- 1Dunhuang Academy, The Conservation Institute, Dunhuang, China
- 2National Research Center for Conservation of Ancient Wall Paintings and Earthen Sites, Dunhuang, China
- 3Joint International Research Laboratory of Environmental and Social Archaeology, Shandong University, Qingdao, China
- 4Institute of Cultural Heritage, Shandong University, Qingdao, China
Our previous study reported that Saccharomyces cerevisiae could induce calcium carbonate (CaCO3) precipitation, but the associated mechanism was unclear. In the present study, Saccharomyces cerevisiae was cultured under various conditions, including the presence of different organic acids and initial pH, and the yields of CaCO3 formation induced by the different organic acids were compared. The metabolism of organic acid by the metabolites of S. cerevisiae was also assessed in vitro. The SEM-EDS and XRD results showed that only acetate acid, pyruvic acid, and α-ketoglutaric acid could induce CaCO3 formation, and the weight order of the produced CaCO3 was pyruvic acid, acetate acid, α-ketoglutaric acid. In addition, the presence of only yeast metabolites and the initial neutral or alkaline environment also limited the CaCO3 formation. These results illustrated that organic acid oxidation intracellularly, especially the tricarboxylic acid cycle, was the major mechanism, and the CaCO3 yield was related to the amount of CO2 produced by the metabolism of organic acids. These findings will deepen the knowledge of the mineralization capacity of S. cerevisiae and provide a theoretical basis for the future application of yeast as an alternative microorganism in MICP.
Introduction
Microbially induced calcium carbonate precipitation (MICP) is a common phenomenon in nature, and the formed calcium carbonate (CaCO3) has become a new green material used in numerous applications, including soil amelioration, building material rehabilitation, and the conservation of stone monuments (Dhami et al., 2013; 2014; Lin et al., 2021; Ortega-Morales and Gaylarde, 2021; Ortega-Villamagua, E., Gudiño-Gomezjurado, M. and Palma-Cando, 2020; Reeksting et al., 2020; Zhang et al., 2023). Urea hydrolysis is the major pathway for the application of MICP because of the higher and faster precipitation rate of CaCO3 (Reeksting et al., 2020; Justo-Reinoso et al., 2021; Lin et al., 2021). However, the associated byproducts, such as ammonia and nitrogen oxide, are environmentally toxic and have a negative impact on the substrate (Reeksting et al., 2020; Justo-Reinoso et al., 2021; Lin et al., 2021; Sidhu et al., 2022). To reduce the environmental impact of ureolytic strains, microorganisms with other pathways of CaCO3 production are also being tested in MICP applications. Oxidation of organic acids is also a pathway of MICP, and the final products are carbon dioxide and water, which are not harmful to the environment (Dhami et al., 2014). In addition, the precipitation of CaCO3 induced by the oxidation of organic acids takes place at a slower rate in comparison with the hydrolysis of urea, increasing the depth and efficacy of the restoration (Reeksting et al., 2020; Justo-Reinoso et al., 2021). Our previous study showed that Saccharomyces cerevisiae could induce CaCO3 precipitation through the oxidation of organic acids (Li and Li, 2022). This suggests that Saccharomyces cerevisiae can be an alternative microorganism for MICP applications.
As shown in Equations 1-3 (Dhami et al., 2014), when organic acids are the main carbon and energy source, their consumption will produce CO2 and increase the surrounding pH, and the presence of Ca2+ favors calcium precipitation as CaCO3. This process can occur both intracellularly and extracellularly and is influenced by several factors. For intracellular process, the type of organic acids and nutrients, pH and some metal ions can influence CaCO3 formation by affecting cell growth, organic acid uptake and inorganic carbon production (Wolf et al., 2003; Peña et al., 2015; Li and Li, 2022). In the extracellular process, proteins secreted by microorganisms consume the organic acids extracellularly to raise the pH and produce inorganic carbon for CaCO3 formation (Yafeng and Chunxiang, 2022). However, some organic matters from metabolites can inhibit the CaCO3 precipitation by competitive adsorption of calcium ions (Rui et al., 2021; Robles-Fernández et al., 2022). Our previous study has confirmed that the type of organic acids can influence the process of calcium carbonate precipitation induced by Saccharomyces cerevisiae, but the further mechanism of this process is not yet clear.
In the present study, we explored the factors influencing yeast-induced CaCO3 precipitation by adjusting different types of organic acid and the initial pH of the medium. In addition, yeast metabolites were used to degrade acetic acid in vitro to determine whether the metabolic process of organic acids occurs intracellularly or extracellularly. Finally, we compared the yield of CaCO3 produced by yeast under different factors. This study further elucidated the mechanism of yeast-induced CaCO3 precipitation and provided a theoretical basis for the application of yeast in MICP.
Materials and methods
Cultivation of Saccharomyces cerevisiae under various conditions
Instant active dry yeast, which is identified as S. cerevisiae, was purchased from Angel Yeast Co., Ltd (China). This strain is a common commercial product on the market, which has the advantages of easy availability and low cost, and it was activated and purified with B4 medium (3.52 g/L calcium acetate monohydrate, 4 g/L yeast extract, 10 g/L glucose). Seven organic acids or their calcium salts were used to culture yeast. The detailed composition of the modified B4 media is presented in Table 1 (a–g). The concentrations of Ca2+ and yeast in each media were 0.67 g/L and 1 × 105 CFU/mL, respectively, and the initial pH was adjusted to 6. In addition, two media, with initial pH of 7 (Table 1) h and 8 (Table 1) i, were also used to culture yeast.
Three parallel experiments were performed for each medium, and yeast was incubated at 30 C for 7 days. The final pH of the media was measured using an S210-K pH meter (Mettler Toledo, Switzerland). The biomasses were filtered with filter paper, washed with deionized water and absolute alcohol, and dried at 60 C to constant weight.
Comparison of CaCO3 yields from cultures with different organic acids
Three acids, acetate acid, pyruvic acid, and α-ketoglutaric acid, related to CaCO3 formation, were selected to culture yeast, and the biomass weight was used as an indicator of the yield of CaCO3 (Li and Li, 2022). Yeast was incubated as described 2.1, and the dry weight of the biomass and the final pH of the media were recorded.
The role of S. cerevisiae metabolites in organic acid oxidation
Yeast was cultured with the media (Table 1) a for 2 days, and then the media were filtered through 0.22 μm membranes. Afterward, 10 mL of a sterile solution of calcium acetate or calcium chloride (the concentration of Ca2+ was 0.2 mol/L) was mixed with the medium without yeast. These solutions continued to be cultured at the same condition for 5 days. Centrifugation (5,000 rpm and 10 min) was then carried out and the deposition that might be present were washed with deionized water and absolute alcohol, and dried at 60 °C to constant weight.
Characterization of S. cerevisiae-induced crystals
The biomasses obtained from the above experiments were analyzed with scanning electron microscope and energy-dispersive X-ray spectroscopy (SEM-EDX), X-ray diffraction (XRD) and Fourier-transform infrared spectroscopy (FTIR). After grinding, the morphological characteristics were observed via SEM (Quattro S, Thermo Fisher, United States), and the elemental composition of the minerals was analyzed via EDX (XFlash 6,160, Bruker, Germany). Both SEM and EDX were conducted under an accelerating voltage of 15 kV. The crystal of the formed minerals was characterized via XRD (D8 Advance, Bruker, Germany), and the samples were analyzed over the 2θ range of 10°–70° at a scan rate of 1°/min in 0.02° increments. Additionally, the biomass was analyzed via FTIR (Nicolet iN 10, Thermo Fisher, United States) with potassium bromide pellets to determine whether it contained the unmetabolized organic calcium.
Results and discussion
Factors influencing S. cerevisiae-induced CaCO3 precipitation
S. cerevisiae was cultured using seven organic acids, and minerals were widely present around the yeast cells cultured with all organic acids (Supplementary Figure S1). Most of the minerals were identified as calcium phosphate (Supplementary Figure S1, Figure 1B). The findings demonstrated that yeast had a high phosphatase activity and could secrete a large amount of phosphate outside the cell (Krumov et al., 2009; Krumov and Posten, 2011; Durán and Favaro, 2018; Qin et al., 2020; Geetha et al., 2021). CaCO3 was only found in the biomass cultured with acetate, pyruvate, and α-ketoglutarate (Figures 1A, 2). In addition, the pH results showed that the metabolism of acetate, pyruvate, α-ketoglutarate, and propionate increased the media from acidic to alkaline. Particularly, the pH of the media containing acetate or pyruvate was above 8. The alkaline environment and amount of dissolved inorganic carbon (DIC) are key factors affecting the process of CaCO3 formation by microorganisms (Li et al., 2018). It indicated that the consumption of acetate, pyruvate and α-ketoglutarate by yeast also provided sufficient CO2, the main source of DIC according to Equations 1–3, for CaCO3 formation. However, no CaCO3 was found in the biomass cultured with propionate, similar to our previous study with lactate (Li and Li, 2022). The failure of the metabolism of these organic acids to produce sufficient CO2 may be the main reason. The above results suggested that the type of organic acids was one of the key factors influencing yeast-induced CaCO3 mineralization.
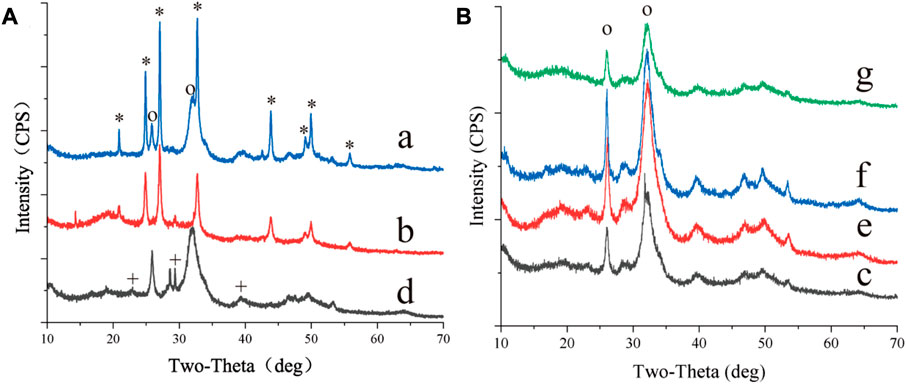
FIGURE 1. XRD of minerals synthesized by S.cerevisiae with different culturing conditions. (A) showed the results of the organic acids (a) acetate (b) pyruvate and (d) α-ketopentate in the medium, (B) showed the results of the organic acids (c) succinate (e) malate (f) formate (g) propionate in the medium. *, the base peak of vaterite, +, the base peak of calcite, o, the base peak of calcium phosphate.
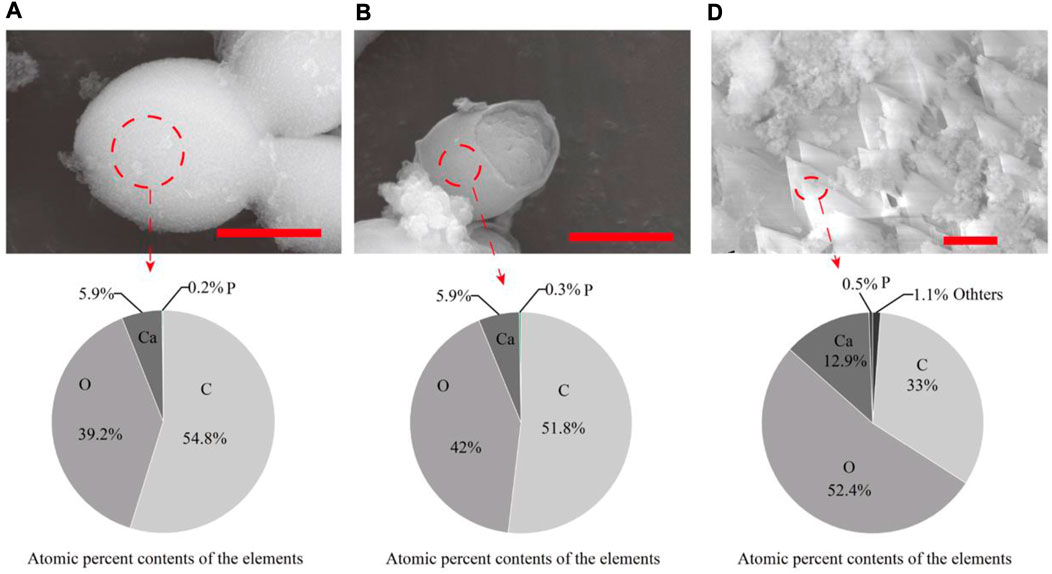
FIGURE 2. SEM-EDS of CaCO3 synthesized by S.cerevisiae with different culturing conditions. (A)acetate (B) pyruvate (D) α-ketopentate in the media. The red bar was 2 μm. The atomic percent contents refer to the elemental composition and proportion of minerals contained in the circled area.
S. cerevisiae was also cultured at different initial pH values, including 6, 7 and 8. However, the pH of the initial neutral and alkaline media decreased to 6.76 and 7.59, respectively, after 7 days of incubation, and no CaCO3 was found in the biomasses (Supplementary Figure S2). Peña et al. (2015) reported that the yeast growth rate remarkably decreased at pH 8.0. High pH also inhibits the uptake of acetate by yeast, but would not affect its metabolism (Mills, 1972; Pera et al., 1972; Peña et al., 2015). In addition, yeast has a high acidification capacity (Conway and Downey, 1950; Peña et al., 2015), and the medium was mainly acidified by CO2 produced by glucose metabolism (Peña et al., 2015). In the present study, neutral and alkaline environments inhibited yeast growth rate and acetate uptake, resulting in a reduction of OH− from acetate metabolism. In contrast, CO2 and organic acids produced by metabolic activities resulted in a small decrease in the pH of the medium. CO2 was mainly in the form of bicarbonate because the pH was less than 8.0, resulting in the nonexistence of CaCO3 in the biomasses. These suggested that acidic initial environment was one of the necessary conditions for CaCO3 precipitation induced by yeast.
Overall, yeast-induced CaCO3 precipitation was influenced by several factors. First, the presence of yeast extract is necessary for carbonate precipitation (Li and Li, 2022). Second, the initial media should be acidic. Lastly, organic acid is also required. When organic acid was used as the main carbon source, only acetate, pyruvate, and α-ketoglutarate contributed to CaCO3 biomineralization, and when glucose was used as the substrate, other organic acids such as lactate and pyruvate also contributed to the process.
Compared to other ureolytic and non-ureolytic microorganisms (Ortega-Villamagua, E., Gudiño-Gomezjurado, M. and Palma-Cando, 2020; Justo-Reinoso et al., 2021; Reeksting et al., 2020), the conditions for yeast-induced calcium carbonate deposition are somewhat limited, which consequently limits its potential application within MICP. However, yeast also has advantages in inducing CaCO3 precipitation. As one of the most widely used microorganisms in brewing and the production of food (Ma et al., 2011), yeast is inexpensive and readily available from the market. In addition, as a widely used model organism, gene modification techniques are well developed in yeast. For example, Barbero et al. (2013) have successfully edited the genes encoding carbonic anhydrase and mineralization peptides into yeast, significantly enhancing the ability to induce CaCO3 precipitation. From these perspectives, yeast can be considered as a potential candidate microorganism for MICP applications.
Metabolic pathway of S. cerevisiae-related MICP
The metabolites of yeast after 2-day cultivation were incubated with calcium acetate and calcium chloride, respectively for 5 days, but no precipitation occurred. This indicated that the consumption of organic acids by yeast only occurred intracellularly.
Transcriptome analysis in our previous study showed that the addition of organic acids could upregulate the genes in many pathways, including the tricarboxylic acid (TCA) cycle (Li and Li, 2022). All three acids capable of inducing calcium carbonate precipitation are associated with the TCA cycle. Acetic acid and pyruvic acid can be transferred to acetyl-CoA, the substrate of the TCA cycle. α-ketoglutaric acid is also an intermediate product in the TCA cycle. According to the pathway, one molecule of pyruvic acid generates three molecules of CO2, while one molecule of acetic acid produces two molecules of CO2, and one molecule of α-ketoglutaric acid only generates one molecules of CO2. We compared the CaCO3 production induced by the three organic acids. The yields of CaCO3 produced using the three organic acids increased in the following order: α-ketoglutarate < acetate < pyruvate (Supplementary Figure S3), similar to the case of CO2 yields.
Citric acid metabolism based on the TCA cycle can also produce CO2, but the low solubility of calcium citrate allows only a few citrates to enter the yeast cells. Our previous study showed that a large amount of calcium citrate occurred in the biomass after cultivation (Li and Li, 2022). The low utilization rate of citrate could not increase the pH of the medium and produce sufficient CO2 for CaCO3 precipitation. Succinic acid and malic acid are also intermediate products in the TCA cycle, and their calcium salts could be absorbed by yeast (Barnett and Kornberg, 1960). Fourier-transform infrared spectroscopy results showed that no calcium succinate and calcium malate occurred in the final biomass (results not shown). However, these acids neither increased the pH nor promoted CaCO3 formation. In the TCA cycle, the metabolism of these acids did not produce CO2. However, they can also produce CO2 via the glycolytic/glycogenic pathway, but this process does not involve the degradation of organic acids and does not produce OH−. These factors contributed to the inability of succinic acid and malic acid to contribute to yeast-induced CaCO3 precipitation.
The above results suggest that the TCA cycle is the main pathway related to yeast-induced CaCO3 precipitation and that organic acids oxidized to produce CO2 and OH− in this pathway are involved in the process (Figure 3, drawn by Figdraw).
Conclusion
The study suggested that organic acids such as acetic acid, pyruvic acid and α-ketoglutarate, as well as the initial acidic pH were the factors driving CaCO3 precipitation by yeast. The TCA cycle was the main pathway for yeast-included CaCO3 precipitation. Oxidation of organic acids was occurred intracellularly and the amount of CO2 produced in the process was related to the amount of calcium carbonate precipitated. This study further explored the mechanisms of S. cerevisiae in MICP and suggested that S. cerevisiae could be an alternative microorganism for MICP applications.
Data availability statement
The original contributions presented in the study are included in the article/Supplementary Material, further inquiries can be directed to the corresponding authors.
Author contributions
TL: Conceptualization, Funding acquisition, Methodology, Writing–original draft. HZ: Investigation, Validation, Writing–review and editing. XT: Investigation, Methodology. Writing–original draft. RZ: Investigation, Methodology, Writing–original draft. FW: Funding acquisition, Validation, Writing–review and editing. ZY: Validation, Writing–review and editing. BS: Conceptualization, Funding acquisition, Validation, Writing–review and editing.
Funding
The author(s) declare financial support was received for the research, authorship, and/or publication of this article. This work was financially supported by the Gansu Provincial Cultural Relics Protection Science and Technology Research Project (GSWW202220), Shandong Provincial Natural Science Foundation, China (ZR2020QC045), Science and Technology Plan of Gansu Province (21JR11RA218), Longyuan Young Talents Project of Gansu Province (No. 00151), National Natural Science Foundation of China Regional Innovation and Development Joint Fund (U21A20282).
Acknowledgments
We thank Qinglin Ma, Institute of Cultural Heritage, Shandong University for his help and continuous support. We thank LetPub (www.letpub.com) for its linguistic assistance during the preparation of this manuscript.
Conflict of interest
The authors declare that the research was conducted in the absence of any commercial or financial relationships that could be construed as a potential conflict of interest.
Publisher’s note
All claims expressed in this article are solely those of the authors and do not necessarily represent those of their affiliated organizations, or those of the publisher, the editors and the reviewers. Any product that may be evaluated in this article, or claim that may be made by its manufacturer, is not guaranteed or endorsed by the publisher.
Supplementary material
The Supplementary Material for this article can be found online at: https://www.frontiersin.org/articles/10.3389/fbioe.2023.1261205/full#supplementary-material
References
Barbero, R., Carnelli, L., Simon, A., Kao, A., Monforte, A., Riccò, M., et al. (2013). Engineered yeast for enhanced CO2 mineralization. Energy and Environ. Sci. 62, 660–674. doi:10.1039/c2ee24060b
Barnett, J. A., and Kornberg, H. L. (1960). The utilization by yeasts of acids of the tricarboxylic acid cycle. J. General Microbiol. 23, 65–82. doi:10.1099/00221287-23-1-65
Conway, E. J., and Downey, M. (1950). An outer metabolic region of the yeast cell. Biochem. J. 47, 347–355. doi:10.1042/bj0470347
Dhami, N. K., Reddy, M. S., and Mukherjee, A. (2014). Application of calcifying bacteria for remediation of stones and cultural heritages. Front. Microbiol. 5, 304. doi:10.3389/fmicb.2014.00304
Dhami, N. K., Reddy, M. S., and Mukherjee, M. S. (2013). Biomineralization of calcium carbonates and their engineered applications: A review. Front. Microbiol. 4, 314. doi:10.3389/fmicb.2013.00314
Duran, N., and Favaro, W. J. (2018). Biogenic synthesis of important environmental minerals: magnesium phosphate compounds and perspectives. Quimica Nova 41, 567–576. doi:10.21577/0100-4042.20170204
Geetha, N., Bhavya, G., Abhijith, P., Shekhar, R., Dayananda, K., and Jogaiah, S. (2021). Insights into nanomycoremediation: secretomics and mycogenic biopolymer nanocomposites for heavy metal detoxification. J. Hazard. Mater. 409, 124541. doi:10.1016/j.jhazmat.2020.124541
Justo-Reinoso, I., Heath, A., Gebhard, S., and Paine, K. (2021). Aerobic non-ureolytic bacteria-based self-healing cementitious composites: A comprehensive review. J. Build. Eng. 42, 102834. doi:10.1016/j.jobe.2021.102834
Krumov, N., Perner-Nochta, I., Oder, S., Gotchev, V., Angelov, A., and Posten, C. (2009). Production of inorganic nanoparticles by microorganisms. Chem. Eng. Technol. 32, 1026–1035. doi:10.1002/ceat.200900046
Krumov, N., and Posten, C. (2011). Metal nanoparticles in microbiology. Metal Nanoparticles Microbiol., 197–221. doi:10.1007/978-3-642-18312-6
Li, T., Hu, Y., and Zhang, B. (2018). Biomineralization induced by colletotrichum acutatum: A potential strategy for cultural relic bioprotection. Front. Microbiol. 9, 1884. doi:10.3389/fmicb.2018.01884
Li, Z., and Li, T. (2022). New insights into microbial induced calcium carbonate precipitation using Saccharomyces cerevisiae. Front. Microbiol. 13, 904095. doi:10.3389/fmicb.2022.904095
Lin, W., Lin, W., Cheng, X., Chen, G., and Ersan, Y. C. (2021). Microbially induced desaturation and carbonate precipitation through denitrification: A review. Appl. Sci. 11, 7842. doi:10.3390/app11177842
Ma, X., Chen, H., Yang, L., Wang, K., Guo, Y., and Yuan, L. (2011). Construction and potential applications of a functionalized cell with an intracellular mineral scaffold. Angew. Chem. 50, 7552–7555. doi:10.1002/ange.20110.0126
Mills, D. (1972). Effect of pH on adenine and amino acid uptake during sporulation in Saccharomyces cerevisiae. J. Bacteriol. 112, 519–526. doi:10.1128/jb.112.1.519-526.1972
Ortega-Morales, B. O., and Gaylarde, C. C. (2021). Bioconservation of historic stone buildings—An updated review. Appl. Sci. 11, 5695. doi:10.3390/app11125695
Ortega-Villamagua, E., Gudiño-Gomezjurado, M., and Palma-Cando, A. (2020). Microbiologically induced carbonate precipitation in the restoration and conservation of cultural heritage materials. Molecules 25, 5499. doi:10.3390/molecules25235499
Pena, A., Cinco, G., Gomezpuy, A., and Tuena, M. (1972). Effect of the pH of the incubation medium on glycolysis and respiration in Saccharomyces cerevisiae. Archives Biochem. Biophysics 153, 413–425. doi:10.1016/0003-9861(72)90359-1
Pena, A., Sanchez, N. S., Alvarez, H., Calahorra, M., and Ramirez, J. (2015). Effects of high medium pH on growth, metabolism and transport in Saccharomyces cerevisiae. FEMS Yeast Res. 15, fou005. doi:10.1093/femsyr/fou005
Qin, W., Wang, C. Y., Ma, Y. X., Shen, M. J., Li, J., Jiao, K., et al. (2019). Microbe-mediated extracellular and intracellular mineralization: environmental, industrial, and biotechnological applications. Adv. Mater. 32, 1907833. doi:10.1002/adma.201907833
Reeksting, B. J., Hoffmann, T. D., Tan, L., Paine, K., and Gebhard, S. (2020). In-depth profiling of calcite precipitation by environmental bacteria reveals fundamental mechanistic differences with relevance to application. Appl. Environ. Microbiol. 86, e02739–19. doi:10.1128/AEM.02739-19
Robles-Fernández, A., Areias, C., Daffonchio, D., Vahrenkamp, V. C., and Sánchez-Román, M. (2022). The role of microorganisms in the nucleation of carbonates, environmental implications and applications. Minerals 12, 1562. doi:10.3390/min12121562
Rui, Y., and Qian, C. (2021). The regulation mechanism of bacteria on the properties of biominerals. J. Cryst. Growth 570, 126214. doi:10.1016/j.jcrysgro.2021.126214
Sidhu, N., Goyal, S., and Reddy, M. S. (2022). Biomineralization of cyanobacteria Synechocystis pevalekii improves the durability properties of cement mortar. Amb. Express 12, 59. doi:10.1186/s13568-022-01403-z
Wolf, F. I., and Cittadini, A. (2003). Chemistry and biochemistry of magnesium. Mol. Aspects Med. 24, 3–9. doi:10.1016/S0098-2997(02)00087-0
Yafeng, R., and Chunxiang, Q. (2022). Characteristics of different bacteria and their induced biominerals. J. Industrial Eng. Chem. 115, 4490465. doi:10.1016/j.jiec.2022.08.032
Keywords: Saccharomyces cerevisiae, microbially induced calcium carbonate precipitation, TCA cycle, initial pH, organic acids
Citation: Li T, Zhang H, Tan X, Zhang R, Wu F, Yu Z and Su B (2023) New insights into Saccharomyces cerevisiae induced calcium carbonate precipitation. Front. Bioeng. Biotechnol. 11:1261205. doi: 10.3389/fbioe.2023.1261205
Received: 19 July 2023; Accepted: 22 August 2023;
Published: 31 August 2023.
Edited by:
Rongzhen Zhang, Jiangnan University, ChinaReviewed by:
Santosh Kumar Karn, Sardar Bhagwan Singh University, IndiaJaunius Urbonavičius, Vilnius Gediminas Technical University, Lithuania
Qianwei Li, China University of Petroleum, China
Copyright © 2023 Li, Zhang, Tan, Zhang, Wu, Yu and Su. This is an open-access article distributed under the terms of the Creative Commons Attribution License (CC BY). The use, distribution or reproduction in other forums is permitted, provided the original author(s) and the copyright owner(s) are credited and that the original publication in this journal is cited, in accordance with accepted academic practice. No use, distribution or reproduction is permitted which does not comply with these terms.
*Correspondence: Bomin Su, c3Vib21pbmdAaG90bWFpbC5jb20=