- 1Department of Orthopedics, The Affiliated Huai’an Hospital of Xuzhou Medical University, Huai’an Second People’s Hospital, Huaian, Jiangsu, China
- 2Department of Nephrology, The Affiliated Huai’an Hospital of Xuzhou Medical University, Huai’an Second People’s Hospital, Huaian, Jiangsu, China
At present, the clinical treatment of osteomyelitis and osteomyelitis-induced bone defects is challenging, easy to recur, drug toxic side effects, secondary or multiple surgeries, etc. The design of biodegradable composite biomaterials to improve antibiotics in the local precise anti-infection at the same time to complete the repair of bone defects is the current research hot spot. Herein, a composite hydrogel with a double bond at the end (FA-MA) was prepared by affinity addition reaction between fish collagen (FA) and methacrylic anhydride (MA) under photoinitiator initiation conditions, then, FA-MA was amino-activated by EDC/NHC, and vancomycin was attached to FA-MA via amide bonding to prepare FA-MA-Van hydrogels, and finally, the composite hydrogel microspheres were prepared by microfluidic technology. The structure of the hydrogel was confirmed by SEM (elemental analysis), optical microscopy, FTIR, and XPS to confirm the successful preparation. The composite hydrogel microspheres showed the better antimicrobial effect of hydrogel microspheres by bacterial coated plate experiments and SEM morphology results, with the antimicrobial class reaching 99.8%. The results of immunofluorescence staining and X-ray experiments showed that the hydrogel microspheres had a better effect on promoting bone repair. This engineered design of hydrogel microspheres provides clinical significance for treating osteomyelitis at a later stage.
1 Introduction
Chronic osteomyelitis is a chronic suppurative inflammation caused by the invasion of pathogenic bacteria into the body and the continuous destruction of bone and surrounding cartilage (El-Hadidi et al., 2018; Masters et al., 2019; Wu et al., 2022). The main routes of infection are blood-borne and traumatic. With the improvement of medical conditions and the quantitative use of antibiotics, patients with chronic blood-borne osteomyelitis gradually decrease. On the contrary, with the rapid development of society, people in high-risk industries such as transportation and construction are prone to fracture, so many internal plants need to be implanted to fix the fracture. The implantation of interior plants increases the infection rate of acute osteomyelitis by 30% (Abulaiti et al., 2022). Without adequate and timely treatment, acute osteomyelitis will gradually evolve into severe chronic traumatic osteomyelitis, whose incidence rate accounts for 80% of osteomyelitis (Zhao and Ferguson, 2020; Wassif et al., 2021; Özkan et al., 2021). Chronic traumatic osteomyelitis is a complex problem in orthopedic treatment due to its complicated condition, long treatment course, uncomplicated fracture, bone defect, poor surgical effect and infection recurrence rate.
The traditional treatment of osteomyelitis is debridement with large doses and the prolonged course of systemic application of antibiotics repeatedly (Cortés-Penfield and Kulkarni, 2019; Metsemakers et al., 2020; Tao et al., 2020). However, due to the poor blood circulation at the focus, the blood drug concentration in bone tissue is less than 20% (Masters et al., 2019; Mirzaei et al., 2020; Nasser et al., 2020), which is difficult to reach the bactericidal concentration. Secondly, reachingthe bactericidal concentration in time is difficult, and the barrier effect of bacterial biofilm is also tricky to effectively kill bacteria (Cobb et al., 2020). In recent years, studies have shown that the refractory infection of osteomyelitis is mainly caused by bacterial biofilm formation (Thonse and Conway, 2007; Jingshu et al., 2018; Jia et al., 2020). The biofilm protects the bacteria from the intervention of antibiotics (Wang et al., 2023), which leads to the “subclinical” state of the focus and is easy to relapse. It is imperative to develop innovative treatment methods. At present, a large number of antibiotic sustained-release materials have been developed to treat osteomyelitis (Hasan et al., 2019; Xu et al., 2021; Feng et al., 2022; Gritsch et al., 2022; Wu et al., 2022), but the treatment effect is still not ideal and the recurrence rate is high. Zhou (Zhang et al., 2023) et al. reported Vancomycin-loaded silk fibroin microspheres in an injectable hydrogel for chronic osteomyelitis therapy, demonstrating a sustained-release profile and good biocompatibility, making it promising for application in osteomyelitis treatment. Ju (Ju et al., 2023) reported a novel Zn2+ incorporated composite polysaccharide microspheres for sustained growth factor release and wound healing. The hydrogel microspheres noted above have good value in treating osteomyelitis and wound infection.
Topical antibiotic sustained release systems have emerged as a new therapeutic modality to address this problem better. This treatment modality’s success is mainly because it provides a sustainable and effective bactericidal concentration at the local level of infection, while avoiding the toxicity of drugs associated with systemic antibiotic therapy. Moreover, local antibiotic drug concentrations can exceed systemic intravenous drug concentrations by a factor of fish collagen,a polymeric protein extracted from fish scales that is non-toxic and biocompatible. It is mainly used as a cosmetic material (Trilaksani et al., 2020; Martins et al., 2023). Due to its unique structure, fish collagen can also be used for the preparation of hydrogel microspheres by chemical cross-linking, for the slow release of drugs, and has some bone repair properties (Eap et al., 2014; Zhou et al., 2020; Oh et al., 2021; Furtado et al., 2022). Vancomycin can inhibit the growth and reproduction of bacteria to kill them. It mainly interferes with the peptide, a vital component of the bacterial cell wall structure, thus affecting the formation of the cell wall and inhibiting the production of phospholipids and peptides in the cell wall. It is mainly used in the treatment of drug-resistant bacterial infections, and has a good therapeutic effect on Staphylococcus aureus, Streptococcus pyogenes, etc. (Kaur et al., 2019; Li et al., 2022; Liu et al., 2022; Xie et al., 2022).
Microfluidics is a science and technology that precisely controls and manipulates micro-scale fluids, with the main characteristics of manipulating fluids in micro-nano-scale space. This technology can prepare monodisperse and uniform hydrogel microspheres (Li et al., 2021; Wang et al., 2021; Illath et al., 2022). The latter is often used as a biodegradable delivery system for carrying and releasing drugs and is widely used in clinical and scientific research fields. In this paper, firstly, methacrylate glycosides were used to modify fish collagen through chemical bonds, and then vancomycin and modified fish collagen were prepared into hydrogel microspheres (FA-MA-Van) by microfluidic technology for the treatment of osteomyelitis. FA-MA-Van can gradually release antibacterial vancomycin at the lesion site, and the degraded fish collagen can also synergistically promote bone repair (Scheme 1).
2 Experimental section
2.1 Materials
Fish collagen, the methacrylic anhydride were obtained from Acros Co., Ltd. Vancomycin was purchased for Sigma Co., Ltd. Absolute ethanol and all the other reagents were obtained from J&K Co., Ltd. Serum, Bovine Serum Albumin (FBS), Glutaraldehyde was purchased Titan Co., Ltd. Ultra-pure water was utilized throughout this work.
2.2 Instrumental
At a resolution of 1 cm-1, FTIR spectra were collected from KBr discs at room temperature on Nicolet IS50 spectrophotometer, and 32 scans were performed from 4,000 to 400 cm-1.1H NMR spectra were obtained on a Bruker 600 MHz instrument. The surface morphologies of the obtained materials were observed by field emission scanning electron microscopy (FESEM). A 1 mg/mL microsphere particle dispersion was prepared, and a few drops of the solution were added dropwise to the silicon wafer. After drying, the sample was fixed on the sample stage with conductive tape for SEM observation. Hydrodynamic particle size testing of different nanomaterials using dynamic light scattering (DLS). Three samples were dispersed in deionized water, filtered and placed in glass vials for testing. Use a Zeta Potential Analyzer to perform Zeta Potential testing on products at various stages in the material synthesis process. Nikon eclipse Ni-U was used to observe the morphology, size and uniformity of cells and microspheres. Bilang Freeze Dryer (FD-2B) is used to freeze dry FA-MA-Van hydrogel, composite hydrogel FA-MA-Van and hydrogel microspheres.
2.3 Preparation of FelMA
Mix 30 g of fish collagen and 300 mL of PBS well and stir for 2 h–4 h to completely dissolve the fish collagen, then stir well again in a water bath at 60°C until the fish collagen is completely dissolved and swelled. 16 mL of methacrylic anhydride was slowly added at a rate of 0.25 mL/min using a micro-stirring pump and allowed to react for 2 h. Add 800 mL aliquots of PBS to stop the reaction. Then, the FA-MA solution was transferred to a dialysis bag and dialyzed in deionized water at 38°C for 10 min, and the gel was frozen and dried for 3–4 days using a freeze dryer.
2.4 Preparation of FA-MA-Van
Firstly, the carboxy-activator was configured as required (EDC-NHS, 200 mL of water 1.95 g of MES, dissolved and pH adjusted to 5.5, NaCl 2.9 g, NHS 0.69 g, EDC 2.3 g, fully dissolved), followed by adding 10 mg vancomycin and 1 g FMMA to 50 mL of carboxy-activator, and the reaction was carried out at 45°C under stirring The reaction was carried out overnight at 45°C to prepare the product FA-MA-Van, which was covalently linked by carboxyl and amino groups, and the reaction product was dialyzed with deionized water for 3 days. The liquid sample was lyophilized to obtain yellow porous FA-MA-Van, sealed, and stored at −20°C.
2.5 Preparation of FA-MA-van microspheres
FelMA-Val microspheres were prepared using microfluidic technology. 5% Tween 80 can be used as the microsphere surfactant, and the oil phase is mineral oil to make the shape of the microspheres more stable. Mineral oil was dissolved in deionized water and 0.5% photoinitiator was added. The oil phase can continuously cut off the water, allowing the FelMA-Val to form droplets, which are then transferred from the outlet to the frozen Petri dish. After irradiation under UV light (365 nm, 30 min), FA-MA-Van droplets can be photocrosslinked to form solid gel microspheres. Wash the microspheres repeatedly with 75% ethanol and acetone to remove surfactants and mineral oil. The washed microspheres were kept at −80°C for 3 h, and then FA-MA-Van microspheres were freeze-dried for 72 h (Scheme 2).
2.6 Cell culture and cell imaging
Bone mesenchymal stem cells (BMSCs) was used in this study, The cell lines present in this study were obtained from Chinese Academy of Sciences cell bank. Cells were seeded in DMEM, supplemented with 10% fetal bovine serum (FBS) at 37°C in a 5% CO2 atmosphere. Then, the cells were cultured in a glass bottom Petri dish having a diameter of 35 mm and allowed to adhere 24 h. Firstly, the cells were washed three times with PBS buffer, FA-MA-Van was added, and incubation was continued for 3 days in a 37°C incubator (5% CO2), then the cells were washed three times with PBS, and experimental cells were imaged by inverted fluorescence microscope. Intracellular fluorescence imaging experiments were performed in living cells by means of inverted fluorescence microscopy with × 20 objective lens.
The cytotoxicity of the FA-MA-Van was studied by methylthiazole diphenyltetrazolium bromide (MTT) method. Hela cells grown in log phase were cultured into 48-well plates at a density of 1,000 for 72 h, and cultured at 37°C and 95% air 5% CO2 for 12 h, 24 h and 48 h, respectively, and the MTT assay was used as described. Each cytotoxicity experiment was reported three times.
2.7 In vivo experiments
Twenty-four hours after the mice were injected, the peripheral serum and urine of the mice were collected to detect the content of Alanine aminotransferase (ALT), creatinine (Cr) and creatine kinase (CK) to evaluate the heart, liver and kidney functions of the mice. At the same time, after 7 days of continuous administration, mice were euthanized using carbon dioxide inhalation according to the laboratory animal-guidelines for euthanasia of the People's Republic of China (GB/T 39760-2021). Then mice were dissected, and the heart, liver, spleen, stomach and kidney were taken for HE staining to evaluate the toxicity of the material. Useing anesthesia in our study, Intraperitoneal injection of 10% Chloral hydrate (0.15 ml/10 g).
3 Results and discussions
3.1 Synthesis and characterization of FA-MA-van
FAMA-Van was prepared by using modified fish collagen and vancomycin through microfluidic technology. The structure was characterized by fourier transform infrared, X-ray photoelectron spectroscopy (XPS) and optical microscopy. It can be seen from Figure 1A that for FA-MA, the stretching vibration absorption peak of OH at 3,490 cm-1, 2,950 cm-1 and 2,867 cm-1 are the stretching vibration absorption peaks of alkyl and alkene, 1720 cm-1 is the stretching vibration absorption peak of carbonyl group (C=O), 1620,1587,1432 cm-1 is the skeleton vibration absorption peak of benzene ring, 1,105 cm-1 is the stretching vibration absorption peak of C-O. For the FAMA-Van microspheres, 3,487 cm-1 is the stretching vibration absorption peak of OH, 3,125 cm-1 is the stretching vibration absorption peak of -NH2, 2,962 cm-1 and 2,875 cm-1 are the stretching vibration absorption peaks of alkyl and alkene, and 1,643 cm-1 is carbonyl group (C=O) stretching vibration absorption peak, 1,604 cm-1 is the skeleton vibration absorption peak of C=N double bond, 1,025 cm-1 is C-Cl stretching vibration absorption peak and demonstrated that FAMA-Van was prepared successfully. The XPS spectra of FAMA-Van microspheres and XPS spectrum after Cl peak separation were also shown the microspheres was successfully synthesized (Figures 1B, C). Meanwhile, XPS analysis determined confidently the chemical composition of FA-MA-Van hydrogel microspheres.
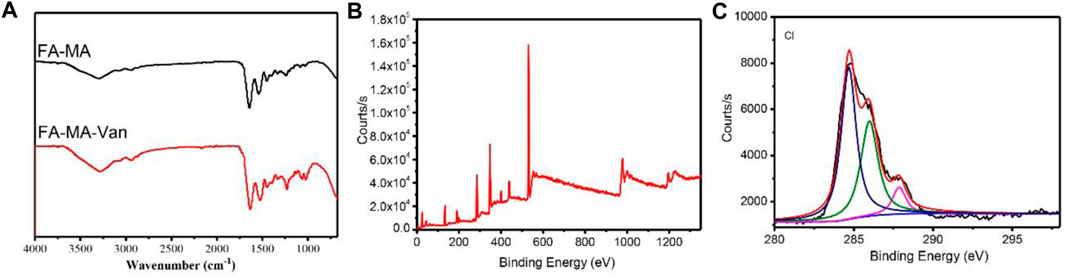
FIGURE 1. (A) Fourier transform infrared spectroscopy of FA-MA and FAMA-Van, (B) XPS of FAMA-Van, (C) XPS spectrum after Cl peak separation.
Figure 2 was a micrograph of the composite microspheres swelled in PBS solution. The hydrogel microspheres have good morphology and are relatively uniform. Figures 3A, B showed the overall and partial magnified images of the lyophilized FA-MA and FA-MA-Van microspheres by scanning electron microscope (SEM). Scanning electron microscopy observed that the freeze-dried microspheres were porous. In addition, SEM showed that the average particle size of FA-MA-Van microspheres is 200 ± 15 nm. Therefore, energy dispersive X-ray spectroscopy (EDS) showed that FA-MA-Van microspheres had uniform C, N, O, Cl element distribution and FA-MA-Van microspheres had uniform magnesium element distribution. At the same time, the release curve of PBS and FA-MA-Van was tested, and the results showed that the drug reached its highest value within 4 days and could last for 25 days.
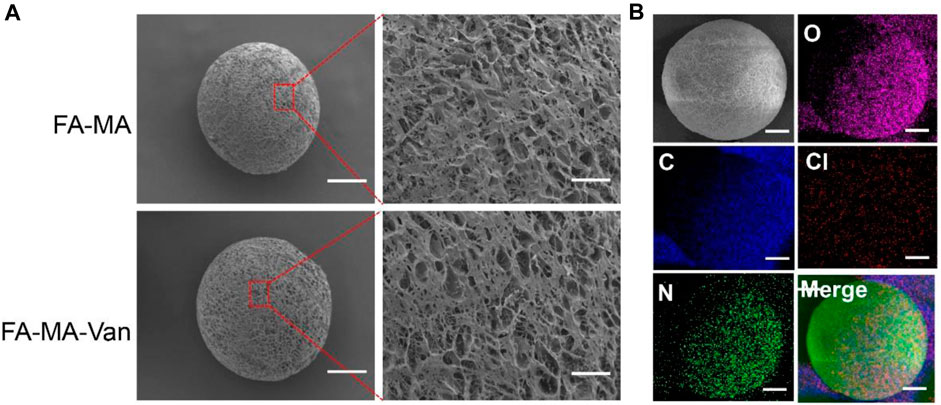
FIGURE 3. (A) SEM image and element distribution, (B) The mapping spectrum analysis of hydrogel microspheres, Scale: 50 μm.
3.2 Cytocompatibility and proliferation evaluation of FA-MA-van
In order to verify the cytotoxicity of FA-MA Val hydrogel microspheres on bone marrow mesenchymal stem cells (BMSCs) in vitro, PBS, FA-MA and 1 μ M FA-MA-Van and BMSCs were co-cultured, and the cultured cells were subjected to live death staining (Figure 4A), CCK-8 detection (Figure 4B) and cell fluorescence counting analysis (Figure 4C) on the 1st, 3rd and 5th day respectively. The results showed that the cell density of the three groups increased with the increase of culture time, and almost all the cells in each group were alive, without obvious dead cells. Compared with the control group, the cell proliferation activity of FA-MA group and FA-MA-Van group were significantly different at each time point, and the cell proliferation activity of FA-MA-Van group was much higher than that of the previous two groups, showing an amazing proliferation-promoting ability. FA-MA group did not promote cell proliferation as FA-MA-Van group, which may be due to the fact that the microsphere and cell co-culture did not release antibacterial vancomycin. In general, the above results show that the materials in this study have good biocompatibility with BMSCs and can effectively promote cell proliferation.
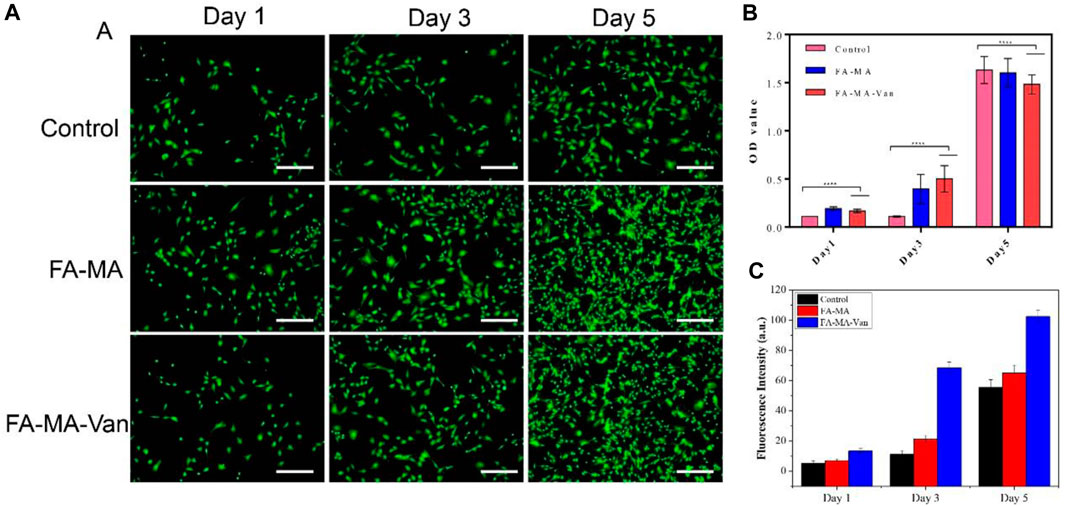
FIGURE 4. (A) Representative fluorescence images of control, FA-MA and FA-MA-Val co-cultured BMSCs by live-dead staining assay; (B) Cell proliferation of control, FA-MA and FA-MA-Van; (C) Fluorescence statistics of control, FA-MA and FA-MA-Van.
In order to further verify the proliferation effect of FA-MA Val hydrogel microspheres on BMSCs cells, the cell scratch test was tested. First, BMSCs cells were inoculated and cultured in a 12-well plate, and the tip of the pipette was scratched to form a wound. Then add the medium containing different ingredients to culture for 0 h, 12 h, and 24 h. It can be seen from Figure 5 that after 24 h of culture, FA-MA-Van has obvious migration wounds. In contrast, the wound area of FA-MA-Val group is 14.50%, significantly lower than that of other groups. (This is the use of scratches to simulate the wound at the cell level) This shows that FA-MA-Van microspheres have the proliferation effect on BMSCs, which is very consistent with the cell compatibility.
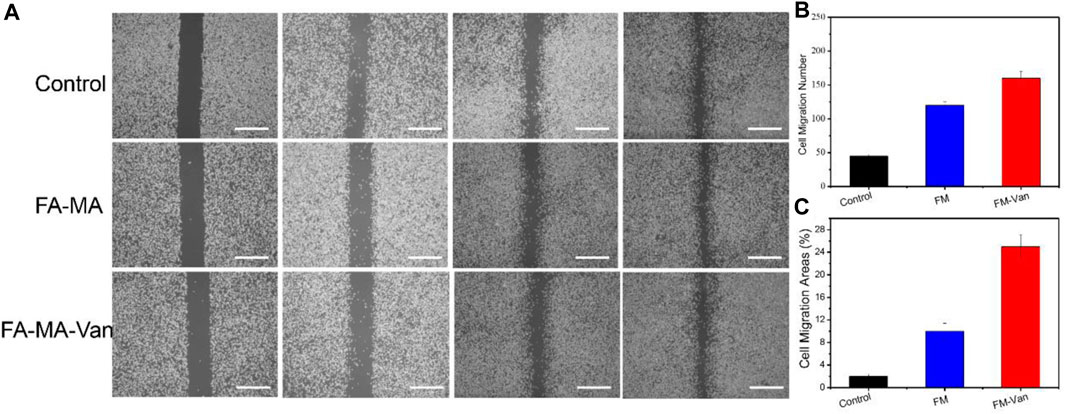
FIGURE 5. (A) BMSCs cell scratch test of FA-MA and FA-MA-Van with concentration 1uM; (B) The number of cell migration in cell scratch, (C) Cell migration region in cell scratch, scale: 50 μm.
3.3 Antibacterial properties of FA-MA-van
After incubating the bacterial solution on the hydrogel material overnight, take out 200 μL solution, drop it on the solid medium, apply it to the plate and incubate it at constant temperature overnight, and observe the surface bacterial colony. The results showed (Figure 6A) that the number of colonies in the hydrogel containing Van was less than that in the hydrogel without Van. The colony number of Staphylococcus aureus gradually decreased with the increase of Val release. There was no obvious Staphylococcus aureus on the agar plate surface after 6 h of release. This result confirmed that FA-MA-Val has antibacterial effect. It is further shown by the live and death staining of bacteria (Figure 6B) that when the bacteria are cultured with FA-MA-Van, all the bacteria have died. This phenomenon is consistent with the above, which indicates that FA-MA-Val has antibacterial effect.
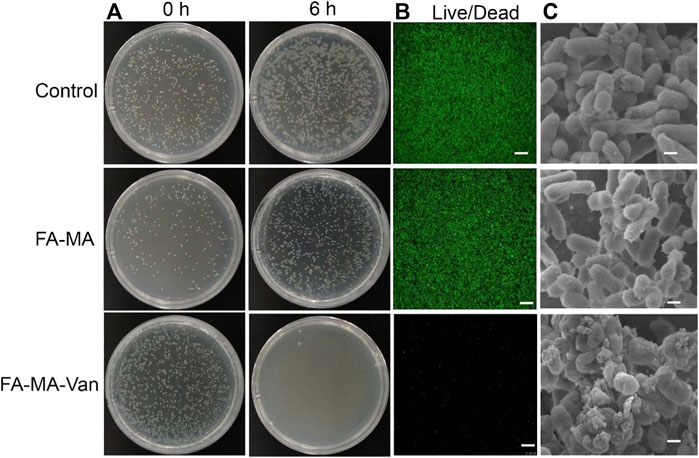
FIGURE 6. FA-MA-Val antibacterial test. (A) Optical image of Staphylococcus aureus. (B) Live-death staining image of bacteria. (C) SEM image of bacteria. Scale: 50 μm.
In order to further study the mechanism of bacterial growth in FA-MA-Van, the bacterial morphology was characterized. SEM results (Figure 6C) show that when Staphylococcus aureus is cultured on the hydrogel without Val, it presents a typical bacterial shape of complete spherical and rod-shaped structure. In contrast, when bacteria are cultured on FA-MA-Van hydrogel, the bacterial colony and structural integrity are greatly affected, and the bacterial colony is small or collapses. The results showed that FA-MA-Van significantly enhanced the antibacterial effect of the hydrogel.
3.4 Promote bone repair of FA-MA-van
It was verified by immunofluorescence that FA-MA-Van had a promoting effect on bone formation of BMSCs. TGF-β 3 is a crucial growth factor in inducing chondrogenic differentiation of stem cells, and also an important component in the chondrogenic induction medium of stem cells in vitro. It is widely used in various cartilage regeneration engineering scaffolds. However, the harsh storage conditions and high market prices of growth factors hinder their widespread application in clinical practice. This study aims to replace TGF-β 3 with fish collagen with more economic advantages and more stable properties for cartilage induction. The solution of FA-MA-Van was used for the induction and culture of BMSCs. Immunofluorescence staining was performed on the induction results to show the expression level of type I collagen in the cell mass (Figure 7A). In addition, DAPI and F-action staining were also performed on BMSCs cells. From Figure 7A, it can be found that the expression level of type I collagen in FA-MA-Van group is significantly higher than that in other groups, and the cytoskeleton and nucleus are damaged. It shows that it can promote osteogenesis. In vivo experiment, the rat osteomyelitis model was first established, and PBS, FA-MA and FA-MA-Van were injected into the joint cavity at the second week after operation. X-ray imaging of knee joint was performed at the 1st and 6th week after operation (Figure 8A). The HE staining and Masson Staining displayed the FA-MA-Van hydrogel microspheres can better repair osteomyelitis. It can be seen from the figure that FA-MA-Van group had the best recovery of focus.
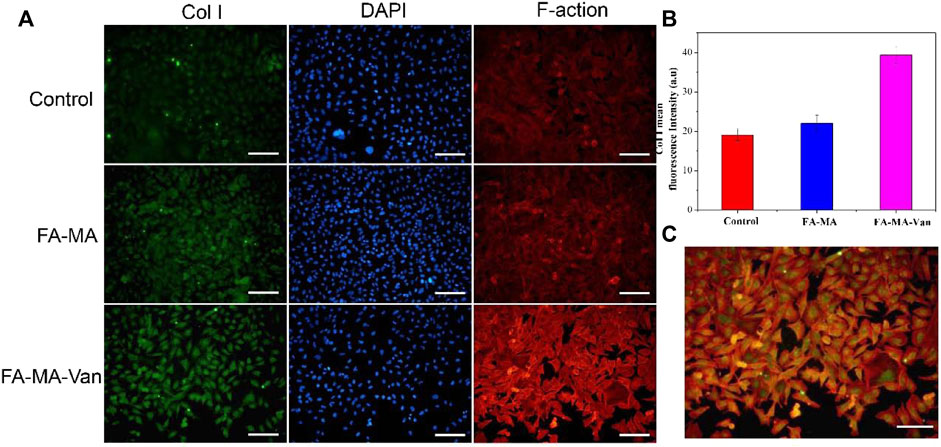
FIGURE 7. (A) Immunofluorescence images of BMSCs. (B) The fluorescence quantification of Col 1 collagen. (C) Merge diagram of red, blue, and green channels in the FA-MA-VAN group. Scale: 50 μm.
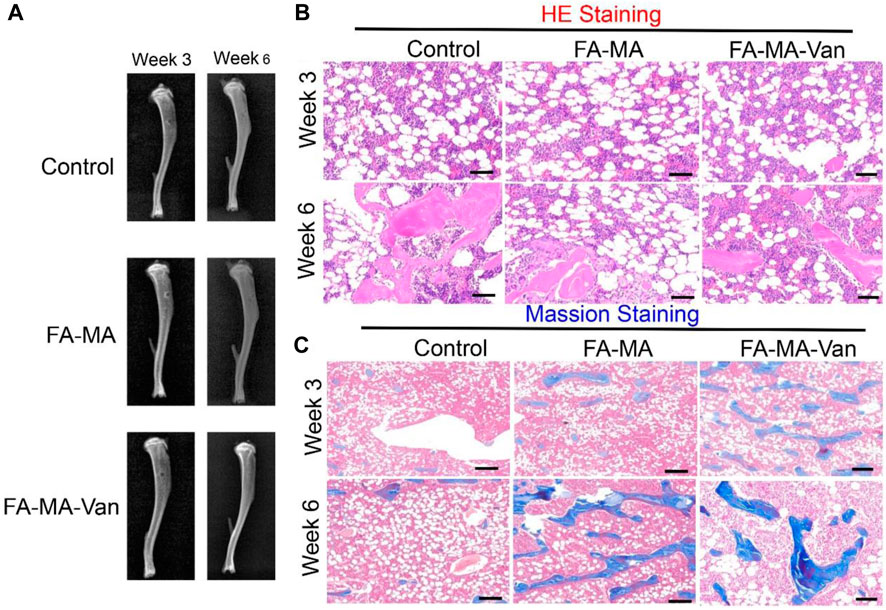
FIGURE 8. (A) X-ray image of mice week 3 and week 6; The (B) the HE staining (C) the massion staining for control, FA-MA and FA-MA-van with week 3 and week 6. Scale: 50 μm.
4 Conclusion
In conclusion, FAMA-Val microspheres were grafted with Van through condensation reactivity and ligand bonding. Thus, functional microfluidic FAMA-Val microspheres were constructed to endow hydrogel microspheres with active trapping, minimally invasive injection and continuous sustained release of Van, thus enabling antimicrobial effects as well as enhancing their ability to activate osteoblasts and endothelial cells. Apparently, grafting Val on FAMA microspheres gives FAMA-Val microspheres strong bacterial trapping and sustained release properties. In vitro experiments, FAMA-Val composite microspheres showed excellent bacterial capture and osteogenic potential, and X-ray experiments further demonstrated that FAMA-Val composite microspheres could promote regeneration of bone defects in rats, and bacterial culture experiments were able to kill bacteria indicating good antibacterial effect. The cell survival rate was higher than 80% even at high concentrations for 48 h, and the cytocompatibility was particularly good. The data from HE staining demonstrated that the material was not toxic in vivo. More importantly, the material has an excellent alleviating effect on osteomyelitis and an effective antibacterial effect.
Data availability statement
The original contributions presented in the study are included in the article/Supplementary Material, further inquiries can be directed to the corresponding author.
Ethics statement
The animal studies were approved by Ethics Committee of Huai’an Second Hospital. The studies were conducted in accordance with the local legislation and institutional requirements. Written informed consent was obtained from the owners for the participation of their animals in this study.
Author contributions
XH, JT, and DZ Conceptualization, Methodology, Data Curation Writing–Original Draft HY Investigation Resources Visualization XL Project administration. All authors contributed to the article and approved the submitted version.
Funding
This work was supported by Research project of Jiangsu Provincial Health Commission (M2021058).
Conflict of interest
The authors declare that the research was conducted in the absence of any commercial or financial relationships that could be construed as a potential conflict of interest.
Publisher’s note
All claims expressed in this article are solely those of the authors and do not necessarily represent those of their affiliated organizations, or those of the publisher, the editors and the reviewers. Any product that may be evaluated in this article, or claim that may be made by its manufacturer, is not guaranteed or endorsed by the publisher.
Supplementary material
The Supplementary Material for this article can be found online at: https://www.frontiersin.org/articles/10.3389/fbioe.2023.1249706/full#supplementary-material
References
Abulaiti, A., Liu, Y., Cai, F., Liu, K., Abula, A., Maimaiti, X., et al. (2022). Bone defects in tibia managed by the bifocal vs. trifocal bone transport technique: A retrospective comparative study. Front. Surg., 9, 858240, doi:10.3389/fsurg.2022.858240
Cobb, L. H., Mccabe, E. M., and Priddy, L. B. (2020). Therapeutics and delivery vehicles for local treatment of osteomyelitis. J. Orthop. Research® 38 (10), 2091–2103. doi:10.1002/jor.24689
Cortés-Penfield, N. W., and Kulkarni, P. A. (2019). The history of antibiotic treatment of osteomyelitis. Oxford, United Kingdom: Oxford University Press US.
Eap, S., Ferrand, A., Schiavi, J., Keller, L., Kokten, T., Fioretti, F., et al. (2014). Collagen implants equipped with ‘fish scale’-like nanoreservoirs of growth factors for bone regeneration. Nanomedicine Nanotechnol. Biol. Med. 9 (8), 1253–1261. doi:10.2217/nnm.13.122
El-Hadidi, T. T., Soliman, H. M., Farouk, H. A., and Radwan, M. (2018). Staged bone grafting for the management of segmental long bone defects caused by trauma or infection using induced-membrane technique. Acta Orthop. Belg. 84 (4), 384–396.
Feng, X., Lei, J., Ma, L., Ouyang, Q., Zeng, Y., Liang, H., et al. (2022). Ultrasonic interfacial engineering of MoS2-modified Zn single-atom catalysts for efficient osteomyelitis sonodynamic ion therapy. Small 18 (8), 2105775. doi:10.1002/smll.202105775
Furtado, M., Chen, L., Chen, Z., Chen, A., and Cui, W. (2022). Development of fish collagen in tissue regeneration and drug delivery. Eng. Regen. 3, 217–231. doi:10.1016/j.engreg.2022.05.002
Gritsch, L., Granel, H., Charbonnel, N., Jallot, E., Wittrant, Y., Forestier, C., et al. (2022). Tailored therapeutic release from polycaprolactone-silica hybrids for the treatment of osteomyelitis: antibiotic rifampicin and osteogenic silicates. Biomaterials Sci. 10 (8), 1936–1951. doi:10.1039/d1bm02015c
Hasan, R., Schaner, K., Schroeder, M., Wohlers, A., Shreffler, J., Schaper, C., et al. (2019). Extended release combination antibiotic therapy from a bone void filling putty for treatment of osteomyelitis. Pharmaceutics 11 (11), 592. doi:10.3390/pharmaceutics11110592
Illath, K., Kar, S., Gupta, P., Shinde, A., Wankhar, S., Tseng, F-G., et al. (2022). Microfluidic nanomaterials: from synthesis to biomedical applications. Biomaterials 280, 121247. doi:10.1016/j.biomaterials.2021.121247
Jia, C., Wang, X., Yu, S., Wu, H., Xie, Z., Huang, Q., et al. (2020). An antibiotic cement-coated locking plate as a temporary fixation for treatment of infected bone defects: A new method of stabilization. J. Orthop. Surg. Res. 15 (1), 44. doi:10.1186/s13018-020-1574-2
Jingshu, F., Xiaohua, W., Hongri, W., Jie, S., Ke, H., Shengpeng, Y., et al. (2018). Induced membrane technique combined with locking compression plate for the treatment of tibia infected defects. Chin. J. Orthop. 12.
Ju, Y., Zeng, H., Ye, X., Dai, M., Fang, B., and Liu, L. (2023). Zn2+ incorporated composite polysaccharide microspheres for sustained growth factor release and wound healing. Mater. today Bio 22, 100739. doi:10.1016/j.mtbio.2023.100739
Kaur, A., Preet, S., Kumar, V., Kumar, R., and Kumar, R. (2019). Synergetic effect of vancomycin loaded silver nanoparticles for enhanced antibacterial activity. Colloids Surfaces B Biointerfaces 176, 62–69. doi:10.1016/j.colsurfb.2018.12.043
Li, Q., Chen, S., Zhu, K., Huang, X., Huang, Y., Shen, Z., et al. (2022). Collateral sensitivity to pleuromutilins in vancomycin-resistant Enterococcus faecium. Nat. Commun. 13 (1), 1888. doi:10.1038/s41467-022-29493-0
Li, Z., Zhang, X., Ouyang, J., Chu, D., Han, F., Shi, L., et al. (2021). Ca2+-supplying black phosphorus-based scaffolds fabricated with microfluidic technology for osteogenesis. Bioact. Mater. 6 (11), 4053–4064. doi:10.1016/j.bioactmat.2021.04.014
Liu, T., Liu, W., Zeng, L., Wen, Z., Xiong, Z., Liao, Z., et al. (2022). Biofunctionalization of 3D printed porous tantalum using a vancomycin–carboxymethyl chitosan composite coating to improve osteogenesis and antibiofilm properties. ACS Appl. Mater. Interfaces 14 (37), 41764–41778. doi:10.1021/acsami.2c11715
Martins, E., Reis, R. L., and Silva, T. H. (2023). In vivo skin hydrating efficacy of fish collagen from Greenland halibut as a high-value active ingredient for cosmetic applications. Mar. Drugs 21 (2), 57. doi:10.3390/md21020057
Masters, E. A., Trombetta, R. P., De Mesy Bentley, K. L., Boyce, B. F., Gill, A. L., Gill, S. R., et al. (2019). Evolving concepts in bone infection: redefining “biofilm”,“acute vs. chronic osteomyelitis”,“the immune proteome” and “local antibiotic therapy”. Bone Res. 7 (1), 20. doi:10.1038/s41413-019-0061-z
Metsemakers, W-J., Morgenstern, M., Senneville, E., Borens, O., Govaert, G. A., Onsea, J., et al. (2020). General treatment principles for fracture-related infection: recommendations from an international expert group. Archives Orthop. Trauma Surg. 140, 1013–1027. doi:10.1007/s00402-019-03287-4
Mirzaei, R., Mohammadzadeh, R., Alikhani, M. Y., Shokri Moghadam, M., Karampoor, S., Kazemi, S., et al. (2020). The biofilm-associated bacterial infections unrelated to indwelling devices. IUBMB life 72 (7), 1271–1285. doi:10.1002/iub.2266
Nasser, A., Azimi, T., Ostadmohammadi, S., and Ostadmohammadi, S. (2020). A comprehensive review of bacterial osteomyelitis with emphasis on Staphylococcus aureus. Microb. Pathog. 148, 104431. doi:10.1016/j.micpath.2020.104431
Oh, G-W., Nguyen, V-T., Heo, S-Y., Ko, S-C., Kim, C. S., Park, W. S., et al. (2021). 3D PCL/fish collagen composite scaffolds incorporating osteogenic abalone protein hydrolysates for bone regeneration application: in vitro and in vivo studies. J. Biomaterials Sci. Polym. Ed. 32 (3), 355–371. doi:10.1080/09205063.2020.1834908
Özkan, S., Adanaş, C., Demir, C., and Hakan, H. (2021). The levels of oxidative dna damage and some antioxidants in chronic osteomyelitis patients: A cross-sectional study. Int. J. Clin. Pract. 75 (11), e14704. doi:10.1111/ijcp.14704
Tao, J., Zhang, Y., Shen, A., Yang, Y., Diao, L., Wang, L., et al. (2020). <p>Injectable chitosan-based thermosensitive hydrogel/nanoparticle-loaded system for local delivery of vancomycin in the treatment of osteomyelitis</p>. Int. J. Nanomedicine 15, 5855–5871. doi:10.2147/ijn.s247088
Thonse, R., and Conway, J. (2007). Antibiotic cement-coated interlocking nail for the treatment of infected nonunions and segmental bone defects. J. Orthop. Trauma 21 (4), 258–268. doi:10.1097/bot.0b013e31803ea9e6
Trilaksani, W., Adnyane, I., Riyanto, B., and Safitri, N. (2020). Nano collagen of the grouper swim bladder in compliance with quality standard of cosmetics materials. Bristol, United Kingdom: IOP Publishing.
Wang, J., Ma, P., Kim, D. H., Liu, B-F., and Demirci, U. (2021). Towards microfluidic-based exosome isolation and detection for tumor therapy. Nano Today 37, 101066. doi:10.1016/j.nantod.2020.101066
Wang, J., Wang, X., Liang, Z., Lan, W., Wei, Y., Hu, Y., et al. (2023). Injectable antibacterial Ag-HA/GelMA hydrogel for bone tissue engineering. Front. Bioeng. Biotechnol. 11, 1219460. doi:10.3389/fbioe.2023.1219460
Wassif, R. K., Elkayal, M., Shamma, R. N., and Elkheshen, S. A. (2021). Recent advances in the local antibiotics delivery systems for management of osteomyelitis. Drug Deliv. 28 (1), 2392–2414. doi:10.1080/10717544.2021.1998246
Wu, Y., Lin, Y., Cong, Z., Chen, K., Xiao, X., Wu, X., et al. (2022). Peptide polymer-doped cement acting as an effective treatment of MRSA-infected chronic osteomyelitis. Adv. Funct. Mater. 32 (7), 2107942. doi:10.1002/adfm.202107942
Xie, F., Jiang, L., Xiao, X., Lu, Y., Liu, R., Jiang, W., et al. (2022). Quaternized polysaccharide-based cationic micelles as a macromolecular approach to eradicate multidrug-resistant bacterial infections while mitigating antimicrobial resistance. Small 18 (12), 2104885. doi:10.1002/smll.202104885
Xu, Z., Xia, Y., Zhou, P., Li, J. J., Yang, M., Zhang, Y., et al. (2021). Silicon incorporation into hydroxyapatite nanocarrier counteracts the side effects of vancomycin for efficient chronic osteomyelitis treatment. Chem. Eng. J. 406, 126821. doi:10.1016/j.cej.2020.126821
Zhang, P., Sun, Y., Yang, H., Liu, D., Zhang, F., Zhang, Y., et al. (2023). Vancomycin-loaded silk fibroin microspheres in an injectable hydrogel for chronic osteomyelitis therapy. Front. Bioeng. Biotechnol. 11, 1163933. doi:10.3389/fbioe.2023.1163933
Zhao, Y., and Ferguson, P. J. (2020). Chronic non-bacterial osteomyelitis and autoinflammatory bone diseases. Clin. Immunol. Orl. Fla 216, 108458. doi:10.1016/j.clim.2020.108458
Keywords: fish collagen, vancomycin, microfluidic, osteomyelitis, boon
Citation: Hu X, Tang J, Yu H, Yang H, Lu X and Zheng D (2023) Preparation of fish collagen and vancomycin microspheres based on microfluidic technology and its application in osteomyelitis. Front. Bioeng. Biotechnol. 11:1249706. doi: 10.3389/fbioe.2023.1249706
Received: 09 July 2023; Accepted: 24 August 2023;
Published: 17 October 2023.
Edited by:
Kang-Nan Wang, National University of Singapore, SingaporeReviewed by:
Jiabing Ran, China Three Gorges University, ChinaHongyao Xu, Donghua University, China
Benhao Li, National University of Singapore, Singapore
Copyright © 2023 Hu, Tang, Yu, Yang, Lu and Zheng. This is an open-access article distributed under the terms of the Creative Commons Attribution License (CC BY). The use, distribution or reproduction in other forums is permitted, provided the original author(s) and the copyright owner(s) are credited and that the original publication in this journal is cited, in accordance with accepted academic practice. No use, distribution or reproduction is permitted which does not comply with these terms.
*Correspondence: Donghui Zheng, emRkd2pqQDEyNi5jb20=