- 1Division of Applied Life Science (BK21 Four), Department of Applied Life Chemistry, Institute of Agriculture and Life Science (IALS), Gyeongsang National University (GNU), Jinju, Republic of Korea
- 2Department of Agricultural Microbiology, Faculty of Agriculture, Ain Shams University, Cairo, Egypt
The escalating waste generation rates, driven by population growth, urbanization, and consumption patterns, have made waste management a critical global concern with significant environmental, social, and economic repercussions. Among the various waste sources, lignocellulosic biomass represents a significant proportion of agricultural, agro-industrial, and municipal wastes. Biofuels are gaining attention as a promising substitute to fossil fuels, and butanol is one such biofuel that has been identified as a potential candidate due to its compatibility with existing fuel infrastructure, lower volatility, and higher energy density. Sustainable management of lignocellulosic biomass waste and its utilization in fermentation are viable alternatives to produce butanol via the promising microbial catalyst clostridia. This review provides an overview of lignocellulosic biomass waste management, focusing on recent advances in strain development for butanol production from renewable biomass with an emphasis on future perspectives.
1 Introduction
With the world’s population increasing, there is a concurrent increase in waste generation, prompting countries and organizations to intensify their efforts in waste reduction. Recently, particular emphasis has been placed on waste treatment to curb environmental pollution and address resource scarcity. Waste biorefinery is a promising approach in this regard. It stands at the forefront of innovation, merging cutting-edge biological conversion processes with advanced facilities to harness the immense potential of lignocellulosic biomass as a sustainable feedstock. This ingenious approach enables the generation of a diverse array of valuable products, ranging from value-added chemicals to biofuels (Sharma et al., 2019; Queneau and Han, 2022; Velvizhi et al., 2022).
Biofuels have emerged as a compelling solution, championed for their cost-effectiveness and environmentally friendly nature, especially in the face of rising petroleum prices and mounting apprehensions about the impact of fossil fuels on global warming. A wealth of studies has demonstrated remarkable yields of biofuels (ethanol and butanol) from diverse waste sources. Biobutanol has garnered significant attention due to its exceptional compatibility with combustion engines, setting it apart from bioethanol. Clostridia are known for their native butanol production and ability to utilize various substrates present in waste biomass hydrolysates (Guo et al., 2013; Du et al., 2021). However, the production of butanol by clostridia from these hydrolysates has some limitations. To overcome these limitations, the development of effective clostridia is needed.
Specifically, the metabolic engineering of clostridia holds the potential to significantly enhance the efficiency of bio-based butanol production, making it a pivotal aspect of this field. Moreover, the utilization of clostridia for butanol production from diverse feedstocks is poised to bolster the economic viability of biobutanol production. However, this mini-review does not delve deeply into the intricate details of metabolic engineering of clostridia for butanol production, as previous review articles discussed butanol production by clostridia from different feedstocks and metabolic engineering as a tool to enhance butanol production (Cheng et al., 2019; Liberato et al., 2019; Bao et al., 2020; Linger et al., 2020; Vamsi Krishna et al., 2022; Yang et al., 2022). In this mini-review, we aim to offer a forward-looking perspective while succinctly emphasizing key points, fostering a holistic comprehension of the subject. Thus, this mini-review briefly discusses recent advances in butanol production from lignocellulosic biomass waste using clostridia. We have also provided a brief overview of the application of metabolically engineered clostridial strains in the production of lignocellulosic butanol. Finally, it addresses the challenges of using clostridia for butanol production from lignocellulosic biomass waste and suggests future research directions.
2 Butanol production from biomass waste
The use of biomass waste is a cost-effective approach for biofuel production. By breaking down this biomass waste, several fermentable sugars like glucose and xylose are obtained, serving as substrates for the butanol production process (Cascone, 2008). Here, several biomass wastes including agricultural, agro-industrial, and municipal solid waste are discussed. Lignocellulosic biomass waste fermentations using Clostridium strains for butanol production are addressed in Table 1. In pursuing sustainable waste biomass utilization, it is crucial to develop high-yielding engineered strains capable of efficiently utilizing the wide range of sugars in biomass waste. This approach facilitates cost-effective butanol production using affordable carbon sources and contributes to environmental preservation by repurposing and harnessing waste materials under waste management. Utilizing waste biomass can minimize waste generation, reduce environmental impact, and promote a more sustainable approach to butanol production.
2.1 Agricultural waste
Agricultural wastes refer to the materials produced at different stages of the agricultural process, including final products, by-products, and raw materials, that are no longer useful and are usually discarded (Ylä-Mella et al., 2022). To tackle the environmental challenges posed by agricultural waste, it is essential to adopt eco-friendly strategies that promote waste reduction and recycling. Instead of simply discarding these materials, they can be valorized and transformed into valuable resources through waste conversion processes. This helps create a more sustainable and circular economy and contributes to socioeconomic development, energy security, and resource conservation. By adopting these strategies, we can effectively manage agricultural waste and promote a cleaner and more prosperous future (Chilakamarry et al., 2022). Growing interest is seen nowadays in producing butanol sustainably using agricultural residues. This is in response to concerns about the competition between fuel and food production, which could potentially drive up food prices (Qureshi et al., 2014).
Waste generated from agricultural activities comprises cellulose, hemicellulose, lignin, ash, and protein extractives, which are complex molecular structures of lignocellulosic biomass. Microorganisms can degrade these waste materials into simple monomers, providing a potential renewable energy source (Ge et al., 2021). The feedstocks mainly comprise agricultural residues and crop wastes, including rice straw, wheat straw, corn cobs, and rice husk.
Numerous research groups have recently shown how biobutanol is produced from agricultural wastes (Marchal et al., 1992; Kapoor et al., 2020; Mujtaba et al., 2023). Rice straw was employed in several studies for biobutanol production (Moradi et al., 2013; Amiri et al., 2014). The bacterial strain C. acetobutylicum MTCC 481 produced 12.7 g/L of butanol from rice straw (Ranjan et al., 2013). In another study, prior to acetone-butanol-ethanol (ABE) fermentation, corn cob bagasse (CCB) was pretreated with a NaOH solution to remove lignin and improve cellulase accessibility. The bacterial strain C. acetobutylicum ABE 1201 could produce 9.4 g/L of butanol from CCB (Cai et al., 2016). In a study on biological treatment, lignin, hemicellulose, and amorphous cellulose levels were reduced, increasing wheat straw fermentability to produce butanol. The treated wheat straw was fermented with a coculture of C. cellulovorans 35296 and C. beijerinckii 10132, resulting in butanol production of 14.2 g/L (Valdez-Vazquez et al., 2015). Wang et al. (2019) used the pretreated switchgrass in simultaneous saccharification and fermentation to produce butanol by C. saccharoperbutylacetonicum N1-4, yielding 8.6 g/L butanol.
2.2 Agro-industrial waste
Agro-industrial wastes are generated every year in large quantities. Only 20% of agro-industrial food waste is being repurposed for animal feed, while the remaining amount is either landfilled, incinerated, or composted. Pang et al. (2016) employed the strain C. acetobutylicum GX01 to produce butanol from alkali-pretreated sugarcane bagasse. The butanol production was 14.17 g/L. Maiti et al. (2016) obtained butanol production of 9.3 g/L from apple pomace ultrafiltration sludge using the bacterial strain C. beijerinckii NRRL B-466. Clostridium sp. AS3 achieved butanol production of 8.1 g/L using cassava waste residue hydrolysate (Johnravindar et al., 2021).
Surplus starchy grains and agro-industrial process waste effluents are frequently used as fermentation feedstock due to their affordability. Using better farming practices, Southeast Asia’s oil and sago palms have been converted into sustainable bioresources. Palm oil mill effluents (POME) and crude palm oil (CPO) are products of the industrial processing of oil palm, with CPO being an essential commodity in the international vegetable oil market. POME disposal is still a problem. However, bioconversion has been considered as a potential pollution-control measure. In nations that produce palm oil, like Malaysia, where 15.2 million tons of POME are produced annually, POME is aimed for ABE fermentation which has the potential to be a cheap substrate (Al-Shorgani et al., 2015). In the ABE fermentation of C. saccharoperbutylacetonicum N1-4 (ATCC 13564), the growth and butanol production were shown to be supported by the hydrolysate of the separator sludge from POME. However, the lack of fermentable sugars in separator sludge hydrolysate contributes to the low product levels. C. saccharoperbutylacetonicum N1-4 (ATCC 13564) produced 10.35 g/L of butanol using separator sludge hydrolysate from POME and sago starch hydrolysate without the need for any extra nutrient supplementation (Hipolito et al., 2008).
2.3 Municipal solid waste
Municipal solid waste (MSW) is a heterogeneous blend of non-biomass combustible materials, plant and animal products, and other garbage. It can be used as a renewable biomass source to make chemicals and fuels (Vuppaladadiyam et al., 2022). Using municipal solid waste instead of gasoline might drastically reduce greenhouse gas emissions by between 29.2% and 86.1% (Shi et al., 2009).
From the biodegradable portion of MSW treated with ethanol organosolv, the strain C. acetobutylicum NRRL B-591 generated 8.57 g/L of butanol (Farmanbordar et al., 2018). To completely use the energy potential of the MSW organic fraction, co-cultivation of the fungal strain Mucor indicus 22424 CCUG with C. acetobutylicum NRRL B-591 was performed, yielding 7.9 g/L of butanol (Ebrahimian et al., 2022). Domestic organic waste (DOW) has been the subject of numerous studies looking at it as a substrate for butanol production (Kartik et al., 2022). ABE was generated from DOW hydrolysate by C. beijerinckii B-592 and C. acetobutylicum DSM 1731 at rates of 0.9 and 1.5 g/L, respectively (Claassen et al., 2000).
3 Bioprocess to overcome the complicated nature of feedstocks
Various fermentation techniques have been used after pretreatment to overcome the challenges posed by the complicated nature of feedstocks and feedback inhibition in the production of waste lignocellulosic butanol. These techniques include separate hydrolysis and fermentation (SHF), simultaneous saccharification and fermentation (SSF), simultaneous saccharification and co-fermentation (SSCF), and consolidated bioprocessing (CBP) (Parisutham et al., 2014; Haldar and Purkait, 2020). By developing strains with maximum butanol yield and ensuring efficient utilization of sugars from waste biomass, integrating the bioprocess with these advancements unlocks their full potential for waste management. In SSF, enzymes break down lignocellulosic material waste into simple sugars, and then butanol is produced using those sugars. Saccharification is carried out simultaneously with fermentation, reducing production cost and overall time (Islam et al., 2021). However, one major limitation of SSF is that the sugars released during hydrolysis can inhibit the activity of the cellulase enzymes, which are responsible for breaking down the lignocellulosic waste. This reduces butanol yield (Haldar and Purkait, 2020). In the SSF process, it is hard to achieve high cellulase activity, total sugar yield, and butanol generation with this temperature matching (He et al., 2017; Li et al., 2018).
A new process with a modification of SSF resulted in simultaneous co-saccharification and fermentation (SCSF). It is an alternative to increase the cellulosic butanol production in C. acetobutylicum using a soluble oligomer and regenerated cellulose (Seifollahi and Amiri, 2020). Continuous ABE fermentation has been made possible to meet expectations using a continuous bioreactor that employs immobilized cells and multi-stage fermentation SCSF. In continuous fermentation, solvent concentrations and productivity are increased because two-stage and multi-stage fermentation systems absorb acids, convert them efficiently into solvents, and utilize the available substrates completely (Chang et al., 2016). Using alkaline-pretreated rice straw, a novel two-stage fermentation process was developed to maximize sugar utilization. Butanol production of 15.9 g/L was attained using the bacterial strain C. beijerinckii NCIMB 8052 (Chi et al., 2018).
Consolidated bioprocessing (CBP) is created by employing a single microbe or microbial consortium in a single continuous process through the fermentation of a mixture of sugars obtained after cellulase synthesis and lignocellulose hydrolysis (Olson et al., 2012; Jiang et al., 2019). Waste lignocellulosic butanol fermentation has been subjected to several CBP processes, either through the genetic modification of strains (Lin et al., 2015; Bao et al., 2019;Tian et al., 2019) or by co-cultivating saccharolytic strains like C. cellulolyticum (Salimi and Mahadevan, 2013) and C. thermocellum (Nakayama et al., 2011) for biofuel production. A microbial consortium of Thermoanaerobacterium thermosaccharolyticum M5 and C. acetobutylicum NJ4 could produce butanol from untreated corn cobs through CBP. The butanol production was 7.61 g/L (Jiang et al., 2020).
4 Clostridia development strategies to overcome the challenges of butanol production from lignocellulosic biomass waste
Based on advances in metabolic engineering and synthetic biology tools, strain development in butanol production is improved. Strain development has been accelerated for butanol production by developing tolerance to toxic compounds, improving substrate utilization and butanol selectivity and productivity in lignocellulosic biomass waste (Birgen et al., 2019). There are different cases of strain developments considering waste biomass treatment and bioprocessing (Figure 1).
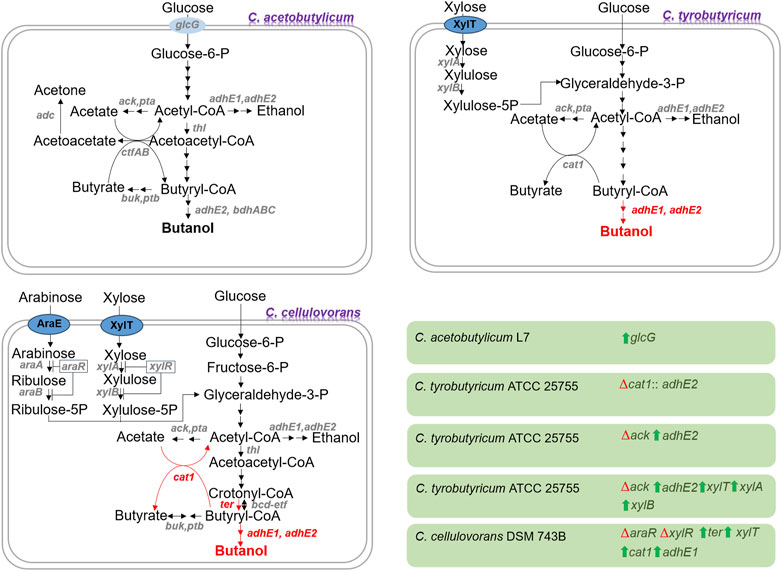
FIGURE 1. Some notable instances of metabolically engineered clostridial strains employed in the production of lignocellulosic butanol. Pathways shown in red color are absent in the wild-type strain. The red triangle and green arrow indicate gene deletion (inactivation) and overexpression, respectively. The genes are displayed in italics, and their corresponding enzymes are as follows: glcG: enzyme II of phosphotransferase system, cat1: CoA transferase, adhE1 and adhE2: bifunctional aldehyde/alcohol dehydrogenase, ack: acetate kinase, pta: phosphotransacetylase, xylT: xylose proton symporter, xylB: xylulokinase, xylA: xylose acetate kinase, xylR: xylose utilization negative regulator, araE: arabinose proton symporter, araR: arabinose utilization negative regulator, araA: arabinose isomerase, araB: ribulokinase, thl: thiolase, ter: trans-enoyl-coenzyme A reductase, adc: acetoacetate decarboxylase, buk: butyrate kinase, ptb: phosphotransbutyrylase, ctfAB: CoA transferase, bcd: butyryl-CoA dehydrogenase, etf: electron transfer flavoprotein, and bdhABC: NADPH-dependent butanol dehydrogenase.
Different physical, chemical, and biological approaches are devised for detoxifying harmful inhibitors. However, these methods still face challenges in generating wastewater, raising energy expenses, and losing sugar, which hinders their economic and environmental feasibility at the industrial level (Xue et al., 2017). Mutagenesis, genetic engineering, and metabolic alterations create inhibitor-resistant strains capable of carrying out butanol fermentation from waste lignocellulosic materials, which eliminates the need for detoxification processes (Cho et al., 2019). One such example is the overexpression of the glucose-specific phosphotransferase system (glcG) gene in the bacterial strain C. acetobutylicum L7, which enabled the strain to utilize the hydrolysate of corn stover without detoxification and produce 10 g/L butanol. This achievement represents a substantial increase of 300% and 400% in butanol production compared to the control and glcG-lacking strains, respectively (Wu et al., 2019). To test the thermotolerance of the engineered strain, it was employed in SSF at 42 °C for butanol production using corn stover pretreated with H2SO4 and NH4OH. It could grow and produce 10.8 g/L of butanol under these conditions (Wu et al., 2021).
Different strategies were employed to modify the bacterium C. cellulovorans DSM 743B to enhance butanol production. It was engineered via integrated metabolic and evolutionary engineering by overexpressing the alcohol/aldehyde dehydrogenase (adhE1), CoA transferase (ctfAB), and acetoacetate decarboxylase (adc) genes from C. acetobutylicum ATCC 824, overexpressing the sporulation regulator (spo0A) gene, and eliminating the sporulation regulator (spo0A*11) gene along with adaptive laboratory evolution. This approach resulted in butanol production of 3.47 g/L from alkali-extracted corn cobs (Wen et al., 2019). In another study, the CoA transferase (cat1) gene from C. tyrobutyricum DSM 2637, and trans-enoyl-coenzyme A reductase (ter) gene from Treponema denticola were overexpressed in C. cellulovorans DSM 743B-adhE1. Also, the xylose metabolism was engineered by inactivating araR (Clocel_1253) and xylR (Clocel_0594) along with overexpressing xylT (CA_C1345). The resulting strain could produce 4.96 g/L of butanol from alkali-extracted corn cobs (Wen et al., 2020).
The bacterium C. tyrobutyricum ATCC 25755 was engineered by overexpressing the aldehyde/alcohol dehydrogenase 2 (adhE2) gene from C. acetobutylicum ATCC 824 and inactivating the acetate kinase (ack) gene to improve butanol production. The engineered strain could produce 10 g/L of butanol from glucose (Yu et al., 2011). The performance of this engineered strain was better when it was employed for butanol production from the hydrolysates of cotton stalk, soybean hull, and sugarcane bagasse, as the butanol titers were 15.8, 14.0, and 11.76 g/L, respectively (Li et al., 2019). Also, its performance was better when it was used for butanol production from the hydrolysate of cassava baggase, as it produced about 15.0 g/L of butanol (Huang et al., 2019). To overcome the glucose catabolite repression and improve xylose utilization, the xylose metabolism genes xylTBA (xylT, xylA, and xylB) from C. acetobutylicum ATCC 824 were co-overexpressed along with the adhE2 gene in C. tyrobutyricum ATCC 25755 (∆ack). The resulting strain could utilize glucose and xylose present in the hydrolysate of soybean hull and produce 15.7 g/L butanol (Yu et al., 2015). Zhang et al. (2018) replaced the butyryl-CoA/acetate CoA transferase (cat1) gene with the adhE2 gene in C. tyrobutyricum ATCC 25755 (KCTC5387). The engineered strain was used for butanol production from the hydrolysate of paper mill sludge supplemented with corn steep liquor, leading to butanol production of 16.5 g/L (Cao et al., 2020).
5 Conclusion and perspectives
Lignocellulosic biomass is a promising feedstock for sustainable butanol production. Clostridia are known for their native butanol production and ability to utilize various substrates present in lignocellulosic biomass hydrolysates (Guo et al., 2013; Du et al., 2021). As a result, several research studies have focused on enhancing the performance of clostridial strains for butanol production and harnessing lignocellulosic biomass waste. The enhanced strains by metabolic engineering have only seen limited application in the context of lignocellulosic biomass utilization for butanol production. While they have primarily been utilized with pure glucose for butanol production (Formanek et al., 1997; Nair et al., 1999; Harris et al., 2000; Harris et al., 2001; Tomas et al., 2003; Jang et al., 2012; Xu et al., 2015; Du et al., 2021; Jang et al., 2023). Several engineered strains are yet to be harnessed for butanol production using lignocellulosic biomass waste. The convergence of these research strands is poised to expedite the development of economically feasible butanol production technologies.
On the other hand, the production of butanol by clostridia from hydrolysates of lignocellulosic biomass has some limitations, including strain inability to tolerate inhibitors present in biomass hydrolysates, strain intolerance to high concentrations of butanol, and low titer. To overcome these limitations, further engineering of clostridia is needed to enhance strain tolerance to inhibitors and butanol, increase strain efficiency in metabolizing the wide range of sugars present in biomass hydrolysates, and redirect the carbon flow toward butanol synthesis for attaining the maximum butanol production capacity from lignocellulosic biomass waste. Identification of the cheapest carbon sources through the exploration of waste lignocellulosic biomass and its pretreatment methods is required. The fermentation process should also be optimized. Combining these areas to produce butanol from the cheapest carbon source with sustainable waste biomass could result in the maximum utilization of waste resources towards effective waste management with the fullest productivity.
Author contributions
SP: Writing–original draft, Investigation. SA: Writing–review and editing, Investigation. ESR: Investigation. Y-SJ: Conceptualization, Funding acquisition, Writing–review and editing.
Funding
The authors declare financial support was received for the research, authorship, and/or publication of this article. This work was supported by the Ministry of Science and ICT through the National Research Foundation of Korea (2022R1A2C1006214).
Conflict of interest
The authors declare that the research was conducted in the absence of any commercial or financial relationships that could be construed as a potential conflict of interest.
Publisher’s note
All claims expressed in this article are solely those of the authors and do not necessarily represent those of their affiliated organizations, or those of the publisher, the editors and the reviewers. Any product that may be evaluated in this article, or claim that may be made by its manufacturer, is not guaranteed or endorsed by the publisher.
References
Al-Shorgani, N. K. N., Shukor, H., Abdeshahian, P., Nazir, M. Y. M., Kalil, M. S., Hamid, A. A., et al. (2015). Process optimization of butanol production by Clostridium saccharoperbutylacetonicum N1-4 (ATCC 13564) using palm oil mill effluent in acetone–butanol–ethanol fermentation. Biocatal. Agric. Biotechnol. 4, 244–249. doi:10.1016/j.bcab.2015.02.004
Amiri, H., Karimi, K., and Zilouei, H. (2014). Organosolv pretreatment of rice straw for efficient acetone, butanol, and ethanol production. Bioresour. Technol. 152, 450–456. doi:10.1016/j.biortech.2013.11.038
Bao, T., Feng, J., Jiang, W., Fu, H., Wang, J., and Yang, S. T. (2020). Recent advances in n-butanol and butyrate production using engineered Clostridium tyrobutyricum. World J. Microbiol. Biotechnol. 36, 138. doi:10.1007/s11274-020-02914-2
Bao, T., Zhao, J., Li, J., Liu, X., and Yang, S. T. (2019). n-Butanol and ethanol production from cellulose by Clostridium cellulovorans overexpressing heterologous aldehyde/alcohol dehydrogenases. Bioresour. Technol. 285, 121316. doi:10.1016/j.biortech.2019.121316
Birgen, C., Dürre, P., Preisig, H. A., and Wentzel, A. (2019). Butanol production from lignocellulosic biomass: revisiting fermentation performance indicators with exploratory data analysis. Biotechnol. Biofuels 12, 167–215. doi:10.1186/s13068-019-1508-6
Cai, D., Dong, Z., Wang, Y., Chen, C., Li, P., Qin, P., et al. (2016). Co-generation of microbial lipid and bio-butanol from corn cob bagasse in an environmentally friendly biorefinery process. Bioresour. Technol. 216, 345–351. doi:10.1016/j.biortech.2016.05.073
Cao, X., Chen, Z., Liang, L., Guo, L., Jiang, Z., Tang, F., et al. (2020). Co-valorization of paper mill sludge and corn steep liquor for enhanced n-butanol production with Clostridium tyrobutyricum Δcat1::adhE2. Bioresour. Technol. 296, 122347. doi:10.1016/j.biortech.2019.122347
Chang, Z., Cai, D., Wang, Y., Chen, C., Fu, C., Wang, G., et al. (2016). Effective multiple stages continuous acetone–butanol–ethanol fermentation by immobilized bioreactors: making full use of fresh corn stalk. Bioresour. Technol. 205, 82–89. doi:10.1016/j.biortech.2016.01.034
Cheng, C., Bao, T., and Yang, S. T. (2019). Engineering Clostridium for improved solvent production: recent progress and perspective. Appl. Microbiol. Biotechnol. 103, 5549–5566. doi:10.1007/s00253-019-09916-7
Chi, X., Li, J., Leu, S. Y., Wang, X., Zhang, Y., and Wang, Y. (2018). Features of a staged acidogenic/solventogenic fermentation process to improve butanol production from rice straw. Energy Fuels 33, 1123–1132. doi:10.1021/acs.energyfuels.8b03095
Chilakamarry, C. R., Sakinah, A. M., Zularisam, A., Sirohi, R., Khilji, I. A., Ahmad, N., et al. (2022). Advances in solid-state fermentation for bioconversion of agricultural wastes to value-added products: opportunities and challenges. Bioresour. Technol. 343, 126065. doi:10.1016/j.biortech.2021.126065
Cho, C., Hong, S., Moon, H. G., Jang, Y. S., Kim, D., and Lee, S. Y. (2019). Engineering clostridial aldehyde/alcohol dehydrogenase for selective butanol production. mBio 10, e02683–18. doi:10.1128/mbio.02683-18
Claassen, P., Budde, M., and Lopez-Contreras, A. (2000). Acetone, butanol and ethanol production from domestic organic waste by solventogenic clostridia. J. Mol. Microbiol. 2, 39–44.
Du, G., Zhu, C., Xu, M., Wang, L., Yang, S. T., and Xue, C. (2021). Energy-efficient butanol production by Clostridium acetobutylicum with histidine kinase knockouts to improve strain tolerance and process robustness. Green Chem. 23, 2155–2168. doi:10.1039/D0GC03993D
Ebrahimian, F., Denayer, J. F., and Karimi, K. (2022). Efficient coproduction of butanol, ethanol, and biohydrogen from municipal solid waste through a cocultivated biorefinery. Energy Convers. Manag. 255, 115303. doi:10.1016/j.enconman.2022.115303
Farmanbordar, S., Amiri, H., and Karimi, K. (2018). Simultaneous organosolv pretreatment and detoxification of municipal solid waste for efficient biobutanol production. Bioresour. Technol. 270, 236–244. doi:10.1016/j.biortech.2018.09.017
Formanek, J., Mackie, R., and Blaschek, H. P. (1997). Enhanced butanol production by Clostridium beijerinckii BA101 grown in semidefined P2 medium containing 6 percent maltodextrin or glucose. Appl. Environ. Microbiol. 63, 2306–2310. doi:10.1128/aem.63.6.2306-2310.1997
Ge, S., Yek, P. N. Y., Cheng, Y. W., Xia, C., Mahari, W. A. W., Liew, R. K., et al. (2021). Progress in microwave pyrolysis conversion of agricultural waste to value-added biofuels: a batch to continuous approach. Renew. Sust. Energ. Rev. 135, 110148. doi:10.1016/j.rser.2020.110148
Guo, T., He, A. Y., Du, T. F., Zhu, D. W., Liang, D. W., Jiang, M., et al. (2013). Butanol production from hemicellulosic hydrolysate of corn fiber by a Clostridium beijerinckii mutant with high inhibitor-tolerance. Bioresour. Technol. 135, 379–385. doi:10.1016/j.biortech.2012.08.029
Haldar, D., and Purkait, M. K. (2020). Lignocellulosic conversion into value-added products: a review. Process Biochem. 89, 110–133. doi:10.1016/j.procbio.2019.10.001
Harris, L. M., Blank, L., Desai, R. P., Welker, N. E., and Papoutsakis, E. T. (2001). Fermentation characterization and flux analysis of recombinant strains of Clostridium acetobutylicum with an inactivated solR gene. J. Ind. Microbiol. Biotechnol. 27, 322–328. doi:10.1038/sj.jim.7000191
Harris, L. M., Desai, R. P., Welker, N. E., and Papoutsakis, E. T. (2000). Characterization of recombinant strains of the Clostridium acetobutylicum butyrate kinase inactivation mutant: need for new phenomenological models for solventogenesis and butanol inhibition? Biotechnol. Bioeng. 67, 1–11. doi:10.1002/(sici)1097-0290(20000105)67:1<1::aid-bit1>3.0.co;2-g
He, C. R., Kuo, Y. Y., and Li, S. Y. (2017). Lignocellulosic butanol production from Napier grass using semi-simultaneous saccharification fermentation. Bioresour. Technol. 231, 101–108. doi:10.1016/j.biortech.2017.01.039
Hipolito, C. N., Crabbe, E., Badillo, C. M., Zarrabal, O. C., Mora, M. A. M., Flores, G. P., et al. (2008). Bioconversion of industrial wastewater from palm oil processing to butanol by Clostridium saccharoperbutylacetonicum N1-4 (ATCC 13564). J. Clean. Prod. 16, 632–638. doi:10.1016/j.jclepro.2007.02.005
Huang, J., Du, Y., Bao, T., Lin, M., Wang, J., and Yang, S. T. (2019). Production of n-butanol from cassava bagasse hydrolysate by engineered Clostridium tyrobutyricum overexpressing adhE2: kinetics and cost analysis. Bioresour. Technol. 292, 121969. doi:10.1016/j.biortech.2019.121969
Islam, M. K., Rehman, S., Guan, J., Lau, C. Y., Tse, H. Y., Yeung, C. S., et al. (2021). Biphasic pretreatment for energy and carbon efficient conversion of lignocellulose into bioenergy and reactive lignin. Appl. Energy 303, 117653. doi:10.1016/j.apenergy.2021.117653
Jang, Y. S., Kim, W. J., Im, J. A., Palaniswamy, S., Yao, Z., Lee, H. L., et al. (2023). Efforts to install a heterologous Wood-Ljungdahl pathway in Clostridium acetobutylicum enable the identification of the native tetrahydrofolate (THF) cycle and result in early induction of solvents. Metab. Eng. 77, 188–198. doi:10.1016/j.ymben.2023.04.005
Jang, Y. S., Lee, J. Y., Lee, J., Park, J. H., Im, J. A., Eom, M. H., et al. (2012). Enhanced butanol production obtained by reinforcing the direct butanol-forming route in Clostridium acetobutylicum. mBio 3, e00314–12. doi:10.1128/mBio.00314-12
Jiang, Y., Lv, Y., Wu, R., Lu, J., Dong, W., Zhou, J., et al. (2020). Consolidated bioprocessing performance of a two-species microbial consortium for butanol production from lignocellulosic biomass. Biotechnol. Bioeng. 117, 2985–2995. doi:10.1002/bit.27464
Jiang, Y., Wu, R., Zhou, J., He, A., Xu, J., Xin, F., et al. (2019). Recent advances of biofuels and biochemicals production from sustainable resources using co-cultivation systems. Biotechnol. Biofuels 12, 155–212. doi:10.1186/s13068-019-1495-7
Johnravindar, D., Elangovan, N., Gopal, N. O., Muthaiyan, A., and Fei, Q. (2021). Biobutanol production from cassava waste residue using Clostridium sp. AS3 in batch culture fermentation. Biofuels 12, 1259–1266. doi:10.1080/17597269.2019.1608671
Kapoor, R., Ghosh, P., Kumar, M., Sengupta, S., Gupta, A., Kumar, S. S., et al. (2020). Valorization of agricultural waste for biogas based circular economy in India: a research outlook. Bioresour. Technol. 304, 123036. doi:10.1016/j.biortech.2020.123036
Kartik, S., Balsora, H. K., Sharma, M., Saptoro, A., Jain, R. K., Joshi, J. B., et al. (2022). Valorization of plastic wastes for production of fuels and value-added chemicals through pyrolysis–A review. Therm. Sci. Eng. Prog. 32, 101316. doi:10.1016/j.tsep.2022.101316
Li, J., Du, Y., Bao, T., Dong, J., Lin, M., Shim, H., et al. (2019). n-Butanol production from lignocellulosic biomass hydrolysates without detoxification by Clostridium tyrobutyricum Δack-adhE2 in a fibrous-bed bioreactor. Bioresour. Technol. 289, 121749. doi:10.1016/j.biortech.2019.121749
Li, T., Wu, Y. R., and He, J. (2018). Heterologous expression, characterization and application of a new β-xylosidase identified in solventogenic Clostridium sp. strain BOH3. Process Biochem. 67, 99–104. doi:10.1016/j.procbio.2018.02.003
Liberato, V., Benevenuti, C., Coelho, F., Botelho, A., Amaral, P., Pereira, N., et al. (2019). Clostridium sp. as bio-catalyst for fuels and chemicals production in a biorefinery context. Catalysts 9, 962. doi:10.3390/catal9110962
Lin, P. P., Mi, L., Morioka, A. H., Yoshino, K. M., Konishi, S., Xu, S. C., et al. (2015). Consolidated bioprocessing of cellulose to isobutanol using Clostridium thermocellum. Metab. Eng. 31, 44–52. doi:10.1016/j.ymben.2015.07.001
Linger, J. G., Ford, L. R., Ramnath, K., and Guarnieri, M. T. (2020). Development of Clostridium tyrobutyricum as a microbial cell factory for the production of fuel and chemical intermediates from lignocellulosic feedstocks. Front. Energy Res. 8, 183. doi:10.3389/fenrg.2020.00183
Maiti, S., Sarma, S. J., Brar, S. K., Le Bihan, Y., Drogui, P., Buelna, G., et al. (2016). Agro-industrial wastes as feedstock for sustainable bio-production of butanol by Clostridium beijerinckii. Food Bioprod. Process 98, 217–226. doi:10.1016/j.fbp.2016.01.002
Marchal, R., Ropars, M., Pourquie, J., Fayolle, F., and Vandecasteele, J. (1992). Large-scale enzymatic hydrolysis of agricultural lignocellulosic biomass. Part 2: conversion into acetone-butanol. Bioresour. Technol. 42, 205–217. doi:10.1016/0960-8524(92)90024-R
Moradi, F., Amiri, H., Soleimanian-Zad, S., Ehsani, M. R., and Karimi, K. (2013). Improvement of acetone, butanol and ethanol production from rice straw by acid and alkaline pretreatments. Fuel 112, 8–13. doi:10.1016/j.fuel.2013.05.011
Mujtaba, M., Fraceto, L., Fazeli, M., Mukherjee, S., Savassa, S. M., de Medeiros, G. A., et al. (2023). Lignocellulosic biomass from agricultural waste to the circular economy: a review with focus on biofuels, biocomposites and bioplastics. J. Clean. Prod. 402, 136815. doi:10.1016/j.jclepro.2023.136815
Nair, R. V., Green, E. M., Watson, D. E., Bennett, G. N., and Papoutsakis, E. T. (1999). Regulation of the sol locus genes for butanol and acetone formation in Clostridium acetobutylicum ATCC 824 by a putative transcriptional repressor. J. Bacteriol. 181, 319–330. doi:10.1128/jb.181.1.319-330.1999
Nakayama, S., Kiyoshi, K., Kadokura, T., and Nakazato, A. (2011). Butanol production from crystalline cellulose by cocultured Clostridium thermocellum and Clostridium saccharoperbutylacetonicum N1-4. Appl. Environ. Microbiol. 77, 6470–6475. doi:10.1128/AEM.00706-11
Olson, D. G., McBride, J. E., Shaw, A. J., and Lynd, L. R. (2012). Recent progress in consolidated bioprocessing. Curr. Opin. Biotechnol. 23, 396–405. doi:10.1016/j.copbio.2011.11.026
Pang, Z. W., Lu, W., Zhang, H., Liang, Z. W., Liang, J. J., Du, L. W., et al. (2016). Butanol production employing fed-batch fermentation by Clostridium acetobutylicum GX01 using alkali-pretreated sugarcane bagasse hydrolysed by enzymes from Thermoascus aurantiacus QS 7-2-4. Bioresour. Technol. 212, 82–91. doi:10.1016/j.biortech.2016.04.013
Parisutham, V., Kim, T. H., and Lee, S. K. (2014). Feasibilities of consolidated bioprocessing microbes: from pretreatment to biofuel production. Bioresour. Technol. 161, 431–440. doi:10.1016/j.biortech.2014.03.114
Queneau, Y., and Han, B. (2022). Biomass: renewable carbon resource for chemical and energy industry. Innovation 3, 100184. doi:10.1016/j.xinn.2021.100184
Qureshi, N., Singh, V., Liu, S., Ezeji, T. C., Saha, B., and Cotta, M. (2014). Process integration for simultaneous saccharification, fermentation, and recovery (SSFR): production of butanol from corn stover using Clostridium beijerinckii P260. Bioresour. Technol. 154, 222–228. doi:10.1016/j.biortech.2013.11.080
Ranjan, A., Mayank, R., and Moholkar, V. S. (2013). Process optimization for butanol production from developed rice straw hydrolysate using Clostridium acetobutylicum MTCC 481 strain. Biomass Convers. biorefin. 3, 143–155. doi:10.1007/s13399-012-0062-2
Salimi, F., and Mahadevan, R. (2013). Characterizing metabolic interactions in a clostridial co-culture for consolidated bioprocessing. BMC Biotechnol. 13, 95. doi:10.1186/1472-6750-13-95
Seifollahi, M., and Amiri, H. (2020). Enhanced production of cellulosic butanol by simultaneous co-saccharification and fermentation of water-soluble cellulose oligomers obtained by chemical hydrolysis. Fuel 263, 116759. doi:10.1016/j.fuel.2019.116759
Sharma, A., Jakhete, A., Sharma, A., Joshi, J. B., and Pareek, V. (2019). Lowering greenhouse gas (GHG) emissions: techno-economic analysis of biomass conversion to biofuels and value-added chemicals. Greenh. Gases Sci. Technol. 9, 454–473. doi:10.1002/ghg.1867
Shi, A. Z., Koh, L. P., and Tan, H. T. (2009). The biofuel potential of municipal solid waste. Glob. Change Biol. Bioenergy 1, 317–320. doi:10.1111/j.1757-1707.2009.01024.x
Tian, L., Conway, P. M., Cervenka, N. D., Cui, J., Maloney, M., Olson, D. G., et al. (2019). Metabolic engineering of Clostridium thermocellum for n-butanol production from cellulose. Biotechnol. Biofuels 12, 186–213. doi:10.1186/s13068-019-1524-6
Tomas, C. A., Welker, N. E., and Papoutsakis, E. T. (2003). Overexpression of groESL in Clostridium acetobutylicum results in increased solvent production and tolerance, prolonged metabolism, and changes in the cell's transcriptional program. Appl. Environ. Microbiol. 69, 4951–4965. doi:10.1128/aem.69.8.4951-4965.2003
Valdez-Vazquez, I., Pérez-Rangel, M., Tapia, A., Buitrón, G., Molina, C., Hernández, G., et al. (2015). Hydrogen and butanol production from native wheat straw by synthetic microbial consortia integrated by species of Enterococcus and Clostridium. Fuel 159, 214–222. doi:10.1016/j.fuel.2015.06.052
Vamsi Krishna, K., Bharathi, N., George Shiju, S., Alagesan Paari, K., and Malaviya, A. (2022). An updated review on advancement in fermentative production strategies for biobutanol using Clostridium spp. Environ. Sci. Pollut. Res. 29, 47988–48019. doi:10.1007/s11356-022-20637-9
Velvizhi, G., Balakumar, K., Shetti, N. P., Ahmad, E., Pant, K. K., and Aminabhavi, T. M. (2022). Integrated biorefinery processes for conversion of lignocellulosic biomass to value added materials: paving a path towards circular economy. Bioresour. Technol. 343, 126151. doi:10.1016/j.biortech.2021.126151
Vuppaladadiyam, A. K., Antunes, E., Vuppaladadiyam, V. S., Shehzad, F., Somasundaram, M., Memon, M. Z., et al. (2022). Discernment of synergy during the co-pyrolysis of lipid-extracted microalgae and digested municipal solid waste: a thermogravimetric–mass spectrometric study. J. Chem. Technol. Biotechnol. 97, 490–500. doi:10.1002/jctb.6702
Wang, P., Chen, Y. M., Wang, Y., Lee, Y. Y., Zong, W., Taylor, S., et al. (2019). Towards comprehensive lignocellulosic biomass utilization for bioenergy production: efficient biobutanol production from acetic acid pretreated switchgrass with Clostridium saccharoperbutylacetonicum N1-4. Appl. Energy 236, 551–559. doi:10.1016/j.apenergy.2018.12.011
Wen, Z., Ledesma-Amaro, R., Lin, J., Jiang, Y., and Yang, S. (2019). Improved n-butanol production from Clostridium cellulovorans by integrated metabolic and evolutionary engineering. Appl. Environ. Microbiol. 85, e02560–18. doi:10.1128/aem.02560-18
Wen, Z., Ledesma-Amaro, R., Lu, M., Jin, M., and Yang, S. (2020). Metabolic engineering of Clostridium cellulovorans to improve butanol production by consolidated bioprocessing. ACS Synth. Biol. 9, 304–315. doi:10.1021/acssynbio.9b00331
Wu, Y., Bai, Y., Zhang, D., Cheng, C., Chen, L., Bai, F., et al. (2019). Pleiotropic regulation of a glucose-specific PTS in Clostridium acetobutylicum for high-efficient butanol production from corn stover without detoxification. Biotechnol. Biofuels 12, 264. doi:10.1186/s13068-019-1604-7
Wu, Y., Wang, Z., Ma, X., and Xue, C. (2021). High temperature simultaneous saccharification and fermentation of corn stover for efficient butanol production by a thermotolerant Clostridium acetobutylicum. Process Biochem. 100, 20–25. doi:10.1016/j.procbio.2020.09.026
Xu, M., Zhao, J., Yu, L., Tang, I. C., Xue, C., and Yang, S. T. (2015). Engineering Clostridium acetobutylicum with a histidine kinase knockout for enhanced n-butanol tolerance and production. Appl. Microbiol. Biotechnol. 99, 1011–1022. doi:10.1007/s00253-014-6249-7
Xue, C., Zhang, X., Wang, J., Xiao, M., Chen, L., and Bai, F. (2017). The advanced strategy for enhancing biobutanol production and high-efficient product recovery with reduced wastewater generation. Biotechnol. Biofuels 10, 148. doi:10.1186/s13068-017-0836-7
Yang, Z., Leero, D. D., Yin, C., Yang, L., Zhu, L., Zhu, Z., et al. (2022). Clostridium as microbial cell factory to enable the sustainable utilization of three generations of feedstocks. Bioresour. Technol. 361, 127656. doi:10.1016/j.biortech.2022.127656
Ylä-Mella, J., Keiski, R. L., and Pongrácz, E. (2022). End-of-Use vs. End-of-Life: when do consumer electronics become waste? Resources 11, 18. doi:10.3390/resources11020018
Yu, L., Xu, M., Tang, I. C., and Yang, S. T. (2015). Metabolic engineering of Clostridium tyrobutyricum for n-butanol production through co-utilization of glucose and xylose. Biotechnol. Bioeng. 112, 2134–2141. doi:10.1002/bit.25613
Yu, M., Zhang, Y., Tang, I. C., and Yang, S. T. (2011). Metabolic engineering of Clostridium tyrobutyricum for n-butanol production. Metab. Eng. 13, 373–382. doi:10.1016/j.ymben.2011.04.002
Keywords: waste management, lignocellulosic biomass, clostridia, butanol, biomass
Citation: Palaniswamy S, Ashoor S, Eskasalam SR and Jang Y-S (2023) Harnessing lignocellulosic biomass for butanol production through clostridia for sustainable waste management: recent advances and perspectives. Front. Bioeng. Biotechnol. 11:1272429. doi: 10.3389/fbioe.2023.1272429
Received: 04 August 2023; Accepted: 16 October 2023;
Published: 25 October 2023.
Edited by:
Jeong Chan Joo, Catholic University of Korea, Republic of KoreaReviewed by:
Joungmin Lee, Korea Institute of Ocean Science and Technology (KIOST), Republic of KoreaZhengming Zhu, Nanjing Tech University, China
Copyright © 2023 Palaniswamy, Ashoor, Eskasalam and Jang. This is an open-access article distributed under the terms of the Creative Commons Attribution License (CC BY). The use, distribution or reproduction in other forums is permitted, provided the original author(s) and the copyright owner(s) are credited and that the original publication in this journal is cited, in accordance with accepted academic practice. No use, distribution or reproduction is permitted which does not comply with these terms.
*Correspondence: Yu-Sin Jang, jangys@gnu.ac.kr