- 1Department of Gastroenterology, Endoscopic Center, Engineering Research Center of Ministry of Education for Minimally Invasive Gastrointestinal Endoscopic Techniques, Shengjing Hospital of China Medical University, Shenyang, China
- 2Research Center for Biomedical Materials, Engineering Research Center of Ministry of Education for Minimally Invasive Gastrointestinal Endoscopic Techniques, Shengjing Hospital of China Medical University, Shenyang, China
- 3Liaoning Research Institute for Eugenic Birth and Fertility, China Medical University, Shenyang, China
Esophageal stricture (ES) results from benign and malignant conditions, such as uncontrolled gastroesophageal reflux disease (GERD) and esophageal neoplasms. Upper gastrointestinal endoscopy is the preferred diagnostic approach for ES and its underlying causes. Stent insertion using an endoscope is a prevalent method for alleviating or treating ES. Nevertheless, the widely used self-expandable metal stents (SEMS) and self-expandable plastic stents (SEPS) can result in complications such as migration and restenosis. Furthermore, they necessitate secondary extraction in cases of benign esophageal stricture (BES), rendering them unsatisfactory for clinical requirements. Over the past 3 decades, significant attention has been devoted to biodegradable materials, including synthetic polyester polymers and magnesium-based alloys, owing to their exceptional biocompatibility and biodegradability while addressing the challenges associated with recurring procedures after BES resolves. Novel esophageal stents have been developed and are undergoing experimental and clinical trials. Drug-eluting stents (DES) with drug-loading and drug-releasing capabilities are currently a research focal point, offering more efficient and precise ES treatments. Functional innovations have been investigated to optimize stent performance, including unidirectional drug-release and anti-migration features. Emerging manufacturing technologies such as three-dimensional (3D) printing and new biodegradable materials such as hydrogels have also contributed to the innovation of esophageal stents. The ultimate objective of the research and development of these materials is their clinical application in the treatment of ES and other benign conditions and the palliative treatment of malignant esophageal stricture (MES). This review aimed to offer a comprehensive overview of current biodegradable esophageal stent materials and their applications, highlight current research limitations and innovations, and offer insights into future development priorities and directions.
1 Introduction
Esophageal stricture (ES) results from prevalent clinical conditions and is categorized into two primary types: benign esophageal stricture (BES) and malignant esophageal stricture (MES). The most frequently encountered BES subtype is peptic strictures (Desai et al., 2020), typically stemming from uncontrolled gastroesophageal reflux disease (GERD), radiation-induced strictures, and iatrogenic injuries (De Wijkerslooth et al., 2011; Liu et al., 2022). In contrast, MES is predominantly associated with esophageal neoplasms and extraneous malignancies that compress the esophagus (Siersema, 2008). Upper gastrointestinal endoscopy serves as the preferred diagnostic modality for identifying ES and its root causes (Siersema, 2008). Patients commonly present with dysphagia once the stenosis reduces the esophageal lumen by 50% (Adler and Siddiqui, 2017). ES can trigger various adverse consequences such as malnutrition, weight loss, acid-base imbalance, and hypoalbuminemia. Consequently, timely intervention is imperative, particularly when these adverse conditions manifest.
Current treatments for ES encompass various modalities, including drug therapy, surgical interventions, endoscopic esophageal dilation, and endoscopic stent placement, among others (Sehgal and Sami, 2021). Pharmacological treatment entails the administration of proton pump inhibitors (PPI) for managing GERD (Peng et al., 2023). Additionally, endoscopy-guided infusion of adrenocorticotropic and cytotoxic drugs into the stenosis site serves to inhibit fibrosis and scar formation in damaged tissue, thereby alleviating scarring-induced permanent ES (Zein et al., 1995). However, these drugs may prove ineffective, lead to disease recurrence upon discontinuation, and even give rise to significant side effects, limiting their standalone usage in current clinical practice. Esophagectomy becomes necessary in severe ES unresponsive to conservative measures such as endoscopic treatment.
In contrast to surgery, endoscopy boasts advantages such as reduced trauma, a high cure rate, and rapid recovery (Chen et al., 2022). Esophageal dilation is one of the most practical and effective techniques for managing BES, with a success rate exceeding 80%–90% (Siersema, 2019). Balloon dilation is the preferred method for achieving this outcome. Moreover, endoscopic dilation of the esophagus, whether by employing a Savary (bougie) or a balloon dilator, serves as the initial step in managing refractory benign esophageal stricture (RBES) (Sehgal and Sami, 2021). Balloon dilation in ES yields immediate relief; however, it cannot ensure sustained dilation. Patients with RBES often require repeated dilations, which can be inconvenient and necessitate high compliance rates (Siersema, 2019). Additionally, balloon dilation can serve as a complementary procedure to esophageal stent insertion by performing several balloon dilations before stent placement, which prepares the stricture for the smooth passage of the stent delivery device. Esophageal stent insertion using endoscopy involves the deployment of a stent to support the stricture and maintain radial pressure, facilitating the remodeling of the stenosis and its surrounding tissue.
Indications for esophageal stent placement include BES, primarily resulting from GERD-induced chronic inflammation (Desai et al., 2020), scarred strictures following chemical burns, and stenosis after post-surgical anastomosis or endoscopic resection procedures such as endoscopic submucosal dissection (ESD) and endoscopic mucosal resection (EMR) (Siersema, 2019). Stent placement is also indicated for MES, including unresectable esophageal or gastric cardiac cancer, local recurrence at the anastomosis site following esophagectomy, and strictures arising after radiation therapy for esophageal cancer. Additionally, stents may be employed to address other conditions such as esophagotracheal fistulas and esophageal ruptures, among others. Among these indications, MES is frequently linked to esophageal cancer, which encompasses two distinct pathological and epidemiological subtypes: esophageal squamous cell carcinoma (ESCC) and esophageal adenocarcinoma (EAC) (Smyth et al., 2017). Esophageal cancer is a global health concern, ranking as the sixth most prevalent cause of cancer-related fatalities each year. Over 50% of patients with esophageal cancer experience dysphagia due to malignant obstruction. Treatment for esophageal cancer hinges on patient-specific factors and tumor characteristics, particularly the TNM stage, which is usually identified using imaging methods and histopathologic biopsies (Liu et al., 2023; Matsunami et al., 2023). Early-stage tumors are typically amenable to endoscopic resection, whereas locally advanced cancers necessitate surgical resection, neoadjuvant chemoradiotherapy, chemotherapy, radiotherapy, or a combination thereof (Chen et al., 2023). However, a considerable proportion of patients present with advanced, unresectable tumors at the time of diagnosis. Treatment objectives in such cases primarily constitute restoring oral food intake and enhancing quality of life (Rozanes et al., 2002). Notably, over half of the patients pursuing curative treatment will experience tumor recurrence, necessitating palliative care for the majority of individuals in the long term (Smyth et al., 2017). Consequently, improved treatment options for esophageal cancer should be explored.
Esophageal stenting has a rich history that dates back over a century. Its origins can be traced back to Symonds’ pioneering proposal in 1885 regarding the advantages of esophageal stent placement. In 1977, Arkinson introduced a cylindrical plastic tube to address inoperable esophageal tumors, marking the inception of the clinical use of esophageal stents. Frimberger first treated ES with a spiral metal stent in 1983, and Domschke applied the Wallstent to treat cancer-related ES in 1990. A pivotal moment occurred in 1996 when Goldin et al. (1996) introduced an esophageal stent constructed from poly-L-lactide (PLLA), which was both biodegradable and self-expandable (Sehgal and Sami, 2021). Subsequently, a diverse array of esophageal stents employing various materials and functions emerged thereafter. Currently, several types of esophageal stents exist (Wang et al, 2021b): self-expandable metal stents (SEMS), self-expandable plastic stents (SEPS), biodegradable stents (BDS), and hybrids that combine elements of the aforementioned stents, such as SEMS with an anti-reflux valve, DES, and radioactive SEMS, among others. Among these options, SEMS has emerged as the preferred and efficacious choice for managing BES and MES (Yang et al., 2016). The most commonly employed endoscopic therapeutic approach for palliation involves the placement of esophageal stents (Rabenstein, 2015), particularly in cases involving patients with cancer and limited life expectancy who require rapid relief from dysphagia or those unresponsive to chemoradiotherapy. However, SEMS has certain limitations. The necessity of stent removal after stenosis resolution in certain benign conditions and the potential complications of metal materials such as bleeding, retrosternal pain, esophageal fistula, tissue ingrowth, and restenosis (Song et al., 1997; Ackroyd et al., 2001; Evrard et al., 2004; Yang et al., 2016), has rendered SEMS less than ideal for ES treatment, particularly BES treatment. SEPS offers advantages, such as reduced tissue trauma, minimal tissue proliferation, and ease of removal (Verschuur et al., 2008; Rabenstein, 2015); however, its long-term effectiveness is disappointing. SEPS often requires reintervention and carries a higher complication rate than SEMS, including complications such as stent migration (Conio et al., 2007; Ham and Kim, 2014; Fuccio et al., 2015). Moreover, its infrequent application for the management of malignant dysphagia can be linked to factors such as the wide diameter of the stent deployment catheter, the complex and unwieldy assembly and operation, and its heightened mobility (Adler and Siddiqui, 2017).
Over the past 3 decades, biodegradable materials, including synthetic polyester polymers and magnesium (Mg)-based alloys (Wu et al., 2012; Yuan et al., 2016; Liu et al., 2022), have garnered substantial attention owing to their distinct advantages when compared to SEMS and SEPS. An ideal biodegradable esophageal stent should possess exceptional mechanical properties, compliance, and histocompatibility, ultimately undergoing complete degradation after providing a period of mechanical support. The resulting degradation products should have no adverse effects on human health (Yang et al., 2016). Considering that a stent’s degradability is pivotal in mitigating the risk of severe long-term complications, stents crafted from biodegradable materials are highly favorable for managing benign conditions (Tamai et al., 2000; Saito et al., 2004; Tanaka et al., 2007). The critical factor in developing an outstanding esophageal stent lies in the selection of appropriate biodegradable materials. Consequently, our discussion centered on the materials and applications of biodegradable esophageal stents, highlighted the current research challenges, and offered insights into future development priorities and directions.
2 Materials used in biodegradable esophageal stents
A diverse array of effective stents constructed from biodegradable materials, encompassing synthetic polyester polymers and Mg-based alloys, has found widespread application in the cardiovascular, airway, esophageal, and urinary systems in contemporary medical practice (Li et al., 2023). In 2006, the US Food and Drug Administration (FDA) approved the use of several synthetic polymers in medical devices (Kohn et al., 2007), including poly-L-lactide (PLLA), poly (lactic-co-glycolic acid) (PLGA), polydioxanone (PDO), poly (ε-caprolactone) (PCL) and poly (1, 3-trimethylene carbonate) (PTMC). Presently, these materials, their copolymers, and their composites constitute the forefront of ongoing research efforts in this field. Notably, the ultimate products of their degradation within the human body are water and carbon dioxide, posing no harm to human health (Li et al., 2023).
Two notable options among the biodegradable polymer stents currently accessible in clinical practice are the PLLA-BD stent (Marui Textile Machinery Co., Ltd., Osaka, Japan), comprising braided PLLA monofilaments (Tanaka et al., 2007), and the SX ELLa-BD stent (Ella-CS, Hradec Kralove, Czech Republic), composed of PDO, which is a type of surgical suture material (Stivaros et al., 2010) (Table 1).
Metals containing elements naturally present in the human body are deemed biocompatible, rendering them suitable for the fabrication of biodegradable stents. Notably, Mg, iron (Fe), zinc (Zn) and their respective alloys are among this category. These metals and alloys offer sustained radial support over a defined period of time, while undergoing spontaneous degradation within the body (Bowen et al., 2016; Liu et al., 2022). Therefore, they can help minimize inflammation from local tissue hyperplasia or prolonged physical stimulation (Ragunath, 2008), precluding the need for secondary stent removal (Chen et al, 2019a). Among these biodegradable alloys, Mg holds particular significance as an essential trace element and a structural component of tissues (Moravej and Mantovani, 2011). Furthermore, Mg is recognized as a non-carcinogenic element (Xu et al., 2007). Additionally, Mg-based alloys exhibit superior strength, specific stiffness, corrosion resistance, and processability (Bowen et al., 2016; Liu et al., 2022). The following section presents a comprehensive review of synthetic polyester polymers, Mg-based alloys and their related substances, focusing on materials, applications and innovative developments.
2.1 Biodegradable polymers
2.1.1 PLLA
Polylactic acid (PLA) is an aliphatic polyester that is biodegradable, bio-based, and can be entirely sourced from renewable materials such as corn, potatoes, sugar cane, and similar resources (Yusoff et al., 2021). PLA demonstrates commendable biocompatibility, showcasing notable attributes such as strength, toughness, and plasticity. PLA hydrolysis yields products such as lactic acid, which is absorbed and metabolized by the human body, ultimately generating carbon dioxide and water that can be efficiently expelled from the body without leaving any residues. Lactic acid is a chiral molecule that exists in L and D isomers; therefore, four types of PLA arise, including isotactic PLA (PLLA), isotactic poly-D-lactic acid (PDLA), and atactic and syndiotactic poly-D, L-lactic acid (PDLLA) (Lasprilla et al., 2012; Zhou et al., 2021). Among them, PLLA, which is one of the stereo configurations of PLA (Wu et al., 2023), is a biodegradable material produced through the polymerization of L-lactic acid. Its versatility has led to its application in surgical sutures, bone screws, and tissue engineering scaffolds (Saito et al., 2007; Tanaka et al., 2007; Shuai et al., 2019; Liu et al., 2020; Shuai et al., 2021; Yin et al., 2022), and an array of stents designed for use in coronary arteries, urinary systems, bronchial passages, and biliary tracts (Tamai et al., 2000; Saito et al., 2004; Isotalo et al., 2006; Song et al., 2022).
In 1996, Goldin et al. (1996) documented the groundbreaking experience with a novel stent that possessed the unique features of self-expansion and biodegradability. The stent was constructed as a coil spring using a solitary strand of PLLA (InStent Inc) and was designed to treat BES. Furthermore, Fry and Fleischer (Fry and Fleischer, 1997) pioneered the application of PLLA-BDS (EsophaCoil; InStent, Eden Prairie, MN, United States) in the United States, particularly for BES caused by radiation-induced injury. This marked a significant expansion of the knowledge and experience in the application of biodegradable esophageal stents.
A limited amount of research has been conducted on the clinical application of PLLA stents (Table 2). In 2006, Tanaka et al. (2007) introduced a new biodegradable Ultraflex-type stent, utilizing machine-knitted polylactic acid monofilaments (Marui Textile Machinery Co., Ltd., Osaka, Japan), to treat two patients with benign strictures in the upper gastrointestinal tract. These stents were layered with a water-soluble contrast agent (gastrografin) to enhance visibility during placement and were subsequently guided into position using a combination of fluoroscopy and endoscopy. A thread was used to fasten the stent’s tip and diminish its diameter, resulting in easier navigation through narrower sections. In case 1, a 19-year-old woman had engaged in a suicide attempt by consuming caustic potash, resulting in the development of ES 2 weeks later. In case 2, a 75-year-old man developed stenosis at the anastomotic site after undergoing surgical resection for esophageal cancer. Balloon dilatation therapy was attempted multiple times; however, neither patient experienced relief from their dysphagia. Consequently, both individuals received the same treatment approach. After two sessions of balloon dilatation, the stents were meticulously placed under combined fluoroscopic and endoscopic guidance, resulting in immediate improvement of their strictures. Remarkably, the stents naturally migrated and were excreted with feces via bowel movements at 10 and 14 days post implantation for cases 1 and 2, respectively, with no associated complications. In case 1, there was no recurrence at the 6-month follow-up examination. However, mild restenosis occurred 1 week later in case 2 but did not necessitate further dilatation therapy during the subsequent 6 months of follow-up.
Saito et al. (2007) used PLLA-BDS (Marui Textile Machinery Co., Ltd., Osaka, Japan) to treat BES in 2007. These stents were composed of PLLA monofilaments (molecular mass 183 kD, diameter 0.23 mm), with a machine-knitted design akin to ultra-flex metallic stents. These stents were applied in various cases, including BES resulting from anastomosis following surgical resection for esophageal cancer, corrosive liquid ingestion in suicide attempts, and the preventive insertion of biodegradable stents for post-ESD strictures. Each PLLA-BDS was custom-tailored to the specific characteristics of the patient’s esophageal condition and meticulously fitted using an endoscope. Remarkably, spontaneous early migration was observed in 77% (10/13) of these stents, possibly attributed to stent degradation, leading to loss of patency. No signs of restenosis were observed during the follow-up period, which ranged from 7 months to 2 years. This study demonstrated the utility of biodegradable stents in managing BES, with a specific focus on their role in preventing post-ESD stenosis. Presently, a rising number of patients diagnosed with early-stage esophageal cancer are opting for full resection through minimally invasive endoscopic techniques such as ESD. However, strictures tend to develop when the mucosal defect encompasses >75% of the esophageal circumference (Oyama et al., 2005), and endoscopic dilation or stenting may be required in these cases. Saito et al. (2008) also reported two cases of using PLLA stents to successfully improve BES following ESD in patients with early ESCC and repeated failed balloon dilatation.
Additionally, researchers reported a case of a patient with recurrent ESCC following chemoradiotherapy (CRT) (Mochizuki et al., 2012). Biodegradable esophageal stents composed of PLLA monofilaments (Saito et al., 2008) were promptly inserted on the same day of ESD to prevent stenosis resulting from the mucosal defect. This study demonstrated the potential efficacy of combining ESD with biodegradable stents, introducing a viable approach for managing recurrent ESCC following CRT and offering a promising avenue for enhancing the treatment outcomes and prognosis of esophageal malignancies.
Tanaka et al. (2007) substantiated the superior radial force of their biodegradable stent, which was constructed from polylactic acid monofilaments, with higher radial force measurements than commercially available metal stents. Moreover, this stent exhibited a marked reduction in various complications typically associated with the use of metal stents in BES treatment. Nonetheless, these studies also highlighted a potential drawback of PLLA stents, namely, the occurrence of early migration. PLLA-BDS take months to degrade; therefore, the migration risk arises from the gradual loss of radial force before complete degradation. No complications were observed with the softened stents during defecation; nevertheless, the ideal approach is to prevent these early displacements to ensure the stents effectively treat strictures. The research group speculated on the necessity of securing the stents in place for a minimum of 2 weeks, which represents the required time for healing inflammatory changes in the lesions. No bowel obstruction caused by PLLA stents was reported in the aforementioned cases; however, vigilant attention should be directed towards the potential risk of bowel obstruction from migrated stents.
In summary, PLLA stents exhibit noteworthy attributes, including excellent expansion capacity, high radial force, and the ability to biodegrade within 3–6 months when exposed to an acidic environment (Yang et al., 2016). These qualities contribute to a low incidence of stent-related complications. However, their inherent brittleness limits their flexibility (Han et al., 2018). Furthermore, PLLA stents tend to experience a relatively high rate of early spontaneous migration as they gradually degrade, necessitating vigilant monitoring for potential complications such as intestinal obstruction.
2.1.2 PLGA
PLGA is a biodegradable and biocompatible polyester copolymer constructed by the polymerization of lactic acid and glycolide (Stevanović et al., 2009; Ma et al., 2011) and has emerged as a principal biodegradable polymer over the past few decades. It serves as the primary polymer for drug delivery (Shakya et al., 2023) and plays a pivotal role in diverse clinical applications (Liu et al., 2016). The hydrolytic breakdown of PLGA yields lactic acid and glycolic acid (Ma et al., 2011; Song et al., 2022), which are ultimately converted into carbon dioxide and water and subsequently eliminated through the body’s normal metabolic processes. PLGA is renowned for its low toxicity and rapid degradation and is widely used in the treatment of bone defects, tissue engineering, surgical sutures, and drug release systems (Stevanović et al., 2009; Fredenberg et al., 2011; Lanao et al., 2011; Babilotte et al., 2021; Jin et al., 2021).
Liu et al. (2016) designed a novel biodegradable stent comprising three curved segments of covered metal mesh linked together by biodegradable PLGA threads. As these PLGA threads degraded, the stent disintegrated and safely passed into the stomach. This study investigated the novel stent’s attributes using in vitro assessments, including evaluations of radial forces, pH levels, and morphology, among others. Additionally, they conducted in vivo animal studies, involving the insertion of these stents into the narrow middle esophagi of rabbits, which had incurred damage from alkali burns. The braided design of these stents enabled a reduction of the radial force, reaching 0 N (Hirdes et al., 2013), without any associated stent-related complications. This innovative design, namely, combining the strengths of metallic stents in terms of radial force with the biodegradability of biodegradable stents, holds considerable promise and offers a novel avenue for the future development of biodegradable stent technology.
Prior studies have demonstrated the promise of utilizing PLGA as a surface coating, particularly on Mg or Zn alloys, owing to its commendable attributes including robust biocompatibility, pronounced hydrophobicity, and proficient drug-loading capabilities (Wang et al., 2006; Zhu and Braatz, 2015; Dou et al., 2023). The versatile applications of PLGA coatings have been extensively explored by researchers in various stent domains, including cardiovascular stents (Fang et al., 2021; Dou et al., 2023), ureteral stents (Antonowicz et al., 2021), and gastrointestinal stents (Kwak et al., 2017), among others.
Liu et al. (2022) designed and tested a groundbreaking Mg-based braided stent coated with PLGA infused with paclitaxel (PTX) to avoid secondary removal by endoscopic procedures and inhibit restenosis of the esophagus (Figure 1). This ingeniously engineered stent was designed to undergo self-degradation within the body after ensuring esophageal patency for a specified duration, precluding the necessity for subsequent removal procedures. In addition, the stent’s surface was coated with anti-proliferative drugs, which can further inhibit local tissue growth and extend the unobstructed period of esophageal function. The safety and effectiveness of this combined stent have been validated through in vitro and in vivo testing, whereas its sustained applicability and worthiness await further evaluation in subsequent studies. In conclusion, this stent effectively amalgamated the merits of biodegradable alloys and polymers, thereby compensating for their limitations. This innovation presents a promising and novel avenue for future research and development in biodegradable stent technology.
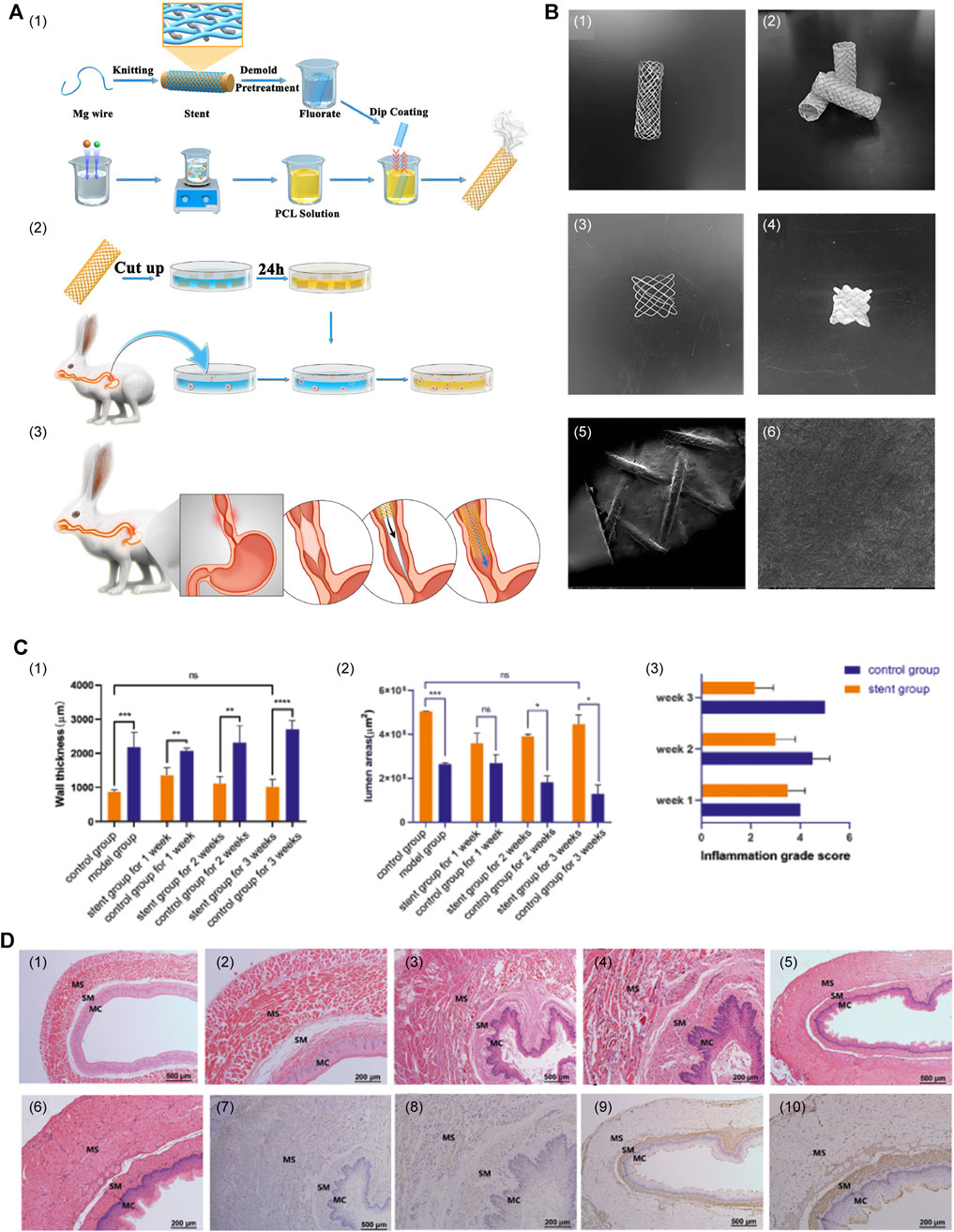
FIGURE 1. (A) Primary procedure and methods. (1) Construction of PLGA-coated drug-eluting Mg stents. (2) Experiments on in vitro degradation, drug release, and cytotoxicity. (3) Insertion of the stent. (B) Macrostructure and surface characteristics of the stent. (1) The Mg alloy bare stent. (2) The PTX-PLGA-coated Mg-based stent. (3) The 1 cm × 1 cm stent sheet of bare stent. (4) The 1 cm × 1 cm stent sheet of the coated stent. (5 and 6) SEM images of the surface characteristics. (C) (1–3) Wall thickness of the esophagus, lumen area, and degree of inflammation between the stent group and sham stent group. (D) (1 and 2) Esophageal tissue of normal control rabbits. (3 and 4) Imaging of esophageal tissue over a period of 3 weeks after successful modeling, with no stent insertion. (5 and 6) Imaging of esophageal tissue 3 weeks after stent insertion following the successful modeling. (7 and 8) Immunohistochemical staining images of stent group and (9 and 10) the sham stent group. Reproduced with permission from ref [(Liu et al., 2022)]. Copyright 2022 Acta Materialia Inc.
2.1.3 PDO
PDO is a biodegradable polymer with a semicrystalline structure, belonging to the polyester family. Its degradation occurs through the random hydrolysis of ester bonds within its molecular structure, with glyoxylic acid, a degradation product, primarily serving as a precursor for oxalic acid and playing a role as an intermediate in the transformation of glycolic acid into glycine. All of PDO’s degradation products and intermediates are non-toxic (Repici et al., 2010). A significant milestone was achieved when PDO gained FDA approval in 1981 for its use in biodegradable sutures (Bartholomew, 1981). PDO can be knitted into tubes of the desired shapes and dimensions, and stents composed of PDO have been inserted into the bronchus, trachea, intestine, and bile duct, in addition to the esophagus (Lischke et al., 2011; Rejchrt et al., 2011; Siiki et al., 2017). PDO’s utility extends even further into domains such as tissue engineering (Boland et al., 2005; Okata et al., 2014; Goonoo et al., 2015), bone regeneration (Saska et al., 2021), and drug delivery systems (Goonoo et al., 2015; Bottino et al., 2019), among others. The SX ELLa-BD stent (Ella-CS, Hradec Kralove, Czech Republic) is a biodegradable esophageal stent constructed using commercially accessible PDO absorbable surgical sutures (Repici et al., 2010). This stent is Conformité Européenne approved and is indicated for use in benign strictures, including digestive, erosive, anastomotic, and post-radiation strictures (van Hooft et al., 2011). The ELLa-BDS comes in various sizes, with stent body diameters ranging from 18 to 25 mm and lengths from 60 to 135 mm when fully deployed. It should be compressed and installed onto a 9.4 mm (28F) delivery system prior to clinical use. Regarding performance, this stent exhibits a relatively high axial force and initially low radial force, which gradually decreases to 0 N (Hirdes et al., 2013). Its structural integrity and radial expansion strength remain stable for a period of 6–8 weeks, after which disintegration occurs within 11–12 weeks post implantation. Notably, low pH can accelerate its degradation; therefore, the use of a PPI is advised to extend the integrity of the stent (Yang et al., 2016).
Several preclinical studies on ELLa-BDS have been conducted. For example, Battersby and Doyle (2010) used a biodegradable PDO self-expanding stent to treat BES in a cat in 2010. A fluoroscopic examination revealed the complete disappearance of the stent 4 months after placement, with no signs of obstruction. In 2012, Pauli et al. (2012) reported ELLa-BDS’s potential to alleviate the development of severe strictures after performing circumferential endoscopic esophageal mucosectomy (EEM) in a porcine model. The findings indicated that the BDS group exhibited significantly extended survival compared to the control group (9.2 weeks vs. 2.4 weeks). However, the stent was unable to entirely prevent the development of high-grade strictures, and the timing of stenosis formation appeared to be linked to the gradual loss of radial force and stent disintegration. A retrospective review (Lam et al., 2013) of records for six dogs with RBES revealed initial improvements in dysphagia following ELLa-BDS insertion (Figure 2). Nonetheless, close monitoring is essential owing to the potential complications, including regurgitation, stent migration, and restenosis.
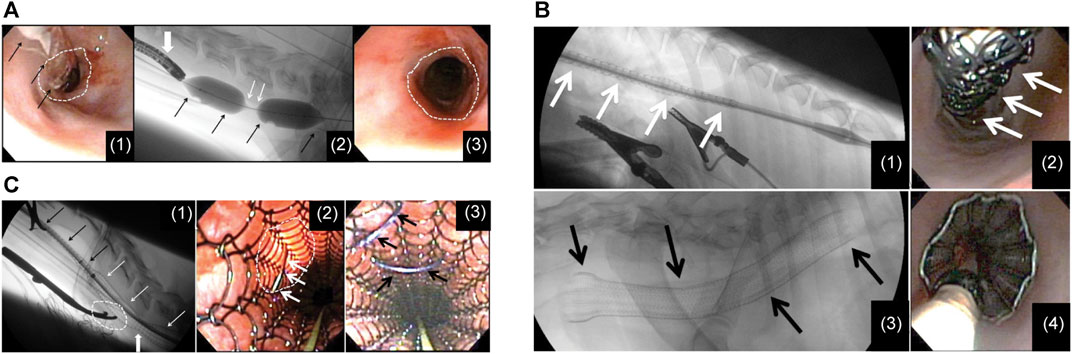
FIGURE 2. (A) (I) A flexible endoscope and dilatation balloon were inserted into the esophagus to visualize the stricture. Balloon dilatation was performed to allow the endoscope to pass through. (II) The stent in a restricted state was advanced with the guidance of fluoroscopy. (III) Following the deployment of the stent, the upper thoracic stricture was resolved at the site of stent constriction, revealing a clear and unobstructed esophageal passage. (B) (I) A restricted stent was advanced through an esophageal constriction within the thoracic area. (II) Endoscopic image of the stent while it was constrained before deployment. (III) Lateral fluoroscopic image following the stent insertion. (IV) The endoscopic picture taken right after stent insertion, illustrating effective esophageal wall apposition. (C) (I) Lateral fluoroscopic image showing an esophageal stent in a state of partial deployment and partial constriction. (II) Endoscopic view showing a luminated area where the surgical approach had been performed and the suture needle passing through the esophageal wall and engaging the stent. (III) Endoscopic view after 2 polypropylene sutures had been placed to tack the stent to the esophageal wall. Reproduced with permission from ref [(Lam et al., 2013)]. Copyright 2013 American College of Veterinary Internal Medicine.
ELLa-BDS is recognized as the first commercially available biodegradable esophageal stent (Vandenplas et al., 2009; Repici et al., 2010; Stivaros et al., 2010) and has been extensively employed in clinical studies to evaluate its effectiveness and safety in the treatment of BES (Table 3). Previous studies have shown that ELLa-BDS offers a practical approach to managing RBES by reducing the need for repetitive endoscopic dilations and cutting treatment costs (Dhar et al., 2009; Repici et al., 2010; van Boeckel et al., 2011; Muñoz et al., 2013). A single BDS placement provides only temporary relief; therefore, the sequential placement of stents emerges as an excellent option to preclude the need for serial dilations (Hirdes et al, 2012a). Moreover, the commendable efficacy and safety of ELLa-BDS in addressing corrosive esophageal strictures and dysphagia from benign anastomotic esophageal strictures have also been demonstrated (Vandenplas et al., 2009; van Hooft et al., 2011). In a noteworthy case (Basha et al., 2013), researchers employed ELLa-BDS to treat a patient with corrosive (sulfuric acid-induced) esophageal and pyloric strictures. The patient underwent transhiatal esophagectomy with colonic transposition 6 months after stent insertion. The examination of the resected esophageal specimen marked the first-ever report of histological evidence in a human case, confirming ELLa-BDS’s excellent biocompatibility, minimal tissue response, and complete degradation capabilities. A cohort study conducted in 2016 (McCain et al., 2016) revealed that ELLa-BDS offered a probability of long-term symptom relief that exceeds 50% for patients with benign conditions. Regarding patients that required re-intervention, the duration of symptom-free periods and the time until re-intervention significantly surpassed expectations in comparison to SEMS, SEPS, or dilation procedures. In 2018, Walter et al. (2018) conducted a comparative study between early dilation and ELLa-BDS insertion in the treatment of recurrent BES and concluded that ELLa-BDS insertion reduced the need for repeated dilations and prolonged the time before recurrent dysphagia, in contrast to dilation. In 2022, a non-randomized, single-arm prospective trial (Yano et al., 2022) conducted across eight institutions provided compelling evidence that ELLa-BDS was indeed effective in treating RBES and demonstrated a satisfactory safety profile. Furthermore, a recent systematic review and proportion meta-analysis conducted by Kailla et al. (2023) in 2023 demonstrated that ELLa-BDS’s high technical sophistication and moderate clinical efficacy support its use in the management of adult BES. However, further evidence is required to substantiate its advantage over alternative approaches such as endoscopic dilation.
ELLa-BDS has been extensively investigated in the management of MES, in addition to BES. In 2010, Stivaros et al. (2010) implanted two ELLa-BDSs in an octogenarian patient with cancer at the gastro-esophageal junction (GOJ). The study’s findings suggested that patients with dysphagia and potentially curable esophageal carcinoma could consider BDS as an alternative to gastrostomy, particularly in the context of neo-adjuvant or radical chemo/radiotherapy. Subsequently, a research group in 2012 treated 16 patients experiencing dysphagia from MES with ELLa-BDS (Griffiths et al., 2012). These patients included those scheduled to undergo neoadjuvant chemotherapy and planned esophagectomy (n = 9), those slated for radical radiotherapy with or without chemotherapy (n = 6), and those with metastatic esophageal cancer. This pioneering study represented the first exploration of BDS use in patients with a variety of malignancies. The results indicated that ELLa-BDS significantly alleviated dysphagia and reduced the need for enteral tubes in patients receiving radical chemoradiotherapy or awaiting esophagectomy. McCain et al. (2016) inserted ELLa-BDS in 11 patients with MES in 2016, further confirming the utility of ELLa-BDS in MES. Their study provided compelling evidence that BDS placement was a safe and effective adjunct in the management of MES. Importantly, it enabled the maintenance of enteral nutrition after staging or neo-adjuvant therapy, without adversely affecting subsequent surgical resection.
The insertion of an esophageal stent delivers instant relief in the palliative treatment of MES-induced dysphagia, whereas brachytherapy provides sustained relief (Hirdes et al, 2012b). A study conducted in 2012 assessed the impact of concurrent brachytherapy and ELLa-BDS insertion in 19 patients diagnosed with unresectable esophageal cancer (Hirdes et al, 2012b). This combination approach successfully restored lumen patency; however, it cannot be recommended for palliative esophageal carcinoma treatment owing to the increased likelihood of severe complications associated with the procedure. In 2014, van den Berg et al. (2014) investigated the safety and efficacy of ELLa-BDS as a surgical bridge preceding CRT in patients with dysphagia from locally advanced esophageal cancer. This study marked the first description of BDS insertion prior to neoadjuvant CRT in individuals diagnosed with locally advanced esophageal cancer. Importantly, no significant adverse incidents or mortality within 30 days associated with the procedure were observed, establishing the approach as safe and feasible. Individuals with esophageal cancer are at risk of developing RBES following ESD or CRT. Yano et al. (2017) confirmed that BDS is an efficient and well-tolerated therapeutic choice for RBES that occurs after esophageal cancer treatment, particularly following ESD or CRT; however, its long-term efficacy remains limited. In 2021, Maishman et al. (2021) employed the SIMON two-stage, single-arm, prospective phase II trial design to assess the efficacy of biodegradable stents in combination with radiotherapy in patients with esophageal cancer-related dysphagia who were not candidates for radical interventions. The observed elevated intervention rates indicate that the proposed alternative treatments may not be sufficiently efficacious to justify their inclusion in larger-scale trial designs. Therefore, additional research is required.
Furthermore, certain complications associated with ELLa-BDS treatment for BES have been documented. These include severe epithelial hyperplasia (Orive-Calzada et al., 2009; Hair and Devonshire, 2010; Dumoulin and Plassmann, 2012; Fischer et al., 2012), tracheoesophageal fistula (Jung et al., 2010), and hepatic abscess (Lopez-Tobaruela et al., 2021). In 2009, researchers inserted ELLa-BDS to address postoperative anastomotic stenosis in a patient (Orive-Calzada et al., 2009). However, the patient developed progressive dysphagia 3 months afterwards. Endoscopic examination showed that the stent had already degraded, and a new severe stenosis had developed, which was attributed to hyperplastic inflammatory tissue. This marked the inaugural report of this particular complication associated with ELLa-BDS, which was effectively managed using balloon dilation. In 2012, Dumoulin and Plassmann (2012) suggested argon plasma coagulation as another potential treatment option for this complication, considering its low risk of complications (Manner, 2008) and the alleviation of tissue hyperplasia once the stent is fully degraded.
In summary, ELLa-BDS demonstrates remarkable flexibility and biocompatibility. As previously mentioned, multiple studies have consistently confirmed the safety and efficacy of ELLa-BDS (van Boeckel et al., 2011; van Hooft et al., 2011; Hirdes et al, 2012a; McCain et al., 2016; Walter et al., 2018; Kailla et al., 2023). ELLa-BDS exhibited more promising results than PLLA-BDS, with a notably low migration rate (Dhar et al., 2009; van Boeckel et al., 2011; van Hooft et al., 2011; Yang et al., 2016). However, it has lower mechanical stability and a faster degradation rate than PLLA, typically undergoing complete degradation within 11–12 weeks. It should be emphasized that this rapid degradation can potentially lead to complications, such as the development of severe epithelial hyperplasia.
Han et al. (2018) designed a groundbreaking PDO and PLLA sheath-core biphasic monofilament to develop a novel BDS with enhanced mechanical characteristics and regulated biodegradability. This monofilament was further enhanced through functionalization with a conjugate of hyaluronic acid (HA) and dopamine (DA) and the addition of BaSO4 to enhance tissue adhesion and radiopacity. This pioneering stent design effectively harnessed PLLA’s relatively high mechanical properties, while mitigating the inherent drawback of PDO’s low mechanical stability during degradation. PDO/PLLA sheath-core monofilaments consistently exhibited superior long-term mechanical stability throughout the in vitro degradation assessment compared to PDO alone. Simultaneously, the stent combined DA’s outstanding binding properties with HA’s enhanced biocompatibility and anti-fouling effects. Furthermore, this design eliminated the need for stent removal and the associated trauma compared to non-degradable stents. Therefore, the performance of the newly developed biodegradable esophageal stent model in this study surpassed that of non-degradable stents and PDO stents. These findings hold significant relevance for future research in this field.
2.1.4 PCL
PCL is an aliphatic polyester that belongs to the category of semi-crystalline polymers. It degrades relatively slowly and generates only minimal acidity during the degradation process (Rai et al., 2016). PCL is distinctive among other biodegradable polyesters owing to its commendable biocompatibility, elastic properties, resistance to fatigue, and cost-effectiveness (Little et al., 2009). PCL has been utilized in a variety of medical domains, including wound dressings, drug delivery devices, tissue engineering scaffolds, artificial blood vessels, and nerve regeneration devices (Sinha et al., 2004; Woodruff and Hutmacher, 2010; Abedalwafa et al., 2013; Mohamed and Yusoh, 2016). Furthermore, PCL is a highly promising candidate for use in drug delivery devices owing to its excellent biodegradability, mechanical characteristics, synthetic adaptability, compatibility with various drugs, and high drug permeability (Guarino et al., 2002; Labet and Thielemans, 2009; Rai et al., 2016; Washington et al., 2017; Thakur et al., 2021; Łukasiewicz et al., 2021).
Lei et al. (2010) employed 5-fluorouracil (5-FU) and PCL to produce multilayered films on esophageal stents. These films comprised a drug-free backing layer and a surface drug layer applied on the primary drug layer, allowing for a unidirectional and controlled drug release while enhancing mechanical characteristics. A series of studies focusing on the release and permeation behavior of the 5-FU-PCL multilayer proved the excellent performance of these multilayer films in the context of drug-controlled release stents. These flexible multilayer films exhibited the capacity to modulate drug release effectively and were regarded as a promising avenue for the development of DES. In 2013, Zhu et al. (2013a) (Zhu et al., 2013b) developed a novel biodegradable PCL-PTX nanofiber-covered metal stent. They evaluated its efficacy in managing benign cardiac stenosis in dogs, demonstrating that this innovative stent delivered ample radial force compared to its bare metal counterpart. Furthermore, PTX was steadily and continuously released through matrix diffusion and degradation, even with prolonged stent placement. This controlled release of PTX reduced inflammation and fibrosis and hindered scar tissue formation, ultimately improving disease prognosis. Notably, PTX exhibited a stable release duration under the acidic condition (pH = 4.0), lasting up to 32 days. In vitro studies showed that fibrous membranes containing a higher PTX concentration exhibited a more significant inhibitory impact on the proliferation rate of smooth muscle cells.
2.1.5 PTMC
PTMC is a flexible amorphous polymer and a linear aliphatic polyester compound renowned for its remarkable elasticity and high toughness under room temperature and in vivo conditions (Jiang et al., 2022). Its exceptional biocompatibility and biodegradability (Hou et al., 2020), coupled with the absence of acidic degradation products during breakdown, effectively prevent severe inflammatory reactions (Yang et al., 2014; Yang et al., 2015). This versatility renders PTMC a valuable material in various biomedical applications, including drug delivery systems (Mohajeri et al., 2020; Hou et al., 2023) and tissue engineering (Li et al., 2020; Brossier et al., 2021; He et al., 2021), among others. In recent studies, researchers have explored hybrid polymers that combine PCL and PTMC as biodegradable coatings on Mg alloy stents, with promising results (Yuan et al., 2016). The growing attention on PTMC indicates its substantial potential for further advancements, despite its underutilization in esophageal stents.
2.2 Biodegradable Mg-based materials
Some studies have reported promising clinical trial outcomes for biodegradable polymer stents; however, they present with shortcomings, primarily concerning mechanical strength. Researchers have focused their attention on Mg alloys, a type of biodegradable metal, owing to their remarkable biocompatibility (Chen et al., 2018) and robust support capabilities. Mg alloys provide ample radial support force, particularly in cases of moderate to severe stenosis, effectively mitigating tissue hyperplasia (Gu et al., 2009; Li et al., 2015). Additionally, the degradation products of Mg alloys are alkaline, capable of neutralizing the acidic pH environment found at cancer sites, thus exhibiting the potential to inhibit cancer cells (Wang et al., 2019). Currently, Mg alloys have widespread applications in the biomedical field, including their use in fracture fixation, treatment of tracheal stenosis, and clinical deployment in coronary vascular stents, among others (Zhang et al., 2010; Perkins et al., 2015; Yue et al., 2015; Han et al., 2016). Nevertheless, a significant barrier that hinders the broad acceptance of Mg-based implants is their swift rate of degradation, with approximately 50% degradation occurring in vitro within just 1 week. This rapid degradation can result in the swift deterioration of the alloy’s mechanical properties, introducing difficulties in maintaining long-term structural support post implantation for effective disease treatment (Wong et al., 2010). Therefore, researchers in the field of biomaterials often focus on enhancing the corrosion resistance of Mg-based alloys, which is a critical advancement for the clinical application of Mg-based stents (Kirkland et al., 2010; Mueller et al., 2010). Some studies have explored methods involving the combination of Mg alloys with other metals or the coating of Mg alloys with non-degradable or degradable materials to address the limitations of pure Mg stents.
2.2.1 Mg-Zn-Y-Nd alloy
Alloying strategies are frequently employed to enhance the properties of Mg alloys. Wang et al. (2020) investigated an Mg-Zn-Y-Nd alloy (Henan Key Laboratory of Advanced Magnesium Alloy, Zhengzhou, China), which has attracted substantial interest in the realm of cardiovascular stents (Chen et al., 2018; Wang et al., 2019). They investigated the viability of using this alloy as a biodegradable material for esophageal stents by conducting a comparative analysis of its mechanical properties against 317L stainless steel (a commercial non-degradable metal) and investigating the function of this alloy in inhibiting esophageal cancer cells. Their findings unveiled the promising potential of the Mg-Zn-Y-Nd alloy, primarily attributed to its low hardness and capacity to impede the growth of pathological cells associated with esophageal cancer. The integration of Mg alloy with advanced surface modification technologies may enhance the effectiveness of esophageal stents, thereby raising the standard of care for individuals with MES (Wang et al., 2020).
2.2.2 Mg-polymer composites
The application of surface coatings on Mg alloys is essential for improving the performance of Mg-based stents. Currently, a variety of polymer materials have been widely employed to protect the surface of Mg alloys. These polymers include PLA (Chen et al., 2011; Li et al., 2018), PLGA (Liu and Xi, 2016; Chen et al, 2019b) and PCL (Chen et al., 2011; Zhang et al., 2016). Liu et al. (2022) focused on surface coating for esophageal stents and designed and tested a braided Mg-based stent coated with PLGA containing PTX, as previously described. This stent combined the strengths of Mg alloy and biodegradable synthetic polymer, with the additional benefit of PTX to inhibit tissue proliferation and prevent restenosis. This innovative approach has paved the way for further research, providing exciting opportunities to explore synergies between stents, coatings, and drug therapies to achieve functional improvements.
However, the acidic degradation products and general degradation behavior of the aforementioned polymers may potentially compromise the corrosion resistance of Mg alloy, rather than enhance it (Chen et al., 2011). Therefore, PTMC has garnered attention owing to its homogeneous surface-eroding behavior, which occurs uniformly from exterior to interior, and the production of charge-neutral degradation products. Its exceptional protective qualities render it a highly promising material for polymer coatings (Wang et al., 2013; Pan et al., 2020; Pan et al., 2022; Tang et al., 2022). Furthermore, silicon is a commonly utilized non-biodegradable coating that has garnered widespread applications in SEMS and SEPS.
Several studies have applied coatings of the aforementioned polymers or silicon to enhance the performance of esophageal stents constructed from Mg-based alloys (Table 4). In 2016, Yuan et al. (2016) selected a blended polymer composed of PCL and PTMC for use as a biodegradable coating on Mg alloy (AZ31, Mg-3Al-1Zn) stents to develop a fully biodegradable esophageal stent. This innovative approach yielded stents characterized by exceptional flexibility, elasticity, and remarkable resistance to lesion compression, as validated through rigorous mechanical testing. The PCL-PTMC membrane played a pivotal role in significantly slowing the degradation rate of Mg alloys in vitro. In vivo research further emphasized the potential of these stents, illustrating their capacity to offer substantial support for a minimum of 4 weeks, devoid of any significant harm or excessive collagen accumulation. This breakthrough holds promise for clinical applications and offers a fresh perspective for the future development of biodegradable stents (Figure 3).
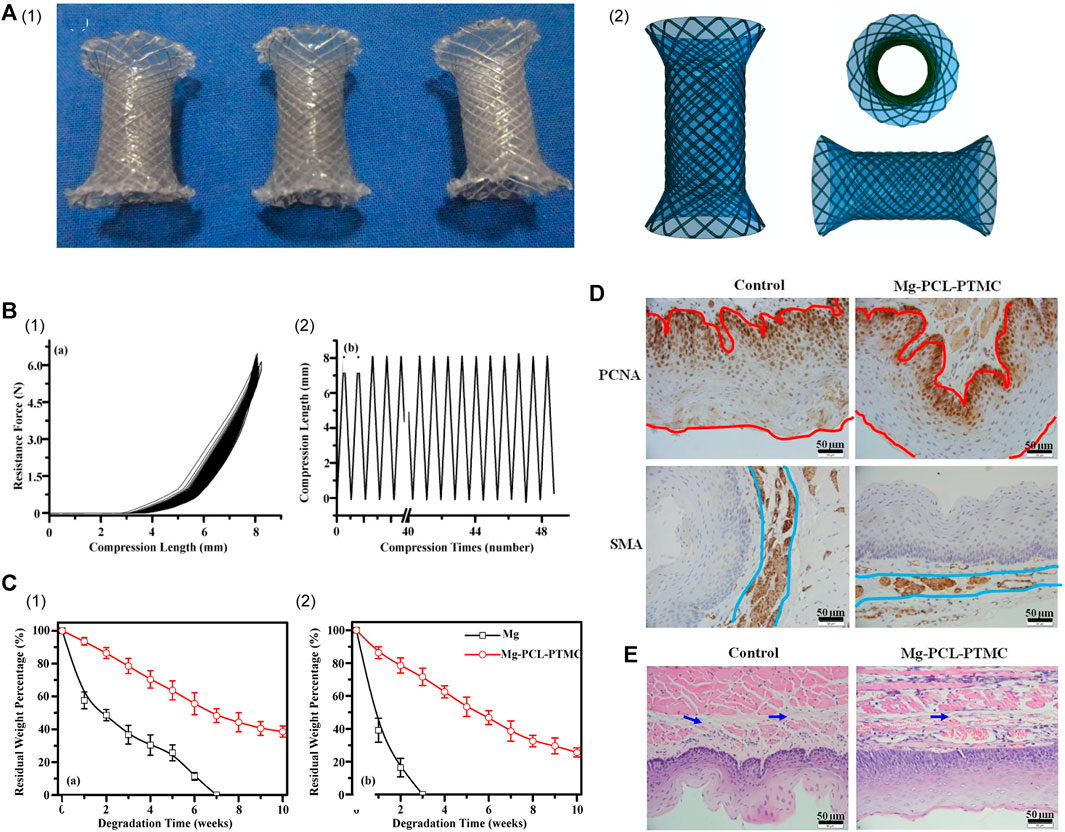
FIGURE 3. (A) (I) Photographs of the ideal expanded PCL-PTMC coated Mg-stent shape. (II) The covered membrane stent’s different profile diagrammatic cross-section. (B) (I) Mechanical compression curve analysis of the stent. (II) Compression-recovery curves of length and time in repeated compression tests (n = 5, constant pressure = 10 N). (C) Analysis of the degradation of the bare Mg and Mg-(PCL-PTMC) stent samples in phosphate-buffered saline with pH values of 7.4 (I) and 4.0 (II). (D) Both the epithelial and smooth muscle cell (SMC) layers were significantly thinner in the Mg-(PCL-PTMC) stent group than in the control group (Mg × 400, the red and blue lines indicate the thickness of the epithelial and SMA layers, respectively). (E) HE trichrome staining showed collagen deposition in the submucosa in the control and Mg-(PCL-PTMC) stent groups. p < 0.05 for comparisons between the control and Mg-(PCL-PTMC) stent groups. Reproduced with permission from ref [(Yuan et al., 2016)]. CC BY 4.0. Copyright 2016 The Author(s).
Yang et al. (2019) (Zhu et al., 2017) developed an Mg stent coated with silicon, which possessed excellent flexibility and elasticity and exhibited impressive resilience to lesion compression during in vivo testing. In their study, the research team inserted the stents into the healthy esophagi of rabbits and observed that they offered sufficient radial force, with the silicon membrane significantly decreasing the rate of Mg biodegradation. Remarkably, the stent facilitated the remodeling of the esophageal wall with minimal tissue damage and elicited a minimal inflammatory response. Importantly, the stent proved capable of meeting crucial clinical requirements, including strength, safety, and reduced complications, with no esophagus injury or stent migration occurring in the rabbit experiments. Therefore, this silicon-coated Mg stent is expected to become a promising strategy for treating BES.
The fusion of Mg alloys with surface modification techniques bestows Mg-based materials with new surface attributes while preserving their intrinsic qualities. The application of suitable coatings onto the surface of Mg-based stents is a potent means to extend the degradation timeline of Mg-based implants, bolster their mechanical properties, and enhance their biological functionalities. Nonetheless, single-layer coatings might fall short of meeting the comprehensive performance requirements, including corrosion resistance and multiple functionalities. Therefore, future endeavors should focus on crafting composite coatings that harness the advantageous properties of each individual layer, thereby elevating the biological activity and adhesion of Mg-based stents (Zhang et al., 2021). In addition, the effective support duration of covered Mg stents remains limited, partly owing to a relatively high migration rate ranging from 40% to 58.3% (Yuan et al., 2016; Zhu et al., 2017; Yang et al., 2019; Liu et al., 2022). Addressing this issue necessitates further research focused on reducing the biodegradation rate and prolonging the duration of support. Extended follow-up studies are also essential for evaluating the efficacy of covered Mg stents, identifying the best time for insertion, and elucidating tissue reactions.
In summary, esophageal stents constructed from synthetic polyester polymers and Mg-based alloy materials have their own individual set of advantages and disadvantages, highlighting the need for further research to explore stents with improved performance. Currently, esophageal stents primarily serve the purpose of enlarging the esophageal lumen, particularly for addressing MES such as tumors. Coated SEMS have shown promise in reducing tumor cell ingrowth (Rozanes et al., 2002); however, they lack inherent anti-tumor or anti-proliferation capabilities. DES has been in development for many years; however, its research and application have been predominantly concentrated in the fields of coronary artery disease and peripheral artery disease, with research on esophageal stents being comparatively underexplored with slow progress. Stent migration is also a common problem with esophageal stents. Further technological innovation and animal and clinical trials are required to address these challenges. Additionally, the existing stent manufacturing technologies and materials are relatively limited, relying mainly on braiding technology, polyester polymers, and alloy materials. Urgent innovation is required to diversify and customize stent types, functions, and manufacturing processes.
3 Innovations and outlook
3.1 Functional innovation
3.1.1 Unidirectional drug-release function
In recent years, DES has garnered increasing attention across various medical disciplines. It typically comprises three primary components: the stent body, the stent coating (generally composed of polymers), and an anti-proliferative drug (Garg et al., 2013). As previously mentioned, researchers are integrating antiproliferative drugs with stent coatings, applying them to either traditional SEMS or emerging BES. This approach preserves the primary function of the stent and facilitates the localized, sustained release of drugs. This effectively inhibits tissue proliferation in restenosis or tumor growth to attain the required local drug concentration, without necessitating potentially harmful systemic doses. Furthermore, some researchers have pioneered innovative drug-loading techniques to enhance the drug-release performance of DES. They have designed double-layer or multi-layer drug-loaded films that enable the unidirectional release of drug molecules onto the esophageal wall, ultimately achieving more precise and efficient treatment.
Guo et al (2007a) (Guo et al, 2007b) fully blended 5-FU particles and ethylene-vinyl acetate (EVA) in various proportions and compressed them into a film using a heat source to create a 5-FU-loaded layer. The drug-loaded layer was combined with a drug-free EVA protective layer, resulting in the development of an esophageal stent coating capable of unidirectional drug molecule release. Subsequently, they wrapped this coating around a nickel-titanium stent under controlled pressure at 70°C to form a 5-FU-coated stent. The findings indicated that regulating the permeation rate of 5-FU through the porcine esophageal mucosa was possible by adjusting the drug content within the coating. The coating containing 20%–60% 5-FU exhibited the ability to endure repeated binding and release through the stent introducer. In a subsequent study by the same team, they inserted the stent into the esophagi of healthy New Zealand albino rabbits. The results revealed that the 5-FU concentration in the esophageal tissue exposed to the stent, particularly the mucosal layer, consistently exceeded that in the serum or liver. This indicated that the stent had high efficiency and long-term local drug delivery capabilities and held the potential for the treatment of esophageal tumors and other tumors within the digestive and respiratory tracts (Guo et al., 2010). In 2015, Liu et al. (2015) (Wang et al., 2015) utilized the aforementioned approach (Guo et al, 2007b; Guo et al., 2010) to prepare nickel-titanium alloy stents. These stents incorporated a bilayered polymer film that contained either 5-FU or PTX. The researchers subsequently inserted these stents into the esophagi of healthy Bama mini-pigs for in vivo evaluation. The 5-FU stent and PTX stent did not induce significant damage to the esophageal wall at their insertion sites and did not exhibit obvious local or systemic toxicity. Furthermore, these stents efficiently suppressed ulceration, inflammation, and the growth of granulation tissue. These two DESs exhibited dual functionality as a stent and a localized drug delivery device, presenting a potential approach characterized by high effectiveness and an absence of systemic toxicity for treating esophageal cancer.
Researchers conducted experiments by incorporating varying concentrations of DTX into PurSil AL 20 (silicone-modified polyurethane polymer, PUS), which serves as a suitable material for covering SEMS, to achieve different loadings (Figure 4) (Shaikh et al., 2015). The solution was mixed evenly by vortex and ultrasonic, resulting in the formation of DTX-loaded films after the solution was dried. Researchers attached a blank film to the DTX-loaded film, creating a bilayer film, to ensure unidirectional drug delivery to the esophagus. Test outcomes demonstrated the physical and chemical compatibility of DTX and PUS, highlighting the outstanding physical and chemical stability of the DTX-loaded PUS membrane. The bilayer film ingeniously designed by the team exhibited sustained release properties (over 30 days) and held promise as a localized, continuous drug delivery system when combined with a stent.
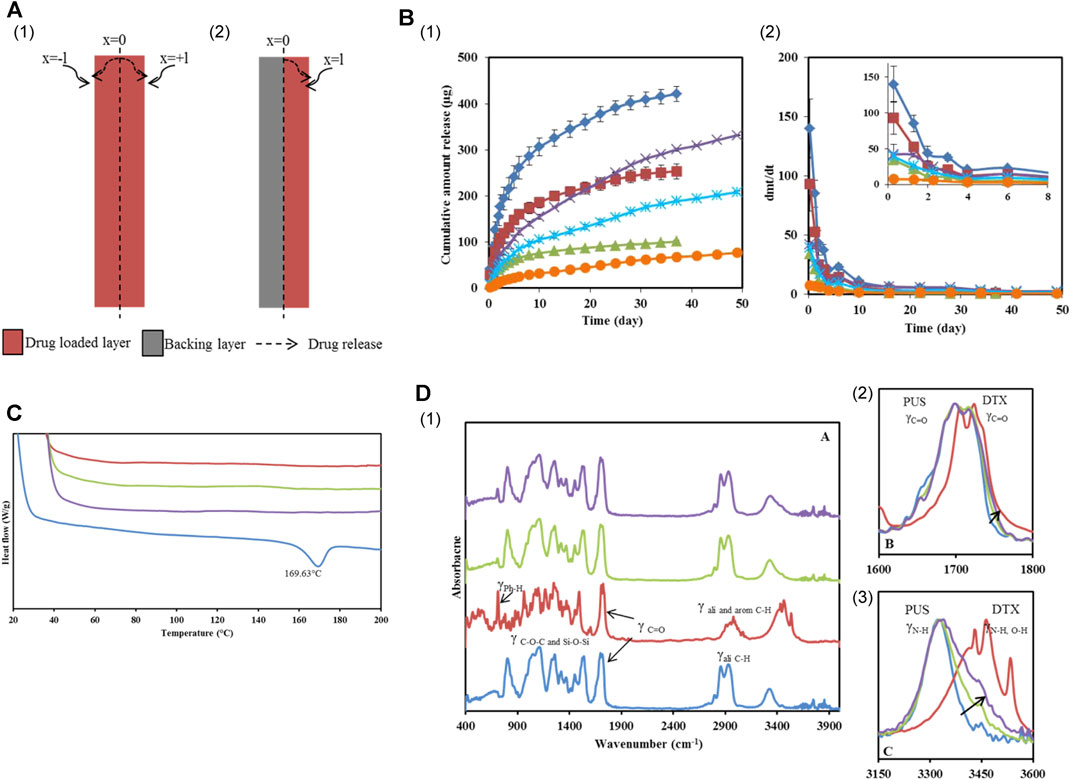
FIGURE 4. (A) Schematic illustration of DTX permeation through the mono and bilayer film. (B) In vitro DTX release profiles from Group 2 and 3 films. (I) Cumulative amount released and (II) Release rate from films loaded with different concentrations. (C) DES thermograms of PUS, 10% and 20% w/w DTX loaded PUS films and DTX. (D) (I) PA-FTIR spectra of PUS, DTX, 10% and 20% w/w DTX loaded films. (II and III) were the magnified spectra of the carbonyl and H-bond stretching regions. Reproduced with permission from ref [(Shaikh et al., 2015)]. Copyright 2015 American Chemical Society.
In addition, Jin et al. (2018) introduced an innovative approach by designing and developing a novel antitumor drug/esophagus stent combination. This combination consisted of a magnetocaloric nitinol stent with a bi-layered film, which included one layer of ethylene-vinyl acetate copolymer (EVA) as a drug-blocking layer and another layer of EVA containing 10% PTX and 30% temperature-sensitive phase-change fatty alcohol (1-tetradecanol, 1-hexadecanol or 1-octadecanol). The stent exhibited excellent compatibility and safe magnetothermal drug release capabilities. The release rate of PTX from the PTX/nitinol complex accelerated upon subjection to an alternating electromagnetic field, resulting in a greater release amount. The penetration of PTX into the esophageal wall or deep esophageal musculature was significantly enhanced compared to that at 37°C. This experiment essentially combined a stent, an anticancer drug, and a phase-change material to enhance drug release and ease the penetration of drugs into esophageal tissue. This novel approach introduced a fresh stent design concept for managing esophageal cancer.
In conclusion, the introduction of drug-eluting esophageal stents and associated technological innovations has introduced new dimensions to the overall progress in esophageal stent development. Conducting valuable research aimed at improving drug-loaded polymers, refining drug coating manufacturing techniques, and performing associated investigations is crucial to further this advancement. Extensive studies of additional animals and patients are imperative to substantiate the efficacy and safety of these promising implants. Additionally, exploring alternatives such as DNA, siRNA, miRNA, and nanoparticles instead of traditional drugs for stent improvement, which is a field more extensively studied in cardiovascular stents (Zhao et al., 2018; Beshchasna et al., 2020), holds significant reference value for the ongoing development of esophageal stents.
3.1.2 Anti-migration function
Due to the inherent physiological characteristics and peristaltic movements of the esophagus, coupled with the limited mechanical resilience of biodegradable stents, especially, there is a notable susceptibility to migration in biodegradable esophageal stents. Previous studies have indeed reported instances of early migration (Saito et al., 2007; Tanaka et al., 2007). Besides, despite the commendable mechanical properties of SEMS, the issue of stent migration persists, particularly in the case of fully-covered SEMS. Consequently, the innovation in the realm of anti-migration function emerges as a pivotal factor in enhancing stent performance.
In a noteworthy advancement in 2018, Lin et al. (2019) utilized 3D printing technology to fabricate esophageal stents with spirals. These stents exhibited substantial potential in mitigating the risk of migration, which will be elaborated upon later. Furthermore, in a recent patent, Clerc et al. (2022) introduced a novel approach by employing dissolvable or degradable adhesive polymers to fashion an anti-migration stent. This stent comprised both an inner and outer surface, with at least a portion of the outer surface incorporating a dissolvable or degradable adhesive polymer. The dissolvable adhesive polymer gradually dissolved in an aqueous environment over time, and the polymer layer was strategically positioned to come into contact with the inner surface of esophagus. Consequently, the activation of these polymer layers enhanced the adhesion of the self-expanding stent to esophagus, effectively resisting migration.
Moreover, it is crucial to design stents that not only minimize the extent of migration within the body lumen but also allow for easy removal and/or repositioning post-deployment. Hynes et al. (2022) introduced an expandable stent with distinct areas: a covered first section (facilitating removal) and an uncovered second section (reducing migration tendency). A biodegradable gripping material, featuring a roughened outer surface to resist post-implantation migration, was applied to at least one area of the biostable covering’s outer surface. The stent was designed to transition from a collapsed to an expanded state, with the second section intended to contact the inner surface of the body cavity in the expanded state.
While the stent designs outlined in the aforementioned patents have not been implemented in animals and clinical patients, their innovative approach to preventing migration has charted a new course for enhancing the performance of esophageal stent in future developments. It is anticipated that, leveraging these designs, multifunctional esophageal stents with enhanced performance can be developed.
3.2 Manufacturing technology innovation
Stent manufacturing technology has undergone continuous breakthroughs and advancements. Five main stent manufacturing methods are currently used: etching, micro-electro discharge machining, electroforming, die-casting, and the most commonly used laser cutting (Kathuria, 2006). Although laser cutting technology has matured, it still presents disadvantages such as high costs and the inability to cater to patients’ personalized customization needs (Wang et al, 2021a). Consequently, attention is shifting towards 3D printing technology, which is cost-effective, allows for personalized customization, and offers a broader range of material options.
3D printing is a digital manufacturing technology that utilizes bondable materials to construct objects layer-by-layer. Various 3D printing technologies, such as inkjet printing, stereolithography, selective laser sintering, and fused deposition modelling (Wang et al, 2021a), provide distinct advantages over traditional methods such as weaving, knitting, laser cutting, and segmentation. This innovative approach overcomes some of the limitations of current stent production and facilitates the customization and personalization of stent designs (Kang, 2019).
In 2018, a group utilized simulated structural parameters to 3D print four types of PLA/polyurethane (TPU) stents that incorporated spirals using a custom-made 3D printer equipped with four sets of PLA/TPU composites (Lin et al., 2019). Their findings indicated that 3D-printed stents with spirals displayed a significant potential for reducing migration risk and exhibited greater expansion force compared to stents lacking spirals. These stents degraded slowly, and their mechanical properties remained relatively stable even after 3 months of insertion, exhibiting the promising potential of 3D-printed esophageal stents in managing malignant esophageal diseases. Notably, this pioneering research marked the initial utilization of 3D printing technology for creating a polymer esophageal stent, showcasing the capability of this technique to produce esophageal stents with varying sizes and shapes. Further research is warranted to fully explore the functionality of 3D-printed esophageal stents (Kang, 2019).
In 2020, Fouladian et al. (2020) fabricated a 3D-printed DES using fused deposition modelling. This innovative stent featured a design where the central segment of the stent was constructed using PU filament loaded with 5-FU, whereas the two ends were constructed with drug-free PU filament. Test results indicated that this stent was capable of consistently releasing 5-FU over an impressive period of 110 days. Additionally, the stent exhibited remarkable stability, even when subjected to sterilization procedures involving gamma and ultraviolet irradiation and during accelerated storage conditions. This research demonstrated the effectiveness of 3D printing as a robust tool for constructing DES, which can be readily personalized to offer patient-specific geometries and precise drug dosages.
3D printing technology has seen limited application in the production of biodegradable esophageal stents; nevertheless, the results from previous experiments and its successful use in manufacturing other stent types are promising. We anticipate ongoing advancements and increased utilization of 3D printing technology to offer more tailored and precise treatments for esophageal stents, including DES with enhanced functionalities.
3.3 Material innovation
Hydrogels arising from the physical or chemical cross-linking of polymer chains represent hydrophilic polymers characterized by a three-dimensional porous structure (Zhang and Khademhosseini, 2017; Liu et al., 2018). They possess a soft and pliable texture, excel in retaining water, and exhibit commendable biocompatibility (Cai et al., 2022). Hydrogels have promising applications across various biomedical fields (Ustürk et al., 2022), including drug delivery systems (Zhang et al., 2019; Kasiński et al., 2020), cell culture substrates (Fukunaga et al., 2019), wound dressings (Mandal et al., 2020), and tissue engineering scaffolds (Neves et al., 2020; Unal and West, 2020).
In 2020, Raman et al. (2020) constructed a tough acrylate ortho-nitrobenzyl (oNB) light-triggered degradable hydrogel with adjustable mechanical characteristics and a modular design that can undergo safe, contact-free degradation at various anatomical sites within the body. The material and its degradation by-products were shown to be biocompatible. This group constructed an oNB esophageal stent using this light-triggerable hydrogel in combination with PCL, envisioning its application for offering structural support and/or localized drug delivery within the esophagus. Results from a series of in vitro and ex vivo trials suggested that these stents would persist in the esophagus when deployed in vivo until they naturally biodegrade. Subsequently, peristaltic waves within the gastrointestinal tract would gently transport the degraded material, eliminating the need for mechanical, thermal, or chemical triggers and minimizing adverse effects on the esophagus. This light-triggering mechanism provided a non-contact approach for the safe extraction of the stent from the lower esophageal sphincter, rendering it suitable for addressing both benign and malignant strictures. In a recent study, researchers developed CNCMA-pHEMA hydrogels using methacrylate cellulose nanocrystals (CNCMAs) as a macro-cross-linking agent in poly (2-hydroxyethyl methacrylate) (pHEMA) hydrogels (Figure 5) (Pruksawan et al., 2022). These hydrogels exhibited significantly enhanced toughness and tensile properties compared to hydrogels cross-linked with conventional agents. The remarkable strength and durability of these hydrogels render them promising candidates for advanced flexible implantable devices, including biodegradable esophageal self-expanding stents. Consequently, biodegradable hydrogels hold tremendous potential for the development of such stents.
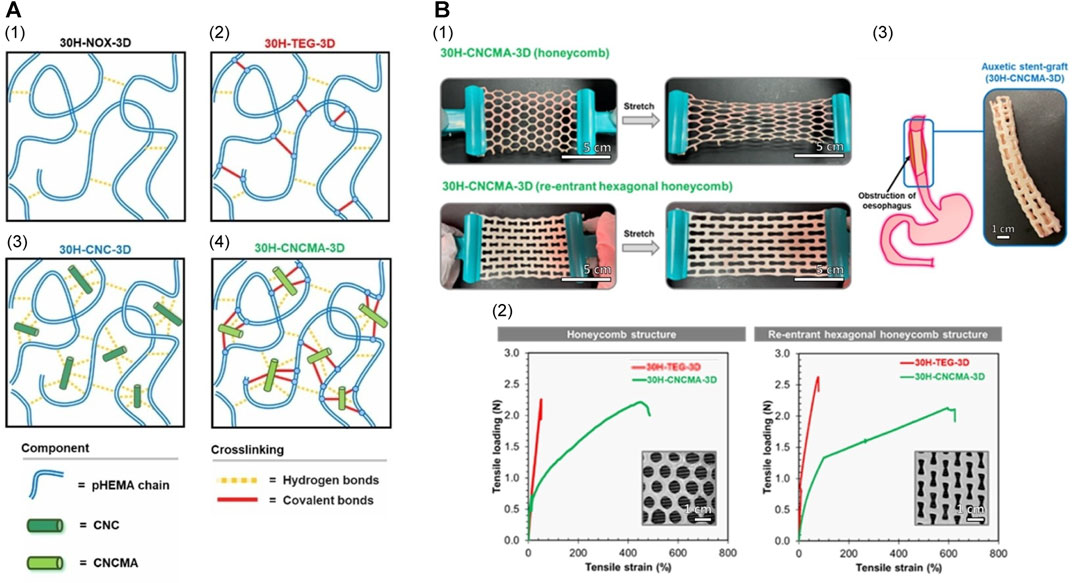
FIGURE 5. (A) Proposed schematic cross-linking networks for each hydrogel system. (B) Mechanical properties of pHEMA hydrogel specimens with conventional honeycomb and auxetic re-entrant hexagonal honeycomb structures. (I) Demonstration of the conventional and auxetic CNCMA-HEMA hydrogel specimens. The auxetic hydrogel specimen was expandable in the lateral direction when stretched while the conventional hydrogel specimen contracted. (II) Tensile load-strain curves of pHEMA hydrogel specimens with conventional and auxetic structures. (III) Esophageal stent made from auxetic CNCMA-HEMA hydrogel. Reproduced with permission from ref [(Pruksawan et al., 2022)]. Copyright 2022 Wiley-VCH GmbH.
3.4 Outlook
Biodegradable esophageal stents have garnered significant attention owing to their favorable biocompatibility and unique advantages, including the avoidance of secondary interventions. These stents have been employed in numerous animal experiments and clinical applications. However, biodegradable stents lag behind metal stents in terms of stiffness and strength. This discrepancy can lead to complications such as early displacement. Additionally, they are susceptible to the acidic environment in the esophagus, hastening the degradation process. The development of a more optimal biodegradable stent remains a challenge, despite the abovementioned ongoing innovative research in functions, technologies, and materials. Future research should delve more deeply into the material’s mechanical properties, degradation time, and surface characteristics to address these issues.
4 Conclusion
Biodegradable esophageal stents approved for clinical use, such as PLLA-BDS, ELLa-BDS, and other stents manufactured from synthetic polyester polymers and Mg-based alloys, have shown promising results in performance tests and clinical applications. However, this is only the beginning of the era of biodegradable esophageal stents, and the stents currently available in the market require further improvement regarding mechanical stability and material degradation rate, among others. Another promising characteristic of stents is their exceptional capacity to transport and locally release drugs to inhibit tissue proliferation or exert antitumor effects. These drugs can be eluted as the stent itself or the coating degrades, assisting in the treatment of stenosis or eliminating the cause of stenosis, with minimal systemic side effects. In addition, unidirectional drug-release function, anti-migration function, 3D printing technology and hydrogel materials are currently valuable innovations that deserve further exploration to develop better biodegradable esophageal stents.
In summary, the development of biodegradable esophageal stents remains in its initial exploration stage. It is anticipated that this field will witness the application of more appropriate biodegradable materials, enhanced and personalized stent manufacturing methods, improved stent structure and performance, and more precise drug-release technology.
Author contributions
YaY: Conceptualization, Data curation, Visualization, Writing–original draft. YuY: Conceptualization, Data curation, Visualization, Writing–original draft. ZH: Conceptualization, Methodology, Visualization, Writing–original draft. TW: Formal Analysis, Methodology, Visualization, Writing–original draft. PW: Conceptualization, Formal Analysis, Supervision, Writing–original draft. LS: Data curation, Formal Analysis, Methodology, Writing–original draft. PL: Formal Analysis, Methodology, Supervision, Writing–original draft. KZ: Funding acquisition, Resources, Supervision, Writing–review and editing. LY: Formal Analysis, Funding acquisition, Methodology, Writing–review and editing. SS: Conceptualization, Project administration, Supervision, Writing–review and editing.
Funding
The author(s) declare financial support was received for the research, authorship, and/or publication of this article. This work was supported by the National Natural Science Foundation of China (Grant No. 82000625), the Natural Science Foundation of Liaoning Province (Grant No. 2022-YGJC-69), the support program for excellent young scholars of China Medical University, Young and middle-aged science and technology innovation talent Program (Grant No. RC220482), the Doctoral Scientific Research Foundation of Liaoning Province (Grant No. 2020-BS-109) and 345 Talent Project.
Conflict of interest
The authors declare that the research was conducted in the absence of any commercial or financial relationships that could be construed as a potential conflict of interest.
Publisher’s note
All claims expressed in this article are solely those of the authors and do not necessarily represent those of their affiliated organizations, or those of the publisher, the editors and the reviewers. Any product that may be evaluated in this article, or claim that may be made by its manufacturer, is not guaranteed or endorsed by the publisher.
Abbreviations
ES, esophageal stricture; BES, benign esophageal stricture; MES, malignant esophageal stricture; RBES, refractory benign esophageal stricture; GERD, gastroesophageal reflux disease; ESD, endoscopic submucosal dissection; EMR, endoscopic mucosal resection; ESCC, esophageal squamous cell carcinoma; EAC, esophageal adenocarcinoma; SEMS, self-expanding metal stents; SEPS, self-expanding plastic stents; BDS, biodegradable stents; FDA, the US Food and Drug Administration; PLA, polylactic acid; PLLA, poly (L-lactic acid); PLGA, poly (lactic-co-glycolic acid); PDO, polydioxanone; PCL, poly (ε-caprolactone); PTMC, poly (1, 3-trimethylene carbonate); 5-FU, 5-fluorouracil.
References
Abedalwafa, M., Wang, F., Wang, L., and Li, C. (2013). Biodegradable poly-epsilon-caprolactone (PCL) for tissue engineering applications: a review. Rev. Adv. Mater. Sci. 34 (2), 123–140.
Ackroyd, R., Watson, D. I., Devitt, P. G., and Jamieson, G. G. (2001). Expandable metallic stents should not be used in the treatment of benign esophageal strictures. J. gastroenterology hepatology 16 (4), 484–487. doi:10.1046/j.1440-1746.2001.02367.x
Adler, D. G., and Siddiqui, A. A. (2017). Endoscopic management of esophageal strictures. Gastrointest. Endosc. 86 (1), 35–43. doi:10.1016/j.gie.2017.03.004
Antonowicz, M., Szewczenko, J., Jaworska, J., Jelonek, K., Joszko, K., Gzik-Zroska, B., et al. (2021). Functional properties of polyurethane ureteral stents with PLGA and papaverine hydrochloride coating. Int. J. Mol. Sci. 22 (14), 7705. doi:10.3390/ijms22147705
Babilotte, J., Martin, B., Guduric, V., Bareille, R., Agniel, R., Roques, S., et al. (2021). Development and characterization of a PLGA-HA composite material to fabricate 3D-printed scaffolds for bone tissue engineering. Mater. Sci. Eng. C 118, 111334. doi:10.1016/j.msec.2020.111334
Bartholomew, R. (1981). PDS (polydioxanone suture): a new synthetic absorbable suture in cataract surgery. Ophthalmologica 183 (2), 81–85. doi:10.1159/000309144
Basha, J., Appasani, S., Vaiphei, K., Gupta, V., Singh, K., and Kochhar, R. (2013). Biodegradable stents: truly biodegradable with good tissue harmony. Endoscopy 45 (S 02), E116–E117. doi:10.1055/s-0032-1326111
Battersby, I., and Doyle, R. (2010). Use of a biodegradable self-expanding stent in the management of a benign oesophageal stricture in a cat. J. Small Animal Pract. 51 (1), 49–52. doi:10.1111/j.1748-5827.2009.00868.x
Beshchasna, N., Saqib, M., Kraskiewicz, H., Wasyluk, Ł., Kuzmin, O., Duta, O. C., et al. (2020). Recent advances in manufacturing innovative stents. Pharmaceutics 12 (4), 349. doi:10.3390/pharmaceutics12040349
Boland, E. D., Coleman, B. D., Barnes, C. P., Simpson, D. G., Wnek, G. E., and Bowlin, G. L. (2005). Electrospinning polydioxanone for biomedical applications. Acta biomater. 1 (1), 115–123. doi:10.1016/j.actbio.2004.09.003
Bottino, M. C., Albuquerque, M. T., Azabi, A., Münchow, E. A., Spolnik, K. J., Nör, J. E., et al. (2019). A novel patient-specific three-dimensional drug delivery construct for regenerative endodontics. J. Biomed. Mater. Res. Part B Appl. Biomaterials 107 (5), 1576–1586. doi:10.1002/jbm.b.34250
Bowen, P. K., Shearier, E. R., Zhao, S., Guillory, R. J., Zhao, F., Goldman, J., et al. (2016). Biodegradable metals for cardiovascular stents: from clinical concerns to recent Zn-Alloys. Adv. Healthc. Mater. 5 (10), 1121–1140. doi:10.1002/adhm.201501019
Brossier, T., Volpi, G., Vasquez-Villegas, J., Petitjean, N., Guillaume, O., Lapinte, V., et al. (2021). Photoprintable gelatin-graft-poly (trimethylene carbonate) by stereolithography for tissue engineering applications. Biomacromolecules 22 (9), 3873–3883. doi:10.1021/acs.biomac.1c00687
Cai, G., Hou, Z., Sun, W., Li, P., Zhang, J., Yang, L., et al. (2022). Recent developments in biomaterial-based hydrogel as the delivery system for repairing endometrial injury. Front. Bioeng. Biotechnol. 10, 894252. doi:10.3389/fbioe.2022.894252
Chen, C., Chen, J., Wu, W., Shi, Y., Jin, L., Petrini, L., et al. (2019a). In vivo and in vitro evaluation of a biodegradable magnesium vascular stent designed by shape optimization strategy. Biomaterials 221, 119414. doi:10.1016/j.biomaterials.2019.119414
Chen, H., Wang, X., Shao, S., Zhang, J., Tan, X., and Chen, W. (2022). Value of EUS in determining infiltration depth of early carcinoma and associated precancerous lesions in the upper gastrointestinal tract. Endosc. ultrasound 11 (6), 503. doi:10.4103/eus-d-21-00218
Chen, L., Li, J., Chang, J., Jin, S., Wu, D., Yan, H., et al. (2018). Mg-Zn-Y-Nd coated with citric acid and dopamine by layer-by-layer self-assembly to improve surface biocompatibility. Sci. China Technol. Sci. 61, 1228–1237. doi:10.1007/s11431-017-9190-2
Chen, L., Sheng, Y., Zhou, H., Li, Z., Wang, X., and Li, W. (2019b). Influence of a MAO+ PLGA coating on biocorrosion and stress corrosion cracking behavior of a magnesium alloy in a physiological environment. Corros. Sci. 148, 134–143. doi:10.1016/j.corsci.2018.12.005
Chen, X., Chen, X., Bao, Y., Zhang, W., Jiang, L., Zhu, J., et al. (2023). EUS–derived maximum tumor thickness and tumor shrinkage rate as independent prognostic factors in locally advanced esophageal squamous cell carcinoma after neoadjuvant chemoradiotherapy. Endosc. Ultrasound 12 (4), 369–376. doi:10.1097/eus.0000000000000008
Chen, Y., Song, Y., Zhang, S., Li, J., Zhao, C., and Zhang, X. (2011). Interaction between a high purity magnesium surface and PCL and PLA coatings during dynamic degradation. Biomed. Mater. 6 (2), 025005. doi:10.1088/1748-6041/6/2/025005
Clerc, C. O., Fredrickson, G., and Boden, M. (2022). Dissolvable or degradable adhesive polymer to prevent stent migration. Google Pat.
Conio, M., Repici, A., Battaglia, G., De Pretis, G., Ghezzo, L., Bittinger, M., et al. (2007). A randomized prospective comparison of self-expandable plastic stents and partially covered self-expandable metal stents in the palliation of malignant esophageal dysphagia. Official J. Am. Coll. Gastroenterology| ACG 102 (12), 2667–2677. doi:10.1111/j.1572-0241.2007.01565.x
Desai, M., Hamade, N., and Sharma, P. (2020). Management of peptic strictures. Official J. Am. Coll. Gastroenterology| ACG 115 (7), 967–970. doi:10.14309/ajg.0000000000000655
De Wijkerslooth, L. R., Vleggaar, F. P., and Siersema, P. D. (2011). Endoscopic management of difficult or recurrent esophageal strictures. Official J. Am. Coll. Gastroenterology| ACG 106 (12), 2080–2091. doi:10.1038/ajg.2011.348
Dhar, A., Topping, J. H., Johns, E., and O'Neill, D. (2009). Biodegradable stents in refractory benign oesophageal strictures-first report of 4 patients from UK. Gastrointest. Endosc. 69 (5), AB254–AB255. doi:10.1016/j.gie.2009.03.655
Dou, Z., Chen, S., Wang, J., Xia, L., Maitz, M. F., Tu, Q., et al. (2023). A “built-up” composite film with synergistic functionalities on Mg–2Zn–1Mn bioresorbable stents improves corrosion control effects and biocompatibility. Bioact. Mater. 25, 223–238. doi:10.1016/j.bioactmat.2023.02.004
Dumoulin, F., and Plassmann, D. (2012). Tissue hyperplasia following placement of a biodegradable stent for a refractory esophageal stricture: treatment with argon plasma coagulation. Endoscopy 44 (S 02), E356–E357. doi:10.1055/s-0032-1310021
Evrard, S., Le Moine, O., Lazaraki, G., Dormann, A., El Nakadi, I., and Devière, J. (2004). Self-expanding plastic stents for benign esophageal lesions. Gastrointest. Endosc. 60 (6), 894–900. doi:10.1016/s0016-5107(04)02278-3
Fang, H., Qi, X., Zhou, S., Yang, S., Hang, C., Tian, Y., et al. (2021). High-efficient vacuum ultraviolet-ozone assist-deposited polydopamine for poly (lactic-co-glycolic acid)-coated pure Zn toward biodegradable cardiovascular stent applications. ACS Appl. Mater. Interfaces 14 (2), 3536–3550. doi:10.1021/acsami.1c21567
Fischer, A., Bausch, D., Baier, P., Braun, A., and Richter-Schrag, H. (2012). Risk of biodegradable stent-induced hypergranulation causing re-stenosis of a gastric conduit after esophageal resection. Endoscopy 44 (S 02), E125–E126. doi:10.1055/s-0031-1291693
Fouladian, P., Kohlhagen, J., Arafat, M., Afinjuomo, F., Workman, N., Abuhelwa, A. Y., et al. (2020). Three-dimensional printed 5-fluorouracil eluting polyurethane stents for the treatment of oesophageal cancers. Biomaterials Sci. 8 (23), 6625–6636. doi:10.1039/d0bm01355b
Fredenberg, S., Wahlgren, M., Reslow, M., and Axelsson, A. (2011). The mechanisms of drug release in poly (lactic-co-glycolic acid)-based drug delivery systems—a review. Int. J. Pharm. 415 (1-2), 34–52. doi:10.1016/j.ijpharm.2011.05.049
Fry, S. W., and Fleischer, D. E. (1997). Management of a refractory benign esophageal stricture with a new biodegradable stent. Gastrointest. Endosc. 45 (2), 179–182. doi:10.1016/s0016-5107(97)70244-x
Fuccio, L., Hassan, C., Frazzoni, L., Miglio, R., and Repici, A. (2015). Clinical outcomes following stent placement in refractory benign esophageal stricture: a systematic review and meta-analysis. Endoscopy 48, 141–148. doi:10.1055/s-0034-1393331
Fukunaga, K., Tsutsumi, H., and Mihara, H. (2019). Self-assembling peptides as building blocks of functional materials for biomedical applications. Bull. Chem. Soc. Jpn. 92 (2), 391–399. doi:10.1246/bcsj.20180293
Garg, S., Bourantas, C., and Serruys, P. W. (2013). New concepts in the design of drug-eluting coronary stents. Nat. Rev. Cardiol. 10 (5), 248–260. doi:10.1038/nrcardio.2013.13
Goldin, E., Fiorini, A., Ratan, Y., Keter, D., Loshakove, A., Globerman, O., et al. (1996). A new biodegradable and self-expandable stent for benign esophageal strictures. Gastrointest. Endosc. 4 (43), 294. doi:10.1016/s0016-5107(96)80017-4
Goonoo, N., Jeetah, R., Bhaw-Luximon, A., and Jhurry, D. (2015). Polydioxanone-based bio-materials for tissue engineering and drug/gene delivery applications. Eur. J. Pharm. Biopharm. 97, 371–391. doi:10.1016/j.ejpb.2015.05.024
Griffiths, E. A., Gregory, C. J., Pursnani, K. G., Ward, J. B., and Stockwell, R. C. (2012). The use of biodegradable (SX-ELLA) oesophageal stents to treat dysphagia due to benign and malignant oesophageal disease. Surg. Endosc. 26, 2367–2375. doi:10.1007/s00464-012-2192-9
Gu, X., Zheng, Y., Cheng, Y., Zhong, S., and Xi, T. (2009). In vitro corrosion and biocompatibility of binary magnesium alloys. Biomaterials 30 (4), 484–498. doi:10.1016/j.biomaterials.2008.10.021
Guarino, V., Gentile, G., Sorrentino, L., and Ambrosio, L. (2002). Polycaprolactone: synthesis, properties, and applications. Encycl. Polym. Sci. Technol., 1–36. doi:10.1002/0471440264.pst658
Guo, Q., Guo, S., and Wang, Z. (2007a). A type of esophageal stent coating composed of one 5-fluorouracil-containing EVA layer and one drug-free protective layer: in vitro release, permeation and mechanical properties. J. Control. Release 118 (3), 318–324. doi:10.1016/j.jconrel.2006.12.030
Guo, Q.-h., Guo, S.-r., and Wang, Z.-m. (2007b). Estimation of 5-fluorouracil-loaded ethylene-vinyl acetate stent coating based on percolation thresholds. Int. J. Pharm. 333 (1-2), 95–102. doi:10.1016/j.ijpharm.2006.10.011
Guo, S.-R., Wang, Z.-M., Zhang, Y.-Q., Lei, L., Shi, J.-M., Chen, K.-M., et al. (2010). In vivo evaluation of 5-fluorouracil-containing self-expandable nitinol stent in rabbits: efficiency in long-term local drug delivery. J. Pharm. Sci. 99 (7), 3009–3018. doi:10.1002/jps.22066
Hair, C., and Devonshire, D. (2010). Severe hyperplastic tissue stenosis of a novel biodegradable esophageal stent and subsequent successful management with high-pressure balloon dilation. Endoscopy 42 (S 02), E132–E133. doi:10.1055/s-0029-1244011
Ham, Y. H., and Kim, G. H. (2014). Plastic and biodegradable stents for complex and refractory benign esophageal strictures. Clin. Endosc. 47 (4), 295–300. doi:10.5946/ce.2014.47.4.295
Han, C.-M., Lih, E., Choi, S.-K., Bedair, T. M., Lee, Y.-J., Park, W., et al. (2018). Biodegradable sheath-core biphasic monofilament braided stent for bio-functional treatment of esophageal strictures. J. Industrial Eng. Chem. 67, 396–406. doi:10.1016/j.jiec.2018.07.014
Han, J., Wan, P., Ge, Y., Fan, X., Tan, L., Li, J., et al. (2016). Tailoring the degradation and biological response of a magnesium–strontium alloy for potential bone substitute application. Mater. Sci. Eng. C 58, 799–811. doi:10.1016/j.msec.2015.09.057
He, J., Lin, Z., Hu, X., Xing, L., Liang, G., Chen, D., et al. (2021). Biocompatible and biodegradable scaffold based on polytrimethylene carbonate-tricalcium phosphate microspheres for tissue engineering. Colloids Surfaces B Biointerfaces 204, 111808. doi:10.1016/j.colsurfb.2021.111808
Hirdes, M., Siersema, P. D., Van Boeckel, P., and Vleggaar, F. P. (2012a). Single and sequential biodegradable stent placement for refractory benign esophageal strictures: a prospective follow-up study. Endoscopy 44 (07), 649–654. doi:10.1055/s-0032-1309818
Hirdes, M. M., van Hooft, J. E., Wijrdeman, H. K., Hulshof, M. C., Fockens, P., Reerink, O., et al. (2012b). Combination of biodegradable stent placement and single-dose brachytherapy is associated with an unacceptably high complication rate in the treatment of dysphagia from esophageal cancer. Gastrointest. Endosc. 76 (2), 267–274. doi:10.1016/j.gie.2012.04.442
Hirdes, M. M., Vleggaar, F. P., De Beule, M., and Siersema, P. D. (2013). In vitro evaluation of the radial and axial force of self-expanding esophageal stents. Endoscopy 45 (12), 997–1005. doi:10.1055/s-0033-1344985
Hou, Z., Li, P., Guo, J., Wang, J., Hu, J., and Yang, L. (2020). The effect of molecular weight on thermal properties and degradation behavior of copolymers based on TMC and DTC. Polym. Degrad. Stab. 175, 109128. doi:10.1016/j.polymdegradstab.2020.109128
Hou, Z., Xu, W., Chen, S., Guo, J., Li, P., Hu, J., et al. (2023). Biodegradable implants based on photo-cross-linked aliphatic polycarbonates for long-acting contraception. J. Mater. Sci. Technol. 156, 129–141. doi:10.1016/j.jmst.2023.01.040
Hynes, M., Folan, M. G., Wulfman, D. R., Keating, T. M., Montague, M., and Nolan, D. V. (2022). Stent including anti-migration capabilities. Google Pat.
Isotalo, T. M., Nuutine, J. P., Vaajanen, A., Martikainen, P. M., Laurila, M., TörmÄLÄ, P., et al. (2006). Biocompatibility properties of a new braided biodegradable urethral stent: a comparison with a biodegradable spiral and a braided metallic stent in the rabbit urethra. BJU Int. 97 (4), 856–859. doi:10.1111/j.1464-410x.2006.06000.x
Jiang, D., Zou, H., Zhang, H., Zhao, W., Lan, Y., and Yuan, M. (2022). Preparation and properties of electrospun PLLA/PTMC scaffolds. Polymers 14 (20), 4406. doi:10.3390/polym14204406
Jin, S., Xia, X., Huang, J., Yuan, C., Zuo, Y., Li, Y., et al. (2021). Recent advances in PLGA-based biomaterials for bone tissue regeneration. Acta biomater. 127, 56–79. doi:10.1016/j.actbio.2021.03.067
Jin, Z., Wu, K., Hou, J., Yu, K., Shen, Y., and Guo, S. (2018). A PTX/nitinol stent combination with temperature-responsive phase-change 1-hexadecanol for magnetocaloric drug delivery: magnetocaloric drug release and esophagus tissue penetration. Biomaterials 153, 49–58. doi:10.1016/j.biomaterials.2017.10.040
Jung, G., Sauer, P., and Schaible, A. (2010). Tracheoesophageal fistula following implantation of a biodegradable stent for a refractory benign esophageal stricture. Endoscopy 42 (S 02), E338–E339. doi:10.1055/s-0030-1256005
Kailla, E., Rezai, F., Kansci, A. K., Akande, O., and Gossage, J. (2023). SX-ELLA biodegradable stent for benign oesophageal strictures: a systematic review and proportion meta-analysis. Surg. Endosc. 37 (4), 2476–2484. doi:10.1007/s00464-022-09767-w
Kang, Y. (2019). A review of self-expanding esophageal stents for the palliation therapy of inoperable esophageal malignancies. Biomed Res. Int. 2019, 1–11. doi:10.1155/2019/9265017
Kasiński, A., Zielińska-Pisklak, M., Oledzka, E., and Sobczak, M. (2020). Smart hydrogels–synthetic stimuli-responsive antitumor drug release systems. Int. J. nanomedicine 15, 4541–4572. doi:10.2147/ijn.s248987
Kathuria, Y. (2006). The potential of biocompatible metallic stents and preventing restenosis. Mater. Sci. Eng. A 417 (1-2), 40–48. doi:10.1016/j.msea.2005.11.007
Kirkland, N., Lespagnol, J., Birbilis, N., and Staiger, M. (2010). A survey of bio-corrosion rates of magnesium alloys. Corros. Sci. 52 (2), 287–291. doi:10.1016/j.corsci.2009.09.033
Kohn, J., Welsh, W. J., and Knight, D. (2007). A new approach to the rationale discovery of polymeric biomaterials. Biomaterials 28 (29), 4171–4177. doi:10.1016/j.biomaterials.2007.06.022
Kwak, T. W., Lee, H. L., Song, Y. H., Kim, C., Kim, J., Seo, S.-J., et al. (2017). Vorinostat-eluting poly (DL-lactide-co-glycolide) nanofiber-coated stent for inhibition of cholangiocarcinoma cells. Int. J. Nanomedicine 12, 7669–7680. doi:10.2147/ijn.s141920
Labet, M., and Thielemans, W. (2009). Synthesis of polycaprolactone: a review. Chem. Soc. Rev. 38 (12), 3484–3504. doi:10.1039/b820162p
Lam, N., Weisse, C., Berent, A., Kaae, J., Murphy, S., Radlinsky, M., et al. (2013). Esophageal stenting for treatment of refractory benign esophageal strictures in dogs. J. Veterinary Intern. Med. 27 (5), 1064–1070. doi:10.1111/jvim.12132
Lanao, R. P. F., Leeuwenburgh, S. C., Wolke, J. G., and Jansen, J. A. (2011). Bone response to fast-degrading, injectable calcium phosphate cements containing PLGA microparticles. Biomaterials 32 (34), 8839–8847. doi:10.1016/j.biomaterials.2011.08.005
Lasprilla, A. J., Martinez, G. A., Lunelli, B. H., Jardini, A. L., and Maciel Filho, R. (2012). Poly-lactic acid synthesis for application in biomedical devices—a review. Biotechnol. Adv. 30 (1), 321–328. doi:10.1016/j.biotechadv.2011.06.019
Lei, L., Liu, X., Guo, S., Tang, M., Cheng, L., and Tian, L. (2010). 5-Fluorouracil-loaded multilayered films for drug controlled releasing stent application: drug release, microstructure, and ex vivo permeation behaviors. J. Control. Release 146 (1), 45–53. doi:10.1016/j.jconrel.2010.05.017
Li, L., Zhang, X., Shi, J., Chen, Y., Wan, H., Herth, F. J., et al. (2023). Airway stents from now to the future: a narrative review. Respiration 102 (6), 439–448. doi:10.1159/000530421
Li, L.-Y., Cui, L.-Y., Zeng, R.-C., Li, S.-Q., Chen, X.-B., Zheng, Y., et al. (2018). Advances in functionalized polymer coatings on biodegradable magnesium alloys–A review. Acta biomater. 79, 23–36. doi:10.1016/j.actbio.2018.08.030
Li, X., Chen, H., Xie, S., Wang, N., Wu, S., Duan, Y., et al. (2020). Fabrication of photo-crosslinkable poly (trimethylene carbonate)/polycaprolactone nanofibrous scaffolds for tendon regeneration. Int. J. Nanomedicine 15, 6373–6383. doi:10.2147/ijn.s246966
Li, X., Chu, C., Liu, L., Liu, X., Bai, J., Guo, C., et al. (2015). Biodegradable poly-lactic acid based-composite reinforced unidirectionally with high-strength magnesium alloy wires. Biomaterials 49, 135–144. doi:10.1016/j.biomaterials.2015.01.060
Lin, M., Firoozi, N., Tsai, C.-T., Wallace, M. B., and Kang, Y. (2019). 3D-printed flexible polymer stents for potential applications in inoperable esophageal malignancies. Acta biomater. 83, 119–129. doi:10.1016/j.actbio.2018.10.035
Lischke, R., Pozniak, J., Vondrys, D., and Elliott, M. J. (2011). Novel biodegradable stents in the treatment of bronchial stenosis after lung transplantation. Eur. J. cardio-thoracic Surg. 40 (3), 619–624. doi:10.1016/j.ejcts.2010.12.047
Little, U., Buchanan, F., Harkin-Jones, E., McCaigue, M., Farrar, D., and Dickson, G. (2009). Accelerated degradation behaviour of poly (ɛ-caprolactone) via melt blending with poly (aspartic acid-co-lactide)(PAL). Polym. Degrad. Stab. 94 (2), 213–220. doi:10.1016/j.polymdegradstab.2008.11.001
Liu, G., Fu, M., Li, F., Fu, W., Zhao, Z., Xia, H., et al. (2020). Tissue-engineered PLLA/gelatine nanofibrous scaffold promoting the phenotypic expression of epithelial and smooth muscle cells for urethral reconstruction. Mater. Sci. Eng. C 111, 110810. doi:10.1016/j.msec.2020.110810
Liu, J., Shang, L., Liu, J., and Qin, C. (2016). A novel biodegradable esophageal stent: results from mechanical and animal experiments. Am. J. Transl. Res. 8 (2), 1108–1114.
Liu, J., Wang, Z., Wu, K., Li, J., Chen, W., Shen, Y., et al. (2015). Paclitaxel or 5-fluorouracil/esophageal stent combinations as a novel approach for the treatment of esophageal cancer. Biomaterials 53, 592–599. doi:10.1016/j.biomaterials.2015.03.009
Liu, J., and Xi, T. (2016). Enhanced anti-corrosion ability and biocompatibility of PLGA coatings on MgZnYNd alloy by BTSE-APTES pre-treatment for cardiovascular stent. J. Mater. Sci. Technol. 32 (9), 845–857. doi:10.1016/j.jmst.2016.06.021
Liu, J.-B., Machado, P., Eisenbrey, J. R., Gummadi, S., Forsberg, F., Wessner, C. E., et al. (2023). Identification of sentinel lymph nodes in esophageal cancer patients using contrast-enhanced EUS with peritumoral injections. Endosc. Ultrasound 12 (4), 362–368. doi:10.1097/eus.0000000000000001
Liu, L., Feng, X., Pei, Y., Wang, J., Ding, J., and Chen, L. (2018). α-Cyclodextrin concentration-controlled thermo-sensitive supramolecular hydrogels. Mater. Sci. Eng. C 82, 25–28. doi:10.1016/j.msec.2017.08.045
Liu, L.-L., Qin, J., Zeng, C.-H., Du, R.-J., Pan, T., Ji, J.-J., et al. (2022). Biodegradable PTX-PLGA-coated magnesium stent for benign esophageal stricture: an experimental study. Acta Biomater. 146, 495–505. doi:10.1016/j.actbio.2022.04.038
Lopez-Tobaruela, J. M., Valverde-Lopez, F., de Hierro-Ruiz, M. L., and Redondo-Cerezo, E. (2021). Hepatic abscess after biodegradable esophageal stent placement: a rare complication. Official J. Am. Coll. Gastroenterology| ACG 116 (1), 222–223. doi:10.14309/ajg.0000000000000821
Łukasiewicz, S., Mikołajczyk, A., Błasiak, E., Fic, E., and Dziedzicka-Wasylewska, M. (2021). Polycaprolactone nanoparticles as promising candidates for nanocarriers in novel nanomedicines. Pharmaceutics 13 (2), 191. doi:10.3390/pharmaceutics13020191
Ma, X., Oyamada, S., Wu, T., Robich, M. P., Wu, H., Wang, X., et al. (2011). In vitro and in vivo degradation of poly (d, l-lactide-co-glycolide)/amorphous calcium phosphate copolymer coated on metal stents. J. Biomed. Mater. Res. Part A 96 (4), 632–638. doi:10.1002/jbm.a.33016
Maishman, T., Sheikh, H., Boger, P., Kelly, J., Cozens, K., Bateman, A., et al. (2021). A phase II study of biodegradable stents plus palliative radiotherapy in oesophageal cancer. Clin. Oncol. 33 (5), e225–e231. doi:10.1016/j.clon.2020.12.010
Mandal, A., Clegg, J. R., Anselmo, A. C., and Mitragotri, S. (2020). Hydrogels in the clinic. Bioeng. Transl. Med. 5 (2), e10158. doi:10.1002/btm2.10158
Manner, H. (2008). Argon plasma coagulation therapy. Curr. Opin. gastroenterology 24 (5), 612–616. doi:10.1097/mog.0b013e32830bf825
Matsunami, Y., Itoi, T., Tsuchiya, T., Ishii, K., Tanaka, R., Tonozuka, R., et al. (2023). Objective evaluation of the resistance forces of 22-gauge EUS-FNA and fine-needle biopsy needles. Endosc. Ultrasound 12 (2), 251. doi:10.4103/eus-d-22-00059
McCain, S., McCain, S., Quinn, B., Gray, R., Morton, J., and Rice, P. (2016). The role of biodegradable stents in the management of benign and malignant oesophageal strictures: a cohort study. Surg. 14 (6), 322–326. doi:10.1016/j.surge.2015.01.002
Mochizuki, Y., Saito, Y., Tanaka, T., Nitta, N., Yamada, H., Tsujikawa, T., et al. (2012). Endoscopic submucosal dissection combined with the placement of biodegradable stents for recurrent esophageal cancer after chemoradiotherapy. J. Gastrointest. cancer 43, 324–328. doi:10.1007/s12029-011-9283-z
Mohajeri, S., Chen, F., de Prinse, M., Phung, T., Burke-Kleinman, J., Maurice, D. H., et al. (2020). Liquid degradable poly (trimethylene-carbonate-co-5-hydroxy-trimethylene carbonate): an injectable drug delivery vehicle for acid-sensitive drugs. Mol. Pharm. 17 (4), 1363–1376. doi:10.1021/acs.molpharmaceut.0c00064
Mohamed, R. M., and Yusoh, K. (2016). A review on the recent research of polycaprolactone (PCL). Adv. Mater. Res. 1134, 249–255. doi:10.4028/www.scientific.net/amr.1134.249
Moravej, M., and Mantovani, D. (2011). Biodegradable metals for cardiovascular stent application: interests and new opportunities. Int. J. Mol. Sci. 12 (7), 4250–4270. doi:10.3390/ijms12074250
Mueller, W.-D., Nascimento, M. L., and De Mele, M. F. L. (2010). Critical discussion of the results from different corrosion studies of Mg and Mg alloys for biomaterial applications. Acta biomater. 6 (5), 1749–1755. doi:10.1016/j.actbio.2009.12.048
Muñoz, D. S., Ortiz–Moyano, C., and Gómez–Rodríguez, B. (2013). Resolution of a refractory anastomotic stricture with a novel biodegradable esophageal stent. Clin. Gastroenterology Hepatology 11 (9), e63. doi:10.1016/j.cgh.2012.11.023
Neves, S. C., Moroni, L., Barrias, C. C., and Granja, P. L. (2020). Leveling up hydrogels: hybrid systems in tissue engineering. Trends Biotechnol. 38 (3), 292–315. doi:10.1016/j.tibtech.2019.09.004
Okata, Y., Hisamatsu, C., Bitoh, Y., Yokoi, A., Nishijima, E., Maeda, K., et al. (2014). Efficacy and histopathological esophageal wall damage of biodegradable esophageal stents for treatment of severe refractory esophageal anastomotic stricture in a child with long gap esophageal atresia. Clin. J. gastroenterology 7, 496–501. doi:10.1007/s12328-014-0537-8
Orive-Calzada, A., Alvarez-Rubio, M., Romero-Izquierdo, S., Martin, M. C., Juanmartiñena, J., Ogueta-Fernández, M., et al. (2009). Severe epithelial hyperplasia as a complication of a novel biodegradable stent. Endoscopy 41 (S 02), E137–E138. doi:10.1055/s-0029-1214634
Oyama, T., Tomori, A., Hotta, K., Morita, S., Kominato, K., Tanaka, M., et al. (2005). Endoscopic submucosal dissection of early esophageal cancer. Clin. Gastroenterology Hepatology 3 (7), S67–S70. doi:10.1016/s1542-3565(05)00291-0
Pan, K., Li, X., Meng, L., Hong, L., Wei, W., and Liu, X. (2020). Photo-cross-linked polycarbonate coating with surface-erosion behavior for corrosion resistance and cytocompatibility enhancement of magnesium alloy. ACS Appl. Bio Mater. 3 (7), 4427–4435. doi:10.1021/acsabm.0c00411
Pan, K., Li, X., Shi, H., Dai, M., Yang, Z., Chen, M., et al. (2022). Preparation of photo-crosslinked aliphatic polycarbonate coatings with predictable degradation behavior on magnesium-alloy stents by electrophoretic deposition. Chem. Eng. J. 427, 131596. doi:10.1016/j.cej.2021.131596
Pauli, E. M., Schomisch, S. J., Furlan, J. P., Marks, A. S., Chak, A., Lash, R. H., et al. (2012). Biodegradable esophageal stent placement does not prevent high-grade stricture formation after circumferential mucosal resection in a porcine model. Surg. Endosc. 26, 3500–3508. doi:10.1007/s00464-012-2373-6
Peng, L., Wan, R., Chen, S., Wu, J., Yang, J., Wang, X., et al. (2023). Efficacy of endoscopic anterior fundoplication with a novel ultrasonic surgical endostapler for gastroesophageal reflux disease: six-month results from a multicenter prospective trial. Endosc. ultrasound 12 (1), 128. doi:10.4103/eus-d-21-00244
Perkins, J., Xu, Z., Smith, C., Roy, A., Kumta, P. N., Waterman, J., et al. (2015). Direct writing of polymeric coatings on magnesium alloy for tracheal stent applications. Ann. Biomed. Eng. 43, 1158–1165. doi:10.1007/s10439-014-1169-3
Pruksawan, S., Chee, H. L., Wang, Z., Luo, P., Chong, Y. T., Thitsartarn, W., et al. (2022). Toughened hydrogels for 3D printing of soft auxetic structures. Chemistry–An Asian J. 17 (19), e202200677. doi:10.1002/asia.202200677
Rabenstein, T. (2015). Palliative endoscopic therapy of esophageal cancer. Visc. Med. 31 (5), 354–359. doi:10.1159/000441175
Ragunath, K. (2008). Refractory benign esophageal strictures: extending the role of expandable stents. Official J. Am. Coll. Gastroenterology 12, 2995–2996. doi:10.1111/j.1572-0241.2008.02178.x
Rai, A., Senapati, S., Saraf, S. K., and Maiti, P. (2016). Biodegradable poly (ε-caprolactone) as a controlled drug delivery vehicle of vancomycin for the treatment of MRSA infection. J. Mater. Chem. B 4 (30), 5151–5160. doi:10.1039/c6tb01623e
Raman, R., Hua, T., Gwynne, D., Collins, J., Tamang, S., Zhou, J., et al. (2020). Light-degradable hydrogels as dynamic triggers for gastrointestinal applications. Sci. Adv. 6 (3), eaay0065. doi:10.1126/sciadv.aay0065
Rejchrt, S., Kopacova, M., Brozik, J., and Bures, J. (2011). Biodegradable stents for the treatment of benign stenoses of the small and large intestines. Endoscopy 43, 911–917. doi:10.1055/s-0030-1256405
Repici, A., Vleggaar, F. P., Hassan, C., van Boeckel, P. G., Romeo, F., Pagano, N., et al. (2010). Efficacy and safety of biodegradable stents for refractory benign esophageal strictures: the BEST (Biodegradable Esophageal Stent) study. Gastrointest. Endosc. 72 (5), 927–934. doi:10.1016/j.gie.2010.07.031
Rozanes, İ., Poyanlı, A., and Acunaş, B. (2002). Palliative treatment of inoperable malignant esophageal strictures with metal stents: one center's experience with four different stents. Eur. J. radiology 43 (3), 196–203. doi:10.1016/s0720-048x(02)00154-7
Saito, Y., Minami, K.-i., Kaneda, H., Okada, T., Maniwa, T., Araki, Y., et al. (2004). New tubular bioabsorbable knitted airway stent: feasibility assessment for delivery and deployment in a dog model. Ann. Thorac. Surg. 78 (4), 1438–1440. doi:10.1016/s0003-4975(03)01408-5
Saito, Y., Tanaka, T., Andoh, A., Minematsu, H., Hata, K., Tsujikawa, T., et al. (2007). Usefulness of biodegradable stents constructed of poly-l-lactic acid monofilaments in patients with benign esophageal stenosis. World J. Gastroenterology WJG 13 (29), 3977. doi:10.3748/wjg.v13.i29.3977
Saito, Y., Tanaka, T., Andoh, A., Minematsu, H., Hata, K., Tsujikawa, T., et al. (2008). Novel biodegradable stents for benign esophageal strictures following endoscopic submucosal dissection. Dig. Dis. Sci. 53, 330–333. doi:10.1007/s10620-007-9873-6
Saska, S., Pilatti, L., Silva, E. S. d. S., Nagasawa, M. A., Câmara, D., Lizier, N., et al. (2021). Polydioxanone-based membranes for bone regeneration. Polymers 13 (11), 1685. doi:10.3390/polym13111685
Sehgal, V., and Sami, S. (2021). Management of chronic refractory oesophageal strictures. Curr. Treat. Options Gastroenterology 19 (3), 443–458. doi:10.1007/s11938-021-00352-z
Shaikh, M., Choudhury, N. R., Knott, R., and Garg, S. (2015). Engineering stent based delivery system for esophageal cancer using docetaxel. Mol. Pharm. 12 (7), 2305–2317. doi:10.1021/mp500851u
Shakya, A. K., Al-Sulaibi, M., Naik, R. R., Nsairat, H., Suboh, S., and Abulaila, A. (2023). Review on PLGA polymer based nanoparticles with antimicrobial properties and their application in various medical conditions or infections. Polymers 15 (17), 3597. doi:10.3390/polym15173597
Shuai, C., Yang, W., Feng, P., Peng, S., and Pan, H. (2021). Accelerated degradation of HAP/PLLA bone scaffold by PGA blending facilitates bioactivity and osteoconductivity. Bioact. Mater. 6 (2), 490–502. doi:10.1016/j.bioactmat.2020.09.001
Shuai, C., Zan, J., Qi, F., Wang, G., Liu, Z., Yang, Y., et al. (2019). nMgO-incorporated PLLA bone scaffolds: enhanced crystallinity and neutralized acidic products. Mater. Des. 174, 107801. doi:10.1016/j.matdes.2019.107801
Siersema, P. D. (2008). Treatment options for esophageal strictures. Nat. Clin. Pract. Gastroenterology Hepatology 5 (3), 142–152. doi:10.1038/ncpgasthep1053
Siersema, P. D. (2019). How to approach a patient with refractory or recurrent benign esophageal stricture. Gastroenterology 156 (1), 7–10. doi:10.1053/j.gastro.2018.11.040
Siiki, A., Rinta-Kiikka, I., Sand, J., and Laukkarinen, J. (2017). Endoscopic biodegradable biliary stents in the treatment of benign biliary strictures: first report of clinical use in patients. Dig. Endosc. 29 (1), 118–121. doi:10.1111/den.12709
Sinha, V., Bansal, K., Kaushik, R., Kumria, R., and Trehan, A. (2004). Poly-ϵ-caprolactone microspheres and nanospheres: an overview. Int. J. Pharm. 278 (1), 1–23. doi:10.1016/j.ijpharm.2004.01.044
Smyth, E. C., Lagergren, J., Fitzgerald, R. C., Lordick, F., Shah, M. A., Lagergren, P., et al. (2017). Oesophageal cancer. Nat. Rev. Dis. Prim. 3 (1), 17048–17121. doi:10.1038/nrdp.2017.48
Song, G., Zhao, H. Q., Liu, Q., and Fan, Z. (2022). A review on biodegradable biliary stents: materials and future trends. Bioact. Mater. 17, 488–495. doi:10.1016/j.bioactmat.2022.01.017
Song, H.-Y., Park, S.-I., Do, Y.-S., Yoon, H. K., Sung, K.-B., Sohn, K.-H., et al. (1997). Expandable metallic stent placement in patients with benign esophageal strictures: results of long-term follow-up. Radiology 203 (1), 131–136. doi:10.1148/radiology.203.1.9122381
Stevanović, M., Maksin, T., Petković, J., Filipič, M., and Uskoković, D. (2009). An innovative, quick and convenient labeling method for the investigation of pharmacological behavior and the metabolism of poly (DL-lactide-co-glycolide) nanospheres. Nanotechnology 20 (33), 335102. doi:10.1088/0957-4484/20/33/335102
Stivaros, S., Williams, L., Senger, C., Wilbraham, L., and Laasch, H.-U. (2010). Woven polydioxanone biodegradable stents: a new treatment option for benign and malignant oesophageal strictures. Eur. Radiol. 20, 1069–1072. doi:10.1007/s00330-009-1662-5
Tamai, H., Igaki, K., Kyo, E., Kosuga, K., Kawashima, A., Matsui, S., et al. (2000). Initial and 6-month results of biodegradable poly-l-lactic acid coronary stents in humans. Circulation 102 (4), 399–404. doi:10.1161/01.cir.102.4.399
Tanaka, T., Takahashi, M., Nitta, N., Furukawa, A., Andoh, A., Saito, Y., et al. (2007). Newly developed biodegradable stents for benign gastrointestinal tract stenoses: a preliminary clinical trial. Digestion 74 (3-4), 199–205. doi:10.1159/000100504
Tang, H., Li, S., Zhao, Y., Liu, C., Gu, X., and Fan, Y. (2022). A surface-eroding poly (1, 3-trimethylene carbonate) coating for magnesium based cardiovascular stents with stable drug release and improved corrosion resistance. Bioact. Mater. 7, 144–153. doi:10.1016/j.bioactmat.2021.05.045
Thakur, M., Majid, I., Hussain, S., and Nanda, V. (2021). Poly (ε-caprolactone): a potential polymer for biodegradable food packaging applications. Packag. Technol. Sci. 34 (8), 449–461. doi:10.1002/pts.2572
Unal, A. Z., and West, J. L. (2020). Synthetic ECM: bioactive synthetic hydrogels for 3D tissue engineering. Bioconjugate Chem. 31 (10), 2253–2271. doi:10.1021/acs.bioconjchem.0c00270
Ustürk, S., Altundag, E. M., and Yilmaz, E. (2022). Pullulan/polyHEMA cryogels: synthesis, physicochemical properties, and cell viability. J. Appl. Polym. Sci. 139 (12), 51822. doi:10.1002/app.51822
van Boeckel, P. G., Vleggaar, F. P., and Siersema, P. D. (2011). A comparison of temporary self-expanding plastic and biodegradable stents for refractory benign esophageal strictures. Clin. Gastroenterology Hepatology 9 (8), 653–659. doi:10.1016/j.cgh.2011.04.006
van den Berg, M. W., Walter, D., de Vries, E. M., Vleggaar, F. P., van Berge Henegouwen, M. I., van Hillegersberg, R., et al. (2014). Biodegradable stent placement before neoadjuvant chemoradiotherapy as a bridge to surgery in patients with locally advanced esophageal cancer. Gastrointest. Endosc. 80 (5), 908–913. doi:10.1016/j.gie.2014.06.004
Vandenplas, Y., Hauser, B., Devreker, T., Urbain, D., and Reynaert, H. (2009). A biodegradable esophageal stent in the treatment of a corrosive esophageal stenosis in a child. J. Pediatr. gastroenterology Nutr. 49 (2), 254–257. doi:10.1097/mpg.0b013e31819de871
van Hooft, J. E., van Berge Henegouwen, M. I., Rauws, E. A., Bergman, J. J., Busch, O. R., and Fockens, P. (2011). Endoscopic treatment of benign anastomotic esophagogastric strictures with a biodegradable stent. Gastrointest. Endosc. 73 (5), 1043–1047. doi:10.1016/j.gie.2011.01.001
Verschuur, E. M., Repici, A., Kuipers, E. J., Steyerberg, E. W., and Siersema, P. D. (2008). New design esophageal stents for the palliation of dysphagia from esophageal or gastric cardia cancer: a randomized trial. Official J. Am. Coll. Gastroenterology| ACG 103 (2), 304–312. doi:10.1111/j.1572-0241.2007.01542.x
Walter, D., Van Den Berg, M. W., Hirdes, M. M., Vleggaar, F. P., Repici, A., Deprez, P. H., et al. (2018). Dilation or biodegradable stent placement for recurrent benign esophageal strictures: a randomized controlled trial. Endoscopy 50 (12), 1146–1155. doi:10.1055/a-0602-4169
Wang, J., He, Y., Maitz, M. F., Collins, B., Xiong, K., Guo, L., et al. (2013). A surface-eroding poly (1, 3-trimethylene carbonate) coating for fully biodegradable magnesium-based stent applications: toward better biofunction, biodegradation and biocompatibility. Acta biomater. 9 (10), 8678–8689. doi:10.1016/j.actbio.2013.02.041
Wang, L., Jiao, L., Pang, S., Yan, P., Wang, X., and Qiu, T. (2021a). The development of design and manufacture techniques for bioresorbable coronary artery stents. Micromachines 12 (8), 990. doi:10.3390/mi12080990
Wang, S., Zhang, X., Li, J., Liu, C., and Guan, S. (2020). Investigation of Mg–Zn–Y–Nd alloy for potential application of biodegradable esophageal stent material. Bioact. Mater. 5 (1), 1–8. doi:10.1016/j.bioactmat.2020.01.002
Wang, S., Zhu, S.-J., Zhang, X.-Q., Li, J.-A., and Guan, S.-K. (2019). Effects of degradation products of biomedical magnesium alloys on nitric oxide release from vascular endothelial cells. Med. Gas Res. 9 (3), 153. doi:10.4103/2045-9912.266991
Wang, T., Cui, H., Shao, Y., Jiang, H., Dong, Y., and Guo, R. (2021b). Esophageal stent types and clinical applications. Int. J. Clin. Exp. Med. 14 (7), 2054–2066.
Wang, X., Venkatraman, S. S., Boey, F. Y., Loo, J. S., and Tan, L. P. (2006). Controlled release of sirolimus from a multilayered PLGA stent matrix. Biomaterials 27 (32), 5588–5595. doi:10.1016/j.biomaterials.2006.07.016
Wang, Z., Liu, J., Wu, K., Shen, Y., Mao, A., Li, J., et al. (2015). Nitinol stents loaded with a high dose of antitumor 5-fluorouracil or paclitaxel: esophageal tissue responses in a porcine model. Gastrointest. Endosc. 82 (1), 153–160. doi:10.1016/j.gie.2015.02.034
Washington, K. E., Kularatne, R. N., Karmegam, V., Biewer, M. C., and Stefan, M. C. (2017). Recent advances in aliphatic polyesters for drug delivery applications. Wiley Interdiscip. Rev. Nanomedicine Nanobiotechnology 9 (4), e1446. doi:10.1002/wnan.1446
Wong, H. M., Yeung, K. W., Lam, K. O., Tam, V., Chu, P. K., Luk, K. D., et al. (2010). A biodegradable polymer-based coating to control the performance of magnesium alloy orthopaedic implants. Biomaterials 31 (8), 2084–2096. doi:10.1016/j.biomaterials.2009.11.111
Woodruff, M. A., and Hutmacher, D. W. (2010). The return of a forgotten polymer—polycaprolactone in the 21st century. Prog. Polym. Sci. 35 (10), 1217–1256. doi:10.1016/j.progpolymsci.2010.04.002
Wu, Q., Zhu, S., Wang, L., Liu, Q., Yue, G., Wang, J., et al. (2012). The microstructure and properties of cyclic extrusion compression treated Mg–Zn–Y–Nd alloy for vascular stent application. J. Mech. Behav. Biomed. Mater. 8, 1–7. doi:10.1016/j.jmbbm.2011.12.011
Wu, Y., Gao, X., Wu, J., Zhou, T., Nguyen, T. T., and Wang, Y. (2023). Biodegradable polylactic acid and its composites: characteristics, processing, and sustainable applications in sports. Polymers 15 (14), 3096. doi:10.3390/polym15143096
Xu, L., Yu, G., Zhang, E., Pan, F., and Yang, K. (2007). In vivo corrosion behavior of Mg-Mn-Zn alloy for bone implant application. J. Biomed. Mater. Res. 83 (3), 703–711. doi:10.1002/jbm.a.31273
Yang, K., Cao, J., Yuan, T.-W., Zhu, Y.-Q., Zhou, B., and Cheng, Y.-S. (2019). Silicone-covered biodegradable magnesium stent for treating benign esophageal stricture in a rabbit model. World J. Gastroenterology 25 (25), 3207–3217. doi:10.3748/wjg.v25.i25.3207
Yang, K., Ling, C., Yuan, T., Zhu, Y., Cheng, Y., and Cui, W. (2016). Polymeric biodegradable stent insertion in the esophagus. Polymers 8 (5), 158. doi:10.3390/polym8050158
Yang, L., Li, J., Meng, S., Jin, Y., Zhang, J., Li, M., et al. (2014). The in vitro and in vivo degradation behavior of poly (trimethylene carbonate-co-ε-caprolactone) implants. Polymer 55 (20), 5111–5124. doi:10.1016/j.polymer.2014.08.027
Yang, L., Li, J., Zhang, W., Jin, Y., Zhang, J., Liu, Y., et al. (2015). The degradation of poly (trimethylene carbonate) implants: the role of molecular weight and enzymes. Polym. Degrad. Stab. 122, 77–87. doi:10.1016/j.polymdegradstab.2015.10.016
Yano, T., Yoda, Y., Nomura, S., Toyosaki, K., Hasegawa, H., Ono, H., et al. (2017). Prospective trial of biodegradable stents for refractory benign esophageal strictures after curative treatment of esophageal cancer. Gastrointest. Endosc. 86 (3), 492–499. doi:10.1016/j.gie.2017.01.011
Yano, T., Yoda, Y., Nonaka, S., Abe, S., Kawata, N., Yoshio, T., et al. (2022). Pivotal trial of a biodegradable stent for patients with refractory benign esophageal stricture. Esophagus 19 (3), 516–524. doi:10.1007/s10388-022-00909-6
Yin, T., Du, R., Wang, Y., Huang, J., Ge, S., Huang, Y., et al. (2022). Two-stage degradation and novel functional endothelium characteristics of a 3-D printed bioresorbable scaffold. Bioact. Mater. 10, 378–396. doi:10.1016/j.bioactmat.2021.08.020
Yuan, T., Yu, J., Cao, J., Gao, F., Zhu, Y., Cheng, Y., et al. (2016). Fabrication of a delaying biodegradable magnesium alloy-based esophageal stent via coating elastic polymer. Materials 9 (5), 384. doi:10.3390/ma9050384
Yue, Y., Wang, L., Yang, N., Huang, J., Lei, L., Ye, H., et al. (2015). Effectiveness of biodegradable magnesium alloy stents in coronary artery and femoral artery. J. interventional Cardiol. 28 (4), 358–364. doi:10.1111/joic.12217
Yusoff, N. H., Pal, K., Narayanan, T., and de Souza, F. G. (2021). Recent trends on bioplastics synthesis and characterizations: polylactic acid (PLA) incorporated with tapioca starch for packaging applications. J. Mol. Struct. 1232, 129954. doi:10.1016/j.molstruc.2021.129954
Zein, N. N., Greseth, J. M., and Perrault, J. (1995). Endoscopic intralesional steroid injections in the management of refractory esophageal strictures. Gastrointest. Endosc. 41 (6), 596–598. doi:10.1016/s0016-5107(95)70198-2
Zhang, E., Yang, L., Xu, J., and Chen, H. (2010). Microstructure, mechanical properties and bio-corrosion properties of Mg–Si (–Ca, Zn) alloy for biomedical application. Acta biomater. 6 (5), 1756–1762. doi:10.1016/j.actbio.2009.11.024
Zhang, L., Zuo, X., Li, S., Sun, M., Xie, H., Zhang, K., et al. (2019). Synergistic therapy of magnetism-responsive hydrogel for soft tissue injuries. Bioact. Mater. 4, 160–166. doi:10.1016/j.bioactmat.2019.03.002
Zhang, W., Chen, Y., Chen, M., Zhao, S., Mao, J., Qu, A., et al. (2016). Strengthened corrosion control of poly (lactic acid)(PLA) and poly (ε-caprolactone)(PCL) polymer-coated magnesium by imbedded hydrophobic stearic acid (SA) thin layer. Corros. Sci. 112, 327–337. doi:10.1016/j.corsci.2016.07.027
Zhang, Y. S., and Khademhosseini, A. (2017). Advances in engineering hydrogels. Science 356 (6337), eaaf3627. doi:10.1126/science.aaf3627
Zhang, Z.-Q., Yang, Y.-X., Li, J.-A., Zeng, R.-C., and Guan, S.-K. (2021). Advances in coatings on magnesium alloys for cardiovascular stents–a review. Bioact. Mater. 6 (12), 4729–4757. doi:10.1016/j.bioactmat.2021.04.044
Zhao, J., Mo, Z., Guo, F., Shi, D., Han, Q. Q., and Liu, Q. (2018). Drug loaded nanoparticle coating on totally bioresorbable PLLA stents to prevent in-stent restenosis. J. Biomed. Mater. Res. Part B Appl. Biomaterials 106 (1), 88–95. doi:10.1002/jbm.b.33794
Zhou, L., Ke, K., Yang, M.-B., and Yang, W. (2021). Recent progress on chemical modification of cellulose for high mechanical-performance Poly (lactic acid)/Cellulose composite: a review. Compos. Commun. 23, 100548. doi:10.1016/j.coco.2020.100548
Zhu, X., and Braatz, R. D. (2015). A mechanistic model for drug release in PLGA biodegradable stent coatings coupled with polymer degradation and erosion. J. Biomed. Mater. Res. Part A 103 (7), 2269–2279. doi:10.1002/jbm.a.35357
Zhu, Y., Cui, W., Cheng, Y., Chang, J., Chen, N., and Yan, L. (2013a). Evaluation of biodegradable paclitaxel-eluting nanofibre-covered metal stents for the treatment of benign cardia stricture in an experimental model. J. Br. Surg. 100 (6), 784–793. doi:10.1002/bjs.9106
Zhu, Y., Hu, C., Li, B., Yang, H., Cheng, Y., and Cui, W. (2013b). A highly flexible paclitaxel-loaded poly (ε-caprolactone) electrospun fibrous-membrane-covered stent for benign cardia stricture. Acta biomater. 9 (9), 8328–8336. doi:10.1016/j.actbio.2013.06.004
Zhu, Y.-Q., Edmonds, L., Wei, L.-M., Zheng, R.-L., Cheng, R.-Y., Cui, W.-G., et al. (2017). Technical feasibility and tissue reaction after silicone-covered biodegradable magnesium stent insertion in the oesophagus: a primary study in vitro and in vivo. Eur. Radiol. 27, 2546–2553. doi:10.1007/s00330-016-4602-1
Keywords: biodegradable esophageal stents, esophageal stricture, esophageal cancer, polydioxanone, poly (L-lactic-acid), magnesium alloy, drug-eluting stents
Citation: Yang Y, Yang Y, Hou Z, Wang T, Wu P, Shen L, Li P, Zhang K, Yang L and Sun S (2023) Comprehensive review of materials, applications, and future innovations in biodegradable esophageal stents. Front. Bioeng. Biotechnol. 11:1327517. doi: 10.3389/fbioe.2023.1327517
Received: 25 October 2023; Accepted: 27 November 2023;
Published: 06 December 2023.
Edited by:
Lin Yu, Fudan University, ChinaReviewed by:
Daokun Shi, MicroPort Medical Science Co., Ltd., ChinaBhupendra Gopalbhai Prajapati, Ganpat University, India
Copyright © 2023 Yang, Yang, Hou, Wang, Wu, Shen, Li, Zhang, Yang and Sun. This is an open-access article distributed under the terms of the Creative Commons Attribution License (CC BY). The use, distribution or reproduction in other forums is permitted, provided the original author(s) and the copyright owner(s) are credited and that the original publication in this journal is cited, in accordance with accepted academic practice. No use, distribution or reproduction is permitted which does not comply with these terms.
*Correspondence: Kai Zhang, zhangkaidoctor@163.com; Liqun Yang, yangliqun@cmu.edu.cn, yanglq@lnszjk.com.cn; Siyu Sun, sun-siyu@163.com
†These authors have contributed equally to this work and share first authorship