have better photocatalytic activity, increased electron transfer, and higher QYs.
Fluorescent CQDs have great applications in sensing, bioimaging and biomedicines (Figure 2). There are various mechanisms to understand the fluorescence in CQDs (Yang et al., 2023). Fluorescence phenomena in CQDs are primarily produced by two mechanisms: (a) surface defects, surface passivation/functionalization (Figure 3), carbon core state, quantum size effect, and (b) band gaps of π-conjugated domains (sp2 hybridized) which resemble aromatic molecules incorporating certain energy band gaps regarding absorption and emission (Song et al., 2014). On the other hand, surface defects arise in CQD owing to the unsymmetrical arrangement of sp2 and sp3 carbon atoms, and the presence of hetero atoms like N, B, P, or S. Due to the surface defect, the solid host creates an environment like aromatic molecules resulting in absorbing UV light to exhibit various colour emissions. The two emission forms are seen in CQDs: excitation-independent emission because of the highly ordered graphitic structure, and excitation-dependent emission (tunable emission) because of different emission sites and particle size distribution (Figure 4). In contrast to conventional organic dyes, CQDs have high photostability and steady fluorescence.
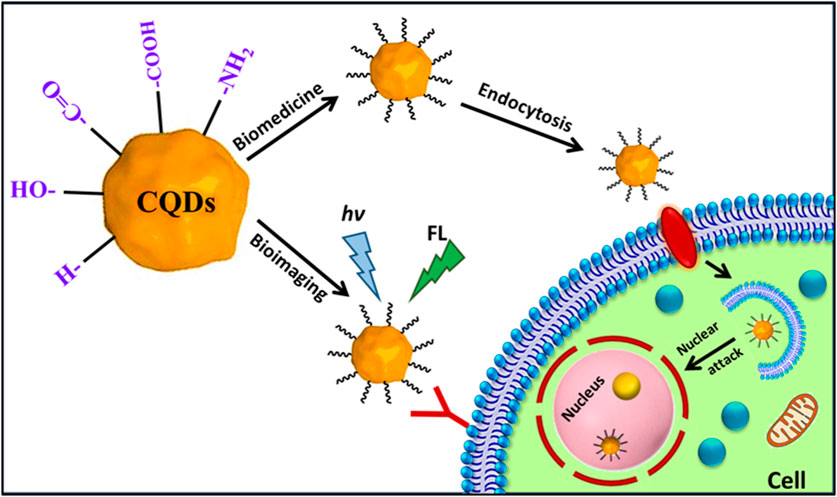
FIGURE 2. Schematic of the mechanism of actions of fluorescent carbon quantum dots inside the cells in bioimaging and biomedicine.
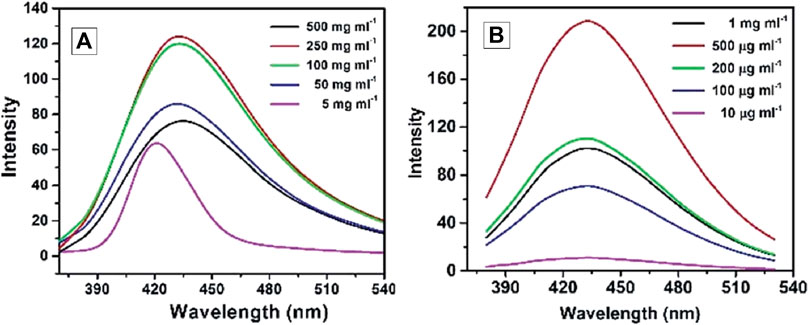
FIGURE 4. Fluorescence emission spectra (with excitation at 346 nm) of hydrophilic CQD (A) and hydrophobic CQD (B) prepared from sucrose and Octadecyl-amine/octadecene respectively. Reprinted (adapted) with permission from Mondal et al. (Mondal et al., 2015) Copyright (2015) Royal Society of Chemistry.
Recent studies have explored the photoluminescence (PL) emission in carbon quantum dots (CQDs), which has gained interest in photocatalysis with other fields (Yang et al., 2023). PL emission wavelengths are larger than the excitation wavelength and can be attributed to band-gap transitions incorporated in conjugated π-domains or deficiencies in graphene structures. Research has shown that excitation-dependent emission of fluorescent CQDs with surface modifications and one-step thermal treatment of 4-amino antipyrine emit excitation-dependent PL emission in 525–660 nm. The investigation looks at the PL emission of CQD, with a particular emphasis on size modification. CQDs were created by an electrochemical method assisted by alkali, and their diameters ranged from 1.2 to 3.8 nm (Hasanzadeh et al., 2021). The PL characteristics fluctuated with particle size, as revealed by optical views, suggesting a highly reliant HOMO-LUMO gap (Zhu et al., 2015). Particles with sizes smaller than 3 nm are more likely to emit visible spectrum light because the band gap narrows with increasing particle size. PL emission from CQD produced from alkyl-gallates is independent of size. In this review, we will discuss the recent advancements in bioimaging and biomedical applications employing all these features and various modifications of CQDs (Figure 5).
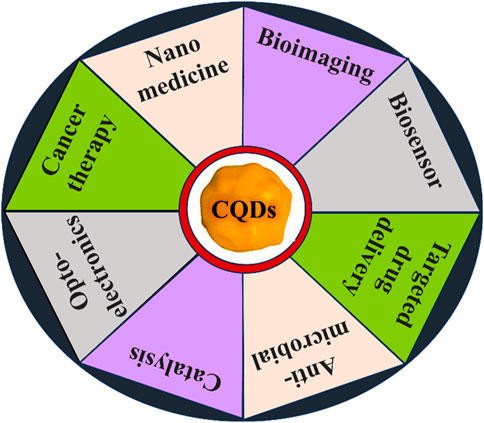
FIGURE 5. Various applications of CQDs. We discussed recent advancements in bioimaging and biomedical applications using CQDs in our review.
5 CQDs in bioimaging
Bioimaging enables real-time, non-invasive imaging of biological phenomena. It is a technique of utmost importance in healthcare units for the diagnosis of human health diseases. The intrinsic fluorescence property of CQDs, great stability, and great advantage of modification with various functional groups offer a suitable candidate for bioimaging (Bhunia et al., 2013; Luo et al., 2014; Liu et al., 2020b; Su et al., 2020). In addition, multi-wave-length emissions, excellent photostability, resistance to photobleaching, and quick and easy methods of preparation, establish CQDs to be the fluorescent probes of the next-generation for in vitro and in vivo imaging (Wegner and Hildebrandt, 2015; Wang et al., 2022; Gedda et al., 2023). Other conventional quantum dots like metal quantum dots and organic dyes are toxic, mostly prohibiting their uses in bioimaging (Su et al., 2020; Wang et al., 2022). Three-dimensional visualization of the biological subcellular compartments as well as tissues and organisms can be obtained with various biocompatible carbon quantum dots (Table 3).
Biocompatible CQDs can readily penetrate various cells through endocytosis, a macropinocytosis-like cell-penetration mechanism based on the shape, surface functionalization, and type of the cells (Magesh et al., 2022; Wang et al., 2022). Bioimaging of such cellular compartments and organelles can lead to a broader understanding of early symptoms and progression of different diseases like Alzheimer’s, Parkinson’s, diabetes, cancers, and many others (Kabanov and Gendelman, 2007; Guerrero et al., 2021). CQD can be employed to understand the interactions with various proteins and track the changes through Bioimaging (Bhunia et al., 2013; Luo et al., 2013; Wang et al., 2022). The unique properties of CQDs, such as their outstanding photostability, low cytotoxicity, conspicuous biocompatibility, and multicolour emission profile, make them a prime option for fluorescence imaging (Luo et al., 2014). Up until recently, almost all CQDs have essentially labelled the cytoplasm and cell membrane without any special alterations. CQDs were used for intracellular imaging with HeLa cells, MCF-7 cells, Caco-2 cells, HepG2 cells, PC12 cells, lung cancer cells, and pancreas stem cells (Li et al., 2018; Xia et al., 2019; Shi et al., 2020; Tian et al., 2020; El-brolsy et al., 2022; Havrdová et al., 2022). Besides, researchers have systematically tracked the biodistribution of CQD in mice cells through in vivo imaging (Shi et al., 2020; Tian et al., 2020). Tao et al., 2012 performed in vivo fluorescence imaging investigations (Figure 6) on CQD-M derived from MWNTs. The researchers labelled CQD-M I125. When the radiolabeling stability was examined in mouse plasma, the amount of I125 detachment from CQDs was found to be satisfactory. The biodistribution and blood radioactivity levels were used to measure the pharmacokinetics of CQDs. According to the study, following intravenous injection, CQDs were mostly collected in the liver and spleen. Early on, there was a significant level of kidney uptake of CQDs, indicating that they might pass through the glomerulus and be eliminated by urine. This study indicates that both renal and faecal excretion could lead to the clearance of CQDs (Tao et al., 2012).
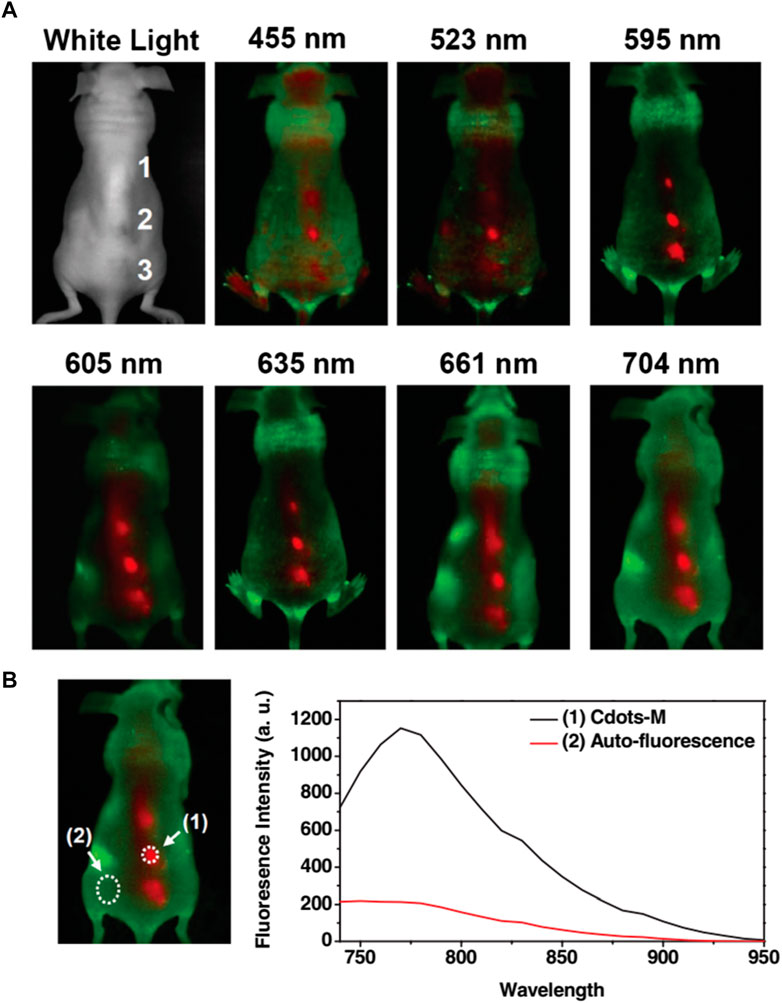
FIGURE 6. (A) Fluorescence imaging in vivo. In vivo fluorescence photos of a mouse administered with CQD. The pictures were captured at 455, 523, 595, 605, 635, 661, and 704 nm at different excitation wavelengths. Tissue autofluorescence and CQD fluorescent signals are shown in red and green, respectively. (B) The spectral picture obtained with NIR (704 nm) excitation has a signal-to-background separation. The background of tissue autofluorescence was clearly distinguished from the CQD fluorescence. High radioactivity of I125 was found in the urine and faeces of the mouse, indicating that both renal and faecal excretions may have contributed to the CQD’s clearance. Reprinted with permission from Tao et al. (2012) Reprinted (adapted) with permission from Liu et al. Copyright (2012) Wiley.
Using CQDs in polymer matrices (Carbonized Polymer Dot or CPD) is an additional new field of study with a broad range of possible applications (Feng et al., 2021). CQDs with polyethyleneimine (PEI) functionalization display tunable fluorescence with multiple wavelength emissions (Chen et al., 2019). The ternary nano-assembly of CD-PEI/Au-PEI/pDNA renders an effective transfecting agent as observed from a fluorescence microscope (Kim et al., 2013). CQD is functionalised with quaternary ammonium groups employed in L929 and NIH/3T3 cell lines of healthy mice and imaged with fluorescence microscopy (Havrdová et al., 2021). This study reveals the intranuclear uptake of the functionalised CQDs utilising their intrinsic fluorescence properties. Photoacoustic (PA) imaging and near-infrared fluorescence are displayed by large amino acid mimicking (LAAM) CQDs synthesised from 1,4,5,8-tetraminoanthraquinone and citric acid (Li et al., 2020). LAAM CQDs can selectively image tumours. CQDs prepared from o-phenylenediamine and terephthalic acid applied for near-infrared (NIR) bioimaging (Ding et al., 2020; Moniruzzaman et al., 2022; Marković et al., 2023). A donor-acceptor (D-π-A) structural approach was introduced for the synthesis of this CQD. CQD-PEG was developed by choosing polyethylene glycol as a passivating agent for the increment in functionality and photoluminescence (PL) properties of CQDs (Peng et al., 2020). CQD-PEG were exceptionally photoresponsive and photoluminescent after the surface modification. PEG passivated CQDs were initially used to stain Caco 2 cells for cellular bioimaging, rendering CQD a potential fluorescent label of the cells. Red emissive CQDs (absorption: 400–750 nm) were prepared using polythiophene phenyl propionic acid (Ge et al., 2015; Liu et al., 2020a; Ding et al., 2020). The red emissive CQDs exhibit a high photothermal conversion efficiency (η ∼ 38.5%) and a strong photoacoustic response. These special qualities allow the red emissive CQDs to be utilised as photoacoustic, multifunctional fluorescent, and biomedicines (discussed in the next section). Most CQDs are synthesized from graphite-based materials (GQD) that exhibit characteristics of graphene and can be utilized for bioimaging applications (Kortel et al., 2020). In the past, some techniques have been used to obtain mitochondrial imaging, such as labelling tumour cells with aptamer AS1411 and causing CQDs to collect at the mitochondrial and lysosomal sites. For imaging the nucleus, graphene-based CQD-PEI (Polyethyleneamine) was used (Tian et al., 2020). Following the addition of CQD/hydrogel loaded without and with 5-fluorouracil, bioimaging of A549 cells was carried out. CQDs were functionalized after being produced with citric acid using hydrothermal carbonization involving RGERPPR and maleimide-polyethylene glycol-amino succinimide succinate (Mal-PEG-NHS) (Gao et al., 2018). The resulting CQD was utilized for bioimaging. Gadolinium-encapsulated carbon dots can exhibit high T1 relaxivity (16.0 × 10−3 M-1 S−1, 7T) and intense fluorescence, enabling an imaging probe with intrinsically dual-mode (Jiao et al., 2022). The increased permeability and retention effect of these carbon dots helps them to accumulate readily in tumours and the unbound Gadolinium excretes from the host via the renal system.
Hydrophilic and hydrophobic CQDs can be obtained with the preferred synthetic routes (Section 2) which is not common for other metal quantum dots for biological applications. Hydrophobic CQDs in parallel to hydrophilic CQDs offer a wide range of applications for the study of membrane proteins and other biological systems. The application of functionalized CQDs as fluorescent cell labels were studied by Bhunia et al., 2013 (Figure 7). They discovered that the CQDs may be imaged with TAT peptide- or folate-functionalization utilizing a standard microscope and can be labelled in one to 2 hours. Because of the low surface charge and tiny hydrodynamic diameter, CQDs have poor non-specific binding to cells which gets improved by this functionalization. The cellular and in vivo imaging applications of fluorescent CQDs are demonstrated by the fact that they are non-toxic at dosages higher than typical concentrations as concluded from MTT assays (Bhunia et al., 2013).
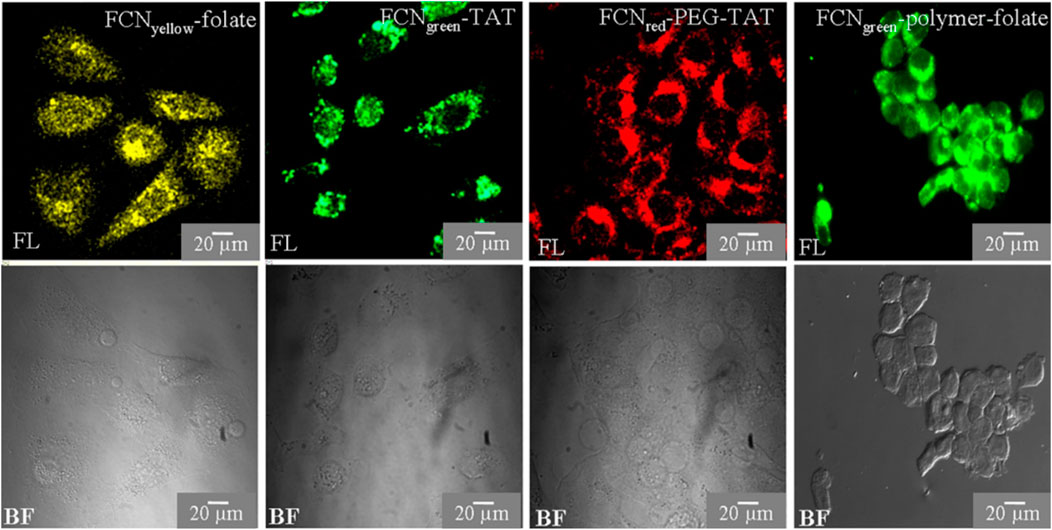
FIGURE 7. CQD as a fluorescent marker of cells. HeLa cells are cultured in CQDs for three to 6 hours, after which the labelled cells are observed under a fluorescent microscope. Using a confocal or Apotome microscope, cells are observed in bright field (BF) and fluorescence (FL) modes. Reprinted (adapted) with permission from Bhunia et al., 2013 Copyright (2013) Nature.
Hetero-atom-doped CQDs offer a wide range of wavelengths with multicolour fluorescence properties, increasing the stability and perfect for real-time cellular bioimaging (Sekar et al., 2022; Khoshnood et al., 2023; Mousa et al., 2023). In addition, when CQDs are co-doped with two heteroatoms from Nitrogen, Boron, or Phosphorus, the resulting CQD becomes more biocompatible with a much higher quantum yield (Atchudan et al., 2020; Kalaiyarasan et al., 2020; Tummala et al., 2021; Sekar et al., 2022). When o-phenylenediamine was treated with boric acid, the hydrothermal reaction yielded N, B-CQDs which is yellow fluorescent (Wei et al., 2020). N, B-CQDs were incorporated with HeLa cells to obtain fluorescence bioimaging. N or B plays a significant role in electrical modification and a remodelled surface pattern in co-doping that advances intense radiation features in N and B-CQDs (Wei et al., 2020). Fluorescent N, P-CQD derived from hydrothermal synthesis of Ganoderma lucidum was utilised for in vivo imaging (Tu et al., 2020). Photoluminescent CQDs produced from alginate have enormous potential as bioimaging probes (Zhou et al., 2016). The functional effect of various element dopants on CQDs in generating multiwavelength emission is still under investigation. Bao et al. looked at the in vivo biodistribution of the CQDs utilizing NIR FL imaging of mice without and with tumours to determine if it would be feasible to use CQDs for tumour diagnosis and treatment (Bao X. et al., 2018). Following intravenous infusion of CQDs into mice with H22 tumours, each mouse’s entire body progressively displayed intense near-infrared fluorescence. The whole-body NIR fluorescence intensity had significantly dropped 3 hours after injection, and the tumour area’s NIR fluorescence signal stood out sharply from the surrounding tissues (Figure 8) (Bao X. et al., 2018). Graphene-based CQDs can also be utilized for red and NIR fluorescence bioimaging.
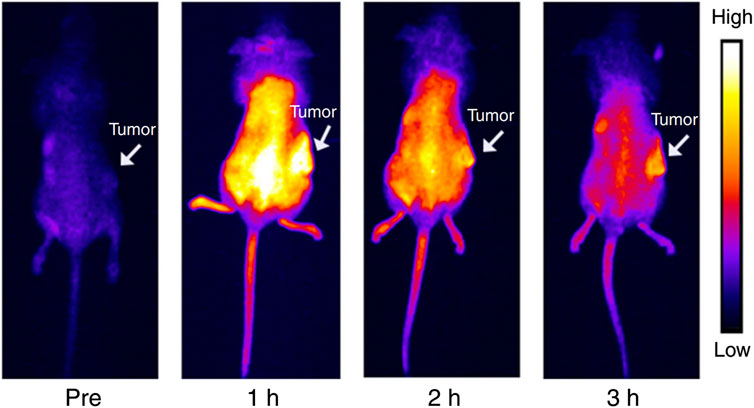
FIGURE 8. NIR emitting CQDs for in vivo imaging: Mouse bodies were imaged using NIR fluorescence at different time intervals following intravenous administration of CQDs (0.2 mL, 1000 μg mL−1). Reprinted (adapted) with permission from Bao X. et al., 2018 Copyright (2018) Nature.
The need for efficient multimodal imaging probes is growing, combining various imaging modalities like magnetic resonance (MRI), photoacoustic (PA), and imaging using computed tomography (CT) (Prabhuswamimath, 2022; Rajasekar et al., 2022; Kanungo et al., 2023; Latha et al., 2023). PA imaging is a hybrid method of imaging that combines optical and ultrasonic modalities for deep tissue penetration and great spatial resolution (Rajasekar et al., 2022; Latha et al., 2023). CQDs exhibit substantial absorption coefficients in the red to near-infrared spectrum when used as PA contrast agents and can transform light into heat (Ge et al., 2015; Prabhuswamimath, 2022). Doping MRI/CT probes into CQDs allows for the formation of further multimodal CQDs as discussed in this section earlier. The spatial resolution and the limited penetration depth with multi-modal CQDs are still under investigation for application in PA, MRI, and CT imaging (Louie, 2010; Zheng et al., 2016; Morato et al., 2021). CQDs have a lot of potential applications in biological imaging as optical nanoprobes in the future (Wang et al., 2022). These findings suggested that cell imaging as an effective technique for in vivo imaging has a bright future. Subsequent research endeavours ought to concentrate on augmenting the QY of CQDs and generating structurally, compositionally, and geometrically well-defined CQDs. When CQD development is unrestricted by size, it can reach incredible ranges of applications.
6 CQDs in biomedicines
Nanotechnology is gaining attention in biomedical applications, particularly in nano-drug delivery systems and nano-cancer imaging (NCI) (Koutsogiannis et al., 2020). In the case of CQDs, the nano-drug delivery methods provide efficient delivery at a fixed rate and time, whilst the latter has the advantage of CQDs providing high sensitivity, improved contrast, and high precision (Azam et al., 2021; Cui et al., 2021; Zhu et al., 2022). The unique photophysical and physicochemical properties of CQDs along with their biocompatibility and facile synthetic route of preparation render CQDs an enticing nanomaterial candidate for biomedical application (Arshad et al., 2020; Azam et al., 2021; Adam et al., 2022). So CQDs have great applications in clinical research. The use of CQDs in drug delivery, gene therapy, and combatting the recent COVID-19 pandemic has been discussed below (Table 4).
6.1 Drug delivery
Like other nanomaterials, CQD-based drug delivery involves drugs onto CQDs through binding or adsorption, for site-specific delivery of the drugs with minimal side effects (Lim et al., 2015; Zhu et al., 2015; Guerrero et al., 2021). The bond between CQD and the drug gets cleaved in the CQD-drug complex in an acidic environment of the diseased site or other stimulating factors (Li et al., 2020). This allows the controlled release of the drug to specific sites. Free CQDs easily get excreted through the renal or hepatobiliary system afterwards (Tian et al., 2020). The controllable surface modification for varying functions, small size, low cost, biocompatibility, and almost no side effects make CQDs an easy choice for the drug target (Figure 9) (Zhang et al., 2018; Lu et al., 2019a). To date, several anticancer and antibacterial drugs have been delivered successfully utilising various modified CQDs (Verma et al., 2020; Mahani et al., 2021).
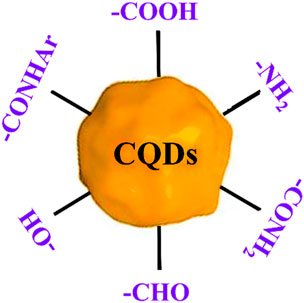
FIGURE 9. Schematic of different functional moieties on CQD which can be synthesized via any preferred methods as discussed in Section 2.
CQDs play a significant part in both the diagnosis and treatment of cancer-like diseases (Palashuddin Sk et al., 2015; Wang et al., 2015; Zhao and Zhu, 2016; Gao et al., 2017; Ghosh et al., 2019a). Doxorubicin is a common chemotherapy medication that has been licensed by the FDA (Li S. et al., 2016). Doxorubicin can be combined using enzymes associated with DNA from malignant cells to speed up DNA base pair intercalation in tumours and inhibit the growth of cancer cells, making it a typical first-line treatment for a variety of malignancies (Tacar et al., 2013). A combination of CQDs and doxorubicin, cisplatin, and docetaxel-like cancer drugs has been developed that exhibits considerable tumour targeting, improved anti-tumour effectiveness, and minimal side effects (Yang et al., 2016; Mahani et al., 2021; Mahani et al., 2021; Sawpari et al., 2023). Carbonized Polymer Dot or CPD has various medical applications (Zhu et al., 2015). CQD/polymer composites exhibit self-release patterns and have important biomedical utilisations in treating cancer (Zhao and Zhu, 2016; Adam et al., 2022; Soumya et al., 2023). They are used in drug delivery systems such as insulin-smart nanocarriers and chemotherapeutic medicines (Devi et al., 2019; Alaghmandfard et al., 2021). The N-doped carbon dots (FN-CQDs) with folic acid are endocytosed specifically (specific cellular absorption rate >93.40%) and remain in autophagic vacuoles in cancer cells for an extended period (Khoshnood et al., 2023). Released FN-CQDs have been proven to effectively kill tumour cells and to be effective against 26 different types of tumour cells by activating the extrinsic and intrinsic apoptotic signalling pathways. Selective tumour targeting of the human glioblastoma cell line (U87MG) was successful in utilizing surface charge modulation of CQD-Doxorubicin incorporating a coating of octylamine-modified polyacrylic acid (cRGD-PAA-OA) and cRGD (Gao et al., 2018). Zhou et. al. produced intensely red emissive CQDs with several coupled α-carboxyl and amino groups to improve drug delivery efficiency and tumour-specific imaging (Liu et al., 2020a; Ding et al., 2020). These CQDs could target tumours such as gliomas because of the large neutral amino acid transporter 1’s multivalent interaction (Liu et al., 2018). Consequently, the CQDs could likewise be used to treat brain tumours by fluorescence/PA imaging while combining with topotecan hydrochloride (Huang et al., 2013; Huang et al., 2013; Li S. et al., 2016; Deng et al., 2018; Li et al., 2020). The schematic representation of CQD-mediated drug delivery is shown in Figure 9 above. CQD on the Porous polycaprolactone (PCL) matrix strengthens the bioactivity for biomineralization and can be used for bone tissue engineering (Luo et al., 2014). Stem cell biology is a significant advancement in biomedicine, with researchers exploring the use of untapped stem cells. Mukherjee et al. propose a strategy using biogenic carbon quantum dots (CQDs) out of garlic peels as a biogenic precursor (Figure 10) (Majood et al., 2023). These CQDs can image mesenchymal stem cells without cytotoxicity and can form reactive oxygen species (ROS) to influence stem cell migration and chondrocyte differentiation without chondrogenic induction factors. The study suggests garlic peel-generated CQDs as a major advancement in stem cell biology.
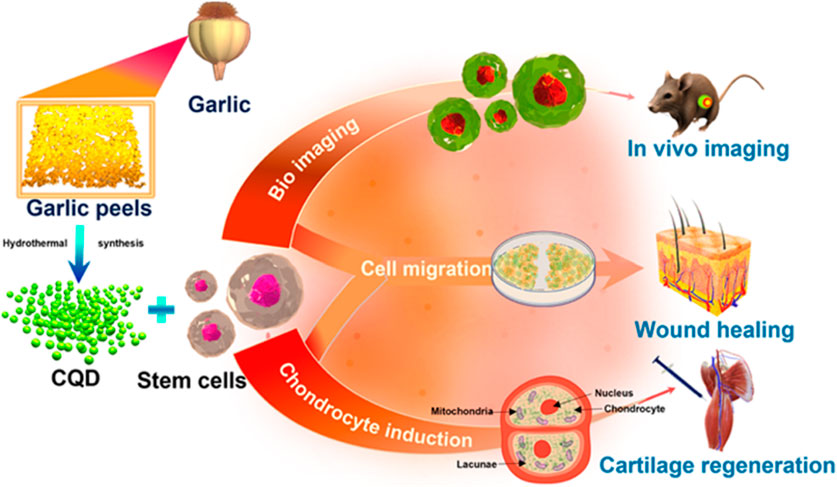
FIGURE 10. CQDs as a biogenic precursor for stem cell biology, demonstrating their ability to mesenchymal stem cell imaging without cytotoxicity and generate reactive oxygen species. Reprinted (adapted) with permission from Majood et al., 2023 (ACS Appl. Mater. Interfaces 2023, 15, 16, 19997–20011) Copyright (2023) ACS.
PEGylation is a common surface functionalization approach used in the CQDs assembly; (CQDs-Pt (IV) @PEG-(PAH/DMMA)), a cisplatin-based platform that stimulates the electrostatic repulsion mechanism that releases positively charged CQDs-Pt (IV) (Feng et al., 2016). In-vivo trials highlight the potential of CQDs for excellent tumour suppression efficacy and few side effects by emphasizing the efficiency of CQDs for transporting cisplatin-based drugs to the site-specific organs for maximal therapeutic effect. Cur-CPDs, CQDs prepared from curcumin, have anti-cancer, and antibacterial, anti-inflammatory, antioxidant properties (Lin et al., 2019). Insulin-smart nanocarriers, a self-release pattern developed by adding insulin to CQD/polymer hydrogels that contain phenyl-boronic acid are widely used in biomedical applications (Adam et al., 2022). An agarose-poly (vinyl alcohol) copolymer and CQDs as a cross-linker were used to generate a pH-responsive hydrogel nanocomposite, and this biodegradable nanocomposite released the antibacterial medication norfloxacin (Date et al., 2020). CQDPAs (Carbon quantum dot polyamines), a highly cationic form of Carbon quantum dot, are used to treat bacterial keratitis and infections due to their potent antibacterial properties (Jian et al., 2017). When compared to negatively charged CQDs, both uncharged and positively charged CQDs demonstrated superior bactericidal action synthesised from ethylene glycol and various amine sources (Verma et al., 2020). CQDs are synthesized from graphene-based materials (GQD) that exhibit characteristics of graphene and can be utilized for biomedical applications. Graphene-based CQDs have a lot of carboxylic groups, which enable them to be functionalized with active biomolecules (Kortel et al., 2020). This makes them useful for developing therapies and delivering drugs (Figure 11). The effectiveness of employing graphene-based CQDs as a low-cytotoxicity inhibitor for the aggregation of Aß peptides as they adhere to the hydrophobic centre of the peptides was demonstrated by Liu et al., 2015. Functionalized GQDs have demonstrated significant effectiveness in recognizing cancer receptors, transporting chemotherapeutic agents—like doxorubicin (DOX) or cisplatin—selectively to the cell nucleus while advancing the cytotoxicity, obstructing the agents’ unintentional transportation into normal cell tissues, and prohibiting drug resistance (Deng et al., 2018). Graphene-based CQDS widely used in photodynamic treatment (PDT) has shown significant therapeutic benefit (Choi et al., 2014). The molecules may be put into the system with the aid of the CQDs, opening the way to the prospect of drug administration with bioimaging capabilities. The functionalized CQDs’ biocompatibility remains a crucial concern for their future use in living cells, tissues, and animals (Tian et al., 2020). This could potentially be a disadvantage for the clinical testing of these CQDs in therapeutic applications in some cases. The disadvantages also include the synthesis of CQDs using different methods such as the need for costly ingredients, severe reaction conditions, and extended reaction times (Section 2).
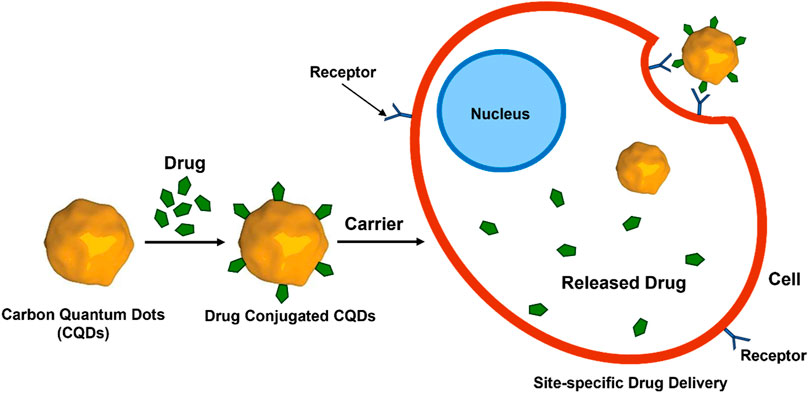
FIGURE 11. CQD-mediated drug delivery to the cells. Drug molecules are conjugated with CQDs followed by binding to the receptor on the cell surface and release of the drug molecules inside the cells.
6.2 Gene therapy
Gene therapy s the technique of modifying a person’s genes to treat or cure an illness (Pham et al., 2023). Gene therapy is regarded as a long-term and potentially curable therapeutic method for a variety of disorders (Dunbar et al., 2018). In this technique, the vector with excellent efficiency of gene transfection can transfer genetic components within the cells. According to reports from the past few years, CQDs can be employed as gene nanocarriers that can be tracked via imaging (Ghosh et al., 2019a; Han and Na, 2019; Pham et al., 2023). Therefore, among various nanoparticles, CQDs take considerable attention in gene therapy as a non-viral vector. Various research has revealed that desirable CQDs/polymeric nanostructures can be a suitable competitor for gene delivery in specific areas like tumours (Molaei, 2019). A hybrid nano-gene vector-based CQDs (CQD-PEI), produced utilizing glycerol along with the branched PEI25k through microwave-aided pyrolysis, has the superior capability to gene transfer because of the outer positive charged polymer layer on the CQDs (Liu et al., 2012). CQDs were employed as a gene carrier for chondrogenesis from fibroblasts (Molaei, 2019). The development of multifunctional nano-carriers has demonstrated potential for use in diagnostics and gene therapy. CQD complexed with PEI and unlabeled plasmid DNA presented real-time monitoring for gene delivery (Kim et al., 2013). While plasmid DNA and polymeric vectors can be labelled with organic dyes and inorganic QDs, research has demonstrated health issues and instability. When compared to PEI alone, CQDs capped with PEI matrix allowed plasmid DNA for a greater transfection into COS-7 and HepG2 cells (Zhu et al., 2022). The hydrogel demonstrated intriguing simultaneous imaging capabilities with several excitation wavelengths, suggesting potential applications for near-infrared emissions imaging in vivo.
Kisor et al. developed CQD-PAMAM conjugates (CDPs) by synthesizing CQDs using peels of sweet lemon as a renewable source and conjugating them with several generations of polyamidoamine (PAMAM) dendrimers (Ghosh et al., 2019b). Between the conjugates of CDP, CDP3 is a potential gene transfer vector for TNBC (triple negative breast cancer) gene therapy because of its better gene complexation along with protection abilities, low level of toxicity, compatibility of blood, and enhanced gene transfection effectiveness, resulting in an intriguing theranostic technique for future therapy (Ghosh et al., 2019b). Furthermore, CDP3 demonstrated extremely specific Cu (II) ion detection, which could assist in determining the metastatic stage of TNBC, which has a larger concentration of Cu (II) ions. To function as an effective pseudo-homogeneous gene transfer vehicle, Ehsan et al. constructed CQDs from chitosan which were functionalized with arginine (Rezaei and Hashemi, 2021). Due to robust photoluminescence properties in both solid and solution states under physiological environments and excellent cellular intake in the human embryonic kidney −293T (HEK-293T) cells without any toxicity, the CQDs decorated with poly-l-lysine (PLL), namely, CQD/PLL core-shell NPs, show significant potential in the application of gene delivery (Hasanzadeh et al., 2021). CQDs, made from sodium alginate, were successfully employed as an effective non-viral gene carrier (Zhou et al., 2016). These CQDs displayed transfection effectiveness that was comparable to lipofectamine, but they also had the added benefits of cell imaging, extremely minimal cytotoxicity, and good biocompatibility. It was also found that multifunctional nanoparticles of polyethyleneimine-based carbon quantum dots (PCD) have the potential to transmit genes within cells. Both sodium alginate-based CQD and PCD can bioimaging probes discussed earlier (Zhou et al., 2016; Han and Na, 2019).
6.3 Combatting COVID-19
COVID-19, a pulmonary viral disease causing severe pulmonary distress with fever, has led to massive global deaths recently (Zhou and Ye, 2021). Scientists are working on rapid diagnosis and therapy when immune cells produce mediators (i.e., interleukins, α-defensins) that induce inflammation (Zhou and Ye, 2021; Soltani-Zangbar et al., 2022). CQDs are employed to diagnose and treat the disease (Xue et al., 2022). The herbs are more effective than medical plants as the precursor of CQDs to fight COVID-19 (Łoczechin et al., 2019; Naik et al., 2022). As an example, curcumin-derived CQDs were effective against enterovirus (EV-71) and garlic-derived CQDs were later found to diminish inflammatory cytokines while reducing coronavirus attachment and penetration (Lin et al., 2019; Kalkal et al., 2021). CQDs have been synthesized by hydrothermal treatment of ethylenediamine and citric acid as precursors of carbon and post-modification with boronic acid ligands, which exhibited concentration-dependent coronaviral inactivation (Łoczechin et al., 2019). The nanoporous membranes made of carbon dots and poly- (vinylidene fluoride) for self-sterilized, recyclable facemasks to combat the virus have been reported (Singh et al., 2021). The composite films have a hydrophobic surface, compact nanopore network, and efficient filtering of particles larger than 100 nm. CQDs have had a significant role in combatting COVID-19.
Systems based on CQDs may find use in the future in antiviral and antibacterial applications. Targeted delivery of medicinal medications loaded on CQDs, biological dye, and biological nanotransporters will become more and more significant. Enhanced photocatalytic antibacterial activity of covalent organic frameworks (COFs) is reported for CQDs as an electron extractant, which encompasses a great possibility for collaboration among material scientists and biochemical researchers for advanced biological and bio-environmental applications (Das et al., 2022b; 2023; Liang et al., 2022).
7 Conclusion
This review provides insight into the various synthetic methods of CQDs, their structure and properties, and the applications of CQDs in bioimaging and biomedicine. The article focuses on recent advancements, future possibilities, and various applications of CQDs and CQDs-based composites, hetero-atom-doped modifications in the field of bioimaging and biomedicines.
The CQDs were found in 2004 during the purification of single-walled carbon nanotubes (SWCNTs) and sanitizing them. Since that time, there have been a lot of techniques reported for the synthesis of CQDs. In general, CQDs can be synthesized using either of two primary methods: top-down or bottom-up. Top-down approaches use physical or chemical mechanisms to split up bigger carbon structures into smaller ones (Wang et al., 2014). Because of their low preparation costs and various advantageous qualities, such as their solubility in aqueous medium, chemical inertness, biocompatibility, and non-toxicity, CQDs have since been used in a wide variety of applications. Right electron doping and right surface passivation on CQDs are utilised in drug delivery due to their biocompatible properties and fluorescent properties. CQDs can be used as carriers for drug delivery, tracking continuous fluorescence emission. We emphasized new applications of CQDs, focusing on the precursors, properties, and synthesis of CQDs following their uses in bioimaging and biomedicine. A variety of biomedical applications have come to rely on CQDs and carbon-based composite materials because of their distinct physiochemical, immune-quiescent, biocompatible, and other characteristics. The powerful light-absorbing ability of CQDs allows the targeted destruction of tumour cells and microorganisms in photothermal therapy, photodynamic therapy, and PTT/PDT combo treatments (Juzenas et al., 2008; Choi et al., 2014). This review’s primary contribution is the suggested framework for the future incorporates ascertaining diverse sources yielding CQDs with adaptable biomedical uses, comprehending the mechanism of action and eventual secretion through renal process from the body.
We have put a special emphasis on highlighting nanocomposites comprising CQDs and composites, featuring efficient uses for bioimaging and biomedicines. The fluorescence off/on the mode of CQD/polymer composites may be applied to detect bioanalytics, hence bringing information from the laboratory scale to commercial and industrial domains (Li W. et al., 2017; Ma et al., 2018; Adam et al., 2022). Preclinical research indicates promise for medication delivery, on-chip labs, cancer therapy, and non-invasive diagnostics. CQDs enable real-time monitoring of the subcellular and tissues, which makes it a quick and easy way to assess patients’ health and an area of great focus in research. Investigations into antivirals based on CQDs are still in their early stages. Currently, targeting and utilizing CQDs to prevent certain viruses is difficult. Research on CQDs-based single-atom nanomedicine aims to clarify the exact mechanism for bio applications and enhance complicated selectivity and loading efficiency. CQDs in polymer matrices (Carbonized Polymer Dot or CPD) and CQDs synthesized from graphene-based materials (GQD) are utilized for various bioimaging and biomedical applications (Liu et al., 2015; Pathak et al., 2023). This helps in the imaging process incorporating various wavelengths, red and NIR wavelengths, easing the drug delivery process, and gene therapy.
More research is needed on CQDs, which offer new applications in bioimaging and biomedicines. Although the synthesis methodologies and potential applications are greatly explored, tuning the sizes of CQDs for desired fluorescence properties and functionalization on CQDs in some cases in a simple, environmentally friendly method is challenging. Future research would focus on raising the QY of CQDs and developing clearly defined geometrically, compositionally, and structurally CQDs. Many unknown features in CQDs might open their extensive use in bioimaging and biomedicines to combat a range of threats in the future.
Author contributions
SD: Writing–original draft, Writing–review and editing. SM: Writing–original draft, Writing–review and editing. DG: Writing–original draft, Writing–review and editing.
Funding
The author(s) declare financial support was received for the research, authorship, and/or publication of this article. Open access funding by ETH Zurich.
Conflict of interest
The authors declare that the research was conducted in the absence of any commercial or financial relationships that could be construed as a potential conflict of interest.
Publisher’s note
All claims expressed in this article are solely those of the authors and do not necessarily represent those of their affiliated organizations, or those of the publisher, the editors and the reviewers. Any product that may be evaluated in this article, or claim that may be made by its manufacturer, is not guaranteed or endorsed by the publisher.
References
Abbas, A., Zhang, C., Hussain, S., Li, Y., Gao, R., Li, J., et al. (2023). A robust switchable oil-in-water emulsion stabilized by electrostatic repulsions between surfactant and similarly charged carbon dots. Small 19, e2206621. doi:10.1002/smll.202206621
Adam, G. O., Sharker, S. M., and Ryu, J. H. (2022). Emerging biomedical applications of carbon dot and polymer composite materials. Appl. Sci. Switz. 12, 10565. doi:10.3390/app122010565
Ahmad, K., Gogoi, S. K., Begum, R., Sk, M. P., Paul, A., and Chattopadhyay, A. (2017). An interactive quantum dot and carbon dot conjugate for pH-sensitive and ratiometric Cu2+ sensing. ChemPhysChem 18, 610–616. doi:10.1002/cphc.201601249
Ahmad, K., Pal, A., Pan, U. N., Chattopadhyay, A., and Paul, A. (2018). Synthesis of single-particle level white-light-emitting carbon dots: via a one-step microwave method. J. Mater Chem. C Mater 6, 6691–6697. doi:10.1039/c8tc01276h
Alaghmandfard, A., Sedighi, O., Tabatabaei Rezaei, N., Abedini, A. A., Malek Khachatourian, A., Toprak, M. S., et al. (2021). Recent advances in the modification of carbon-based quantum dots for biomedical applications. Mater. Sci. Eng. C 120, 111756. doi:10.1016/j.msec.2020.111756
Arshad, F., Pal, A., Alam, T., Khan, J. A., and Sk, M. P. (2020). Luminescent carbogenic dots for the detection and determination of hemoglobin in real samples. New J. Chem. 44, 6213–6221. doi:10.1039/D0NJ00401D
Atchudan, R., Edison, T. N. J. I., Perumal, S., Muthuchamy, N., and Lee, Y. R. (2020). Hydrophilic nitrogen-doped carbon dots from biowaste using dwarf banana peel for environmental and biological applications. Fuel 275, 117821. doi:10.1016/j.fuel.2020.117821
Azam, N., Najabat Ali, M., and Javaid Khan, T. (2021). Carbon quantum dots for biomedical applications: review and analysis. Front. Mater 8. doi:10.3389/fmats.2021.700403
Bao, R., Chen, Z., Zhao, Z., Sun, X., Zhang, J., Hou, L., et al. (2018a). Green and facile synthesis of nitrogen and phosphorus co-doped carbon quantum dots towards fluorescent ink and sensing applications. Nanomaterials 8, 386. doi:10.3390/nano8060386
Bao, X., Yuan, Y., Chen, J., Zhang, B., Li, D., Zhou, D., et al. (2018b). In vivo theranostics with near-infrared-emitting carbon dots—highly efficient photothermal therapy based on passive targeting after intravenous administration. Light Sci. Appl. 7, 91. doi:10.1038/s41377-018-0090-1
Bhunia, S. K., Saha, A., Maity, A. R., Ray, S. C., and Jana, N. R. (2013). Carbon nanoparticle-based fluorescent bioimaging probes. Sci. Rep. 3, 1473. doi:10.1038/srep01473
Biazar, N., Poursalehi, R., and Delavari, H. (2018). Optical and structural properties of carbon dots/TiO2 nanostructures prepared via DC arc discharge in liquid. AIP Conf. Proc. 1920 (1), 020033. doi:10.1063/1.5018965
Campos, B. B., Oliva, M. M., Contreras-Cáceres, R., Rodriguez-Castellón, E., Jiménez-Jiménez, J., da Silva, J. C. G. E., et al. (2016). Carbon dots on based folic acid coated with PAMAM dendrimer as platform for Pt(IV) detection. J. Colloid Interface Sci. 465, 165–173. doi:10.1016/j.jcis.2015.11.059
Cao, L., Wang, X., Meziani, M. J., Lu, F., Wang, H., Luo, P. G., et al. (2007). Carbon dots for multiphoton bioimaging. J. Am. Chem. Soc. 129, 11318–11319. doi:10.1021/ja073527l
Cheki, M., Moslehi, M., and Assadi, M. (2013). Marvelous applications of quantum dots. Eur. Rev. Med. Pharmacol. Sci. 17, 1141–1148.
Chen, L., Raohao, F., Changchang, Z., Xiang, L., and Changhua, Z. (2019). Fluorescent enhancement of polyethyleneimine nano-polymers and the application in cellar imaging. Polym. Degrad. Stab. 163, 7–14. doi:10.1016/j.polymdegradstab.2019.01.013
Cheng, C., Shi, Y., Li, M., Xing, M., and Wu, Q. (2017). Carbon quantum dots from carbonized walnut shells: structural evolution, fluorescence characteristics, and intracellular bioimaging. Mater. Sci. Eng. C 79, 473–480. doi:10.1016/j.msec.2017.05.094
Choi, Y., Kim, S., Choi, M.-H., Ryoo, S.-R., Park, J., Min, D.-H., et al. (2014). Highly biocompatible carbon nanodots for simultaneous bioimaging and targeted photodynamic therapy in vitro and in vivo. Adv. Funct. Mat. 24, 5781–5789. doi:10.1002/adfm.201400961
Cotta, M. A. (2020). Quantum dots and their applications: what lies ahead? ACS Appl. Nano Mater 3, 4920–4924. doi:10.1021/acsanm.0c01386
Cui, L., Ren, X., Sun, M., Liu, H., and Xia, L. (2021). Carbon dots: synthesis, properties and applications. Nanomaterials 11, 3419. doi:10.3390/nano11123419
Cui, L., Ren, X., Wang, J., and Sun, M. (2020). Synthesis of homogeneous carbon quantum dots by ultrafast dual-beam pulsed laser ablation for bioimaging. Mater. Today 12, 100091. doi:10.1016/j.mtnano.2020.100091
Das, P., Ganguly, S., Agarwal, T., Maity, P., Ghosh, S., Choudhary, S., et al. (2019). Heteroatom doped blue luminescent carbon dots as a nano-probe for targeted cell labeling and anticancer drug delivery vehicle. Mater Chem. Phys. 237, 121860. doi:10.1016/j.matchemphys.2019.121860
Das, S., Chowdhury, I. H., Chakraborty, A., Naskar, M. K., Sarkar, M., and Manirul Islam, S. K. (2022a). Porous organic polymer (POP) nanosheets: an efficient photo-catalyst for visible-light assisted CO2 reduction. Mater Adv. 3, 3165–3173. doi:10.1039/d1ma01021b
Das, S., Hazra Chowdhury, I., Hazra Chowdhury, A., Singh, N., Sarkar, M., and Islam, Sk. M. (2022b). Metal-free covalent organic framework for facile production of solar fuel via CO2 reduction. Ind. Eng. Chem. Res. 61, 17044–17056. doi:10.1021/acs.iecr.2c02902
Das, S., Sarkar, P., Goswami, M., Ali, S. M., Mollah, M. R., and Islam, S. M. (2023). A sustainable strategy for the visible-light-driven facile N-formylation of amines using a Co(ii)-embedded covalent organic framework as an efficient photocatalyst. Mater Chem. Front. 7, 3349–3364. doi:10.1039/D3QM00042G
Date, P., Tanwar, A., Ladage, P., Kodam, K. M., and Ottoor, D. (2020). Carbon dots-incorporated pH-responsive agarose-PVA hydrogel nanocomposites for the controlled release of norfloxacin drug. Polym. Bull. 77, 5323–5344. doi:10.1007/s00289-019-03015-3
Deng, J., Lu, Q., Mi, N., Li, H., Liu, M., Xu, M., et al. (2014). Electrochemical synthesis of carbon nanodots directly from alcohols. Chem. – A Eur. J. 20, 4993–4999. doi:10.1002/chem.201304869
Deng, T., Zhang, R., Wang, J., Song, X., Bao, F., Gu, Y., et al. (2018). Carbon dots-cluster-DOX nanocomposites fabricated by a Co-Self-Assembly strategy for tumor-targeted bioimaging and therapy. Part. Part. Syst. Charact. 35. doi:10.1002/ppsc.201800190
Devi, P., Saini, S., and Kim, K.-H. (2019). The advanced role of carbon quantum dots in nanomedical applications. Biosens. Bioelectron. 141, 111158. doi:10.1016/j.bios.2019.02.059
Dey, S., Govindaraj, A., Biswas, K., and Rao, C. N. R. (2014). Luminescence properties of boron and nitrogen doped graphene quantum dots prepared from arc-discharge-generated doped graphene samples. Chem. Phys. Lett. 595–596, 203–208. doi:10.1016/j.cplett.2014.02.012
Ding, H., Zhou, X. X., Wei, J. S., Li, X. B., Qin, B. T., Chen, X. B., et al. (2020). Carbon dots with red/near-infrared emissions and their intrinsic merits for biomedical applications. Carbon N. Y. 167, 322–344. doi:10.1016/j.carbon.2020.06.024
Doñate-Buendía, C., Fernández-Alonso, M., Lancis, J., and Mínguez-Vega, G. (2020). Pulsed laser ablation in liquids for the production of gold nanoparticles and carbon quantum dots: from plasmonic to fluorescence and cell labelling. J. Phys. Conf. Ser. 1537, 012013. doi:10.1088/1742-6596/1537/1/012013
Dong, S., Yuan, Z., Zhang, L., Lin, Y., and Lu, C. (2017). Rapid screening of oxygen states in carbon quantum dots by chemiluminescence probe. Anal. Chem. 89, 12520–12526. doi:10.1021/acs.analchem.7b03711
Dong, Y., Zhou, N., Lin, X., Lin, J., Chi, Y., and Chen, G. (2010). Extraction of electrochemiluminescent oxidized carbon quantum dots from activated carbon. Chem. Mater. 22, 5895–5899. doi:10.1021/cm1018844
Dubey, A., Mondal, S., Chandra, K., and Atreya, H. S. (2016). Rapid identification of amino acid types in proteins using phase modulated 2D HN(CACB) and 2D HN(COCACB). J. Magnetic Reson. 267, 22–29. doi:10.1016/j.jmr.2016.04.004
Dunbar, C. E., High, K. A., Joung, J. K., Kohn, D. B., Ozawa, K., and Sadelain, M. (2018). Gene therapy comes of age. Sci. Wash. D.C. U. S.) 359, eaan4672. doi:10.1126/science.aan4672
El-brolsy, H. M. E. M., Hanafy, N. A. N., and El-Kemary, M. A. (2022). Fighting non-small lung cancer cells using optimal functionalization of targeted carbon quantum dots derived from natural sources might provide potential therapeutic and cancer bio image strategies. Int. J. Mol. Sci. 23, 13283. doi:10.3390/ijms232113283
Feng, T., Ai, X., An, G., Yang, P., and Zhao, Y. (2016). Charge-convertible carbon dots for imaging-guided drug delivery with enhanced in vivo cancer therapeutic efficiency. ACS Nano 10, 4410–4420. doi:10.1021/acsnano.6b00043
Feng, X., and Zhang, Y. (2019). A simple and green synthesis of carbon quantum dots from coke for white light-emitting devices. RSC Adv. 9, 33789–33793. doi:10.1039/c9ra06946a
Feng, Z., Adolfsson, K. H., Xu, Y., Fang, H., Hakkarainen, M., and Wu, M. (2021). Carbon dot/polymer nanocomposites: from green synthesis to energy, environmental and biomedical applications. Sustain. Mater. Technol. 29, e00304. doi:10.1016/j.susmat.2021.e00304
Gangasani, S., and Student, B. E. (2007). A study on quantum dot and its applications. Int. J. Innovative Res. Sci. Eng. Technol. 5, 3297. doi:10.15680/IJIRSET.2016.0505119
Gao, G., Jiang, Y. W., Yang, J., and Wu, F. G. (2017). Mitochondria-targetable carbon quantum dots for differentiating cancerous cells from normal cells. Nanoscale 9, 18368–18378. doi:10.1039/c7nr06764j
Gao, L., Zhao, X., Wang, J., Wang, Y., Yu, L., Peng, H., et al. (2018). Multiple functionalized carbon quantum dots for targeting glioma and tissue imaging. Opt. Mater (Amst) 75, 764–769. doi:10.1016/j.optmat.2017.11.044
García de Arquer, F. P., Talapin, D. V., Klimov, V. I., Arakawa, Y., Bayer, M., and Sargent, E. H. (2023). Semiconductor quantum dots: technological progress and future challenges. Science 373, eaaz8541. doi:10.1126/science.aaz8541
Ge, J., Jia, Q., Liu, W., Guo, L., Liu, Q., Lan, M., et al. (2015). Red-emissive carbon dots for fluorescent, photoacoustic, and thermal theranostics in living mice. Adv. Mater. 27, 4169–4177. doi:10.1002/adma.201500323
Gedda, G., Sankaranarayanan, S. A., Putta, C. L., Gudimella, K. K., Rengan, A. K., and Girma, W. M. (2023). Green synthesis of multi-functional carbon dots from medicinal plant leaves for antimicrobial, antioxidant, and bioimaging applications. Sci. Rep. 13, 6371. doi:10.1038/s41598-023-33652-8
Ghosh, S., Ghosal, K., Mohammad, S. A., and Sarkar, K. (2019a). Dendrimer functionalized carbon quantum dot for selective detection of breast cancer and gene therapy. Chem. Eng. J. 373, 468–484. doi:10.1016/j.cej.2019.05.023
Ghosh, S., Ghosal, K., Mohammad, S. A., and Sarkar, K. (2019b). Dendrimer functionalized carbon quantum dot for selective detection of breast cancer and gene therapy. Chem. Eng. J. 373, 468–484. doi:10.1016/j.cej.2019.05.023
Guerrero, E. D., Lopez-Velazquez, A. M., Ahlawat, J., and Narayan, M. (2021). Carbon quantum dots for treatment of amyloid disorders. ACS Appl. Nano Mater 4, 2423–2433. doi:10.1021/acsanm.0c02792
Gurung, S., Neha, , Arun, N., Joshi, M., Jaiswal, T., Pathak, A. P., et al. (2023). Dual metal ion (Fe3+ and As3+) sensing and cell bioimaging using fluorescent carbon quantum dots synthesised from Cynodon dactylon. Chemosphere 339, 139638. doi:10.1016/j.chemosphere.2023.139638
Han, J., and Na, K. (2019). Transfection of the TRAIL gene into human mesenchymal stem cells using biocompatible polyethyleneimine carbon dots for cancer gene therapy. J. Ind. Eng. Chem. 80, 722–728. doi:10.1016/j.jiec.2019.02.015
Han, S., Zhang, H., Xie, Y., Liu, L., Shan, C., Li, X., et al. (2015). Application of cow milk-derived carbon dots/Ag NPs composite as the antibacterial agent. Appl. Surf. Sci. 328, 368–373. doi:10.1016/j.apsusc.2014.12.074
Hasanzadeh, A., Mofazzal Jahromi, M. A., Abdoli, A., Mohammad-Beigi, H., Fatahi, Y., Nourizadeh, H., et al. (2021). Photoluminescent carbon quantum dot/poly-L-Lysine core-shell nanoparticles: a novel candidate for gene delivery. J. Drug Deliv. Sci. Technol. 61, 102118. doi:10.1016/j.jddst.2020.102118
Havrdová, M., Urbančič, I., Bartoň Tománková, K., Malina, L., Štrancar, J., and Bourlinos, A. B. (2021). Self-targeting of carbon dots into the cell nucleus: diverse mechanisms of toxicity in NIH/3T3 and l929 cells. Int. J. Mol. Sci. 22, 5608. doi:10.3390/ijms22115608
Havrdová, M., Urbančič, I., Tománková, K. B., Malina, L., Poláková, K., Štrancar, J., et al. (2022). Intracellular trafficking of cationic carbon dots in cancer cell lines MCF-7 and HeLa—time lapse microscopy, concentration-dependent uptake, viability, DNA damage, and cell cycle profile. Int. J. Mol. Sci. 23, 1077. doi:10.3390/ijms23031077
Hou, Y., Lu, Q., Deng, J., Li, H., and Zhang, Y. (2015). One-pot electrochemical synthesis of functionalized fluorescent carbon dots and their selective sensing for mercury ion. Anal. Chim. Acta 866, 69–74. doi:10.1016/j.aca.2015.01.039
Huang, C., Dong, H., Su, Y., Wu, Y., Narron, R., and Yong, Q. (2019). Synthesis of carbon quantum dot nanoparticles derived from byproducts in bio-refinery process for cell imaging and in vivo bioimaging. Nanomaterials 9, 387. doi:10.3390/nano9030387
Huang, H., Cui, Y., Liu, M., Chen, J., Wan, Q., Wen, Y., et al. (2018). A one-step ultrasonic irradiation assisted strategy for the preparation of polymer-functionalized carbon quantum dots and their biological imaging. J. Colloid Interface Sci. 532, 767–773. doi:10.1016/j.jcis.2018.07.099
Huang, X., Zhang, F., Zhu, L., Choi, K. Y., Guo, N., Guo, J., et al. (2013). Effect of injection routes on the biodistribution, clearance, and tumor uptake of carbon dots. ACS Nano 7, 5684–5693. doi:10.1021/nn401911k
Huo, X., Liu, L., Bai, Y., Qin, J., Yuan, L., and Feng, F. (2022). Facile synthesis of yellowish-green emitting carbon quantum dots and their applications for phoxim sensing and cellular imaging. Anal. Chim. Acta 1206, 338685. doi:10.1016/j.aca.2021.338685
Jaipuria, G., Krishnarjuna, B., Mondal, S., Dubey, A., and Atreya, H. S. (2012). Amino acid selective labeling and unlabeling for protein resonance assignments. Adv. Exp. Med. Biol. 992, 95–118. doi:10.1007/978-94-007-4954-2_6
Jaipuria, G., Shet, D., Malik, S., Swain, M., Atreya, H. S., Galea, C. A., et al. (2022). IGF-dependent dynamic modulation of a protease cleavage site in the intrinsically disordered linker domain of human IGFBP2. Proteins 90, 1732–1743. doi:10.1002/prot.26350
Jian, H.-J., Wu, R.-S., Lin, T.-Y., Li, Y.-J., Lin, H.-J., Harroun, S. G., et al. (2017). Super-cationic carbon quantum dots synthesized from spermidine as an eye drop formulation for topical treatment of bacterial keratitis. ACS Nano 11, 6703–6716. doi:10.1021/acsnano.7b01023
Jiao, M., Wang, Y., Wang, W., Zhou, X., Xu, J., Xing, Y., et al. (2022). Gadolinium doped red-emissive carbon dots as targeted theranostic agents for fluorescence and MR imaging guided cancer phototherapy. Chem. Eng. J. 440, 135965. doi:10.1016/j.cej.2022.135965
Juzenas, P., Chen, W., Sun, Y. P., Coelho, M., Generalov, R., Generalova, N., et al. (2008). Quantum dots and nanoparticles for photodynamic and radiation therapies of cancer. Adv. Drug Deliv. Rev. 60, 1600–1614. doi:10.1016/j.addr.2008.08.004
Kabanov, A. V., and Gendelman, H. E. (2007). Nanomedicine in the diagnosis and therapy of neurodegenerative disorders. Prog. Polym. Sci. Oxf. 32, 1054–1082. doi:10.1016/j.progpolymsci.2007.05.014
Kalaiyarasan, G., Joseph, J., and Kumar, P. (2020). Phosphorus-doped carbon quantum dots as fluorometric probes for iron detection. ACS Omega 5, 22278–22288. doi:10.1021/acsomega.0c02627
Kalkal, A., Allawadhi, P., Pradhan, R., Khurana, A., Bharani, K. K., and Packirisamy, G. (2021). Allium sativum derived carbon dots as a potential theranostic agent to combat the COVID-19 crisis. Sensors Int. 2, 100102. doi:10.1016/j.sintl.2021.100102
Kanungo, S., Gupta, N., Rawat, R., Jain, B., Solanki, A., Panday, A., et al. (2023). Doped carbon quantum dots reinforced hydrogels for sustained delivery of molecular cargo. J. Funct. Biomater. 14, 166. doi:10.3390/jfb14030166
Kausar, A. (2019). Polymer/carbon-based quantum dot nanocomposite: forthcoming materials for technical application. J. Macromol. Sci. Part A 56, 341–356. doi:10.1080/10601325.2019.1578614
Khoshnood, A., Farhadian, N., Abnous, K., Matin, M. M., Ziaee, N., and Yaghoobi, E. (2023). N doped-carbon quantum dots with ultra-high quantum yield photoluminescent property conjugated with folic acid for targeted drug delivery and bioimaging applications. J. Photochem Photobiol. A Chem. 444, 114972. doi:10.1016/j.jphotochem.2023.114972
Kim, J., Park, J., Kim, H., Singha, K., and Kim, W. J. (2013). Transfection and intracellular trafficking properties of carbon dot-gold nanoparticle molecular assembly conjugated with PEI-pDNA. Biomaterials 34, 7168–7180. doi:10.1016/j.biomaterials.2013.05.072
Knoblauch, C., Griep, M., and Friedrich, C. (2014). Recent advances in the field of bionanotechnology: an insight into optoelectric bacteriorhodopsin, quantum dots, and noble metal nanoclusters. Sensors (Basel) 14, 19731–19766. doi:10.3390/s141019731
Ko, N., Nafiujjaman, M., Cherukula, K., Lee, S., Hong, S.-J., Lim, H.-N., et al. (2017). Microwave-assisted synthesis of biocompatible silk fibroin-based carbon quantum dots. Part. Part. Syst. Charact. 35, 1700300. doi:10.1002/ppsc.201700300
Kortel, M., Mansuriya, B. D., Santana, N. V., and Altintas, Z. (2020). Graphene quantum dots as flourishing nanomaterials for bio-imaging, therapy development, and micro-supercapacitors. Micromachines (Basel) 11, 866. doi:10.3390/MI11090866
Koutsogiannis, P., Thomou, E., Stamatis, H., Gournis, D., and Rudolf, P. (2020). Advances in fluorescent carbon dots for biomedical applications. Adv. Phys. X 5, 1758592. doi:10.1080/23746149.2020.1758592
Lan, M., Zhao, S., Zhang, Z., Yan, L., Guo, L., Niu, G., et al. (2017). Two-photon-excited near-infrared emissive carbon dots as multifunctional agents for fluorescence imaging and photothermal therapy. Nano Res. 10, 3113–3123. doi:10.1007/s12274-017-1528-0
Latha, B. D., Soumya, K., More, N., Mounika, C., Guduru, A. T., Singh, G., et al. (2023). Fluorescent carbon quantum dots for effective tumor diagnosis: a comprehensive review. Biomed. Eng. Adv. 5, 100072. doi:10.1016/j.bea.2023.100072
Li, C., Wang, Y., Zhang, X., Guo, X., Kang, X., Du, L., et al. (2018). Red fluorescent carbon dots with phenylboronic acid tags for quick detection of Fe(III) in PC12 cells. J. Colloid Interface Sci. 526, 487–496. doi:10.1016/j.jcis.2018.05.017
Li, H., He, X., Kang, Z., Huang, H., Liu, Y., Liu, J., et al. (2010). Water-soluble fluorescent carbon quantum dots and photocatalyst design. Angew. Chem. Int. Ed. 49, 4430–4434. doi:10.1002/anie.200906154
Li, J.-Y., Liu, Y., Shu, Q.-W., Liang, J.-M., Zhang, F., Chen, X.-P., et al. (2017a). One-Pot hydrothermal synthesis of carbon dots with efficient up- and down-converted photoluminescence for the sensitive detection of morin in a dual-readout assay. Langmuir 33, 1043–1050. doi:10.1021/acs.langmuir.6b04225
Li, M., Hu, C., Yu, C., Wang, S., Zhang, P., and Qiu, J. (2015). Organic amine-grafted carbon quantum dots with tailored surface and enhanced photoluminescence properties. Carbon N. Y. 91, 291–297. doi:10.1016/j.carbon.2015.04.083
Li, S., Amat, D., Peng, Z., Vanni, S., Raskin, S., De Angulo, G., et al. (2016a). Transferrin conjugated nontoxic carbon dots for doxorubicin delivery to target pediatric brain tumor cells. Nanoscale 8, 16662–16669. doi:10.1039/c6nr05055g
Li, S., Su, W., Wu, H., Yuan, T., Yuan, C., Liu, J., et al. (2020). Targeted tumour theranostics in mice via carbon quantum dots structurally mimicking large amino acids. Nat. Biomed. Eng. 4, 704–716. doi:10.1038/s41551-020-0540-y
Li, W., Zhang, H., Zheng, Y., Chen, S., Liu, Y., Zhuang, J., et al. (2017b). Multifunctional carbon dots for highly luminescent orange-emissive cellulose based composite phosphor construction and plant tissue imaging. Nanoscale 9, 12976–12983. doi:10.1039/c7nr03217j
Li, X., Wang, H., Shimizu, Y., Pyatenko, A., Kawaguchi, K., and Koshizaki, N. (2011). Preparation of carbon quantum dots with tunable photoluminescence by rapid laser passivation in ordinary organic solvents. Chem. Commun. 47, 932–934. doi:10.1039/C0CC03552A
Li, Y.-J., Harroun, S. G., Su, Y.-C., Huang, C.-F., Unnikrishnan, B., Lin, H.-J., et al. (2016b). Synthesis of self-assembled spermidine-carbon quantum dots effective against multidrug-resistant bacteria. Adv. Healthc. Mat. 5, 2545–2554. doi:10.1002/adhm.201600297
Liang, J., Li, W., Chen, J., Huang, X., Liu, Y., Zhang, X., et al. (2022). Carbon dots as an electron extractant for enhanced photocatalytic antibacterial activity of covalent organic frameworks. J. Mater Chem. A Mater 451, 23384–23394. doi:10.1039/d2ta03978h
Liang, Q., Ma, W., Shi, Y., Li, Z., and Yang, X. (2013). Easy synthesis of highly fluorescent carbon quantum dots from gelatin and their luminescent properties and applications. Carbon N. Y. 60, 421–428. doi:10.1016/j.carbon.2013.04.055
Lim, S. Y., Shen, W., and Gao, Z. (2015). Carbon quantum dots and their applications. Chem. Soc. Rev. 44, 362–381. doi:10.1039/c4cs00269e
Lin, C., Chang, L., Chu, H., Lin, H., Chang, P., Wang, R. Y. L., et al. (2019). High amplification of the antiviral activity of curcumin through transformation into carbon quantum dots. Small 15, 1902641. doi:10.1002/smll.201902641
Liu, C., Zhang, P., Zhai, X., Tian, F., Li, W., Yang, J., et al. (2012). Nano-carrier for gene delivery and bioimaging based on carbon dots with PEI-passivation enhanced fluorescence. Biomaterials 33, 3604–3613. doi:10.1016/j.biomaterials.2012.01.052
Liu, H., Ye, T., and Mao, C. (2007). Fluorescent carbon nanoparticles derived from candle soot. Angew. Chem. - Int. Ed. 46, 6473–6475. doi:10.1002/anie.200701271
Liu, J., Geng, Y., Li, D., Yao, H., Huo, Z., Li, Y., et al. (2020a). Deep red emissive carbonized polymer dots with unprecedented narrow full width at half maximum. Adv. Mater. 32, e1906641. doi:10.1002/adma.201906641
Liu, J., Li, R., and Yang, B. (2020b). Carbon dots: a new type of carbon-based nanomaterial with wide applications. ACS Cent. Sci. 6, 2179–2195. doi:10.1021/acscentsci.0c01306
Liu, R., Wu, D., Liu, S., Koynov, K., Knoll, W., and Li, Q. (2009). An aqueous route to multicolor photoluminescent carbon dots using silica spheres as carriers. Angew. Chem. Int. Ed. Engl. 48, 4598–4601. doi:10.1002/anie.200900652
Liu, Y., Liu, J., Zhang, J., Li, X., Lin, F., Zhou, N., et al. (2018). Noninvasive brain tumor imaging using red emissive carbonized polymer dots across the blood-brain barrier. ACS Omega 3, 7888–7896. doi:10.1021/acsomega.8b01169
Liu, Y., Xu, L.-P., Dai, W., Dong, H., Wen, Y., and Zhang, X. (2015). Graphene quantum dots for the inhibition of β amyloid aggregation. Nanoscale 7, 19060–19065. doi:10.1039/C5NR06282A
Łoczechin, A., Séron, K., Barras, A., Giovanelli, E., Belouzard, S., Chen, Y. T., et al. (2019). Functional carbon quantum dots as medical countermeasures to human coronavirus. ACS Appl. Mater Interfaces 11, 42964–42974. doi:10.1021/acsami.9b15032
Louie, A. (2010). Multimodality imaging probes: design and challenges. Chem. Rev. 110, 3146–3195. doi:10.1021/cr9003538
Lu, S., Liu, L., Wang, H., Zhao, W., Li, Z., Qu, Z., et al. (2019a). Synthesis of dual functional gallic-acid-based carbon dots for bioimaging and antitumor therapy. Biomater. Sci. 7, 3258–3265. doi:10.1039/c9bm00570f
Lu, S., Liu, L., Wang, H., Zhao, W., Li, Z., Qu, Z., et al. (2019b). Synthesis of dual functional gallic-acid-based carbon dots for bioimaging and antitumor therapy. Biomater. Sci. 7, 3258–3265. doi:10.1039/C9BM00570F
Luo, P. G., Sahu, S., Yang, S.-T., Sonkar, S. K., Wang, J., Wang, H., et al. (2013). Carbon “quantum” dots for optical bioimaging. J. Mater Chem. B 1, 2116. doi:10.1039/c3tb00018d
Luo, P. G., Yang, F., Yang, S. T., Sonkar, S. K., Yang, L., Broglie, J. J., et al. (2014). Carbon-based quantum dots for fluorescence imaging of cells and tissues. RSC Adv. 4, 10791. doi:10.1039/c3ra47683a
Ma, C., Dai, K., Hou, H., Ji, X., Chen, L., Ivey, D. G., et al. (2018). High ion-conducting solid-state composite electrolytes with carbon quantum dot nanofillers. Adv. Sci. 5, 1700996. doi:10.1002/advs.201700996
Ma, Y., Zhang, M., Wang, H., Wang, B., Huang, H., Liu, Y., et al. (2020). N-doped carbon dots derived from leaves with low toxicity via damaging cytomembrane for broad-spectrum antibacterial activity. Mater Today Commun. 24, 101222. doi:10.1016/j.mtcomm.2020.101222
Magesh, V., Sundramoorthy, A. K., and Ganapathy, D. (2022). Recent advances on synthesis and potential applications of carbon quantum dots. Front. Mater 9. doi:10.3389/fmats.2022.906838
Mahani, M., Pourrahmani-Sarbanani, M., Yoosefian, M., Divsar, F., Mousavi, S. M., and Nomani, A. (2021). Doxorubicin delivery to breast cancer cells with transferrin-targeted carbon quantum dots: an in vitro and in silico study. J. Drug Deliv. Sci. Technol. 62, 102342. doi:10.1016/j.jddst.2021.102342
Mai, X.-D., Phan, Y. T. H., and Nguyen, V.-Q. (2020). Excitation-independent emission of carbon quantum dot solids. Adv. Mater. Sci. Eng. 2020, 1–5. doi:10.1155/2020/9643168
Majood, M., Selvam, A., Agrawal, O., Chaurasia, R., Rawat, S., Mohanty, S., et al. (2023). Biogenic carbon quantum dots as a neoteric inducer in the game of directing chondrogenesis. ACS Appl. Mater Interfaces 15, 19997–20011. doi:10.1021/acsami.3c02007
Malik, S. A., Mondal, S., and Atreya, H. S. (2019). Enhanced stability of an intrinsically disordered protein against proteolytic cleavage through interactions with silver nanoparticles. RSC Adv. 9 (49), 28746–28753. doi:10.1039/c9ra05514b
Marković, Z. M., Budimir, M. D., Danko, M., Milivojević, D. D., Kubat, P., Zmejkoski, D. Z., et al. (2023). Structural, optical, and bioimaging characterization of carbon quantum dots solvothermally synthesized from o -phenylenediamine. Beilstein J. Nanotechnol. 14, 165–174. doi:10.3762/bjnano.14.17
Mingcong, R., Feng, Y., Wang, Y., and Chen, X. (2017). One-pot solid phase pyrolysis synthesis of nitrogen-doped carbon dots for Fe3+ sensing and bioimaging. Sens. Actuators B Chem. 245, 868–874. doi:10.1016/j.snb.2017.02.014
Molaei, M. J. (2019). Carbon quantum dots and their biomedical and therapeutic applications: a review. RSC Adv. 9, 6460–6481. doi:10.1039/c8ra08088g
Mondal, S., Shet, D., Prasanna, C., and Atreya, H. S. (2013). High yield expression of proteins in E. coli for NMR studies. Adv. Biosci. Biotechnol. 04, 751–767. doi:10.4236/abb.2013.46099
Mondal, S., Thirupathi, R., and Atreya, H. S. (2015). Carbon quantum dots as a macromolecular crowder. RSC Adv. 5, 4489–4492. doi:10.1039/c4ra14019b
Mondal, S., Thirupathi, R., Rao, L. P., Atreya, H. S., Dreyer, D. R., Park, S., et al. (2016). Unraveling the dynamic nature of protein–graphene oxide interactions. RSC Adv. 6, 52539–52548. doi:10.1039/C6RA03759C
Moniruzzaman, M., Dutta, S. D., Lim, K. T., and Kim, J. (2022). Perylene-derived hydrophilic carbon dots with polychromatic emissions as superior bioimaging and NIR-responsive photothermal bactericidal agent. ACS Omega 7, 37388–37400. doi:10.1021/acsomega.2c04130
Morato, Y. L., Paredes, K. O., Chamizo, L. L., Marciello, M., and Filice, M. (2021). Recent advances in multimodal molecular imaging of cancer mediated by hybrid magnetic nanoparticles. Polym. (Basel) 13, 2989. doi:10.3390/polym13172989
Mousa, M. A., Abdelrahman, H. H., Fahmy, M. A., Ebrahim, D. G., and Moustafa, A. H. E. (2023). Pure and doped carbon quantum dots as fluorescent probes for the detection of phenol compounds and antibiotics in aquariums. Sci. Rep. 13, 12863. doi:10.1038/s41598-023-39490-y
Naik, K., Chaudhary, S., Ye, L., and Parmar, A. S. (2022). A strategic review on carbon quantum dots for cancer-diagnostics and treatment. Front. Bioeng. Biotechnol. 10, 882100. doi:10.3389/fbioe.2022.882100
Nasrin, A., Hassan, M., Mann, G., and Gomes, V. G. (2020). Conjugated ternary doped carbon dots from vitamin B derivative: multispectral nanoprobes for targeted melanoma bioimaging and photosensitization. J. Lumin 217, 116811. doi:10.1016/j.jlumin.2019.116811
Nazir, S., Hussain, T., Ayub, A., Rashid, U., and MacRobert, A. J. (2014). Nanomaterials in combating cancer: therapeutic applications and developments. Nanomedicine 10, 19–34. doi:10.1016/j.nano.2013.07.001
Niu, F., Xu, Y., Liu, J., Song, Z., Liu, M., and Liu, J. (2017a). Controllable electrochemical/electroanalytical approach to generate nitrogen-doped carbon quantum dots from varied amino acids: pinpointing the utmost quantum yield and the versatile photoluminescent and electrochemiluminescent applications. Electrochim Acta 236, 239–251. doi:10.1016/j.electacta.2017.03.085
Niu, N., Ma, Z., He, F., Li, S., Li, J., Liu, S., et al. (2017b). Preparation of carbon dots for cellular imaging by the molecular aggregation of cellulolytic enzyme lignin. Langmuir 33, 5786–5795. doi:10.1021/acs.langmuir.7b00617
Pal, A., Ahmad, K., Dutta, D., and Chattopadhyay, A. (2019). Boron doped carbon dots with unusually high photoluminescence quantum yield for ratiometric intracellular pH sensing. ChemPhysChem 20, 1018–1027. doi:10.1002/cphc.201900140
Palashuddin Sk, M., Goswami, U., Ghosh, S. S., and Chattopadhyay, A. (2015). Cu2+-embedded carbon nanoparticles as anticancer agents. J. Mater Chem. B 3, 5673–5677. doi:10.1039/C5TB00567A
Pan, Q., Xu, Z., Deng, S., Zhang, F., Li, H., Cheng, Y., et al. (2019). A mechanochemically synthesized porous organic polymer derived CQD/chitosan-graphene composite film electrode for electrochemiluminescence determination of dopamine. RSC Adv. 9, 39332–39337. doi:10.1039/c9ra06912g
Park, S. Y., Lee, H., Park, E., Lee, S., Lee, J., Jeong, S., et al. (2014). Photoluminescent green carbon nanodots from food-waste-derived sources: large-scale synthesis, properties, and biomedical applications. ACS Appl. Mater Interfaces 6, 3365–3370. doi:10.1021/am500159p
Pathak, R., Punetha, V. D., Bhatt, S., and Punetha, M. (2023). Multifunctional role of carbon dot-based polymer nanocomposites in biomedical applications: a review. J. Mater Sci. 58, 6419–6443. doi:10.1007/s10853-023-08408-4
Peng, Z., Ji, C., Zhou, Y., Zhao, T., and Leblanc, R. M. (2020). Polyethylene glycol (PEG) derived carbon dots: preparation and applications. Appl. Mater Today 20, 100677. doi:10.1016/j.apmt.2020.100677
Pham, Q. D. M., Thomson, S. M., Schaible, B. N., Mills, K. D., Atala, A., Porada, C. D., et al. (2023). Acceptability of prenatal diagnosis and prenatal treatment of haemophilia using cell and gene therapies within US haemophilia community. Haemophilia 29, 1024–1031. doi:10.1111/hae.14805
Pierrat, P., Wang, R., Kereselidze, D., Lux, M., Didier, P., Kichler, A., et al. (2015). Efficient invitro and invivo pulmonary delivery of nucleic acid by carbon dot-based nanocarriers. Biomaterials 51, 290–302. doi:10.1016/j.biomaterials.2015.02.017
Pires, N. R., Santos, C. M. W., Sousa, R. R., De Paula, R. C. M., Cunha, P. L. R., and Feitosa, J. P. A. (2015). Novel and fast microwave-assisted synthesis of carbon quantum dots from raw cashew gum. J. Braz Chem. Soc. 26, 1274–1282. doi:10.5935/0103-5053.20150094
Pourmadadi, M., Rahmani, E., Rajabzadeh-Khosroshahi, M., Samadi, A., Behzadmehr, R., Rahdar, A., et al. (2023). Properties and application of carbon quantum dots (CQDs) in biosensors for disease detection: a comprehensive review. J. Drug Deliv. Sci. Technol. 80, 104156. doi:10.1016/j.jddst.2023.104156
Prabhuswamimath, S. C. (2022). “Chapter 9 - PA Imaging: a promising tool for targeted therapeutic implications in Cancer,” in Biomedical imaging instrumentation. Editors M. Suar, N. Misra, and N. S. Bhavesh (Academic Press), 131–160. doi:10.1016/B978-0-323-85650-8.00009-7
Praneerad, J., Nueangnoraj, K., In, I., and Paoprasert, P. (2019). Environmentally friendly supercapacitor based on carbon dots from durian peel as an electrode. Key Eng. Mater 803, 115–119. doi:10.4028/www.scientific.net/KEM.803.115
Qi, L., and Gao, X. (2008). Emerging application of quantum dots for drug delivery and therapy. Expert Opin. Drug Deliv. 5, 263–267. doi:10.1517/17425247.5.3.263
Qian, J., Quan, F., Zhao, F., Wu, C., Wang, Z., and Zhou, L. (2018). Aconitic acid derived carbon dots: conjugated interaction for the detection of folic acid and fluorescence targeted imaging of folate receptor overexpressed cancer cells. Sens. Actuators B Chem. 262, 444–451. doi:10.1016/j.snb.2018.01.227
Qian, Z., Shan, X., Chai, L., Ma, J., Chen, J., and Feng, H. (2014). Si-doped carbon quantum dots: a facile and general preparation strategy, bioimaging application, and multifunctional sensor. ACS Appl. Mater Interfaces 6, 6797–6805. doi:10.1021/am500403n
Rajasekar, B., Nirmala, P., Bhuvaneswari, P., Radhika, R., Asha, S., Kavitha, K. R., et al. (2022). A feasible multimodal photoacoustic imaging approach for evaluating the clinical symptoms of inflammatory arthritis. Biomed. Res. Int. 2022, 1–12. doi:10.1155/2022/7358575
Ren, R., Zhang, Z., Zhao, P., Shi, J., Han, K., Yang, Z., et al. (2019a). Facile and one-step preparation carbon quantum dots from biomass residue and their applications as efficient surfactants. J. Dispers. Sci. Technol. 40, 627–633. doi:10.1080/01932691.2018.1475239
Ren, X., Zhang, F., Guo, B., Gao, N., and Zhang, X. (2019b). Synthesis of N-doped Micropore carbon quantum dots with high quantum yield and dual-wavelength photoluminescence emission from biomass for cellular imaging. Nanomaterials 9, 495. doi:10.3390/nano9040495
Rezaei, A., and Hashemi, E. (2021). A pseudohomogeneous nanocarrier based on carbon quantum dots decorated with arginine as an efficient gene delivery vehicle. Sci. Rep. 11, 13790. doi:10.1038/s41598-021-93153-4
Ross, S., Wu, R. S., Wei, S. C., Ross, G. M., and Chang, H. T. (2020). The analytical and biomedical applications of carbon dots and their future theranostic potential: a review. J. Food Drug Anal. 28, 678–696. doi:10.38212/2224-6614.1154
Sahiner, N., Suner, S. S., Sahiner, M., and Silan, C. (2019). Nitrogen and sulfur doped carbon dots from amino acids for potential biomedical applications. J. Fluoresc. 29, 1191–1200. doi:10.1007/s10895-019-02431-y
Sahu, S., Behera, B., Maiti, T. K., and Mohapatra, S. (2012). Simple one-step synthesis of highly luminescent carbon dots from orange juice: application as excellent bio-imaging agents. Chem. Commun. (Camb) 48, 8835–8837. doi:10.1039/c2cc33796g
Sangjan, A., Boonsith, S., Sansanaphongpricha, K., Thinbanmai, T., Ratchahat, S., Laosiripojana, N., et al. (2022). Facile preparation of aqueous-soluble fluorescent polyethylene glycol functionalized carbon dots from palm waste by one-pot hydrothermal carbonization for colon cancer nanotheranostics. Sci. Rep. 12, 10550. doi:10.1038/s41598-022-14704-x
Sasaki, D., Kusamori, K., Takayama, Y., Itakura, S., Todo, H., and Nishikawa, M. (2021). Development of nanoparticles derived from corn as mass producible bionanoparticles with anticancer activity. Sci. Rep. 11, 22818. doi:10.1038/s41598-021-02241-y
Sawpari, R., Samanta, S., Banerjee, J., Das, S., Dash, S. S., Ahmed, R., et al. (2023). Recent advances and futuristic potentials of nano-tailored doxorubicin for prostate cancer therapy. J. Drug Deliv. Sci. Technol. 81, 104212. doi:10.1016/j.jddst.2023.104212
Sekar, R., Basavegowda, N., Jena, S., Jayakodi, S., Elumalai, P., Chaitanyakumar, A., et al. (2022). Recent developments in heteroatom/metal-doped carbon dot-based image-guided photodynamic therapy for cancer. Pharmaceutics 14, 1869. doi:10.3390/pharmaceutics14091869
Shereema, R. M., Sankar, V., Raghu, K. G., Rao, T. P., and Shankar, S. S. (2015). One step green synthesis of carbon quantum dots and its application towards the bioelectroanalytical and biolabeling studies. Electrochim Acta 182, 588–595. doi:10.1016/j.electacta.2015.09.145
Shi, X., Hu, Y., Meng, H.-M., Yang, J., Qu, L., Zhang, X.-B., et al. (2020). Red emissive carbon dots with dual targetability for imaging polarity in living cells. Sens. Actuators B Chem. 306, 127582. doi:10.1016/j.snb.2019.127582
Singh, S., Shauloff, N., Sharma, C. P., Shimoni, R., Arnusch, C. J., and Jelinek, R. (2021). Carbon dot-polymer nanoporous membrane for recyclable sunlight-sterilized facemasks. J. Colloid Interface Sci. 592, 342–348. doi:10.1016/j.jcis.2021.02.049
Soltani-Zangbar, M. S., Parhizkar, F., Abdollahi, M., Shomali, N., Aghebati-Maleki, L., Shahmohammadi Farid, S., et al. (2022). Immune system-related soluble mediators and COVID-19: basic mechanisms and clinical perspectives. Cell Commun. Signal. 20, 131. doi:10.1186/s12964-022-00948-7
Song, Y., Zhu, S., and Yang, B. (2014). Bioimaging based on fluorescent carbon dots. RSC Adv. 4, 27184. doi:10.1039/c3ra47994c
Soumya, K., More, N., Choppadandi, M., Aishwarya, D. A., Singh, G., and Kapusetti, G. (2023). A comprehensive review on carbon quantum dots as an effective photosensitizer and drug delivery system for cancer treatment. Biomed. Technol. 4, 11–20. doi:10.1016/j.bmt.2023.01.005
Sousa, H. B. A., Martins, C. S. M., and Prior, J. A. V. (2021). You don’t learn that in school: an updated practical guide to carbon quantum dots. Nanomaterials 11, 611–688. doi:10.3390/nano11030611
Su, W., Wu, H., Xu, H., Zhang, Y., Li, Y., Li, X., et al. (2020). Carbon dots: a booming material for biomedical applications. Mater Chem. Front. 4, 821–836. doi:10.1039/c9qm00658c
Su, Y., Xie, M., Lu, X., Wei, H., Geng, H., Yang, Z., et al. (2014). Facile synthesis and photoelectric properties of carbon dots with upconversion fluorescence using arc-synthesized carbon by-products. RSC Adv. 4, 4839–4842. doi:10.1039/C3RA45453C
Sun, Y., Zheng, S., Liu, L., Kong, Y., Zhang, A., Xu, K., et al. (2020). The cost-effective preparation of green fluorescent carbon dots for bioimaging and enhanced intracellular drug delivery. Nanoscale Res. Lett. 15, 55. doi:10.1186/s11671-020-3288-0
Sun, Y.-P., Zhou, B., Lin, Y., Wang, W., Fernando, K. A. S., Pathak, P., et al. (2006). Quantum-sized carbon dots for bright and colorful photoluminescence. J. Am. Chem. Soc. 128, 7756–7757. doi:10.1021/ja062677d
Tacar, O., Sriamornsak, P., and Dass, C. R. (2013). Doxorubicin: an update on anticancer molecular action, toxicity and novel drug delivery systems. J. Pharm. Pharmacol. 65, 157–170. doi:10.1111/j.2042-7158.2012.01567.x
Tao, H., Yang, K., Ma, Z., Wan, J., Zhang, Y., Kang, Z., et al. (2012). In vivo NIR fluorescence imaging, biodistribution, and toxicology of photoluminescent carbon dots produced from carbon nanotubes and graphite. Small 8, 281–290. doi:10.1002/smll.201101706
NobelPrize.org. (2024). Nobel Prize Outreach AB. Available at: https://www.nobelprize.org/prizes/chemistry/2023/summary/.
Tian, X., Zeng, A., Liu, Z., Zheng, C., Wei, Y., Yang, P., et al. (2020). Carbon quantum dots: in vitro and in vivo studies on biocompatibility and biointeractions for optical imaging. Int. J. Nanomedicine 15, 6519–6529. doi:10.2147/IJN.S257645
Tiron, C. E., Luta, G., Butura, M., Zugun-Eloae, F., Stan, C. S., Coroaba, A., et al. (2020). NHF-derived carbon dots: prevalidation approach in breast cancer treatment. Sci. Rep. 10, 12662. doi:10.1038/s41598-020-69670-z
Tu, Y., Wang, S., Yuan, X., Wei, Y., Qin, K., Zhang, Q., et al. (2020). A novel fluorescent nitrogen, phosphorus-doped carbon dots derived from Ganoderma Lucidum for bioimaging and high selective two nitrophenols detection. Dyes Pigments 178, 108316. doi:10.1016/j.dyepig.2020.108316
Tummala, S., Lee, C. H., and Ho, Y. P. (2021). Boron, and nitrogen co-doped carbon dots as a multiplexing probe for sensing of p-nitrophenol, Fe (III), and temperature. Nanotechnology 32, 265502. doi:10.1088/1361-6528/abeeb6
Verma, A., Arshad, F., Ahmad, K., Goswami, U., Samanta, S. K., Sahoo, A. K., et al. (2020). Role of surface charge in enhancing antibacterial activity of fluorescent carbon dots. Nanotechnology 31, 095101. doi:10.1088/1361-6528/ab55b8
Wang, B., Cai, H., Waterhouse, G. I. N., Qu, X., Yang, B., and Lu, S. (2022). Carbon dots in bioimaging, biosensing and therapeutics: a comprehensive review. Small Sci. 2. doi:10.1002/smsc.202200012
Wang, L.-W., Peng, C.-W., Chen, C., and Li, Y. (2015). Quantum dots-based tissue and in vivo imaging in breast cancer researches: current status and future perspectives. Breast Cancer Res. Treat. 151, 7–17. doi:10.1007/s10549-015-3363-x
Wang, N., Zheng, A.-Q., Liu, X., Chen, J.-J., Yang, T., Chen, M.-L., et al. (2018). Deep eutectic solvent-assisted preparation of nitrogen/chloride-doped carbon dots for intracellular biological sensing and live cell imaging. ACS Appl. Mater Interfaces 10, 7901–7909. doi:10.1021/acsami.8b00947
Wang, Q., Liu, X., Zhang, L., and Lv, Y. (2012). Microwave-assisted synthesis of carbon nanodots through an eggshell membrane and their fluorescent application. Analyst 137, 5392–5397. doi:10.1039/c2an36059d
Wang, X., Gao, S., Xu, N., Xu, L., Chen, S., Mei, C., et al. (2021). Facile synthesis of phosphorus-nitrogen doped carbon quantum dots from cyanobacteria for bioimaging. Can. J. Chem. Eng. 99, 1926–1939. doi:10.1002/cjce.23927
Wang, Y., and Hu, A. (2014). Carbon quantum dots: synthesis, properties and applications. J. Mater Chem. C Mater 2, 6921. doi:10.1039/C4TC00988F
Wang, Y., Hu, A., Xu, X., Ray, R., Gu, Y., Ploehn, H. J., et al. (2014). Carbon quantum dots: synthesis, properties and applications. J. Mater Chem. C Mater 2, 6921. doi:10.1039/C4TC00988F
Wegner, K. D., and Hildebrandt, N. (2015). Quantum dots: bright and versatile in vitro and in vivo fluorescence imaging biosensors. Chem. Soc. Rev. 44, 4792–4834. doi:10.1039/C4CS00532E
Wei, Y., Chen, L., Wang, J., Liu, X., Yang, Y., and Yu, S. (2020). Rapid synthesis of B-N co-doped yellow emissive carbon quantum dots for cellular imaging. Opt. Mater (Amst) 100, 109647. doi:10.1016/j.optmat.2019.109647
Werbeck, N. D., Shukla, V. K., Kunze, M. B. A., Yalinca, H., Pritchard, R. B., Siemons, L., et al. (2020). A distal regulatory region of a class I human histone deacetylase. Nat. Commun. 11, 3841. doi:10.1038/s41467-020-17610-w
Wu, F., Su, H., Wang, K., Wong, W.-K., and Zhu, X. (2017). Facile synthesis of N-rich carbon quantum dots from porphyrins as efficient probes for bioimaging and biosensing in living cells. Int. J. Nanomedicine 12, 7375–7391. doi:10.2147/IJN.S147165
Wu, L.-N., Yang, Y.-J., Huang, L.-X., Zhong, Y., Chen, Y., Gao, Y.-R., et al. (2022). Levofloxacin-based carbon dots to enhance antibacterial activities and combat antibiotic resistance. Carbon N. Y. 186, 452–464. doi:10.1016/j.carbon.2021.10.020
Xia, J., Kawamura, Y., Suehiro, T., Chen, Y., and Sato, K. (2019). Carbon dots have antitumor action as monotherapy or combination therapy. Drug Discov. Ther. 13, 114–117. doi:10.5582/ddt.2019.01013
Xu, X., Ray, R., Gu, Y., Ploehn, H. J., Gearheart, L., Raker, K., et al. (2004). Electrophoretic analysis and purification of fluorescent single-walled carbon nanotube fragments. J. Am. Chem. Soc. 126, 12736–12737. doi:10.1021/ja040082h
Xue, B., Yang, Y., Sun, Y., Fan, J., Li, X., and Zhang, Z. (2019). Photoluminescent lignin hybridized carbon quantum dots composites for bioimaging applications. Int. J. Biol. Macromol. 122, 954–961. doi:10.1016/j.ijbiomac.2018.11.018
Xue, M., Zhan, Z., Zou, M., Zhang, L., and Zhao, S. (2016). Green synthesis of stable and biocompatible fluorescent carbon dots from peanut shells for multicolor living cell imaging. New J. Chem. 40, 1698–1703. doi:10.1039/C5NJ02181B
Xue, Y., Liu, C., Andrews, G., Wang, J., and Ge, Y. (2022). Recent advances in carbon quantum dots for virus detection, as well as inhibition and treatment of viral infection. Nano Converg. 9, 15. doi:10.1186/s40580-022-00307-9
Yadav, P. K., Chandra, S., Kumar, V., Kumar, D., and Hasan, S. H. (2023). Carbon quantum dots: synthesis, structure, properties, and catalytic applications for organic synthesis. Catalysts 13, 422. doi:10.3390/catal13020422
Yan, Z., Xiao, A., Lu, H., Liu, Z., and Chen, J. (2014). Determination of metronidazole by a flow-injection chemiluminescence method using ZnO-doped carbon quantum dots. New Carbon Mater. 29, 216–224. doi:10.1016/S1872-5805(14)60136-0
Yang, H.-L., Bai, L.-F., Geng, Z.-R., Chen, H., Xu, L.-T., Xie, Y.-C., et al. (2023). Carbon quantum dots: preparation, optical properties, and biomedical applications. Mater Today Adv. 18, 100376. doi:10.1016/j.mtadv.2023.100376
Yang, L., Wang, Z., Wang, J., Jiang, W., Jiang, X., Bai, Z., et al. (2016). Doxorubicin conjugated functionalizable carbon dots for nucleus targeted delivery and enhanced therapeutic efficacy. Nanoscale 8, 6801–6809. doi:10.1039/c6nr00247a
Yang, S. T., Cao, L., Luo, P. G., Lu, F., Wang, X., Wang, H., et al. (2009). Carbon dots for optical imaging in vivo. J. Am. Chem. Soc. 131, 11308–11309. doi:10.1021/ja904843x
Yang, Y., Wu, D., Han, S., Hu, P., and Liu, R. (2013). Bottom-up fabrication of photoluminescent carbon dots with uniform morphology via a soft–hard template approach. Chem. Commun. 49, 4920–4922. doi:10.1039/C3CC38815H
Yang, Z.-C., Li, X., and Wang, J. (2011). Intrinsically fluorescent nitrogen-containing carbon nanoparticles synthesized by a hydrothermal process. Carbon N. Y. 49, 5207–5212. doi:10.1016/j.carbon.2011.07.038
Yao, Y.-Y., Gangaraju, G., Girma, W., Yen, C.-L., Ling, Y., and Chang, J.-Y. (2017). Magnetofluorescent carbon dots derived from crab shell for targeted dual-modality bioimaging and drug delivery. ACS Appl. Mater Interfaces 9, 13887–13899. doi:10.1021/acsami.7b01599
Yin, J.-Y., Liu, H.-J., Jiang, S., Chen, Y., and Yao, Y. (2013). Hyperbranched polymer functionalized carbon dots with multistimuli-responsive property. ACS Macro Lett. 2, 1033–1037. doi:10.1021/mz400474v
Zhang, B., Liu, C., and Liu, Y. (2010). A novel one-step approach to synthesize fluorescent carbon nanoparticles. Eur. J. Inorg. Chem. 2010, 4411–4414. doi:10.1002/ejic.201000622
Zhang, F., Zhang, M., Zheng, X., Tao, S., Zhang, Z., Sun, M., et al. (2018). Berberine-based carbon dots for selective and safe cancer theranostics. RSC Adv. 8, 1168–1173. doi:10.1039/c7ra12069a
Zhang, H., Wang, G., Zhang, Z., Lei, J. H., Liu, T. M., Xing, G., et al. (2022). One step synthesis of efficient red emissive carbon dots and their bovine serum albumin composites with enhanced multi-photon fluorescence for in vivo bioimaging. Light Sci. Appl. 11, 113. doi:10.1038/s41377-022-00798-5
Zhang, R., and Chen, W. (2014). Nitrogen-doped carbon quantum dots: facile synthesis and application as a “turn-off” fluorescent probe for detection of Hg2+ ions. Biosens. Bioelectron. 55, 83–90. doi:10.1016/j.bios.2013.11.074
Zhao, A., Chen, Z., Zhao, C., Gao, N., Ren, J., and Qu, X. (2015). Recent advances in bioapplications of C-dots. Carbon N. Y. 85, 309–327. doi:10.1016/j.carbon.2014.12.045
Zhao, C., Wang, X., Wu, L., Wu, W., Zheng, Y., Lin, L., et al. (2019). Nitrogen-doped carbon quantum dots as an antimicrobial agent against Staphylococcus for the treatment of infected wounds. Colloids Surf. B Biointerfaces 179, 17–27. doi:10.1016/j.colsurfb.2019.03.042
Zhao, C., Wang, X., Yu, L., Wu, L., Hao, X., Liu, Q., et al. (2022). Quaternized carbon quantum dots with broad-spectrum antibacterial activity for the treatment of wounds infected with mixed bacteria. Acta Biomater. 138, 528–544. doi:10.1016/j.actbio.2021.11.010
Zhao, C., Wu, L., Wang, X., Weng, S., Ruan, Z., Liu, Q., et al. (2020). Quaternary ammonium carbon quantum dots as an antimicrobial agent against gram-positive bacteria for the treatment of MRSA-infected pneumonia in mice. Carbon N. Y. 163, 70–84. doi:10.1016/j.carbon.2020.03.009
Zhao, M.-X., and Zhu, B.-J. (2016). The research and applications of quantum dots as nano-carriers for targeted drug delivery and cancer therapy. Nanoscale Res. Lett. 11, 207. doi:10.1186/s11671-016-1394-9
Zheng, D. W., Li, B., Li, C. X., Fan, J. X., Lei, Q., Li, C., et al. (2016). Carbon-dot-decorated carbon nitride nanoparticles for enhanced photodynamic therapy against hypoxic tumor via water splitting. ACS Nano 10, 8715–8722. doi:10.1021/acsnano.6b04156
Zhou, J., Deng, W., Wang, Y., Cao, X., Chen, J., Wang, Q., et al. (2016). Cationic carbon quantum dots derived from alginate for gene delivery: one-step synthesis and cellular uptake. Acta Biomater. 42, 209–219. doi:10.1016/j.actbio.2016.06.021
Zhou, J., Sheng, Z., Han, H., Zou, M., and Li, C. (2012). Facile synthesis of fluorescent carbon dots using watermelon peel as a carbon source. Mater Lett. 66, 222–224. doi:10.1016/j.matlet.2011.08.081
Zhou, X., and Ye, Q. (2021). Cellular immune response to COVID-19 and potential immune modulators. Front. Immunol. 12, 646333. doi:10.3389/fimmu.2021.646333
Zhu, H., Wang, X., Li, Y., Wang, Z., and Yang, X. (2009). Microwave synthesis of fluorescent carbon nanoparticles with electrochemiluminescence properties. Chem. Commun. (Camb) 4, 5118–5120. doi:10.1039/b907612c
Zhu, P., Wang, S., Zhang, Y., Li, Y., Liu, Y., Li, W., et al. (2022). Carbon dots in biomedicine: a review. ACS Appl. Bio Mater 5, 2031–2045. doi:10.1021/acsabm.1c01215
Zhu, S., Meng, Q., Wang, L., Zhang, J., Song, Y., Jin, H., et al. (2013). Highly photoluminescent carbon dots for multicolor patterning, sensors, and bioimaging. Angew. Chem. Int. Ed. 52, 3953–3957. doi:10.1002/anie.201300519
Zhu, S., Song, Y., Zhao, X., Shao, J., Zhang, J., and Yang, B. (2015). The photoluminescence mechanism in carbon dots (graphene quantum dots, carbon nanodots, and polymer dots): current state and future perspective. Nano Res. 8, 355–381. doi:10.1007/s12274-014-0644-3
Zou, C., Foda, M. F., Tan, X., Shao, K., Wu, L., Lu, Z., et al. (2016). Carbon-dot and quantum-dot-coated dual-emission core–satellite silica nanoparticles for ratiometric intracellular Cu2+ imaging. Anal. Chem. 88, 7395–7403. doi:10.1021/acs.analchem.6b01941
Keywords: carbon quantum dots, biocompatible quantum dots, bioimaging, biomedicine, metal-doped CQD, CQD-polymer composite (CPD), graphene-based carbon dots (GQD), carbon dots
Citation: Das S, Mondal S and Ghosh D (2024) Carbon quantum dots in bioimaging and biomedicines. Front. Bioeng. Biotechnol. 11:1333752. doi: 10.3389/fbioe.2023.1333752
Received: 05 November 2023; Accepted: 29 December 2023;
Published: 22 January 2024.
Edited by:
Sameer Hussain, Xi’an Jiaotong University, ChinaReviewed by:
Kafeel Ahmad, Sungkyunkwan University, Republic of KoreaAnsar Abbas, Xi’an Jiaotong University, China
Siavash Iravani, Independent Researcher, Isfahan, Iran
Copyright © 2024 Das, Mondal and Ghosh. This is an open-access article distributed under the terms of the Creative Commons Attribution License (CC BY). The use, distribution or reproduction in other forums is permitted, provided the original author(s) and the copyright owner(s) are credited and that the original publication in this journal is cited, in accordance with accepted academic practice. No use, distribution or reproduction is permitted which does not comply with these terms.
*Correspondence: Surya Das, dassurya394@gmail.com; Somnath Mondal, somomsom@gmail.com; Dhiman Ghosh, dhiman.ghosh@phys.chem.ethz.ch
†These authors have contributed equally to this work