- 1School and Hospital of Stomatology, China Medical University, Liaoning Provincial Key Laboratory of Oral Diseases, Shenyang, China
- 2School of Mechanical Engineering and Automation, Northeastern University, Shenyang, China
Titanium (Ti) and its alloys have good biocompatibility, mechanical properties and corrosion resistance, making them attractive for biomedical applications. However, their biological inertness and lack of antimicrobial properties may compromise the success of implants. In this review, the potential of micro-arc oxidation (MAO) technology to create bioactive coatings on Ti implants is discussed. The review covers the following aspects: 1) different factors, such as electrolyte, voltage and current, affect the properties of MAO coatings; 2) MAO coatings affect biocompatibility, including cytocompatibility, hemocompatibility, angiogenic activity, corrosion resistance, osteogenic activity and osseointegration; 3) antibacterial properties can be achieved by adding copper (Cu), silver (Ag), zinc (Zn) and other elements to achieve antimicrobial properties; and 4) MAO can be combined with other physical and chemical techniques to enhance the performance of MAO coatings. It is concluded that MAO coatings offer new opportunities for improving the use of Ti and its alloys in biomedical applications, and some suggestions for future research are provided.
1 Introduction
Metal implants have been a staple in biomedical applications since the onset of the 19th century, with Ti and its alloys becoming the prime choice for medical implant materials with high biocompatibility, excellent corrosion resistance, high specific strength and the best osteogenic potential (Kaur and Singh, 2019). Since the mid-twentieth century, due to their mechanical and chemical properties, titanium and its alloys have been widely used in industrial and biomedical applications, particularly in dentistry and orthopaedics such as dental implants, bone fusion, bone fixation and arthroplasty (joint replacement surgery) (Anderson et al., 2021; Hoque et al., 2022; Lim et al., 2022).
Although titanium and its alloys have been extensively used, they are inert metals that do not effectively promote the proliferation of osteoblasts and osteocytes, which can lead to implant failure. In addition, titanium-based implants still face some clinical problems, such as slow biological reactions to the material surface, slow early bone integration, and implantable diseases caused by bacterial colonization, leading to implant loosening and survival ability loss (Zhang et al., 2020b). Surface morphology, composition, hydrophilicity and roughness are key factors of implant tissue interaction and osseointegration (Le Guéhennec et al., 2007). The bioactive calcium phosphate (Ca-P)-containing coatings on titanium are similar to biomimetic implant materials used for bone tissue. Therefore, we can focus on the development of Ca-P-based surface coatings on titanium-based materials for load-bearing implant applications. Hence, typical coating methodologies have emerged in recent years. The methodologies can be divided into three categories: mechanical methods (fine machining, grinding, tumbling, and sandblasting), physical methods [physical vapour deposition (PVD), thermal spraying, and ion implantation] and chemical methods [pickling, chemical vapour deposition (CVD), anodic oxidation, sol-gel, and micro-arc oxidation (MAO)] (Shen et al., 2019; Gabor et al., 2020). These methods can improve the properties of titanium and its alloys, such as their better wear and corrosion resistance, providing strong mechanical adhesion between bone and implant, enhancing osteo-induction and osteo-conduction, improving bioactivity and biocompatibility, and accelerating healing time at the implant site (Sasikumar et al., 2019; Xue et al., 2020). At the same time, they have different advantages and disadvantages (as shown in Table 1).
MAO [plasma electrolytic oxidation (PEO), or anodic spark deposition (ASD)] has attracted substantial attention as an emerging surface coating methodology. MAO uses an arc discharge to enhance and activate the reactions occurring on an anode to form a ceramic film on the surface of a metal through the interaction of the workpiece and electrolyte (Wang Y. et al., 2015; Kaseem and Ko, 2019b; Kaseem and Ko, 2019a; Zhang R. et al., 2021; Xia et al., 2021). This approach can incorporate bioactive electrolyte components such as Ca and P into the coating. Ca-P coatings, particularly HA coatings, can facilitate the process of early and rapid osseointegration. The bioactivity of the coating is determined by its physicochemical characteristics, including roughness, porosity, phase, elemental composition, and adhesive strength (Wang et al., 2022).
In addition, the process of MAO can be regulated by multiple factors. On the one hand, a porous bioactive Ca-P-based composite layer can be deposited on Ti-based implant surfaces according to the selected electrolyte, which would enhance the biocompatibility and bonding strength of the coated layer (Zhao et al., 2007; Sun et al., 2008). On the other hand, antibacterial metal elements can be incorporated into implant surfaces to inhibit the initial adhesion of bacteria and prevent postsurgical complications, thus enhancing the antibacterial properties (Ferraris and Spriano, 2016). Furthermore, the contents of bioactive elements and antibacterial metal elements on the MAO coating surface can be tuned by controlling the voltage, electrolyte components and MAO time.
This review aims to collect and compare recent scientific papers concerning Ti and its alloys modified by MAO technology in the biomedical field. This review is focused on several aspects, including the influences of different factors on the performance of MAO coatings, the biocompatibility of MAO coatings and the combination of MAO with other physical or chemical techniques. In addition, implant-associated infection remains one of the most devastating postoperative complications (Oh-Hyun et al., 2018). It is highly desirable to introduce antimicrobial agents into implant surfaces to provide antibacterial activities and prevent peri-implant infections. Due to their perfect stabilities, superior broad-spectrum antibacterial properties and relatively low toxicity levels, inorganic antibacterial metal elements [e.g., silver (Ag), copper (Cu) and zinc (Zn)] have attracted great attention, which was elaborated in detail in our review. Finally, we highlight the potential challenges and future applications of MAO-modified titanium and its alloys in the biomedical field.
2 MAO process parameters for Ti and its alloys
Although more research is needed to fully understand the mechanisms at play, experts have identified three key components of the MAO process: electrochemical oxidation, plasma chemistry, and thermal diffusion in the electrolyte (Hussein et al., 2013). During this process, Ti and its alloys are submerged in an electrolyte containing modified species in the form of dissolved salts, such as silicates (Si), phosphates(P) and Ca salts (Sarbishei et al., 2014). The Ti and its alloys are used as the anode, while stainless steel plates serve as the cathode in the electrolytic bath (as depicted in Figure 1) Typically, MAO treatments last between 5 and 180 min, with current densities ranging from 500 to 2,000 A-m-2 and voltages reaching up to 1,000 V (Snizhko et al., 2004).
As the metal comes into contact with the electrolyte, a protective film gradually forms. As voltage increases, a porous oxide coating emerges under conditions of dielectric breakdown. When voltage surpasses the dielectric breakdown of the oxide coating, spark discharges occur, forming larger pores and an interconnected microstructure. The cyclic formation and breakdown of the oxide coating cause potential fluctuations, allowing for the formation of a ceramic oxide coating through material dissolution and electrolyte gasification. (Figure 2).
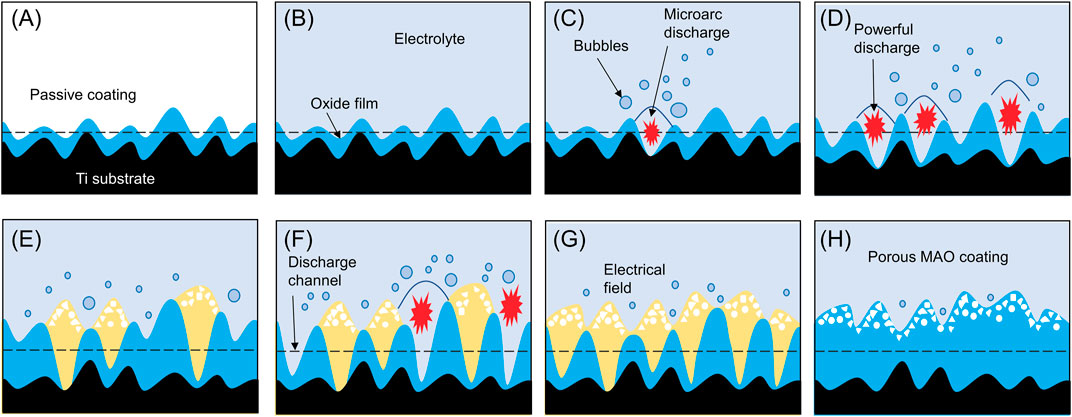
FIGURE 2. Schematic of the formation process of MAO porous coating. When exposed to air, there will be a layer of passive coating (A) on the surface of titanium metal. When the metal is exposed to the electrolyte, a protective film (B) will be formed. As the voltage increases, a porous oxide coating (C,D) is formed under dielectric breakdown conditions. When the voltage exceeds the dielectric breakdown of the oxide coating, spark discharge will occur, forming larger pores and interconnected microstructure (E,F). The cyclic formation and decomposition of oxide coatings cause potential fluctuations (G), thereby allowing the formation of ceramic oxide coatings by material dissolution and electrolyte gasification (H).
In the case of Ti and its alloys, the formation of the coating during MAO is influenced by various factors, including electrolyte composition, voltage, current density, frequency, reaction time and duty cycle (Chen et al., 2019; Li Z. et al., 2020; Zuo et al., 2020; Huang C. et al., 2021; Yong et al., 2021; Wu and Jiang, 2023). The coating properties obtained after MAO may vary depending on the influence of different parameters. Parameter control aims to obtain a homogeneous, stable, viscous and biologically active surface.
2.1 Electrolyte composition
In the MAO process, the choice of electrolyte is of critical significance. The electrolyte not only determines the environment in which MAO occurs but also determines the elemental composition of the final ceramic coating. The electrolyte is important because during the MAO process, the anions in the electrolyte are driven by high voltages, and they strongly bombard the titanium surface, causing them to melt and be deposited in the ceramic coating. Therefore, the chemical composition of the MAO coating depends on the anionic species in the chosen electrolyte. Changing the chemical composition of the electrolyte changes the chemical bonds between the ceramic and titanium, forming the primary bonding force in titanium-ceramic restorations. The electrolyte composition used is established by mixing silicon, calcium, Zn, manganese(Mg) and magnesium particles into the following electrolytes: KOH, Na2SiO3, NaF, Na2P4O7, and NaAlO2 (Pesode and Barve, 2021). To date, the most commonly used electrolytes in MAO technology are Si, P and aluminate-based electrolytes (Walsh et al., 2009; Khorasanian et al., 2011; Shokouhfar et al., 2012). An important factor in the composition of the electrolyte that affects the structure, morphology, and corrosion resistance of MAO coatings on Ti and its alloys is the composition of the electrolyte used (Venkateswarlu et al., 2012; Venkateswarlu et al., 2013; Jiang et al., 2016; Fattah-Alhosseini et al., 2018; Sousa et al., 2021).
Generally, adding silicates to the electrolyte results in thick coatings, rough surfaces and low adhesion. MAO oxide films formed on commercially pure Ti with electrolyte solutions containing silicates have round holes of multiple sizes, whereas P solutions produce mainly round and crater-shaped holes (Shokouhfar et al., 2011; Shokouhfar et al., 2012). In addition, various sodium-based additives in the working electrolyte can significantly affect the corrosion resistance of MAO-modified pure Ti, in terms of microstructure and phase composition (Molaei et al., 2019). Additives such as K2ZrF6 and K2TiF6 in silicate electrolytes can improve the sealed microstructure, increase the hardness and improve the corrosion resistance (Yang et al., 2018). Additionally, wear-resistant ceramic coatings have been generated on novel commercially pure titanium grade 4+ alloys by MAO in an aluminate- and zirconia-containing electrolyte, increasing the hardness and wear resistance of the MAO coatings (Lederer et al., 2021). The research conducted by Xiaohui Yuan et al. shows that using Na2SiO3 or MgSiF6 electrolytes in MAO treatment significantly enhances the bonding strength of titanium-ceramic interfaces. Na2SiO3 and MgSiF6 groups exhibited uniformly porous MAO coatings, reducing oxygen diffusion and cracks at the titanium-ceramic interface (Yuan et al., 2017).
Phosphate-based electrolytes have low roughness and coating thickness but excellent adhesion. It has been shown that coatings obtained from Ca-P electrolytes exhibit higher surface thickness and corrosion resistance values than coatings obtained from silicate electrolytes (Venkateshwarlu et al., 2012). MAO coatings on valve metals are normally white in appearance, however, colourants, which are normally the salts of transition metal ions, can be used to prepare coloured coatings. Research has shown that MAO treatment can be performed on Ti-6Al-4V alloy in concentrated sodium silicate electrolyte. By controlling the oxidation time, black and white TiO2 coatings with silicon dioxide bases are obtained. The short treatment time results in a black coating with excellent corrosion resistance, which is attributed to the presence of Ti2+ and Ti3+ in the coating. The white coating exhibits good surface roughness and super hydrophilicity. The bond strengths of the black and white coatings on Ti-6Al-4V alloy are approximately 14.4 and 4.3 MPa, respectively (Han et al., 2018). It has been found that MAO treatment of Ti-6Al-4V in a phosphate electrolyte may produce a yellowish colour, while when vanadate is added to the coating, the colour of the coating may change from yellow to brown (Jiang et al., 2016).
A.L. Yerokhin compared the processing and performance of oxide films formed on a Ti-6Al-4V alloy by MAO in aqueous solutions containing aluminate, phosphate, silicate and sulphate anions and certain combinations of these materials. The film produced by the aluminate-phosphate electrolyte is dense and uniform, which was thicker than that produced by the phosphate electrolyte, but both showed good corrosion behaviour. Thicker SiO2/TiO2-based films with high bulk porosity produced from silicate and silicate-aluminate electrolytes exhibit good corrosion behaviour in H2SO4 solutions with high chemical stability (Yerokhin et al., 2000).
Hardness is a basic mechanical property of materials. In Gaoqiang Xu’s research, it was found that the hardness of native titanium is low, 265 HV, but the MAO coating on titanium causes a significant increase in the hardness of titanium. It can make the hardness of natural titanium reach 342 HV. After incorporating SiO2 nanoparticles, the hardness of MAO-Si increases to 467 HV. The authors concluded that the highest hardness value of MAO-Si can be attributed to the presence of hard SiO2 nanoparticles and fewer micropores on the surface of MAO-Si (Xu and Shen, 2019).
2.2 Voltage
Voltage variation is another key factor affecting the performance parameters of MAO coatings on Ti and its alloys. Studies have shown that different choices of voltage affect the porosity, pore size, elemental content of the coating, high-temperature resistance, surface morphology, contact angle and surface free energy of the coating (Sedelnikova et al., 2017; Du et al., 2018; Komarova et al., 2020b; Yu et al., 2020). Qing Du’s experimental results show SEM (scanning electron microscopy) images of pure Ti and MAO coatings formed at 300, 350, 400, 450 and 500 V. It was confirmed that a rough and porous surface structure was observed on the MAO-treated Ti plate. A large number of micropores with a size of approximately 1∼3 μm were observed on the MAO coating formed at 300 V. However, as the applied voltage (350∼500 V) increases, the average micropore size on the MAO coating surface increases, while the micropore density decreases. In addition, when the applied voltage is 350–500 V, the uniformity of micropore size decreases. The results show that the applied voltage has an important influence on the surface morphology of MAO coating (Du et al., 2018). Increasing voltage during MAO results in a linear increase in thickness, roughness and porosity of the MAO coatings on Ti and Ti-40Nb substrates, as well as a decrease in adhesion strength values (Komarova et al., 2020a). In addition, Zhao Wang et al. used the MAO technique to fabricate ceramic coatings on AlTiCrVZr alloys and adjusted the voltage to 360, 390, 420 and 450 V during the process (Wang et al., 2023). The resulting coating composition was dominated by Al2O3, TiO2, Cr2O3, V2O5, ZrO2, and SiO2. Additionally, the results showed that the MAO coating gradually became smooth and dense with increasing voltage, the surface roughness decreased the coating thickness increased, and the coating prepared at 420 V showed the best high-temperature oxidation resistance after oxidation for 20 h.
2.3 Current
The density, frequency, duty cycle and type of current also have a significant effect on the thickness, surface roughness, corrosion resistance and porosity of MAO coatings on Ti and its alloys (Sobolev et al., 2019; Lederer et al., 2021; Grigoriev et al., 2022; Muntean et al., 2023). For example, Grigoriev et al. discovered that higher current densities increased the coating thickness and surface roughness of Ti-6Al-4V alloys (Grigoriev et al., 2022). Meanwhile, increasing the current density, frequency and duty cycle results in thicker and denser coatings with better tribological properties on pure Ti surfaces (Lederer et al., 2021). Higher current pulse frequencies will produce denser and less porous coatings, resulting in improved corrosion resistance (Sobolev et al., 2019). Finally, for smoother MAO coatings with lower porosity and denser structures on Ti and its alloys, pulsed currents can be used in place of direct currents (Muntean et al., 2023).
Research by Mónica Echeverry-Rendón et al. tested different electrolytes, voltages, current densities and anodization times to obtain surfaces with different properties. The obtained materials were characterized by different techniques such as X-ray diffraction (XRD), SEM and glow discharge emission spectroscopy (GDOES). The results show that compared with the untreated surface, MAO treatment can obtain a super-hydrophilic surface with a contact angle of about 0°, which is hydrophilic, and this situation remains stable after several weeks of MAO in some cases (Echeverry-Rendón et al., 2017).
In summary, the MAO process is a complex technique that requires careful parameter control to achieve desired coating properties. Electrolyte composition, voltage variations and current parameters influence the topography, thickness, roughness, colour, porosity, corrosion resistance, hardness, hydrophilicity, etc., of MAO coatings on Ti and its alloys (Figure 3).
3 The biocompatibility of the MAO coating
Biocompatibility is the ability of a material to come into contact with a living organism without causing any adverse or rejection reaction. It is mainly determined by how an organism’s cells, tissues and immune system respond to the material. In the fields of medicine and biology, it is essential to ensure good biocompatibility of materials. MAO technology facilitates the formation of highly biocompatible oxide coatings on Ti and its alloys. Studies on the biocompatibility of MAO coatings on Ti and its alloys focus on cytocompatibility, hemocompatibility, corrosion resistance, angiogenic activity, osteogenic activity and osseointegration (as shown in Figure 4).
3.1 Cytocompatibility
Cytocompatibility is the ability of a material to interact with biological cells without causing a toxic or immune response.
Various cell types have been shown to adhere and proliferate better on MAO-modified Ti and its alloys, including bone marrow stromal stem cells (BMSCs), MC3T3-E1 cells, MG63 cell lines, hMSCs, NIH-3T3, immortalized skin fibroblasts, rabbit mesenchymal stem cells and endothelial cells (Xiu et al., 2016; Zhang X. et al., 2018; Zhou W. et al., 2019; Zhou et al., 2019 J.; Liao et al., 2020; Shen Y. et al., 2022; Kostelac et al., 2022; Molaei et al., 2022). MAO-treated specimens have highly porous layers that can be observed under SEM. Cells on MAO exhibit polygonal shapes with filamentous and lamellar extensions of pseudopods, improved spreading, increased cell density and pseudopods anchored in micropores (Zhou J. et al., 2019; Pan et al., 2019; Zhang et al., 2019; Li Y. et al., 2020; Kostelac et al., 2022; Wang et al., 2022). The degrees of cell adhesion and proliferation on Ti and its alloy MAO coatings can be influenced by adding different elements or by adjusting the ion concentration in the electrolyte (Wang L. J. et al., 2021; Shen Y. et al., 2022). The longer the MAO process is, the larger the pore size on the coating and the more cells are attached (Zhou W. et al., 2019). Cell adhesion and extension assay on MAO coating on Ti and its alloy as shown in (Figure 5) (Zhou J. et al., 2019; Zhou W. et al., 2019; Zhang et al., 2019; Shen Y. et al., 2022). In addition, under an SEM electron microscope, the smooth titanium surface has parallel scratches arranged along the grinding direction. The surface of the coating treated by micro-arc oxidation shows a typical nano-porous structure. As the duty cycle increases, the surface of the coating shows micron-sized grooves and nano-sized pores that are evenly distributed and connected. Cell proliferation is increasingly obvious in structures with nanoscale porous and micron-scale grooves. (Pan et al., 2019).
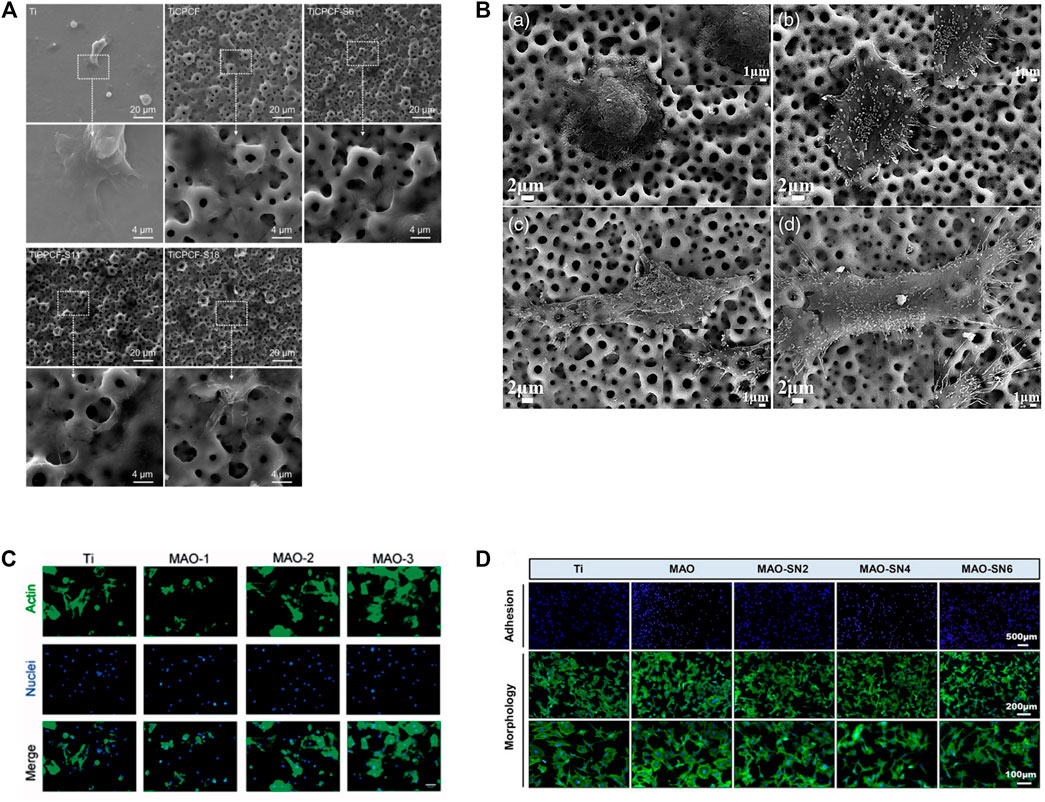
FIGURE 5. Cell adhesion and extension assay. (A) SEM micrographs of MSCs cultured for 3 days. Reprinted from Ref. (Zhou J. et al., 2019). Copyright (2019) Springer Nature. (B) SEM images of morphologies of SaOS-2 cells adhered to the MAO: A 4 h and C 24 h; MHTZn: B 4 h and D 24 h. Adapted from Ref. (Zhang et al., 2019). © 2019 The Author(s). Published by Informa UK Limited, trading as Taylor & Francis Group. (C) BMSCs were stained by FITC-phalloidin and DAPI after incubation for 4 h on four samples. Scale bar = 50 mm. Reprinted from Ref. (Zhou W. et al., 2019). Copyright (2019) Taylor & Francis. (D) Representative staining images of the early adhesion and morphology of MC3T3-E1 cells. Reprinted from Ref. (Shen Y. et al., 2022). Copyright (2022) Frontiers.
Scholars have shown that the MAO-treated Ti surface creates a safe coating that reduces the cytotoxicity of the metal. For example, Ti and its alloys covered with MAO coatings containing Mg, Ca, P, Sr, Co and F show no cellular toxicity, differing from untreated Ti (Zhou J. et al., 2019; Li X. et al., 2020). Additionally, Lorena Kostelac conducted cell adhesion tests to confirm the cytocompatibility of the hydroxyapatite (HAP) coating obtained on the surface of titanium alloys by MAO. Human skin fibroblasts are first incubated with the samples for 48 h and then stained with calcein-AM to visualize viable cells on titanium substrates. The results showed that after 2 days of cell culture, no cytotoxic effects were observed, and fibroblasts spread and colonized all available areas on the sample surface. Furthermore, higher cell densities are observed in all MAO-treated substrates relative to untreated substrates. (Kostelac et al., 2022). However, it is important to note that the presence of excess Cu ions in the coating inhibits cellular activity (Zhang X. et al., 2018). There are also high and low levels of cytotoxicity for each coating, with one study finding that Ti-Ag2O coatings are less cytotoxic than Ti-Ag coatings (Zhang et al., 2021c).
In the design of multifunctional surface coatings for titanium and its alloys, the nanoscale is sometimes involved. Nanoparticles (NPs) can bind to antibodies, ligands, and drugs, thereby enhancing their potential applicability in biotechnology, drug and gene delivery, magnetic separation, and imaging; in addition to their favour properties, they can cause harmful effects if they enter living biological systems and tissues (Attarilar et al., 2020). The formation of MAO coatings on Ti-6Al-4V alloy surfaces with ZrO2 and ZnO nanoparticles significantly improves the corrosion resistance of MAO-treated Ti and its alloys, with corrosion rates that are approximately 14 times lower than those of the substrate (Nadimi and Dehghanian, 2021). These results suggest that MAO treatment is beneficial in reducing cytotoxicity.
Therefore, MAO treatment can create a safe coating on the surface of Ti and its alloys to enhance the cytocompatibility of Ti and its alloys to promote cell adhesion, proliferation and differentiation.
3.2 Hemocompatibility
Hemocompatibility refers to the biological reactions and effects that occur when a material comes into contact with blood. One of the important indicators to examine the hemocompatibility of blood contact materials is the hemolysis rate. Studies have shown that the hemolysis rates of MAO-treated Ti-6Al-4V alloy and untreated Ti-6Al-4V alloy are 0.85% and 1.51% respectively, indicating that the former has better hemocompatibility. Furthermore, MAO treatment reduces platelet adhesion and activation to the surface of Ti and its alloys which exhibit a uniform but rough porous microstructure (crater-like), inhibits thrombosis and inflammatory responses, and ultimately reduces the risk of vascular endothelial cell damage (Jiang et al., 2015). However, it should be noted that not all MAO treatments improve hemocompatibility and, in some cases, may even reduce haemotolerance. For example, it has been shown that platelet coverage on MAO-treated Ti surfaces can be higher than that without MAO treatment (Klein et al., 2020). Wang Maosheng et al. also found that platelets spread on MAO-treated surfaces and exhibited extensive pseudopods. The interaction between platelets and the MAO-treated surface resulted in the formation of an almost connected platelet layer (Wang M.-S. et al., 2015).
3.3 Corrosion resistance
To be able to evaluate the quality of the coatings produced by MAO on Ti and its alloys, it is necessary to test their corrosion resistance, particularly regarding evaluating their biocompatibility (Molaeipour et al., 2022). For biomedical applications, simulated body fluids (SBF) are often used as electrolytes because of their ionic composition, which is similar to that of human blood plasma. Studies have shown that pure Ti with MAO coating immersed in SBF exhibited no significant damage or cracking, while untreated CP-Ti showed ulcerative corrosion (Molaei et al., 2022). In addition, the corrosion current density of the Ti alloy samples with MAO coating decreased by more than 15 times in SBF. A comparative analysis of the corrosion behaviour of samples with and without protective coating in SBF was performed. Potentiodynamic polarization data reveals an improvement in corrosion properties after surface coating with an MAO layer (Mashtalyar et al., 2020). Likewise, a study by Gaoqiang Xu showed that the MAO technique can form wear and corrosion-resistant coatings on Ti and its alloys (Xu and Shen, 2019). These results suggest that in the human body, MAO-treated Ti and its alloy implants may possess good corrosion resistance.
3.4 Angiogenic activity
Studies have demonstrated that the application of MAO coatings onto the surface of Ti and its alloys can augment the attachment, multiplication, differentiation, and transmigration of vascular endothelial cells and fibroblasts. This ultimately results in invigorating tissue repair and regeneration by inspiring vascular rejuvenation. For instance, research by Yiding Shen et al. revealed that MAO-modified titanium evinced noteworthy enhancement in the viability and translocation of human umbilical vein endothelial cells when compared to untreated titanium. Cells on the surface of MAO-treated titanium and its alloys exhibited intricate vascular networks and significantly high levels of vascular genes, indicating the potential for rapid angiogenesis in vitro (Shen Y. et al., 2022). Additionally, mesenchymal stem cells co-cultured with MAO coatings witnessed an elevation in angiogenic factors like HIF-1a and VEGF (Zhou J. et al., 2019). Adding Cu NPs to TiO2 coatings was also found to amplify endothelial cell proliferation and VEGF secretion (Zhang X. et al., 2018). In conclusion, these findings suggest that MAO-treated Ti and its alloys have immense potential for enhancing tissue repair and regeneration by stimulating angiogenesis.
3.5 Osteogenic activity
Osteogenic activity refers to the ability of a material to promote the proliferation and differentiation of bone cells, thereby accelerating the repair and regeneration of bone tissue. The MAO coating on the surface of Ti and its alloys, with its porous structure, have been found to improve the adhesion and bioactivity of osteoblasts while releasing beneficial ions and molecules that stimulate the proliferation and differentiation of osteoblasts and the formation of new bone tissue. Various studies have shown that MAO coatings can increase extracellular matrix mineralization and bone-like apatite deposition by osteoblasts, indicating desirable osteogenic activity (Zhang X. et al., 2018; Zhang. et al., 2021c; Shen Y. et al., 2022). In addition, MAO treatment has been found to increase alkaline phosphatase (ALP) activity, along with a wider positive area for ALP staining than the untreated group (Zhou W. et al., 2019; Zhao et al., 2020). The expression of genes related to osteogenesis is also critical in determining osteoblast activity. qRT-PCR studies have shown that MAO treatment significantly increased the expression levels of osteogenic genes such as Runx2, ALP and osteocalcin (OCN) in Ti and its alloys compared to the untreated group (Wang et al., 2022).
Furthermore, the effect of MAO coating on osteoblasts plays an important role in determining osteoblast activity. Studies have shown that MAO-coated Ti and its alloys promote the spread and growth of osteoclasts on their surface and increase osteoclasts (Santos-Coquillat et al., 2019).
Inappropriate use of antibiotics leads to the proliferation of drug-resistant bacteria and the emergence of “superbugs,” such as methicillin-resistant Staphylococcus aureus (MRSA), Multidrug-resistant (MDR) E. coli (Escherichia coli), Multidrug-resistant Pneumonia Klebsiella, and vancomycin-resistant Enterococcus (VRE). (Xia et al., 2023). The misuse of antibiotics has increased in cases of osteomyelitis. In the study conducted by Teng Zhang and others, they developed a novel multilevel structured MAO 3D-printed porous Ti6Al4V scaffold for the sustained release of vancomycin. The scholars designed polydopamine (PDA) as an adhesive anchor for heparin; the adhesive was affixed to the underlying microporous MAO-TiO2/CaP layers. As confirmed by high-performance liquid chromatography, this system demonstrates high loading capacity and sustained vancomycin release kinetics. In vivo experiments, they injected 0.1 mL of 108 colony-forming units (CFU) of MRSA into the tibiae of rabbits to induce severe osteomyelitis. Physical, haematological, radiological, microbiological, and histopathological analyses were conducted to assess the therapeutic effects. It has been found that rabbits treated with vancomycin-loaded MAO scaffolds inhibit bone infection and enhance bone formation. (Zhang et al., 2020).
3.6 Osseointegration
Osseointegration is an important aspect of ensuring the long-term stability and function of artificial implants in the host bone tissue. It shows that the MAO coating process promotes osseointegration by creating a highly porous and rough surface that improves interfacial bonding between bone tissue and Ti and its alloy implants (Huang L. et al., 2021).
MAO-treated Ti-6Al-4V alloys can promote bone growth and implantation in healthy adult male New Zealand rabbits, with early osteogenesis occurring primarily on the implant surface, followed by external expansion (Xiu et al., 2016). Histological examination showed that the microporous clocks of MAO-treated Ti had more bone tissue surrounded by a thicker and continuous bone matrix than untreated Ti, demonstrating the effectiveness of the MAO coating process in promoting osseointegration (Xiu et al., 2016). Similar experiments have shown that 8 weeks after the placement of Ti implants in adult rabbits, the pure Ti surface was still covered with fibrous tissue, while new bone formed on the surface of the titanium implants with MAO coating (Zhao et al., 2020). Other experiments have shown that MAO-coated implants have better bone-to-implant contact and coverage area attached to the implant, the highest density of newly grown paragenetic bone tissue, and can withstand greater shear (Chen et al., 2021; Ding et al., 2022; Ni et al., 2022). Wang et al.'s study demonstrated good osseointegration of the MAO coating was found by observing gross observation and micro-CT reconstruction of the femoral condyle after implant implantation and new bone formation was observed by toluidine blue and fuchsia-methylene blue staining. (Figure 6); (Wang L. J. et al., 2021). Compared to smooth surfaces, the MAO-treated group had more aggressive contact and much distant osteogenesis (Li et al., 2018).
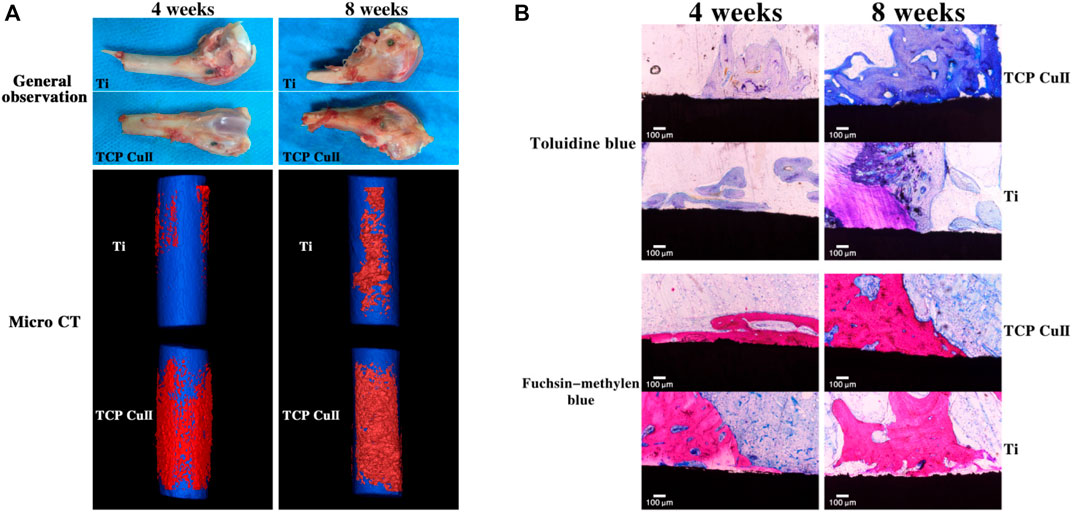
FIGURE 6. Osseointegration assay. (A) Gross observation and micro-CT reconstruction of the femoral condyle were observed at 4 and 8 weeks after implantation. (B) Toluidine blue and fuchsin-methylene blue staining of new bone formation at 4 and 8 weeks after implantation. Reprinted from Ref. (Wang L. J. et al., 2021). Copyright (2021) Springer Nature.
Strontium (Sr), as an essential microelement that acts similarly to calcium with potent bone-seeking properties, has been proven to be an integral feature in the development of bone and/or teeth. Sr-doped implants have been proven to significantly promote early osseointegration under normal and osteoporotic conditions. (Lu W. et al., 2022). It has been reported that Sr prevents osteoporosis via two mechanisms, simultaneously stimulating osteogenesis and restraining osteoclastogenesis via diverse cellular signalling pathways. Recently, Zhou et al. discovered that Sr-doped titanium samples can significantly promote osteogenesis by decreasing ROS expression and inhibiting adipogenic differentiation of mesenchymal stem cells in ageing rats. (Zhou C. et al., 2019). In addition, scientists have implanted MAO coatings into osteoporotic rats and found that high concentrations of Sr2+ significantly reduce the expression of ROS in osteoporosis, showing good results (Shen X. et al., 2022). Overall, MAO significantly improves fixation strength, bone formation, and osseointegration with the Ti and its alloy implant surface.
HA is a biologically active material widely used to enhance the osseointegration of titanium dental implants. During the MAO process, an electrolyte solution containing calcium and phosphate ions is utilized, which can result in the formation of HA within the oxide layer. Subsequent hydrothermal treatment can be employed to increase the HA content. This phenomenon in a titanium oxide surface with high porosity, controlled thickness, and a considerable density of HA. (Lugovskoy and Lugovskoy, 2014).
According to research in the literature, the utilization of HA coatings with a nanoparticle structure can facilitate the adhesion, spreading, and differentiation of bone cells (MC3T3-E1), leading to an improvement in their overall activity. It should be noted, however, that excessive deposition of a large quantity of nano-HA particles on the surface may have adverse effects on cell proliferation (Wang X. et al., 2021).
Additionally, by applying MAO treatment and microwave hydrothermal treatment, a coating containing HA crystals can be rapidly formed on the titanium surface. Research results show that HA can dissolve in SBF, thus increasing the local supersaturation of Ca and phosphorus(P) elements near the coating surface. Furthermore, the material exhibits a good crystallographic match with the deposited apatite, accelerating the formation of the deposited apatite layer. Therefore, this surface demonstrates good cell activity and osseointegration capabilities due to its favourable wettability, high surface energy, and aptitude for apatite formation. (Du et al., 2019).
In summary, the MAO technique can form a layer of biocompatible coating on the surface of Ti and its alloys. This coating promotes the proliferation, adhesion and differentiation of many cell types on the surface and has high cytocompatibility and haem compatibility. In addition, this coating has excellent corrosion resistance and remains stable during long-term use. Importantly, this coating can promote bone formation and therefore has broad application prospects in the medical field.
4 The antibacterial properties of the MAO coating
Bacterial infection remains a significant global threat worldwide owing to its potential to cause multiple organ damage and increase the risk of other diseases. (Tang et al., 2021; Huang et al., 2023; Ji et al., 2023; Lin et al., 2023). Similarly, the use of MAO-treated materials for medical applications is also often hampered by the potential risk of bacterial infection. To address this issue, researchers have explored the use of metallic elements such as Cu, Ag, Zn, Mg, etc., as well as biological antimicrobial agents to enhance the antimicrobial properties of MAO coatings on Ti and its alloys. (Figure 7). Incorporating these elements into the coating can help reduce the risk of bacterial infection, making MAO-treated materials more suitable for medical applications (Supplementary Table S1).
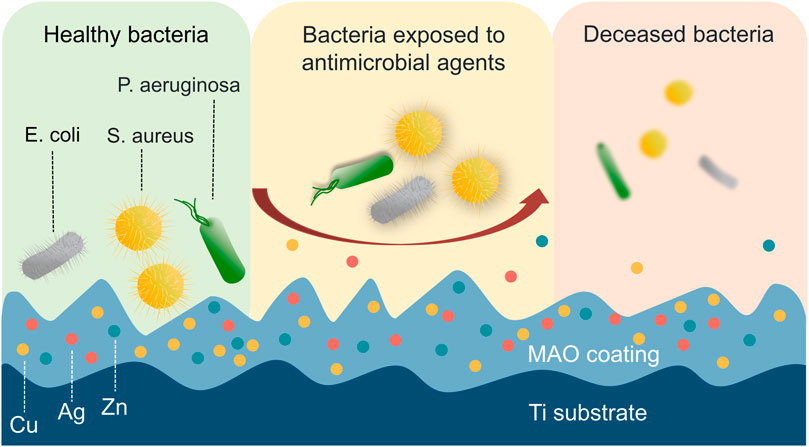
FIGURE 7. Direct contact with antimicrobial elements causes physical damage and cell death in bacteria. The bacteria can experience physical damage to their membrane upon direct contact with antimicrobial elements, including Cu, Ag, and Zn, which ultimately leads to cell death.
4.1 Cu-containing MAO coating
Cu is a vital trace element in the human body with excellent antibacterial properties (Gross et al., 2019). Studies have shown that Cu can enhance lipid peroxidation, inhibit bacterial active DNA and related enzymes, interfere with bacterial energy metabolism, and exhibit a low potential for drug resistance (Shimabukuro, 2020). In addition, Cu ions can kill bacteria or disrupt their replication using direct contact and ion release (Huang et al., 2018; Wang et al., 2019a; He et al., 2020).
The MAO process can be used to successfully produce Cu-containing coatings on Ti and its alloy, resulting in substantially antimicrobial properties (Lu X. et al., 2022). In a related study by Binbin Kang, Cu doping has been found to effectively promote the proliferation of BMSCs and enhance osteogenic differentiation when compared to TiO2 coating. Furthermore, the antibacterial experiments conducted revealed that Cu-doped TiO2 coating had a significant impact on Streptococcus mutans (S. aureus) and Porphyromonas gingivalis (P. gingivalis), demonstrating exceptional antibacterial properties (Figure 8) (Kang et al., 2022).
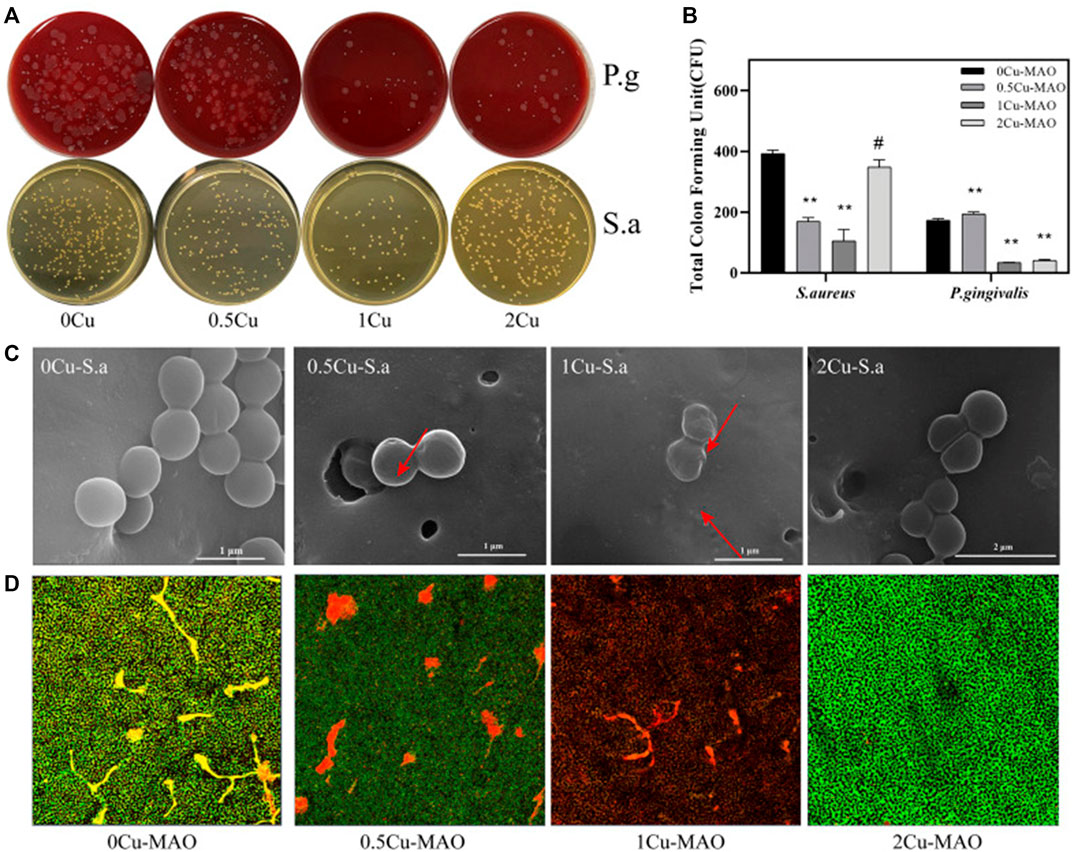
FIGURE 8. Antibacterial activities of Cu-doped coatings against S. aureus and P. gingivalis (A); CFU results after S. aureus and P. gingivalis were cocultured with the Cu-MAO coating for 24 h. Data are presented as mean ± SD; (**p < 0.01, vs. 0Cu-MAO group); (B); Live/Dead assay (D) and the corresponding SEM images (C) of S. aureus cultured on different surface after 24 h of incubation. Source: Reprinted from Ref. (Kang et al., 2022). Copyright © 2022 kang, Lan, Yao, Liu, Chen and Qi, with permission from Frontiers in Bioengineering and Biotechnology.
Furthermore, studies have shown that Cu ions exhibit high bioactivity and excellent antibacterial properties over a range of concentrations and that higher concentrations of Cu lead to better antibacterial effects. The research findings indicate that all samples exhibit morphological features indicative of sedimentation phenomena, with porosity following descending order: Ti−10Cu (∼1.87%) > Ti−5Cu (∼1.55%) > Ti−2Cu (∼0.82%). In addition to the presence of flocculent deposits, the Ti−2Cu coating also exhibits spherical, rod-like, and lamellar deposits. Similarly, typical micron-scale clusters containing sediment of various morphologies are observed in the Ti−5Cu coating. Conversely, the Ti−10Cu coating exclusively features lamellar deposits. The antimicrobial capability of the Ti−2Cu, Ti−5Cu, and Ti−10Cu coatings was assessed by measuring the OD600 value of the medium after 24 h of incubation. The results showed that the OD600 value of the copper-doped TiO2 coating was significantly reduced compared with the titanium substrate, indicating that the copper-doped was beneficial to its antibacterial effect. However, the antibacterial efficacy is inconsistent with its Cu content, because the Ti−2Cu coating shows higher antibacterial efficacy than the Ti−5Cu coating, and the Ti−5Cu coating is similar to the Ti−10Cu coating (Zhang X. et al., 2018).
The studies show that the antimicrobial concentrations of Cu ranging from 0.54% to 0.72% on the surface of MAO coatings showed antibacterial and fungicidal properties (Zheng et al., 2018; Rokosz et al., 2020). However, Zhang Xinxin et al. found that the antibacterial rate (AR) of the TiO2 coating formed by adding 2 g/L Na2Cu EDTA was similar to that of adding 10 g/L; both were superior to the 5 g/L coating (Zhang et al., 2021b). Therefore, the modulation of Cu concentration needs to be further investigated.
Additionally, aside from Cu concentration, the antimicrobial properties of MAO coatings on Ti and its alloys are influenced by the price of Cu ions. It has been shown that Cu-doped TiO2 coatings in the Cu2+ enriched region have better antimicrobial activity compared to those with high Cu + content (Zhang et al., 2020e). Furthermore, t increasing the Cu+/Cu2+ content of the coating leads to better antibacterial and antifungal effects (Rokosz et al., 2020).
MAO coatings with Cu ions have been found to possess powerful long-term antibacterial properties. For example, a porous three-dimensional (3D) MAO coating containing 1.92 wt% Cu (Cu-1.92 wt%) developed on a Ti-6Al-4V alloy maintained 100% antimicrobial activity against S. aureus (S. aureus) even after being immersed in a Hanks solution for 14 days (Liang et al., 2020). In addition, studies have shown Cu-based MAO coatings on Ti alloys exhibit exceptional antibacterial activity against Gram-positive and Gram-negative bacteria even after being submerged in normal brine for 28 days (Shimabukuro et al., 2020b).
The Cu-containing MAO coating achieved 98.7 antibacterial activity against S. mutans, effectively killing S. mutans on almost all surfaces, and also demonstrated potent antibacterial effects against S. aureus and E. coli (Shen et al., 2020a). These results indicate that Cu-based MAO coatings on Ti and its alloys have strong antibacterial properties.
4.2 Ag-containing MAO coating
Ag is a prevalent metal known for its antibacterial properties. The presence of Ag ions can disrupt the membrane integrity of bacterial cells, leading to their death. Many studies have demonstrated that Ag-containing TiO2 coatings can impede the proliferation and adhesion of bacteria such as S. aureus while showing extremely high bactericidal activity against multiple antibacterial strains of S. aureus (e.g., USA300), E. coli and Pseudomonas aeruginosa (P. aeruginosa), which can destroy planktonic and adherent bacteria (Sedelnikova et al., 2019; Zhang L. et al., 2020; Zhang et al., 2021c; van Hengel et al., 2020; Zhao et al., 2022).
Through SEM analysis, it was observed the Ag-MAO coating was uniformly covered with micro/nanoporous oxide layers. Furthermore, the surface morphology of the biofunctionalized implants remained unaltered by the addition of AgNPs when compared to pure titanium and pure titanium combined with strontium implants. Results indicated that after 24 h, Ag-MAO coating completely prevented bacteria from adhering to the surface. After 48 h, little to no bacteria was observed on the surface of the Ag-MAO samples, whereas the control group had significant bacterial adhesion. In vivo experiments on murine femurs showed that the Ag-MAO samples were effective in eliminating bacterial inoculum (Thukkaram et al., 2020). Besides, it has been found that higher concentrations of AgNPs or Ag2O nanoparticles in the electrolyte yield better antibacterial effects for Ag-doped TiO2 coatings. (Lv et al., 2019; Thukkaram et al., 2020). Moreover, TiO2 coatings containing 18.5 wt% Sr and 0.58 wt% Ag showed good osteogenic activity on MC3T3-E1 cells and strong short- and long-term antibacterial effects (Zhang Y.-Y. et al., 2021).
While Ag-rich MAO coatings are highly biocompatible with U2OS cell lines in some studies (Oleshko et al., 2020b), it is important to note that excessive amounts of Ag content can reduce the cytocompatibility of the coating. For instance, coatings synthesised with a concentration of ≥0.001 mol L−1 AgC2H3O2 to the electrolyte have been shown to harm the proliferation of Saos-2 cells (Teker Aydogan et al., 2018). In their research, Masaya Shimabukuro et al. found that MAO coatings of Ti prepared with an electrolyte containing Ag nitrate at a concentration of 0.04 mm or higher exhibited good antibacterial effects, but when Ag concentration exceeded 2.5 mm, the resulting samples inhibited the activity of MC3T3-E1 cells (Shimabukuro et al., 2019). Therefore, it is crucial to pay attention to the concentration of Ag when using it in the MAO treatment of Ti and its alloys, selecting concentrations that are highly effective against bacteria while still exhibiting biocompatibility.
4.3 Zn-containing MAO coating
Zn is an essential trace element for the human body and an important component of superoxide dismutase (Siddiqi et al., 2018). Studies have shown that the incorporation of Zn onto the surface of Ti and its alloys has been shown to not only act as an effective antimicrobial agent but also improve cytocompatibility (Hu et al., 2022; Qin et al., 2022). It was reported that TiO2 coatings significantly inhibited bacterial growth without causing cytotoxicity at concentrations of Zn ions between 10−5 and 10−4 M (Zhang et al., 2016).
In addition, Zn was doped on the surface of Ti-15Mo MAO coating, which was found to be effective in reducing the number of attached Staphylococcus epidermidis (ATCC 700296), indicating that Zn can prevent the formation of bacterial biofilm on the implant surface (Leśniak-Ziółkowska et al., 2020). Oleksandr Oleshko et al. used MAO technology to combine ZnO nanoparticles (NPS) with TiZrNb alloys to form high-contact angle ceramic coatings on TiZrNb alloys. Observe the sample with SEM, the surface is covered with an oxide layer, containing small circular holes and craters of various sizes. This coating containing ZnO NP prevents the adhesion of S. aureus and significantly improves the antimicrobial properties of TiZrNb alloys (Oleshko et al., 2020a).
4.4 Multiple elements-containing MAO coating
Recent research has shown that incorporating multiple elements into MAO coatings can lead to a stronger antibacterial effect than using a single metal element. For instance, TiO2 coatings mixed with both Ag and Zn showed higher antibacterial activity against S. aureus than Ti substrates containing only Ag or Zn alone (Lv et al., 2020; Lv et al., 2021b). Cu, Zn, and P were doped into Ti-6Al-4V Ti alloy coatings via MAO, which showed good antibacterial properties against E. coli, S. aureus, and MRSA (Wang et al., 2020). Meanwhile, TiO2 coatings doped with Mg, Cu, and fluoride (F) elements on the Ti surface were more effective in inhibiting S. aureus than pure Ti and oxide coatings containing Mg, Cu, or F alone (Zhao et al., 2019). In addition, doping multiple metal elements into the coatings not only improved the antibacterial properties of Ti and its alloys but also enhanced biocompatibility. For example, co-doping 0.55 wt% Cu and 2.53 wt% Zn further boosted the proliferation of L-929 cells compared to TiO2 doped with 0.77 wt% Cu alone (Zhang et al., 2018a).
Recent studies have revealed that additional elements beyond the usual Cu, Ag, and Zn elements can be added to MAO coatings to confer antibacterial effects on Ti and its alloys. Elements like Zirconium (Zr), Boron (B), Manganese (Mn), Tungsten (W), Yttrium (Y), and Fluorine (F), have demonstrated bactericidal effects against S. aureus, P. aeruginosa, and E. coli (Sopchenski et al., 2018; Zhou J. et al., 2019; Zhou et al., 2019 T.; Zhang et al., 2020d; Zhang et al., 2020a; Nikoomanzari et al., 2020; Molaei et al., 2022).
In studies evaluating MAO coatings on Ti and its alloys for antibacterial, scholars have also utilized electrolytes containing bioactive elements like Na2SiO3-5H2O, C4H6O4Ca, NaNO3, and C3H7Na2O6P to form bioactive glass-based coatings (MAO-BG). MAO-BG coatings were found to reduce pathogenic bacteria associated with biofilms and have positive effects on various microbial biofilms. This suggests that incorporating bioactive elements into Ti materials could be a useful way to impart antimicrobial properties (Costa et al., 2020).
To summarize, the incorporation of multiple elements into MAO coatings has the potential to enhance the antimicrobial properties and biocompatibility of Ti and its alloys. In addition to commonly used elements like Cu, Ag, and Zn, alternative elements like Zr, B, Mn, W, Y, and F have shown notable bactericidal effects in MAO coatings. Moreover, MAO-BG coatings formed by electrolytes containing bioactive elements can effectively impart antimicrobial properties to Ti materials and positively impact different microbial biofilms. These discoveries offer novel approaches and concepts for developing more powerful antimicrobial coatings.
5 Combined application of MAO and other treatment methods
In modern times, it has been discovered that relying solely on MAO technology may not always suffice for certain biomedical surface modification needs. As a result, researchers have delved into exploring the use of MAO combination with other surface treatment methods, including but not limited to hydrothermal method, sandblasting and acid etching (SLA), selective laser melting (SLM), high-energy shot peening (HESP), sliding friction treatment (SFT), sol-gel coating method (SG), ultrasonic vibration (UV), and bioactive factors (Figure 9). By utilizing a combination of these methods, researchers aim to achieve the desired level of effect (as outlined in Table 2).
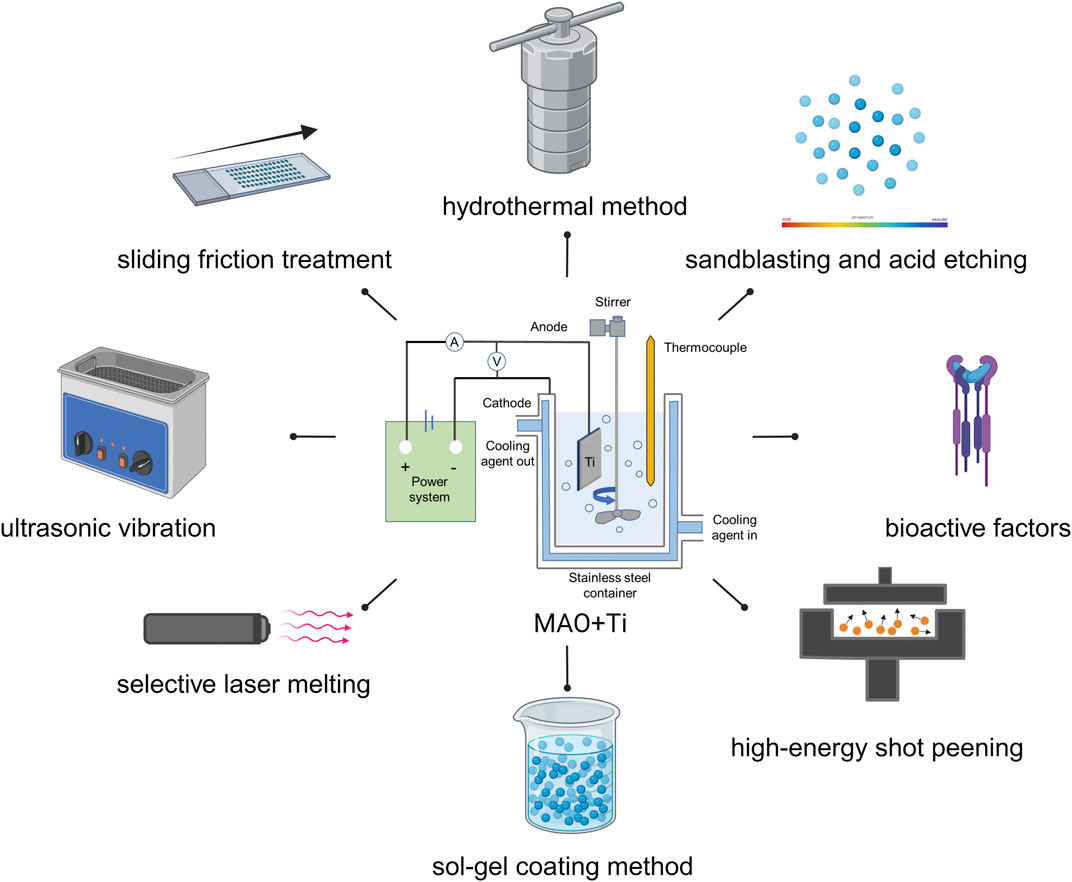
FIGURE 9. Combined application of MAO and other treatment methods. Created with BioRender.com.
5.1 The combined application of MAO and hydrothermal method
By subjecting a material to high temperature and pressure, the hydrothermal method activates water molecules to dissolve or react with surface substances. This promotes crystal growth and alters the structure, morphology, and porosity of the material. Recent studies have shown that combining the hydrothermal method with MAO can result in the formation of micro/nano coatings on Ti and its alloys, which possess excellent biocompatibility and bone integration ability (Huang L. et al., 2021). Some studies also indicate that microwave-assisted hydrothermal technology combined with MAO, can regulate stem cell differentiation (Lin et al., 2020). Furthermore, applying HA coating on the Ti surfaces using MAO and hydrothermal steam treatment (SHT) not only shows good biocompatibility but also stimulates endothelial cells to secrete vascular endothelial growth factor, promoting the formation of capillary-like networks and inhibiting inflammation (Wang X. et al., 2021).
5.2 The combined application of MAO and SLA
SLA are commonly used method to improve the surface roughness of implants. However, using sharp alumina sand and caustic soda may not completely remove these materials, which may negatively affect bone formation around the implant and interfere with bone bonding. To solve this problem, some researchers have combined large particle sandblasting with MAO to create micro- and submicron-level 3D porous structures on Ti implants. This optimizes surface properties and improves new bone formation and bonding (He et al., 2019). Jinyoung Kim’s research on enhancing the surface roughness of Ti by combining sandblasting, acid etching and MAO. The typical crater-like porous structure can be observed under an SEM, which can help enhance the adhesion and differentiation of pre-osteoblasts, ultimately improving the overall biocompatibility (Kim et al., 2021).
5.3 The combined application of MAO and HESP
Different from sandblasting technology, shot peening (SP) technology surface cleaning can also enhance residual compressive stress and improve fatigue resistance. In a study conducted by Xinkun Shen et al., Ti substrates were treated with HESP and MAO to create porous TiO2 films containing Ca and P. The results showed that HESP pretreatment improved the stability of TiO2 coating and the bioactivity of MAO-treated samples (Shen et al., 2020c) Furthermore, the research revealed that using HESP-assisted MAO to produce Si/Cu-MAO coatings resulted in osteogenic properties and bactericidal activity against S. aureus and S. mutans (Shen et al., 2020b).
5.4 The combined application of MAO and SFT
SFT technology is widely used to refine the surface grain structure and improve the mechanical properties, as well as to improve the performance and life of biomaterials through severe surface plastic deformation. Yu Sen and other researchers combined SFT with MAO to form a uniform coating with nearly circular or oval crater-like pores of less than one micron on the surface of Ti3Zr2Sn3Mo25Nb alloy. This coating has demonstrated excellent biocompatibility and can promote the attachment and growth of cells (Yu et al., 2022).
5.5 The combined application of MAO and SLM
To achieve optimal new bone growth in porous scaffolds, it is crucial to maintain precise control and optimization of pore size, porosity, and connectivity throughout the fabrication process. Recently, SLM has garnered significant attention as an additive manufacturing technique for personalized orthopaedic implant production. Junsi Luo’s research team leveraged SLM technology to precisely manufacture Ti alloy implants, using MAO technology to modify their surface for micro/nano-level roughness before coating them with HA. These novel HA coatings possess appropriate mechanical properties, excellent biocompatibility and bioactivity, and enhanced cell viability in vitro, as well as new bone formation in vivo. They may be a promising alternative treatment option for high-risk or younger patients in need of more durable implants (Luo et al., 2021). In another study, researchers synthesized porous Ti implants using SLM and functionalized them with Zn and Ag nanoparticles embedded through MAO with Ti oxide layers, effectively preventing implant-associated infections (IAI) caused by antibiotic-resistant bacteria (Iaj et al., 2020).
5.6 The combined application of MAO and Sol-gel coating method
Researchers have utilized a sol-gel coating method capable of forming uniform nanofilms on the material surface, resulting in bioactive antimicrobial polymer/Ca-P bio-composites on MAO-modified titanium for dental and orthopaedic implant coatings (Yılmaz, 2021). The findings suggest that the coating promotes cell recruitment and improves cell proliferation during the initial stages of culture, potentially extending the lifespan of the implant (Li R. et al., 2020). To improve the performance of oral implants, researchers used MAO and sol-gel techniques to fabricate a multifunctional composite coating consisting of silica particles and zirconium hydrogen phosphate on the Ti surface, which exhibits higher friction and corrosion resistance, as well as better anti-inflammatory and osteogenic properties (Zhu et al., 2022).
5.7 The combined application of MAO and UV
UV is a commonly used auxiliary technique to tune the morphological and structural properties of MAO coatings to enhance their performance. Ultrasound-assisted micro-arc oxidation (UMAO) is a promising surface modification method for fabricating high-quality titanium-based orthopaedic implants. Studies have shown that the use of UV in the MAO process can increase the corrosion resistance and bonding strength of TiO2 coatings, improving their biological properties (Lv et al., 2021a). UMAO can also be used to enhance the corrosion resistance, antimicrobial properties, and cytocompatibility of Cu-doped TiO2 coatings, creating functional surfaces with higher uniformity and performance for biomedical materials (Zhang et al., 2021d). Additionally, UMAO can modify the surface of Ti-Cu alloys to improve their antibacterial properties and cell biocompatibility (Hu et al., 2020).
5.8 The combined application of MAO and bioactive factors
Recent research has shown that combining MAO with other bioactive elements such as Bone Morphogenetic Proteins (BMPs), can stimulate new bone formation. However, the effectiveness of BMPs in promoting osteogenesis depends on the careful control of carrier type and release rate. In a study conducted by Teng et al., a unique approach was employed involving 3D printing, MAO treatment, and the coprecipitation of calcium and P layers with BMP-2 technology to fabricate porous titanium alloy-based implants featuring interconnected channel structures.
The growth factor BMP-2 exhibited a continuous diffusion pattern from the central region to the periphery of the implant, thereby facilitating the proliferation, differentiation, and mineralization of bone cells. In vivo experiments further revealed the infiltration of bone tissue and blood vessels into the central area of the implant. It was observed that MAO-CaP-BMP-2 outperformed both the MAO and MAO-CaP groups in terms of promoting new bone formation, underscoring the potential of MAO-CaP-BMP2 in enhancing bone healing. As a result, MAO-CaP-BMP2 stands as a promising candidate for applications as a growth factor vector. (Teng et al., 2019).
In addition, some studies have found that a petal-like HA/TiO2 composite coating was prepared on the Ti surface through one-step MAO, and then pure chitosan (CS) was used and BMP-2 respectively loaded with CS coatings on the HA/TiO2 surface using a dip coating method, which can give Ti good antibacterial and biological properties, and the thicker the HA layer, the higher the load of BMP-2 and CS. The larger the amount, the better the bonding strength, antibacterial activity and biocompatibility between coatings. (Wang et al., 2019b).
To sum up, the combined MAO technology with other surface treatment methods has demonstrated immense potential in improving surface properties and enhancing the biological performance of biomedical materials. The integration of MAO with diverse techniques such as hydrothermal method, SLA, HESP, SFT, SLM, sol-gel coating method, UV and bioactive factors has resulted in the development of novel coatings that possess superior biocompatibility, antibacterial properties, corrosion resistance, and osteogenic properties, thereby making them well-suited for orthopaedic and dental implant applications. These advancements hold great promise for enhancing the long-term outcomes of implant surgeries while mitigating associated risks. Continued research and development of these hybrid technologies could lead to further improvements in the field of biomedical surface modification, ultimately benefiting patients worldwide.
6 Limitations and future scope
MAO coatings have shown tremendous potential for medical applications, particularly in titanium and its alloys. These coatings enhance surface properties such as biocompatibility, corrosion resistance, wear resistance, and hardness, making them attractive for improving the performance and safety of medical devices. Unfortunately, the use of MAO coatings in medical applications is not without limitations. One major issue is that the coating process can be both expensive and complex, thereby limiting its widespread use. Additionally, the uniformity and thickness of the coating may vary depending on the materials used and the process parameters applied, which can affect the overall performance and reliability of the coating material. Furthermore, while the porous morphologies of MAO coatings theoretically can enhance the bonding between implants and bone tissue, promoting bone tissue growth, the porosity may create channels for accelerated corrosion ion (Cl−) penetration, thus limiting the application of some biodegradable materials. Therefore, researchers exploring the use of magnesium (Mg) in the biomedical field tend to produce MAO coatings with self-sealing pores.
The parameters of MAO are crucial for producing coatings with excellent performance. Process parameters include electrolyte composition, voltage, current density, frequency, response time, and duty cycle. Parameter control aims to obtain a surface that is uniform, stable, adhesive, and bioactive. Although preliminary research has shown promising results, our understanding of the long-term biocompatibility of MAO coatings remains limited. Further research is needed to evaluate their durability and biocompatibility over time. Regarding antibacterial applications, MAO coatings have great potential to reduce bacterial adhesion and growth. However, the efficacy of these coatings depends on several factors, such as coating thickness, roughness, and bacterial strain. Incorporating high concentrations of metal ions into the coating may have adverse effects on cell biocompatibility, and suitable concentrations need to be investigated. Additionally, further research is needed to explore the mechanisms by which these coatings affect cells and bacteria (Zhang et al., 2018b). Furthermore, MAO may need to be combined with other antibacterial agents and treatments to achieve optimal results. Photodynamic antibacterial therapy is receiving increasing attention due to its bactericidal efficiency and safety being higher than those of metal ions. For example, Han et al. found that a MoS2-modified TiO2 coating prepared using a hybrid process exhibits excellent antibacterial activity when exposed to 808 nm near-infrared light (Han et al., 2021). Scholars also indicate that functional TiO2 coatings co-doped with nitrogen and bismuth achieve antibacterial effects under visible light with good biocompatibility and reactivation potential (Nagay et al., 2019).
Additionally, Wenyue Yang et al. reported that TiO2 nanoparticles can be incorporated into the β titanium alloy Ti-35Nb-2Ta-3Zr (TNTZ) matrix using friction stir processing (FSP) as a bioactive reinforcement, which can build an integrated micro-nano composite layer that facilitates the adhesion and proliferation of BMSCs (Yang et al., 2023). Therefore, MAO technology can be considered in the future to build micro-nanostructures on the surface of titanium alloys.
In summary, despite these limitations, researchers continue to explore methods for optimizing coatings, developing new materials and formulations, and combining MAO with other physical and chemical methods to improve its biocompatibility, antibacterial properties, and other properties. This technology has tremendous potential in enhancing the safety and performance of medical biomaterials made from titanium and its alloys.
7 Conclusion
In this review, we delve into recent advancements in the biomedical application of MAO coatings for Ti and its alloys. We focus specifically on process parameters, biocompatibility, antibacterial properties and combinations with other technical aspects. Our conclusions are summarized below:
1) We analyse various process parameters such as electrolyte composition, voltage, and current, that can influence the characteristics of MAO coatings, including their surface roughness, porosity, structure, and morphology attributes.
2) We emphasize the importance of studying the biocompatibility of MAO coatings for Ti and its alloys. Our findings suggest that these coatings have significant potential to enhance cell compatibility, blood compatibility, corrosion resistance, angiogenesis activity, osteogenic activity, and bone integration.
3) Another aspect we highlight is the incorporation of metal elements, such as Cu, Ag, and Zn into MAO coatings. This integration is proven to be an effective technique for imparting antibacterial properties to Ti and its alloys.
4) We discuss several pretreatment and posttreatment hybrid technologies developed to improve the biological properties of MAO coatings. The combination of MAO with hydrothermal methods, SLA, HESP, SFT, SLM, the sol-gel coating method, UV, and bioactive factors provide advantages over using individual techniques.
5) Finally, while considerable progress has been made in researching bioactive MAO coatings on Ti and its alloys, several challenges still need to be addressed. Specifically, further understanding of the mechanism of MAO is needed to facilitate its wider adoption in the industry.
In our review, we emphasize the enormous potential of micro-arc oxidation coatings in improving performance and expanding the applications of titanium and its alloys. In addition, it emphasizes the continuous necessity of MAO technology research in the field of biomedicine. To further promote the application of Ti and its alloys in medical applications, we suggest that future researchers focus on real-world application scenarios, delve into the complex mechanisms behind MAO coatings, and strive to advance this field.
Reducing the gap between laboratory findings and actual medicine is the future research direction. Understanding the complex interactions between MAO coatings and biological systems at the molecular level not only helps to develop customized coatings but also promotes the safe and effective integration of titanium-based materials in the human body. In addition, collaborative efforts bringing together materials scientists, biologists, medical practitioners, and engineers will play a crucial role in promoting the transformation of MAO-coated titanium and its alloys into innovative medical devices and implants. These interdisciplinary methods can bring breakthroughs, redefine the prospects of medical technology, provide enhanced treatment for patients, and improve their quality of life.
In summary, our review serves as a catalyst, and it highlights the potential and necessity for research in the field of micro-arc oxidation coatings on titanium and its alloys. It is through joint efforts and a deeper understanding of basic science that we can fully utilize the capabilities of MAO technology, completely transform biomedical applications, and usher in a new era of medical innovation.
Author contributions
XW: Conceptualization, Investigation, Visualization, Writing–original draft, Writing–review and editing. YL: Investigation, Resources, Writing–review and editing. FX: Investigation, Visualization, Writing–review and editing. XZ: Investigation, Resources, Writing–review and editing. YK: Funding acquisition, Project administration, Supervision, Writing–review and editing.
Funding
The authors declare financial support was received for the research, authorship, and/or publication of this article. This work was supported by the National Natural Science Foundation of China (82002886), and the Shenyang Science and Technology Plan Project (21-173-9-40).
Conflict of interest
The authors declare that the research was conducted in the absence of any commercial or financial relationships that could be construed as a potential conflict of interest.
Publisher’s note
All claims expressed in this article are solely those of the authors and do not necessarily represent those of their affiliated organizations, or those of the publisher, the editors and the reviewers. Any product that may be evaluated in this article, or claim that may be made by its manufacturer, is not guaranteed or endorsed by the publisher.
Supplementary material
The Supplementary Material for this article can be found online at: https://www.frontiersin.org/articles/10.3389/fbioe.2023.1282590/full#supplementary-material
References
Anderson, L. A., Christie, M., Blackburn, B. E., Mahan, C., Earl, C., Pelt, C. E., et al. (2021). 3D-printed titanium metaphyseal cones in revision total knee arthroplasty with cemented and cementless stems. Bone Jt. J. 103-B, 150–157. doi:10.1302/0301-620X.103B6.BJJ-2020-2504.R1
Antunes, R. A., and de Oliveira, M. C. L. (2009). Corrosion processes of physical vapor deposition-coated metallic implants. Crit. Rev. Biomed. Eng. 37, 425–460. doi:10.1615/critrevbiomedeng.v37.i6.10
Attarilar, S., Yang, J., Ebrahimi, M., Wang, Q., Liu, J., Tang, Y., et al. (2020). The toxicity phenomenon and the related occurrence in metal and metal oxide nanoparticles: a brief review from the biomedical perspective. Front. Bioeng. Biotechnol. 8, 822. doi:10.3389/fbioe.2020.00822
Azzouz, I., Khlifi, K., Faure, J., Dhiflaoui, H., Larbi, A. B. C., and Benhayoune, H. (2022). Mechanical behavior and corrosion resistance of sol-gel derived 45S5 bioactive glass coating on Ti6Al4V synthesized by electrophoretic deposition. J. Mech. Behav. Biomed. Mater. 134, 105352. doi:10.1016/j.jmbbm.2022.105352
Chen, H.-T., Lin, H.-I., Chung, C.-J., Tang, C.-H., and He, J.-L. (2021). Osseointegrating and phase-oriented micro-arc-oxidized titanium dioxide bone implants. J. Appl. Biomaterials Funct. Mater. 19. doi:10.1177/22808000211006878
Chen, Z., Yan, X., Chang, Y., Xie, S., Ma, W., Zhao, G., et al. (2019). Effect of polarization voltage on the surface componentization and biocompatibility of micro-arc oxidation modified selective laser melted Ti6Al4V. Mater. Res. Express 6, 086425. doi:10.1088/2053-1591/ab1abc
Costa, R. C., Souza, J. G. S., Cordeiro, J. M., Bertolini, M., de Avila, E. D., Landers, R., et al. (2020). Synthesis of bioactive glass-based coating by plasma electrolytic oxidation: untangling a new deposition pathway toward titanium implant surfaces. J. Colloid Interface Sci. 579, 680–698. doi:10.1016/j.jcis.2020.06.102
Ding, M., Shi, J., Wang, W., Li, D., and Tian, L. (2022). Early osseointegration of micro-arc oxidation coated titanium alloy implants containing Ag: a histomorphometric study. BMC Oral Health 22, 628. doi:10.1186/s12903-022-02673-6
Du, Q., Wei, D., Wang, S., Cheng, S., Wang, Y., Li, B., et al. (2019). Rapidly formation of the highly bioactive surface with hydroxyapatite crystals on the titania micro arc oxidation coating by microwave hydrothermal treatment. Appl. Surf. Sci. 487, 708–718. doi:10.1016/j.apsusc.2019.05.165
Du, Q., Wei, D., Wang, Y., Cheng, S., Liu, S., Zhou, Y., et al. (2018). The effect of applied voltages on the structure, apatite-inducing ability and antibacterial ability of micro arc oxidation coating formed on titanium surface. Bioact. Mater 3, 426–433. doi:10.1016/j.bioactmat.2018.06.001
Echeverry-Rendón, M., Galvis, O., Aguirre, R., Robledo, S., Castaño, J. G., and Echeverría, F. (2017). Modification of titanium alloys surface properties by plasma electrolytic oxidation (PEO) and influence on biological response. J. Mater Sci. Mater Med. 28, 169. doi:10.1007/s10856-017-5972-x
Fattah-Alhosseini, A., Keshavarz, M. K., Molaei, M., and Gashti, S. O. (2018). Plasma electrolytic oxidation (PEO) process on commercially pure Ti surface: effects of electrolyte on the microstructure and corrosion behavior of coatings. Metall. Mater Trans. A 49, 4966–4979. doi:10.1007/s11661-018-4824-8
Ferraris, S., and Spriano, S. (2016). Antibacterial titanium surfaces for medical implants. Mater Sci. Eng. C Mater Biol. Appl. 61, 965–978. doi:10.1016/j.msec.2015.12.062
Gabor, R., Doubkova, M., Gorosova, S., Malanik, K., Vandrovcova, M., Cvrcek, L., et al. (2020). Preparation of highly wettable coatings on Ti–6Al–4V ELI alloy for traumatological implants using micro-arc oxidation in an alkaline electrolyte. Sci. Rep. 10. doi:10.1038/s41598-020-76448-w
Gan, L., and Pilliar, R. (2004). Calcium phosphate sol–gel-derived thin films on porous-surfaced implants for enhanced osteoconductivity. Part I: synthesis and characterization. Biomaterials 25, 5303–5312. doi:10.1016/j.biomaterials.2003.12.038
Grigoriev, S., Peretyagin, N., Apelfeld, A., Smirnov, A., Yanushevich, O., Krikheli, N., et al. (2022). Investigation of MAO coatings characteristics on titanium products obtained by EBM method using additive manufacturing. Materials 15, 4535. doi:10.3390/ma15134535
Gross, T. M., Lahiri, J., Golas, A., Luo, J., Verrier, F., Kurzejewski, J. L., et al. (2019). Copper-containing glass ceramic with high antimicrobial efficacy. Nat. Commun. 10, 1979. doi:10.1038/s41467-019-09946-9
Han, J., Cheng, Y., Tu, W., Zhan, T.-Y., and Cheng, Y. (2018). The black and white coatings on Ti-6Al-4V alloy or pure titanium by plasma electrolytic oxidation in concentrated silicate electrolyte. Appl. Surf. Sci. 428, 684–697. doi:10.1016/j.apsusc.2017.09.109
Han, X., Zhang, G., Chai, M., and Zhang, X. (2021). Light-assisted therapy for biofilm infected micro-arc oxidation TiO2 coating on bone implants. Biomed. Mater. 16, 025018. doi:10.1088/1748-605X/abdb72
He, W., Yin, X., Xie, L., Liu, Z., Li, J., Zou, S., et al. (2019). Enhancing osseointegration of titanium implants through large-grit sandblasting combined with micro-arc oxidation surface modification. J. Mater Sci. Mater Med. 30, 73. doi:10.1007/s10856-019-6276-0
He, X., Zhang, G., Zhang, H., Hang, R., Huang, X., Yao, X., et al. (2020). Cu and Si co-doped microporous TiO2 coating for osseointegration by the coordinated stimulus action. Appl. Surf. Sci. 503, 144072. doi:10.1016/j.apsusc.2019.144072
Hoque, M. E., Showva, N. N., Ahmed, M., Rashid, A. B., Sadique, S. E., El-Bialy, T., et al. (2022). Titanium and titanium alloys in dentistry: current trends, recent developments, and future prospects. Heliyon 8, e11300. doi:10.1016/j.heliyon.2022.e11300
Hsieh, M.-F., Perng, L.-H., and Chin, T.-S. (2002). Hydroxyapatite coating on Ti6Al4V alloy using a sol–gel derived precursor. Mater. Chem. Phys. 74, 245–250. doi:10.1016/S0254-0584(01)00474-6
Hu, J., Li, H., Wang, X., Yang, L., Chen, M., Wang, R., et al. (2020). Effect of ultrasonic micro-arc oxidation on the antibacterial properties and cell biocompatibility of Ti-Cu alloy for biomedical application. Mater Sci. Eng. C Mater Biol. Appl. 115, 110921. doi:10.1016/j.msec.2020.110921
Hu, Y., Zhou, H., Liu, T., Yang, M., Zhang, Q., Pan, C., et al. (2022). Construction of mussel-inspired dopamine-Zn2+ coating on titanium oxide nanotubes to improve hemocompatibility, cytocompatibility, and antibacterial activity. Front. Bioeng. Biotechnol. 10, 884258. doi:10.3389/fbioe.2022.884258
Hu, Z., Cai, J., Song, G., Tian, Y., and Zhou, M. (2021). Anodic oxidation of organic pollutants: anode fabrication, process hybrid and environmental applications. Curr. Opin. Electrochem. 26, 100659. doi:10.1016/j.coelec.2020.100659
Huang, C., Li, H., Li, D., and Lin, S. (2021a). The performance of titanium composite coatings obtained through thermal spraying and microarc oxidation. Compos. Adv. Mater. 30, 2633366X20974686. doi:10.1177/2633366X20974686
Huang, J., Jiang, Y., Lin, W., Chen, R., Zhou, J., Guo, S., et al. (2023). Virulence and adhesion of the Treponema pallidum nichols strain simultaneously decrease in a continuous-infection New Zealand white rabbit model. ACS Infect. Dis. 9, 1221–1231. doi:10.1021/acsinfecdis.2c00601
Huang, L., Cai, B., Huang, Y., Wang, J., Zhu, C., Shi, K., et al. (2021b). Comparative study on 3D printed Ti6Al4V scaffolds with surface modifications using hydrothermal treatment and microarc oxidation to enhance osteogenic activity. ACS Omega 6, 1465–1476. doi:10.1021/acsomega.0c05191
Huang, Q., Li, X., Elkhooly, T. A., Liu, X., Zhang, R., Wu, H., et al. (2018). The Cu-containing TiO2 coatings with modulatory effects on macrophage polarization and bactericidal capacity prepared by micro-arc oxidation on titanium substrates. Colloids Surfaces B Biointerfaces 170, 242–250. doi:10.1016/j.colsurfb.2018.06.020
Hussein, R. O., Nie, X., and Northwood, D. O. (2013). An investigation of ceramic coating growth mechanisms in plasma electrolytic oxidation (PEO) processing. Electrochimica Acta 112, 111–119. doi:10.1016/j.electacta.2013.08.137
Iaj, van H., Ne, P., Mwam, T., Minneboo, M., Ac, F., Le, F.-A., et al. (2020). Biofunctionalization of selective laser melted porous titanium using silver and zinc nanoparticles to prevent infections by antibiotic-resistant bacteria. Acta biomater. 107. doi:10.1016/j.actbio.2020.02.044
Ishikawa, K., Garskaite, E., and Kareiva, A. (2020). Sol–gel synthesis of calcium phosphate-based biomaterials—a review of environmentally benign, simple, and effective synthesis routes. J. Sol-Gel Sci. Technol. 94, 551–572. doi:10.1007/s10971-020-05245-8
Ji, Z., Zheng, J., Ma, Y., Lei, H., Lin, W., Huang, J., et al. (2023). Emergency treatment and photoacoustic assessment of spinal cord injury using reversible dual-signal transform-based selenium antioxidant. Small 19, 2207888. doi:10.1002/smll.202207888
Jiang, J. Y., Xu, J. L., Liu, Z. H., Deng, L., Sun, B., Liu, S. D., et al. (2015). Preparation, corrosion resistance and hemocompatibility of the superhydrophobic TiO2 coatings on biomedical Ti-6Al-4V alloys. Appl. Surf. Sci. 347, 591–595. doi:10.1016/j.apsusc.2015.04.075
Jiang, Y., Wang, J., Hu, B., Yao, Z., Xia, Q., and Jiang, Z. (2016). Preparation of a novel yellow ceramic coating on Ti alloys by plasma electrolytic oxidation. Surf. Coatings Technol. 307, 1297–1302. doi:10.1016/j.surfcoat.2016.05.027
Kang, B., Lan, D., Yao, C., Liu, P., Chen, X., and Qi, S. (2022). Evaluation of antibacterial property and biocompatibility of Cu doped TiO2 coated implant prepared by micro-arc oxidation. Front. Bioeng. Biotechnol. 10, 941109. doi:10.3389/fbioe.2022.941109
Kaseem, M., Fatimah, S., Nashrah, N., and Ko, Y. G. (2021). Recent progress in surface modification of metals coated by plasma electrolytic oxidation: principle, structure, and performance. Prog. Mater. Sci. 117, 100735. doi:10.1016/j.pmatsci.2020.100735
Kaseem, M., and Ko, Y. G. (2019a). Effect of starch on the corrosion behavior of Al-Mg-Si alloy processed by micro arc oxidation from an ecofriendly electrolyte system. Bioelectrochemistry 128, 133–139. doi:10.1016/j.bioelechem.2019.04.004
Kaseem, M., and Ko, Y. G. (2019b). Morphological modification and corrosion response of MgO and Mg3(PO4)2 composite formed on magnesium alloy. Compos. Part B Eng. 176, 107225. doi:10.1016/j.compositesb.2019.107225
Kaur, M., and Singh, K. (2019). Review on titanium and titanium based alloys as biomaterials for orthopaedic applications. Mater Sci. Eng. C Mater Biol. Appl. 102, 844–862. doi:10.1016/j.msec.2019.04.064
Khorasanian, M., Dehghan, A., Shariat, M. H., Bahrololoom, M. E., and Javadpour, S. (2011). Microstructure and wear resistance of oxide coatings on Ti–6Al–4V produced by plasma electrolytic oxidation in an inexpensive electrolyte. Surf. Coatings Technol. 206, 1495–1502. doi:10.1016/j.surfcoat.2011.09.038
Kim, J., Lee, H., Jang, T.-S., Kim, D., Yoon, C.-B., Han, G., et al. (2021). Characterization of titanium surface modification strategies for osseointegration enhancement. Metals 11, 618. doi:10.3390/met11040618
Klein, M., Kuhn, Y., Woelke, E., Linde, T., Ptock, C., Kopp, A., et al. (2020). In vitro study on the hemocompatibility of plasma electrolytic oxidation coatings on titanium substrates. Artif. Organs 44, 419–427. doi:10.1111/aor.13592
Komarova, E. G., Sharkeev, Y. P., Sedelnikova, M. B., Prosolov, K. A., Khlusov, I. A., Prymak, O., et al. (2020a). Zn- or Cu-containing CaP-based coatings formed by Micro-arc oxidation on titanium and Ti-40Nb alloy: part I—microstructure, composition and properties. Materials 13, 4116. doi:10.3390/ma13184116
Komarova, E. G., Sharkeev, Y. P., Sedelnikova, M. B., Prymak, O., Epple, M., Litvinova, L. S., et al. (2020b). Zn- or Cu-containing CaP-based coatings formed by Micro-Arc oxidation on titanium and Ti-40Nb alloy: part II—wettability and biological performance. Materials 13. doi:10.3390/ma13194366
Kostelac, L., Pezzato, L., Settimi, A. G., Franceschi, M., Gennari, C., Brunelli, K., et al. (2022). Investigation of hydroxyapatite (HAP) containing coating on grade 2 titanium alloy prepared by plasma electrolytic oxidation (PEO) at low voltage. Surfaces Interfaces 30, 101888. doi:10.1016/j.surfin.2022.101888
Lederer, S., Arat, S., and Fuerbeth, W. (2021). Influence of process parameters on the tribological behavior of PEO coatings on CP-titanium 4+ alloys for biomedical applications. Mater. (Basel, Switz. 14. doi:10.3390/ma14185364
Le Guéhennec, L., Soueidan, A., Layrolle, P., and Amouriq, Y. (2007). Surface treatments of titanium dental implants for rapid osseointegration. Dent. Mater 23, 844–854. doi:10.1016/j.dental.2006.06.025
Leśniak-Ziółkowska, K., Kazek-Kęsik, A., Rokosz, K., Raaen, S., Stolarczyk, A., Krok-Borkowicz, M., et al. (2020). Electrochemical modification of the Ti-15Mo alloy surface in solutions containing ZnO and Zn3(PO4)2 particles. Mater. Sci. Eng. C 115, 111098. doi:10.1016/j.msec.2020.111098
Li, G., Ma, F., Liu, P., Qi, S., Li, W., Zhang, K., et al. (2023). Review of micro-arc oxidation of titanium alloys: mechanism, properties and applications. J. Alloys Compd. 948, 169773. doi:10.1016/j.jallcom.2023.169773
Li, R., Wei, Y., Gu, L., Qin, Y., and Li, D. (2020a). Sol–gel-assisted micro-arc oxidation synthesis and characterization of a hierarchically rough structured Ta–Sr coating for biomaterials. RSC Adv. 10, 20020–20027. doi:10.1039/D0RA01079K
Li, X., Wang, M., Zhang, W., Bai, Y., Liu, Y., Meng, J., et al. (2020b). A magnesium-incorporated nanoporous titanium coating for rapid osseointegration. Int. J. Nanomedicine 15, 6593–6603. doi:10.2147/IJN.S255486
Li, X., Xu, H., Zhao, B., and Jiang, S. (2018). Accelerated and enhanced osteointegration of MAO-treated implants: histological and histomorphometric evaluation in a rabbit model. Int. J. Oral Sci. 10, 11. doi:10.1038/s41368-018-0008-z
Li, Y., Wang, W., Yu, F., Wang, D., Guan, S., Li, Y., et al. (2020c). Characterization and cytocompatibility of hierarchical porous TiO(2) coatings incorporated with calcium and strontium by one-step micro-arc oxidation. Mater Sci. Eng. C Mater Biol. Appl. 109, 110610. doi:10.1016/j.msec.2019.110610
Li, Z., Cai, Z., Ding, Y., Cui, X.-J., Yang, Z., and Zhu, M. (2020d). Characterization of graphene oxide/ZrO2 composite coatings deposited on zirconium alloy by micro-arc oxidation. Appl. Surf. Sci. 506, 144928. doi:10.1016/j.apsusc.2019.144928
Liang, T., Wang, Y., Zeng, L., Liu, Y., Qiao, L., Zhang, S., et al. (2020). Copper-doped 3D porous coating developed on Ti-6Al-4V alloys and its in vitro long-term antibacterial ability. Appl. Surf. Sci. 509, 144717. doi:10.1016/j.apsusc.2019.144717
Liao, S.-C., Chang, C.-T., Chen, C.-Y., Lee, C.-H., and Lin, W.-L. (2020). Functionalization of pure titanium MAO coatings by surface modifications for biomedical applications. Surf. Coatings Technol. 394, 125812. doi:10.1016/j.surfcoat.2020.125812
Lim, H. K., Choi, Y. J., Choi, W. C., Song, I. S., and Lee, U. L. (2022). Reconstruction of maxillofacial bone defects using patient-specific long-lasting titanium implants. Sci. Rep. 12, 7538. doi:10.1038/s41598-022-11200-0
Lin, D.-J., Fuh, L.-J., and Chen, W.-C. (2020). Nano-morphology, crystallinity and surface potential of anatase on micro-arc oxidized titanium affect its protein adsorption, cell proliferation and cell differentiation. Mater. Sci. Eng. C 107, 110204. doi:10.1016/j.msec.2019.110204
Lin, W., Huang, J., Guo, S., Zhao, M., Chen, X., Shang, Q., et al. (2023). A tunable fluorescent probe for superoxide anion detection during inflammation caused by Treponema pallidum. J. Mater. Chem. B 11, 4523–4528. doi:10.1039/D3TB00747B
Liu, Y.-C., Lin, G.-S., Lee, Y.-T., Huang, T.-C., Chang, T.-W., Chen, Y.-W., et al. (2020). Microstructures and cell reaction of porous hydroxyapatite coatings on titanium discs using a novel vapour-induced pore-forming atmospheric plasma spraying. Surf. Coatings Technol. 393, 125837. doi:10.1016/j.surfcoat.2020.125837
Lu, W., Zhou, Y., Yang, H., Cheng, Z., and He, F. (2022a). Efficacy of strontium supplementation on implant osseointegration under osteoporotic conditions: a systematic review. J. Prosthet. Dent. 128, 341–349. doi:10.1016/j.prosdent.2020.12.031
Lu, X., Zhang, T., Lv, Y., Zhang, X., and Dong, Z. (2022b). Corrosion behviour of micro-arc oxidized titanium in NaCl solution with H2O2 and albumin. Mater. Chem. Phys. 276, 125376. doi:10.1016/j.matchemphys.2021.125376
Lugovskoy, A., and Lugovskoy, S. (2014). Production of hydroxyapatite layers on the plasma electrolytically oxidized surface of titanium alloys. Mater. Sci. Eng. C 43, 527–532. doi:10.1016/j.msec.2014.07.030
Luo, J., Wu, Z., Dai, Y., Wang, X., Ye, R., Huang, H., et al. (2021). Biofunctional micro/nanostructured “volcano-like” layer-coated 3D porous Ti-10Ta-2Nb-2Zr scaffolds improve osteogenesis and osseointegration for dental implants in vitro and in vivo. Surf. Coatings Technol. 427, 127852. doi:10.1016/j.surfcoat.2021.127852
Lv, Y., Cai, G., Zhang, X., Fu, S., Zhang, E., Yang, L., et al. (2020). Microstructural characterization and in vitro biological performances of Ag, Zn co-incorporated TiO2 coating. Ceram. Int. 46, 29160–29172. doi:10.1016/j.ceramint.2020.08.089
Lv, Y., Sun, S., Zhang, X., Lu, X., and Dong, Z. (2021a). Construction of multi-layered Zn-modified TiO2 coating by ultrasound-auxiliary micro-arc oxidation: microstructure and biological property. Mater. Sci. Eng. C 131, 112487. doi:10.1016/j.msec.2021.112487
Lv, Y., Wu, Y., Lu, X., Yu, Y., Fu, S., Yang, L., et al. (2019). Microstructure, bio-corrosion and biological property of Ag-incorporated TiO2 coatings: influence of Ag2O contents. Ceram. Int. 45, 22357–22367. doi:10.1016/j.ceramint.2019.07.265
Lv, Y., Zhang, T., Zhang, X., Fu, S., Yang, L., Dong, Z., et al. (2021b). The synergistic effect of Ag and ZnO on the microstructure, corrosion resistance and in vitro biological performance of titania coating. Surf. Coatings Technol. 426, 127798. doi:10.1016/j.surfcoat.2021.127798
Mashtalyar, D. V., Nadaraia, K. V., Gnedenkov, A. S., Imshinetskiy, I. M., Piatkova, M. A., Pleshkova, A. I., et al. (2020). Bioactive coatings formed on titanium by plasma electrolytic oxidation: composition and properties. Mater. (Basel) 13, 4121. doi:10.3390/ma13184121
Molaei, M., Fattah-Alhosseini, A., and Keshavarz, M. K. (2019). Influence of different sodium-based additives on corrosion resistance of PEO coatings on pure Ti. J. Asian Ceram. Soc. 7, 247–255. doi:10.1080/21870764.2019.1604609
Molaei, M., Fattah-alhosseini, A., Nouri, M., Mahmoodi, P., Navard, S. H., and Nourian, A. (2022). Enhancing cytocompatibility, antibacterial activity and corrosion resistance of PEO coatings on titanium using incorporated ZrO2 nanoparticles. Surfaces Interfaces 30, 101967. doi:10.1016/j.surfin.2022.101967
Molaeipour, P., Allahkaram, S. R., and Akbarzadeh, S. (2022). Corrosion inhibition of Ti6Al4V alloy by a protective plasma electrolytic oxidation coating modified with boron carbide nanoparticles. Surf. Coatings Technol. 430, 127987. doi:10.1016/j.surfcoat.2021.127987
Muntean, R., Brîndușoiu, M., Buzdugan, D., Nemeș, N. S., Kellenberger, A., and Uțu, I. D. (2023). Characteristics of hydroxyapatite-modified coatings based on TiO2 obtained by plasma electrolytic oxidation and electrophoretic deposition. Mater. (Basel) 16, 1410. doi:10.3390/ma16041410
Nadimi, M., and Dehghanian, C. (2021). Incorporation of ZnO–ZrO2 nanoparticles into TiO2 coatings obtained by PEO on Ti–6Al–4V substrate and evaluation of its corrosion behavior, microstructural and antibacterial effects exposed to SBF solution. Ceram. Int. 47, 33413–33425. doi:10.1016/j.ceramint.2021.08.248
Nagay, B. E., Dini, C., Cordeiro, J. M., Ricomini-Filho, A. P., de Avila, E. D., Rangel, E. C., et al. (2019). Visible-light-Induced photocatalytic and antibacterial activity of TiO2 codoped with nitrogen and bismuth: new perspectives to control implant-biofilm-related diseases. ACS Appl. Mater. Interfaces 11, 18186–18202. doi:10.1021/acsami.9b03311
Ni, R., Jing, Z., Xiong, C., Meng, D., Wei, C., and Cai, H. (2022). Effect of micro-arc oxidation surface modification of 3D-printed porous titanium alloys on biological properties. Ann. Transl. Med. 10, 710. doi:10.21037/atm-22-2536
Nikoomanzari, E., Fattah-alhosseini, A., Pajohi Alamoti, M. R., and Keshavarz, M. K. (2020). Effect of ZrO2 nanoparticles addition to PEO coatings on Ti–6Al–4V substrate: microstructural analysis, corrosion behavior and antibacterial effect of coatings in Hank’s physiological solution. Ceram. Int. 46, 13114–13124. doi:10.1016/j.ceramint.2020.02.084
Oh-Hyun, C., In-Gyu, B., Mi, M., Yeon, P., Gyung, Y., and Nae, Y. (2018). Therapeutic outcome of spinal implant infections caused by Staphylococcus aureus A retrospective observational study. Medicine 97. doi:10.1097/MD.0000000000012629
Oleshko, O., Husak, Y., Korniienko, V., Pshenychnyi, R., Varava, Y., Kalinkevich, O., et al. (2020a). Biocompatibility and antibacterial properties of ZnO-incorporated anodic oxide coatings on TiZrNb alloy. Nanomaterials 10, 2401. doi:10.3390/nano10122401
Oleshko, O., Liubchak, I., Husak, Y., Korniienko, V., Yusupova, A., Oleshko, T., et al. (2020b). In vitro biological characterization of silver-doped anodic oxide coating on titanium. Materials 13. doi:10.3390/ma13194359
Pan, X., Li, Y., Abdullah, A. O., Wang, W., Qi, M., and Liu, Y. (2019). Micro/nano-hierarchical structured TiO2 coating on titanium by micro-arc oxidation enhances osteoblast adhesion and differentiation. R. Soc. Open Sci. 6, 182031. doi:10.1098/rsos.182031
Pesode, P., and Barve, S. (2021). Surface modification of titanium and titanium alloy by plasma electrolytic oxidation process for biomedical applications: a review. Mater. Today Proc. 46, 594–602. doi:10.1016/j.matpr.2020.11.294
Qin, Y., Yang, H., Liu, A., Dai, J., Wen, P., Zheng, Y., et al. (2022). Processing optimization, mechanical properties, corrosion behavior and cytocompatibility of additively manufactured Zn-0.7Li biodegradable metals. Acta Biomater. 142, 388–401. doi:10.1016/j.actbio.2022.01.049
Rautray, T. R., Narayanan, R., Kwon, T.-Y., and Kim, K.-H. (2010). Surface modification of titanium and titanium alloys by ion implantation. J. Biomed. Mater. Res. Part B Appl. Biomaterials 93B, 581–591. doi:10.1002/jbm.b.31596
Rokosz, K., Hryniewicz, T., Kacalak, W., Tandecka, K., Raaen, S., Gaiaschi, S., et al. (2020). Porous coatings containing copper and phosphorus obtained by plasma electrolytic oxidation of titanium. Mater. (Basel) 13, 828. doi:10.3390/ma13040828
Santos-Coquillat, A., Mohedano, M., Martinez-Campos, E., Arrabal, R., Pardo, A., and Matykina, E. (2019). Bioactive multi-elemental PEO-coatings on titanium for dental implant applications. Mater. Sci. Eng. C 97, 738–752. doi:10.1016/j.msec.2018.12.097
Sarbishei, S., Faghihi Sani, M. A., and Mohammadi, M. R. (2014). Study plasma electrolytic oxidation process and characterization of coatings formed in an alumina nanoparticle suspension. Vacuum 108, 12–19. doi:10.1016/j.vacuum.2014.05.008
Sasikumar, Y., Indira, K., and Rajendran, N. (2019). Surface modification methods for titanium and its alloys and their corrosion behavior in biological environment: a review. J. Bio Tribo Corros. 5, 36. doi:10.1007/s40735-019-0229-5
Sedelnikova, M. B., Komarova, E. G., Sharkeev, Y. P., Tolkacheva, T. V., Khlusov, I. A., Litvinova, L. S., et al. (2017). Comparative investigations of structure and properties of micro-arc wollastonite-calcium phosphate coatings on titanium and zirconium-niobium alloy. Bioact. Mater 2, 177–184. doi:10.1016/j.bioactmat.2017.01.002
Sedelnikova, M. B., Komarova, E. G., Sharkeev, Y. P., Ugodchikova, A. V., Tolkacheva, T. V., Rau, J. V., et al. (2019). Modification of titanium surface via Ag-, Sr- and Si-containing micro-arc calcium phosphate coating. Bioact. Mater. 4, 224–235. doi:10.1016/j.bioactmat.2019.07.001
Seyidaliyeva, A., Rues, S., Evagorou, Z., Hassel, A. J., Büsch, C., Rammelsberg, P., et al. (2022). Predictability and outcome of titanium color after different surface modifications and anodic oxidation. Dent. Mater. J. 41, 930–936. doi:10.4012/dmj.2022-075
Shemtov-Yona, K., Rittel, D., and Dorogoy, A. (2014). Mechanical assessment of grit blasting surface treatments of dental implants. J. Mech. Behav. Biomed. Mater 39, 375–390. doi:10.1016/j.jmbbm.2014.07.027
Shen, X., Al-Baadani, M. A., He, H., Cai, L., Wu, Z., Yao, L., et al. (2019). Antibacterial and osteogenesis performances of LL37-loaded titania nanopores in vitro and in vivo. Int. J. Nanomedicine 14, 3043–3054. doi:10.2147/IJN.S198583
Shen, X., Fang, K., Ru Yie, K. H., Zhou, Z., Shen, Y., Wu, S., et al. (2022a). High proportion strontium-doped micro-arc oxidation coatings enhance early osseointegration of titanium in osteoporosis by anti-oxidative stress pathway. Bioact. Mater 10, 405–419. doi:10.1016/j.bioactmat.2021.08.031
Shen, X., Hu, W., Ping, L., Liu, C., Yao, L., Deng, Z., et al. (2020a). Antibacterial and osteogenic functionalization of titanium with silicon/copper-doped high-energy shot peening-assisted Micro-Arc oxidation technique. Front. Bioeng. Biotechnol. 8. doi:10.3389/fbioe.2020.573464
Shen, X., Hu, W., Ping, L., Liu, C., Yao, L., Deng, Z., et al. (2020b). Antibacterial and osteogenic functionalization of titanium with silicon/copper-doped high-energy shot peening-assisted Micro-Arc oxidation technique. Front. Bioeng. Biotechnol. 8, 573464. doi:10.3389/fbioe.2020.573464
Shen, X., Ping, L., Wang, L., Liu, C., Liu, J., and Deng, Z. (2020c). Improving the stability and bioactivity of micro-arc oxidized calcium phosphate/titania porous coatings by high energy shot peening pretreatment. Ceram. Int. 46, 2041–2048. doi:10.1016/j.ceramint.2019.09.183
Shen, Y., Fang, K., Xiang, Y., Xu, K., Yu, L., Chen, J., et al. (2022b). Improvement in osteogenesis, vascularization, and corrosion resistance of titanium with silicon-nitride doped micro-arc oxidation coatings. Front. Bioeng. Biotechnol. 10, 1023032. doi:10.3389/fbioe.2022.1023032
Shimabukuro, M. (2020). Antibacterial property and biocompatibility of silver, copper, and zinc in titanium dioxide layers incorporated by one-step Micro-Arc oxidation: a review. Antibiot. (Basel) 9, 716. doi:10.3390/antibiotics9100716
Shimabukuro, M., Hiji, A., Manaka, T., Nozaki, K., Chen, P., Ashida, M., et al. (2020a). Time-transient effects of silver and copper in the porous titanium dioxide layer on antibacterial properties. J. Funct. Biomater. 11. doi:10.3390/jfb11020044
Shimabukuro, M., Tsutsumi, Y., Nozaki, K., Chen, P., Yamada, R., Ashida, M., et al. (2020b). Investigation of antibacterial effect of copper introduced titanium surface by electrochemical treatment against facultative anaerobic bacteria. Dent. Mater. J. 39, 639–647. doi:10.4012/dmj.2019-178
Shimabukuro, M., Tsutsumi, Y., Yamada, R., Ashida, M., Chen, P., Doi, H., et al. (2019). Investigation of realizing both antibacterial property and osteogenic cell compatibility on titanium surface by simple electrochemical treatment. ACS Biomater. Sci. Eng. 5, 5623–5630. doi:10.1021/acsbiomaterials.8b01058
Shokouhfar, M., Dehghanian, C., and Baradaran, A. (2011). Preparation of ceramic coating on Ti substrate by Plasma electrolytic oxidation in different electrolytes and evaluation of its corrosion resistance. Appl. Surf. Sci. 257, 2617–2624. doi:10.1016/j.apsusc.2010.10.032
Shokouhfar, M., Dehghanian, C., Montazeri, M., and Baradaran, A. (2012). Preparation of ceramic coating on Ti substrate by plasma electrolytic oxidation in different electrolytes and evaluation of its corrosion resistance: part II. Appl. Surf. Sci. 258, 2416–2423. doi:10.1016/j.apsusc.2011.10.064
Siddiqi, K. S., ur Rahman, A., Tajuddin, , and Husen, A. (2018). Properties of zinc oxide nanoparticles and their activity against microbes. Nanoscale Res. Lett. 13, 141. doi:10.1186/s11671-018-2532-3
Snizhko, L. O., Yerokhin, A. L., Pilkington, A., Gurevina, N. L., Misnyankin, D. O., Leyland, A., et al. (2004). Anodic processes in plasma electrolytic oxidation of aluminium in alkaline solutions. Electrochimica Acta 49, 2085–2095. doi:10.1016/j.electacta.2003.11.027
Sobolev, A., Kossenko, A., and Borodianskiy, K. (2019). Study of the effect of current pulse frequency on Ti-6Al-4V alloy coating formation by micro arc oxidation. Mater. (Basel) 12, 3983. doi:10.3390/ma12233983
Sopchenski, L., Cogo, S., Dias-Ntipanyj, M. F., Elifio-Espósito, S., Popat, K. C., and Soares, P. (2018). Bioactive and antibacterial boron doped TiO2 coating obtained by PEO. Appl. Surf. Sci. 458, 49–58. doi:10.1016/j.apsusc.2018.07.049
Sousa, L., Mendes, A. R., Pinto, A. M. P., Toptan, F., and Alves, A. C. (2021). Influence of calcium acetate concentration in electrolyte on tribocorrosion behaviour of MAO treated titanium. Metals 11, 1985. doi:10.3390/met11121985
Sun, J., Han, Y., and Cui, K. (2008). Microstructure and apatite-forming ability of the MAO-treated porous titanium. Surf. Coatings Technol. 202, 4248–4256. doi:10.1016/j.surfcoat.2008.03.020
Szmukler-Moncler, S., Perrin, D., Ahossi, V., Magnin, G., and Bernard, J. P. (2004). Biological properties of acid etched titanium implants: effect of sandblasting on bone anchorage. J. Biomed. Mater. Res. Part B Appl. Biomaterials 68B, 149–159. doi:10.1002/jbm.b.20003
Tang, Y., Liu, S., Deng, Y., Zhang, Y., Yin, L., and Zheng, W. (2021). An improved method for soft tissue modeling. Biomed. Signal Process. Control 65, 102367. doi:10.1016/j.bspc.2020.102367
Teker Aydogan, D., Muhaffel, F., Menekse Kilic, M., Karabiyik Acar, O., Cempura, G., Baydogan, M., et al. (2018). Optimisation of micro-arc oxidation electrolyte for fabrication of antibacterial coating on titanium. Mater. Technol. 33, 119–126. doi:10.1080/10667857.2017.1391931
Teng, F.-Y., Tai, I.-C., Ho, M.-L., Wang, J.-W., Weng, L. W., Wang, Y. J., et al. (2019). Controlled release of BMP-2 from titanium with electrodeposition modification enhancing critical size bone formation. Mater. Sci. Eng. C 105, 109879. doi:10.1016/j.msec.2019.109879
Thukkaram, M., Cools, P., Nikiforov, A., Rigole, P., Coenye, T., Van Der Voort, P., et al. (2020). Antibacterial activity of a porous silver doped TiO2 coating on titanium substrates synthesized by plasma electrolytic oxidation. Appl. Surf. Sci. 500, 144235. doi:10.1016/j.apsusc.2019.144235
van Hengel, I. A. J., Gelderman, F. S. A., Athanasiadis, S., Minneboo, M., Weinans, H., Fluit, A. C., et al. (2020). Functionality-packed additively manufactured porous titanium implants. Mater. Today Bio 7, 100060. doi:10.1016/j.mtbio.2020.100060
Velasco, E., Monsalve-Guil, L., Jimenez, A., Ortiz, I., Moreno-Muñoz, J., Nuñez-Marquez, E., et al. (2016). Importance of the roughness and residual stresses of dental implants on fatigue and osseointegration behavior. in vivo study in rabbits. J. Oral Implant. 42, 469–476. doi:10.1563/aaid-joi-D-16-00088
Venkateswarlu, K., Rameshbabu, N., Sreekanth, D., Bose, A. C., Muthupandi, V., Babu, N. K., et al. (2012). Role of electrolyte additives on in-vitro electrochemical behavior of micro arc oxidized titania films on Cp Ti. Appl. Surf. Sci. 258, 6853–6863. doi:10.1016/j.apsusc.2012.03.118
Venkateswarlu, K., Rameshbabu, N., Sreekanth, D., Bose, A. C., Muthupandi, V., Babu, N. K., et al. (2013). Role of electrolyte chemistry on electronic and in vitro electrochemical properties of micro-arc oxidized titania films on Cp Ti. Electrochimica Acta 105, 468–480. doi:10.1016/j.electacta.2013.05.032
Walsh, F. C., Low, C. T. J., Wood, R. J. K., Stevens, K. T., Archer, J., Poeton, A. R., et al. (2009). Plasma electrolytic oxidation (PEO) for production of anodised coatings on lightweight metal (Al, Mg, Ti) alloys. Trans. IMF 87, 122–135. doi:10.1179/174591908X372482
Wang, F., Wang, X., Xie, E., Wang, W., Gan, Q., Li, F., et al. (2022a). Simultaneous incorporation of gallium oxide and tantalum microparticles into micro-arc oxidation coating of titanium possessing antibacterial effect and stimulating cellular response. Biomater. Adv. 135. doi:10.1016/j.bioadv.2022.212736
Wang, G., Li, J., Zhang, W., Xu, L., Pan, H., Wen, J., et al. (2014). Magnesium ion implantation on a micro/nanostructured titanium surface promotes its bioactivity and osteogenic differentiation function. Int. J. Nanomedicine 9, 2387–2398. doi:10.2147/IJN.S58357
Wang, L. J., Ni, X. H., Zhang, F., Peng, Z., Yu, F. X., Zhang, L. B., et al. (2021a). Osteoblast response to copper-doped microporous coatings on titanium for improved bone integration. Nanoscale Res. Lett. 16, 146. doi:10.1186/s11671-021-03602-2
Wang, M.-S., Lee, F.-P., Shen, Y.-D., Chen, C.-H., Ou, K.-L., and Ou, S.-F. (2015a). Surface, biocompatible and hemocompatible properties of meta-amorphous titanium oxide film. Int. J. Appl. Ceram. Technol. 12, 341–350. doi:10.1111/ijac.12184
Wang, R., Ni, S., Ma, L., and Li, M. (2022b). Porous construction and surface modification of titanium-based materials for osteogenesis: a review. Front. Bioeng. Biotechnol. 10, 973297. doi:10.3389/fbioe.2022.973297
Wang, X., Dong, H., Liu, J., Qin, G., Chen, D., and Zhang, E. (2019a). In vivo antibacterial property of Ti-Cu sintered alloy implant. Mater. Sci. Eng. C 100, 38–47. doi:10.1016/j.msec.2019.02.084
Wang, X., Li, B., and Zhang, C. (2019b). Preparation of BMP-2/chitosan/hydroxyapatite antibacterial bio-composite coatings on titanium surfaces for bone tissue engineering. Biomed. Microdevices 21, 89. doi:10.1007/s10544-019-0437-2
Wang, X., Mei, L., Jiang, X., Jin, M., Xu, Y., Li, J., et al. (2021b). Hydroxyapatite-coated titanium by Micro-Arc oxidation and steam-hydrothermal treatment promotes osseointegration. Front. Bioeng. Biotechnol. 9, 625877. doi:10.3389/fbioe.2021.625877
Wang, X., Liu, W., Yu, X., Wang, B., Xu, Y., Yan, X., et al. (2022). Advances in surface modification of tantalum and porous tantalum for rapid osseointegration: a thematic review. Front. Bioeng. Biotechnol. 10, 983695. doi:10.3389/fbioe.2022.983695
Wang, Y., Yu, H., Chen, C., and Zhao, Z. (2015b). Review of the biocompatibility of micro-arc oxidation coated titanium alloys. Mater. Des. 85, 640–652. doi:10.1016/j.matdes.2015.07.086
Wang, Y., Zhao, S., Li, G., Zhang, S., Zhao, R., Dong, A., et al. (2020). Preparation and in vitro antibacterial properties of anodic coatings co-doped with Cu, Zn, and P on a Ti–6Al–4V alloy. Mater. Chem. Phys. 241, 122360. doi:10.1016/j.matchemphys.2019.122360
Wang, Z., Cheng, Z., Zhang, Y., Shi, X., Rao, M., and Wu, S. (2023). Effect of voltage on the microstructure and high-temperature oxidation resistance of Micro-Arc oxidation coatings on AlTiCrVZr refractory high-entropy alloy. Coatings 13, 14. doi:10.3390/coatings13010014
Wennerberg, A., and Albrektsson, T. (2009). Effects of titanium surface topography on bone integration: a systematic review. Clin. Oral Implants Res. 20 (4), 172–184. doi:10.1111/j.1600-0501.2009.01775.x
Wu, M., and Jiang, F. (2023). Effect of Na2SiO3 concentration on corrosion resistance and wear resistance of MAO ceramic film on the Al-Mg-Sc alloy. Int. J. Appl. Ceram. Technol. 20. doi:10.1111/ijac.14320
Xia, J., Li, Y., He, C., Yong, C., Wang, L., Fu, H., et al. (2023). Synthesis and biological activities of oxazolidinone pleuromutilin derivatives as a potent anti-MRSA agent. ACS Infect. Dis. 9, 1711–1729. doi:10.1021/acsinfecdis.3c00162
Xia, Q., Li, X., Yao, Z., and Jiang, Z. (2021). Investigations on the thermal control properties and corrosion resistance of MAO coatings prepared on Mg-5Y-7Gd-1Nd-0.5Zr alloy. Surf. Coatings Technol. 409, 126874. doi:10.1016/j.surfcoat.2021.126874
Xiu, P., Jia, Z., Lv, J., Yin, C., Cheng, Y., Zhang, K., et al. (2016). Tailored surface treatment of 3D printed porous Ti6Al4V by microarc oxidation for enhanced osseointegration via optimized bone in-growth patterns and interlocked bone/implant interface. ACS Appl. Mater Interfaces 8, 17964–17975. doi:10.1021/acsami.6b05893
Xu, G., and Shen, X. (2019). Fabrication of SiO2 nanoparticles incorporated coating onto titanium substrates by the micro arc oxidation to improve the wear resistance. Surf. Coatings Technol. 364, 180–186. doi:10.1016/j.surfcoat.2019.01.069
Xue, T., Attarilar, S., Liu, S., Liu, J., Song, X., Li, L., et al. (2020). Surface modification techniques of titanium and its alloys to functionally optimize their biomedical properties: thematic review. Front. Bioeng. Biotechnol. 8, 603072. doi:10.3389/fbioe.2020.603072
Yang, W., Liu, X., Fang, Y., Attarilar, S., Zhu, C., Wang, L., et al. (2023). Osteogenic differentiation of bone marrow stromal stem cells on a novel β titanium alloy-based micro-nano composite. J. Mater. Res. Technol. 24, 5864–5875. doi:10.1016/j.jmrt.2023.04.188
Yang, W., Xu, D., Guo, Q., Chen, T., and Chen, J. (2018). Influence of electrolyte composition on microstructure and properties of coatings formed on pure Ti substrate by micro arc oxidation. Surf. Coatings Technol. 349, 522–528. doi:10.1016/j.surfcoat.2018.06.024
Yerokhin, A. L., Nie, X., Leyland, A., and Matthews, A. (2000). Characterisation of oxide films produced by plasma electrolytic oxidation of a Ti–6Al–4V alloy. Surf. Coatings Technol. 130, 195–206. doi:10.1016/S0257-8972(00)00719-2
Yılmaz, E. (2021). Modification of the micro arc-oxidized Ti surface for implant applications. J. Bionic Eng. 18, 1391–1399. doi:10.1007/s42235-021-00101-z
Yong, J., Li, H., Li, Z., Chen, Y., Wang, Y., and Geng, J. (2021). Effect of (NH4)2ZrF6, voltage and treating time on corrosion resistance of Micro-Arc oxidation coatings applied on ZK61M magnesium alloys. Materials 14, 7410. doi:10.3390/ma14237410
Yu, S., Guo, D., Han, J., Sun, L., Zhu, H., Yu, Z., et al. (2020). Enhancing antibacterial performance and biocompatibility of pure titanium by a two-step electrochemical surface coating. ACS Appl. Mater. Interfaces 12, 44433–44446. doi:10.1021/acsami.0c10032
Yu, S., Zeng, D., Zhu, H., Zhang, W., Wang, L., Yu, Z., et al. (2022). Improvement on biocompatibility and corrosion resistance of a Ti3Zr2Sn3Mo25Nb alloy through surface nanocrystallization and micro-arc oxidation. J. Mater Sci. 57, 5298–5314. doi:10.1007/s10853-022-06977-4
Yuan, X., Tan, F., Xu, H., Zhang, S., Qu, F., and Liu, J. (2017). Effects of different electrolytes for micro-arc oxidation on the bond strength between titanium and porcelain. J. Prosthodont. Res. 61, 297–304. doi:10.1016/j.jpor.2016.11.001
Zhang, B., Li, B., Gao, S., Li, Y., Cao, R., Cheng, J., et al. (2020a). Y-doped TiO2 coating with superior bioactivity and antibacterial property prepared via plasma electrolytic oxidation. Mater. Des. 192, 108758. doi:10.1016/j.matdes.2020.108758
Zhang, B., Li, J., He, L., Huang, H., and Weng, J. (2020b). Bio-surface coated titanium scaffolds with cancellous bone-like biomimetic structure for enhanced bone tissue regeneration. Acta Biomater. 114, 431–448. doi:10.1016/j.actbio.2020.07.024
Zhang, L., Guo, J., Yan, T., and Han, Y. (2018). Fibroblast responses and antibacterial activity of Cu and Zn co-doped TiO2 for percutaneous implants. Appl. Surf. Sci. 434, 633–642. doi:10.1016/j.apsusc.2017.10.169
Zhang, L., Li, B., Zhang, X., Wang, D., Zhou, L., Li, H., et al. (2020c). Biological and antibacterial properties of TiO2 coatings containing Ca/P/Ag by one-step and two-step methods. Biomed. Microdevices 22, 24. doi:10.1007/s10544-020-00482-8
Zhang, R., Xu, N., Liu, X., Yang, X., Yan, H., Ma, J., et al. (2019). Micro/nanostructured TiO2/ZnO coating enhances osteogenic activity of SaOS-2 cells. Artif. Cells, Nanomedicine, Biotechnol. 47, 2838–2845. doi:10.1080/21691401.2018.1546187
Zhang, R., Zhong, S., Zeng, L., Li, H., Zhao, R., Zhang, S., et al. (2021a). Novel Mg-incorporated Micro-Arc oxidation coatings for orthopedic implants application. Mater. (Basel) 14. doi:10.3390/ma14195710
Zhang, X., Li, J., Wang, X., Wang, Y., Hang, R., Huang, X., et al. (2018c). Effects of copper nanoparticles in porous TiO2 coatings on bacterial resistance and cytocompatibility of osteoblasts and endothelial cells. Mater. Sci. Eng. C 82, 110–120. doi:10.1016/j.msec.2017.08.061
Zhang, X., Lu, X., Lv, Y., Yang, L., Zhang, E., and Dong, Z. (2021b). Enhancement of corrosion resistance and biological performances of Cu-incorporated hydroxyapatite/TiO2 coating by adjusting Cu chemical configuration and hydroxyapatite contents. ACS Appl. Bio Mater. 4, 903–917. doi:10.1021/acsabm.0c01390
Zhang, X., Lv, Y., Cai, G., Fu, S., Yang, L., Ma, Y., et al. (2021c). Reactive incorporation of Ag into porous TiO2 coating and its influence on its microstructure, in vitro antibacterial efficacy and cytocompatibility. Prog. Nat. Sci. Mater. Int. 31, 215–229. doi:10.1016/j.pnsc.2021.02.002
Zhang, X., Lv, Y., Shan, F., Wu, Y., Lu, X., Peng, Z., et al. (2020d). Microstructure, corrosion resistance, osteogenic activity and antibacterial capability of Mn-incorporated TiO2 coating. Appl. Surf. Sci. 531, 147399. doi:10.1016/j.apsusc.2020.147399
Zhang, X., Peng, Z., Lu, X., Lv, Y., Cai, G., Yang, L., et al. (2020e). Microstructural evolution and biological performance of Cu-incorporated TiO2 coating fabricated through one-step micro-arc oxidation. Appl. Surf. Sci. 508, 144766. doi:10.1016/j.apsusc.2019.144766
Zhang, X., Wang, H., Li, J., He, X., Hang, R., Huang, X., et al. (2016). Corrosion behavior of Zn-incorporated antibacterial TiO2 porous coating on titanium. Ceram. Int. 42, 17095–17100. doi:10.1016/j.ceramint.2016.07.220
Zhang, X., Zhang, T., Lv, Y., Zhang, Y., Lu, X., Xiao, J., et al. (2021d). Enhanced uniformity, corrosion resistance and biological performance of Cu-incorporated TiO2 coating produced by ultrasound-auxiliary micro-arc oxidation. Appl. Surf. Sci. 569, 150932. doi:10.1016/j.apsusc.2021.150932
Zhang, Y.-Y., Zhu, Y., Lu, D.-Z., Dong, W., Bi, W.-J., Feng, X.-J., et al. (2021e). Evaluation of osteogenic and antibacterial properties of strontium/silver-containing porous TiO2 coatings prepared by micro-arc oxidation. J. Biomed. Mater. Res. Part B Appl. Biomaterials 109, 505–516. doi:10.1002/jbm.b.34719
Zhao, B. H., Lee, I. S., Han, I. H., Park, J. C., and Chung, S. M. (2007). Effects of surface morphology on human osteosarcoma cell response. Curr. Appl. Phys. 7, e6. doi:10.1016/j.cap.2006.11.004
Zhao, Q., Wu, J., Li, Y., Xu, R., Zhu, X., Jiao, Y., et al. (2022). Promotion of bone formation and antibacterial properties of titanium coated with porous Si/Ag-doped titanium dioxide. Front. Bioeng. Biotechnol. 10, 1001514. doi:10.3389/fbioe.2022.1001514
Zhao, Q., Yi, L., Hu, A., Jiang, L., Hong, L., and Dong, J. (2019). Antibacterial and osteogenic activity of a multifunctional microporous coating codoped with Mg, Cu and F on titanium. J. Mater. Chem. B 7, 2284–2299. doi:10.1039/C8TB03377C
Zhao, Q.-M., Li, X.-K., Guo, S., Wang, N., Liu, W.-W., Shi, L., et al. (2020). Osteogenic activity of a titanium surface modified with silicon-doped titanium dioxide. Mater Sci. Eng. C Mater Biol. Appl. 110, 110682. doi:10.1016/j.msec.2020.110682
Zheng, K., Wu, J., Li, W., Dippold, D., Wan, Y., and Boccaccini, A. (2018). Incorporation of Cu-containing bioactive glass nanoparticles in gelatin-coated scaffolds enhances bioactivity and osteogenic activity. ACS biomaterials Sci. Eng. 4. doi:10.1021/acsbiomaterials.8b00051
Zhou, C., Chen, Y. Q., Zhu, Y. H., Lin, G. F., Zhang, L. F., Liu, X. C., et al. (2019a). Antiadipogenesis and osseointegration of strontium-doped implant surfaces. J. Dent. Res. 98, 795–802. doi:10.1177/0022034519850574
Zhou, J., Wang, X., and Zhao, L. (2019b). Antibacterial, angiogenic, and osteogenic activities of Ca, P, Co, F, and Sr compound doped titania coatings with different Sr content. Sci. Rep. 9, 14203. doi:10.1038/s41598-019-50496-3
Zhou, T., Liu, J., Zhang, X., Shen, B., Yang, J., Hu, W., et al. (2019c). The antibacterial W-containing microarc oxidation coating on Ti6Al4V. Surf. Coatings Technol. 374, 242–252. doi:10.1016/j.surfcoat.2019.05.089
Zhou, W., Huang, O., Gan, Y., Li, Q., Zhou, T., and Xi, W. (2019d). Effect of titanium implants with coatings of different pore sizes on adhesion and osteogenic differentiation of BMSCs. Artif. Cells Nanomed Biotechnol. 47, 290–299. doi:10.1080/21691401.2018.1553784
Zhu, Y., Shen, Y., Xiang, Y., Fang, K., Xu, K., Ma, P., et al. (2022). Combined application of silica particles and zirconium hydrogen phosphate coating to improve the friction resistance and osteogenic/anti-inflammatory properties of micro-arc oxidation-treated titanium. Surf. Coatings Technol. 451, 129037. doi:10.1016/j.surfcoat.2022.129037
Keywords: Ti and its alloys, micro-arc oxidation, biological properties, antibacterial properties, coating
Citation: Wen X, Liu Y, Xi F, Zhang X and Kang Y (2023) Micro-arc oxidation (MAO) and its potential for improving the performance of titanium implants in biomedical applications. Front. Bioeng. Biotechnol. 11:1282590. doi: 10.3389/fbioe.2023.1282590
Received: 24 August 2023; Accepted: 18 October 2023;
Published: 07 November 2023.
Edited by:
Manjie Zhang, Harbin Medical University, ChinaReviewed by:
Shokouh Attarilar, Shanghai Jiao Tong University, ChinaAlina Vladescu (Dragomir), National Institute for Research and Development in Optoelectronics, Romania
Copyright © 2023 Wen, Liu, Xi, Zhang and Kang. This is an open-access article distributed under the terms of the Creative Commons Attribution License (CC BY). The use, distribution or reproduction in other forums is permitted, provided the original author(s) and the copyright owner(s) are credited and that the original publication in this journal is cited, in accordance with accepted academic practice. No use, distribution or reproduction is permitted which does not comply with these terms.
*Correspondence: Yuanyuan Kang, MjAwODIwNjhAY211LmVkdS5jbg==