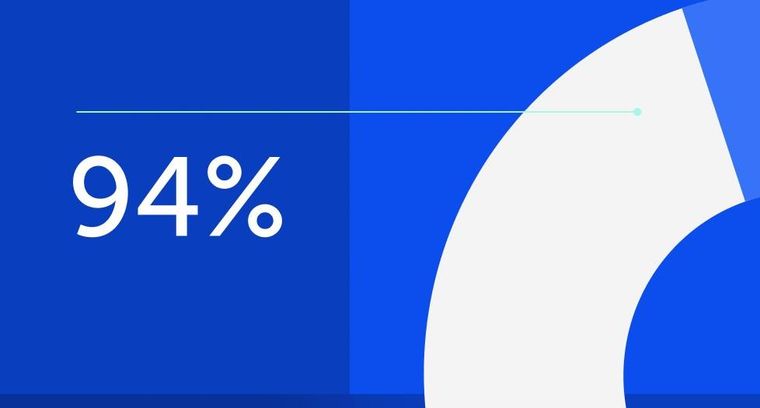
94% of researchers rate our articles as excellent or good
Learn more about the work of our research integrity team to safeguard the quality of each article we publish.
Find out more
REVIEW article
Front. Bioeng. Biotechnol., 29 November 2023
Sec. Bioprocess Engineering
Volume 11 - 2023 | https://doi.org/10.3389/fbioe.2023.1247711
Urban wastewater, as the main influent type of Waste Water Treatment Plants (WWTPs), has the characteristic of low carbon to nitrogen ratio (C/N). In the biological nitrogen removal (BNR) process, insufficient carbon source often affects the nitrogen removal efficiency and leads to more N2O emissions. We review recent researches on N2O emissions in the BNR process of wastewater with low C/N. The availability of carbon sources affects heterotrophic denitrification (HD) and autotrophic nitrification/denitrification processes, which are the main reasons for N2O emissions in BNR. For the sustainable development of BNR in WWTPs, we introduce strategies suitable for reducing N2O emissions in the BNR process of low C/N wastewater from two aspects: traditional process innovation and new process development. These strategies mainly include carbon source addition, adjustment of aeration strategy, optimization of oxidation ditch and biofilm facilities, and application of Anammox related processes. In the future, it is still necessary to further deepen this research direction through the normalization of N2O emission quantification standards, exploration of N2O metabolism mechanisms, assessment of environmental effects of emission reduction strategies, and practical application of new processes.
With the rapid pace of urbanization, urban wastewater (also known as domestic wastewater) has become the primary type of wastewater in sewage treatment, characterized by low water pollution load. Especially in regions with abundant rainfall, like in southern China, rainwater gets mixed with sewage in the pipe network, coupled with the illegal discharge of industrial wastewater, resulting in the prevalent issue of low carbon and high nitrogen in sewage (Hu et al., 2019). In recent decades, the influent of most Waste Water Treatment Plants (WWTPs) has exhibited a low C (chemical oxygen demand; COD)/N (total nitrogen; TN) ratio, indicating an insufficient carbon source (Zhang et al., 2018). The average C/N ratios for major cities such as Shanghai and Beijing are 3.3 and 4.0, respectively (Jin et al., 2014; Hao et al., 2015; Sun et al., 2016). Based on the comprehensive investigation results, urban wastewater typically has a COD concentration of less than 200 mg/L and a C/N ratio of less than 4 (Liang et al., 2015).
Excessive discharge of nitrogen and phosphorus in sewage can lead to eutrophication of the water system and harm the water environment. Biological nitrogen removal (BNR) is a primary method for reducing nitrogen emissions in WWTPs. Denitrification microorganisms in BNR usually compete with phosphorus-accumulating organisms (PAOs) for available carbon sources (Meinhold et al., 1999). Therefore, the denitrification efficiency of low C/N ratio urban wastewater is inhibited, making it a crucial research focus in the field of BNR in recent years. In order to improve the efficiency of BNR, the more robust methods are the improved Anaerobic/Anoxic/Oxic (A2/O), multi-stage Anaerobic/Oxic (A/O), Membrane Bio-Reactor (MBR) and Membrane Aeration Bioreactor (MABR) to support new BNR processes, such as partial nitrification (PN), partial denitrification (PD), simultaneous nitrification/denitrification, and anammox (Sun et al., 2010), which could reduce the addition of carbon source. Alternatively, adding carbon sources like brewery wastewater, kitchen waste leachate, and waste sludge can supplement the pre-treated wastewater carbon sources (Bodik et al., 2009; Wang et al., 2021).
However, while improving nitrogen removal efficiency, the potential issue of nitrous oxide (N2O) emission during BNR may have been overlooked in previous development processes, contributing to the global greenhouse effect (Bogner et al., 2008). According to the IPCC guidelines, global warming potentials (GWP) of N2O is 265 times that of CO2 over a 100 years time span (Edenhofer et al., 2014), and even lower emission fluxes can generate a considerable amount of carbon footprint. The direct emissions of N2O caused by anaerobic decomposition of organic matter and BNR during sewage treatment contribute to 3% of its total global emissions (Zawartka et al., 2020). Therefore, N2O emission reduction is of great significance for the further development of BNR processes.
Urban wastewater with a low C/N ratio is more likely to produce N2O than wastewater with a high C/N ratio. For example, in an activated sludge sequencing batch reactor (SBR), under the operating condition of Biochemical Oxygen Demand (BOD5)/TN ratio of 2.6, the total N2O emission in the denitrification stage is about 270 times higher than that when the BOD5/TN ratio is 4.5 (Kishida et al., 2004). For the A2/O process, increasing the influent C/N ratio significantly reduced N2O production during nitrification and denitrification (Yan et al., 2017). In the MBR, reducing the C/N ratio from 10 to 2 leads to a decrease in the efficiency of the denitrification process to 14.7% of the original, concurrently increasing N2O emissions in both gaseous and dissolved phases (Mannina et al., 2018a; Mannina et al., 2018b).
For new BNR methods that can improve the nitrogen removal efficiency of low C/N wastewater, like PN, some scholars found that N2O production in these methods may exceed the that of traditional BNR processes (Joss et al., 2009; Desloover et al., 2011; Domingo-Felez et al., 2014). Monitoring gas emissions from actual sized suspended sludge PN reactors, it can be known that N2O emissions account for 3.7% of the nitrogen load, while the formation of N2O during the anoxic stage accounts for 66% of N2O emissions (Mampaey et al., 2016). However, the maximum accumulation of N2O in the mainstream PD/A process was only 0.7% of the influent nitrogen, much lower than previously reported for conventional nitrification-denitrification or PN processes (Du et al., 2020).
Wastewater with a low C/N ratio is more likely to produce N2O during both traditional and new BNR processes. We aim to summarize the studies of N2O emission in BNR process of low C/N wastewater in recent years, clarify the impact and mechanism of the C/N ratio on N2O emission in different BNR methods, and provide suggestions for effective emission reduction strategies and future studies.
In recent years, a multitude of studies have examined N2O emissions in the BNR process of wastewater with varying C/N ratios (Table 1). Because each BNR process has different reaction principles and characteristics, we have categorized them into three groups: complete nitrification/denitrification, partial nitrification-denitrification (PN-D), and new BNR processes for discussion, and summarized the patterns of N2O emission from wastewater with different C/N ratios in various processes. Experimental evidence that urban wastewater with low C/N may have more N2O emission will be provided, laying the foundation for scientifically mitigating N2O emissions in BNR processes.
TABLE 1. Summary of N2O emissions and denitrification efficiency of wastewater with lower and higher C/N ratios in BNR processes.
Researchers began to investigate the impact of the C/N ratio on N2O production during BNR as early as around 2000. At first, the influent of urban wastewater was simulated at a laboratory scale to measure N2O. During the denitrification process of the mixed flow reactor, when the COD/NO3−-N ratio was 1.5 or 2.5, 3%–12% of the influent NO3−-N was converted into N2O. When the COD/NO3−-N ratio was 3.5 or 4.5, however, the N2O conversion rate dropped below 4% (Table 1). Additionally, an insufficient sludge retention time (SRT) was also likely to enhance N2O emissions (Hanaki et al., 1992). Studies of cyclic operation demonstrate that for intermittent aeration wastewater treatment systems, the maximum N2O emission rate occurs during the initial aerobic stage, not the anaerobic stage (Park et al., 2000).
For the intermittent aeration BNR process of high concentration wastewater, in a bioreactor with an influent COD/N ratio less than 3.5, 20%–30% of the influent nitrogen is discharged in the form of N2O; However, with the increase of the C/N ratio to 5.0–5.5, the N2O conversion rate dropped below 1% (Itokawa et al., 2001). The nitrification/denitrification process of aquaculture wastewater also has a similar trend. When the C/N ratio of wastewater increased from 2.6 to 4.5, the conversion rate of N2O to influent nitrogen decreased from 17.7% to 1.71% (Kishida et al., 2004).
With the advancements of BNR process, the hydraulic retention time (HRT) for nitrification and denitrification has progressively decreased from a maximum of 10 days to less than 1 day. In the complete nitrification process carried by the A/O SBR reactor, when the influent C/N increased from 7.5 to 14.5, the removal rates of TN and NH4+slightly increased, but the conversion rate of N2O (N2O-N/TN removed) decreased from 6.0% to 1.3% (Hu et al., 2013b). Researchers have also explored a broader range of C/N ratios. When the C/N ratios of synthetic wastewater were 6.5, 3.3, and 1.3, respectively, N2O accounts for 0.32%, 88.57%, and 16.97% of the nitrogen loss (TN loss) respectively (Yang et al., 2021), indicating that N2O emissions do not increase monotonicity as the C/N ratio of influent water decreases, and further in-depth mechanism analysis may be needed to explain the tendency of wastewater with low C/N ratios to have higher N2O or overall GHG emissions. For the nitrification and denitrification separation system, the ratio of N2O production rate to NO2− accumulation rate is defined as the N2O conversion rate (rN2O-N/rNO2−-N). When the C/N ratio was 4 and 1, the N2O conversion rate was 11.98% and 22.63%, respectively (Wu et al., 2014). The N2O emission factor during the nitrification stage ranged from 0.24% to 0.78%, while that of the denitrification stage decreased as the C/N ratio increased, spanning from 12.0% to 26.6%. According to the research findings of other independent nitrification and denitrification processes (Hanaki et al., 1992; Alinsafi et al., 2008; Lee et al., 2019), it can also be observed that the N2O emissions from the denitrification process are generally greater than those from the nitrification process. Different studies have different nitrogen indicators compared to the generated N2O when defining the N2O conversion rate for different processes. Generally, influent nitrogen content (TN load) and nitrogen removal (TN removed or TN loss) are taken as reference. Considering that most studies employ different statistical units for N2O emissions and emission rates, the N2O conversion rate can serve as a relatively unified quantitative standard for N2O emissions in BNR processes. However, when comparing emissions across different studies, it is necessary to take into account the variations in the conversion rate based on nitrogen indicators for scientifically accurate comparisons.
In addition, monitoring the dynamic changes in N2O emission concentration at different stages of the nitrogen removal process and paying attention to emission peaks can provide insights into the emission situation in wastewater treatment with different C/N ratios. In the process of nitrite denitrification, when COD/N was 6, the cumulative amount and duration of N2O were significantly lower than that when COD/N is 1 or 4. The peak N2O concentrations at COD/N ratios of 6 and 1 were 1.976 and 9.028 mg/L, respectively (Wang et al., 2019). These values can serve as a basis for characterizing emission characteristics.
Recently, N2O emissions during BNR of actual wastewater with different C/N ratios have also been monitored. Continuous monitoring of N2O emissions from SBR reactors in sewage treatment plants for 1 year revealed a significant positive correlation between N2O emissions and influent COD/N (R2 = 0.346, p = 0.044 < 0.05), except for August and September. A lower influent COD/N (less than 6) corresponded to a higher N2O emission (Sun et al., 2013). Similarly, in an A2/O bioreactor system, when the influent C/N decreased from 10.3/10.7 to 3.5/3.8, N2O-N conversion rate increased from 0.043%–0.061% to 6.15%–9.18% (Ren et al., 2015). The research on A2/O system in 2017 also found that N2O emission and generation and the total conversion rate of N2O-N decreased significantly with the increase of influent C/N. And N2O was mainly produced through the denitrification process in anaerobic and anoxic ponds (Yan et al., 2017) (Figure 1A).
FIGURE 1. N2O generation and emission in different processes. (A) Complete nitrification/denitrification (A2/O); (B) Partial nitrification/anammox (PN/A). The proportions of N2O emissions from each reactor depicted in the figure are average values based on measured values found in the relevant literature (refer to Tables 1–3). It is important to note that these estimates are provided for reference purposes only.
In order to improve nitrogen removal efficiency and reduce resource input, partial nitrification/denitrification (PN-D) process (or shortcut biological nitrogen removal-denitrification, SBNR-D) came into being. The BNR process involves partially oxidizing ammonia to nitrite and directly reducing nitrite to nitrogen (N2) without requiring complete oxidation through NO3−-N (Schmidt et al., 2003). PN reduces the oxygen required by 25%, and the carbon source required for denitrification by 40% (Tseng et al., 1998; Pollice et al., 2002), making it an economical alternative to the complete nitrification/denitrification process. Due to the low dissolved oxygen (DO) concentration and high NO2− concentration increasing N2O emissions in the PN process, which may attribute to heterotrophic denitrification. N2O emission characteristics may differ from those of the complete nitrification process (Gabarro et al., 2014).
In 2006, researchers measured N2O emissions from laboratory scale SBNR processes. For wastewater with a C/N ratio of 1, the process was able to remove 81.2% of TN. And its N2O is mainly produced in the anoxic denitrification section, but the emissions were over 90% lower than that of the complete nitrification process under the same conditions (Hwang et al., 2006). For the UASB reactor with a COD/NH4+ ratio of 1.6 treating the effluent of concentrated black water, PN exhibits a similar effect, with the N2O conversion rate accounting for 1.9% of the TN load (de Graaff et al., 2010). In the continuous aeration PN process, for the wastewater with a C/N ratio of 6, the N2O emissions were found to range from 18.67 to 330.09 μg/h, and the conversion rate (N2O-N/NH4+-N) was relatively low (0.42%) (Liu et al., 2021a), which also reflected the advantages of PN-D in reducing N2O emissions during wastewater treatment.
In recent years, in addition to complete nitrification/denitrification and PN-D to improve efficiency, several new BNR processes have been developed, such as Completely Autotrophic Nitrogen removal Over Nitrite (CANON) process, Coupled Aerobic-anoxic Nitrous Decomposition Operation (CANDO), Anammox related process, n-DAMO (Nitrate-dependent anaerobic methane oxidation) process, etc. These new processes minimize the demand for organic carbon and the oxygen required for nitrification (Horstmeyer et al., 2017), making them more suitable for treating wastewater with low C/N ratios.
In the BNR process dominated by anammox, the enrichment of anammox bacteria by anaerobic carrier biofilm enhanced the denitrification efficiency under C/N = 2.7–5 (Li et al., 2019). However, A2/O process combined with denitrification and anammox increased the denitrification efficiency of actual wastewater with low COD/N by about 16.9%. With enhanced denitrification, N2O emissions remained relatively low. In the A2/O process combined with anoxic carrier biofilm (AM-A2/O), the actual N2O conversion rate for wastewater with C/N ratios ranging from 1.2 to 7.9 was only 0.02%–0.08% (Li et al., 2019). The maximum N2O emission during sewage treatment with low COD/N (2.7 ± 0.4) was only 0.15 mg/L (Li J W et al., 2020). Recently, researchers have combined nitrification, PN and anammox into a comprehensive process, called INPDA (integrated nitrification, partial denitrification and anammox). The TN removal rate of this process could reach 94.1%, and the N2O conversion rate (N2O-N/TN load) for treating wastewater with a C/N of 2.5 is calculated to be 2.2% (Zhou et al., 2020). Compared with previous processes in A2/O or A/O sequencing batch biofilm reactors (SBBR), the above combined processes have lower N2O emissions when treating wastewater with low C/N.
For the CANDO process that focuses on eliminating NO2−, when the C/N ratio was 3, the N2O yield (N2O-N/NO2−-N) was 62.5%, and when the COD/N ratio was 5, the N2O yield decreased to 60.8%, accompanied by an increase in the nitrogen removal rate (Weissbach et al., 2018). In the unipolar CANON system, a similar pattern was observed. When the C/N ratio decreased from 1 to 0, the total N2O emissions increased from 1.32% to 1.62%. Comparisons reveal that carbon source has no significant impact on N2O emissions during hydroxylamine (NH2OH) oxidation and heterotrophic denitrification (HD). The enhancement of nitrifying bacteria denitrification in wastewater with lower C/N ratio is the primary reason for the rise in total N2O emissions in this process (Fang et al., 2020).
Summarizing the N2O emissions from BNR in different processes, it can be seen that the processes of low C/N wastewater treatment tend to result in higher N2O production, whereas new processes like anammox combined with PN or PD and treatment systems with added biofilm exhibit lower N2O conversion rates under low C/N conditions.
Based on the monitoring and research results of emissions, we found that low C/N wastewater tends to emit more N2O in BNR compared to high C/N wastewater, greatly enhancing the greenhouse effect of BNR process. Consequently, it is imperative to investigate the factors responsible for this pattern and understand how the decreased carbon load in urban wastewater affects the metabolic pathway of N2O.
Microbial-mediated denitrification plays a crucial role in the denitrification of wastewater. Nitrate (NO3−) and nitrite (NO2−) are reduced to harmless nitrogen gas (N2). This process is usually mediated by heterotrophic bacteria (HB), including four steps mediated by nitrate reductase (NAR), nitrite reductase (NIR), nitric oxide reductase (NOR) and nitrous oxide reductase (N2OR) respectively (Zumft, 1997). HB utilize carbon sources as electron donors. Specifically, after decomposing the carbon source in the environment, these denitrification bacteria can store the carbon source in the form of polyhydroxyalkanoates (PHAs) and glycogen in the anaerobic stage, as electron donor for endogenous denitrification, used for biomass production and anaerobic reduction of nitrogen oxides, and generate energy (Jetten et al., 1997; Oehmen et al., 2005; Miao et al., 2015).
When the denitrification process is not in optimal balance, the intermediate gaseous products nitric oxide (NO) and nitrous oxide (N2O) may be discharged into the environment. Among them, N2O is generated through the sequential action of NO3−, NO2−, and NO reductase (Richardson et al., 2009) (Figure 2). Taking Alcaligenes faecalis, a denitrification culture, as an example, N2O emissions are higher in the absence of carbon sources; When the electron donor is increased due to the addition of carbon source, the output of N2 increases, but the production of N2O does not increase (Schalk-Otte et al., 2000), which proves that HB may not be able to complete the denitrification process under the condition of insufficient carbon source, and incomplete denitrification makes a large amount of intermediate N2O produced. However, the inclusion of a carbon source facilitates complete denitrification and the generation of the final product, N2. It was observed in batch experiments that N2O accumulated at C/N ratios of 1.28 and 2.57, while complete denitrification occurred at C/N ratios of 5.14 and 12.85 (Lee et al., 2019).
FIGURE 2. The Mechanism of carbon source regulating nitrogen metabolism in heterotrophic denitrification.
In the process of denitrification, when the electron supply rate of the oxidation process cannot meet the demand for electrons of the four reduction steps, electronic competition will occur between the four reduction steps (Richardson et al., 2009), so the availability of electrons can adjust the activities of various denitrification enzymes. In research, PHAs are commonly used as the primary endogenous electron donor, serving as a bridge between the external carbon source and the electron utilization of denitrification enzymes. The electronic availability in the system can be known by monitoring the PHAs content, and the PHAs will increase with the increase of influent C/N, which will alleviate the competition of each denitrification process for carbon to some extent (Ge et al., 2018).
Specifically, N2OR competes with NOR and NIR for electrons. An additional electron supply can enhance the electron pool of cytochrome C, thereby reducing competitive pressure and boosting N2OR activity (Schalk-Otte et al., 2000). Therefore, under different carbon load conditions, it may be the electronic competition intensity between denitrification reductases, rather than the C/N ratio itself, that determines the N2O accumulation in the process of denitrification (Lu and Chandran, 2010).
Further experiments have demonstrated that electronic competition occurs not only under carbon-limiting conditions but also in situations with excessive carbon sources. This is because the various denitrification processes are interrelated. In the traditional concept, BNR may be carried out by a variety of organisms in sequence, for example, one denitrification bacterium converts nitrate into nitrite, and then another denitrification bacterium converts nitrite into nitrogen (Zumft, 1997). Is there no electronic competition between nitrate reductase and other reductases in subsequent steps of denitrification?
The current view is limited. The fact is that over 60% of the colonies in the denitrification enrichment belong to Sphingobacteriales and Flavobacteriales, and the bacteria of this order have complete nitrogen removal pathways (Caspi et al., 2012). All denitrification enzymes compete for electrons from a common electronic supply system. As the carbon loading rate changes, electrons will be differentially distributed among various reductases, and when the electron flux of reducing nitrite is greater than that of reducing N2O, N2O will accumulate (Pan et al., 2013a). In the same year, the research team developed an electron carrier model, indicating that carbon oxidation provides electrons for carriers, and nitrogen oxides receive electrons from these carriers for reduction. The carbon oxidation process and nitrogen reduction process are closely connected. This model enhanced the prediction of N2O accumulation ability during denitrification by employing different affinity constants and reduction carriers to describe the relative competitive ability of electrons in each denitrification step (Pan et al., 2013b).
Nitrous oxide reductase (N2OR) is the sole enzyme responsible for catalyzing the decomposition of N2O into N2, and the structural gene nosZ encoding N2OR is co-transcribed with nosR. N2OR is not only influenced by the electronic competitive activity of other denitrification reductases but is also highly sensitive to DO in the environment. The anoxic and anaerobic enrichment conditions will also lead to N2O accumulation (Conthe et al., 2018).
In addition to the contribution of HB, N2O emission from BNR processes are partially attributed to ammonia oxidizing bacteria (AOB) and ammonia oxidizing archaea (AOA) during the nitrification process in the mixed system. Under low C/N conditions, it was found that the contributions of heterotrophic denitrification activity and autotrophic nitrification activity to N2O production were similar. Therefore, some researchers have created a process model that includes both heterotrophic and autotrophic denitrification paths, resulting in a slightly better prediction effect for N2O emissions compared to the single-path denitrification model (Domingo-Felez et al., 2017). In the process of AOB metabolism, the oxidation of NH2OH produces a byproduct N2O (Chandran et al., 2011); similarly to HB, it uses ammonia or hydrogen as the electron donor to reduce nitrite, resulting in the production of N2O (Figure 3). This autotrophic ammonia oxidizing bacteria with denitrification ability is primarily classified as Nitrosomonas (Bock et al., 1995), and N2O is the end product of its nitrogen metabolism (Kim et al., 2010; Law et al., 2012). The model shows that in most cases, the AOB denitrification pathway is dominant, while the NH2OH oxidation pathway becomes more significant at high DO levels (e.g., 3.5 mg O2/L) (Peng et al., 2015a).
FIGURE 3. The main pathway of N2O emission and the role of carbon sources in autotrophic nitrification/denitrification.
Unlike heterotrophic organisms, the nitrogen metabolism pathway of autotrophic AOB bacteria is affected by the availability of inorganic carbon (IC). In nitrifying sludge rich in AOB and nitrite oxidizing bacteria (NOB), autotrophic growth can lead to a lack of IC, which in turn limits the oxidation activity of AOB towards NH3 (Todt and Dorsch, 2016). In the PN/A process, N2O is also produced through pathways associated with AOB. Decreasing IC/N will inhibit the activity of AOB, thereby enhancing N2O generation through NH2OH oxidation (Ma et al., 2015; Li L et al., 2020) (Figure 2). Enhancing the availability of IC will result in a higher reaction rate between AOB and related N2O (Peng et al., 2015b). However, further research is needed to elucidate the mechanism of N2O generation under different IC conditions, and more evidence is required to support the IC/N concentration that can achieve N2O emission reduction in actual wastewater treatment.
In the treatment of low C/N wastewater, certain specific conditions can cause an increase in N2O production of AOB. If the nitrite concentration in the influent is high, it will promote the denitrification of AOB and effectively convert nitrite to N2O (Colliver and Stephenson, 2000). However, the limited COD availability in low C/N wastewater will also cause nitrite accumulation (Hanaki et al., 1992), further enhancing the N2O generation pathway of AOB denitrification. Other conditions, such as low pH, can cause nitrification stress in AOB, leading to protonation of NO2− into HNO2. HNO2 inhibits the activity of NOB in the mixed system, causing the accumulation of NO2−, which in turn promotes N2O production (Peng et al., 2015b). Additionally, nitrifying sludge is more likely to produce N2O from AOB under anaerobic conditions. Experiments have shown that the N2O generation rate reaches its maximum at a DO of 0.85 mg O2/L, while the N2O emission factor decreased with an increase in DO from 0.35 mg O2/L to 3.5 mg O2/L (Peng et al., 2015a).
In terms of metabolism and gene expression, the AOB denitrification pathway includes NIR that reduces NO2− to NO and NOR that reduces NO to N2O (Kozlowski et al., 2016b). Studies on bacteria, such as eutrophic Nitrosomonas europaea, have found that AOB requires NOR activity to convert NO into N2O during the processes of nitrification and denitrification, that is, it is not the nitrite reductase gene NirK that is necessary for N2O production, but the nitric oxide reductase gene NorB (Kozlowski et al., 2014; Kozlowski et al., 2016a). For oligotrophic AOB and AOA lacking NOR activity, it is more important that abiotic reactions (chemical denitrification) convert NO into N2O and discharge it in vitro (Kozlowski et al., 2016a). Recent experiments have proven that N2O in PN with higher nitrogen removal efficiency for low C/N wastewater was also produced by mixing biological and abiotic nitrosation (Terada et al., 2017). During the aerobic ammonia oxidation process, extracellular NH2OH undergoes a non-biological reaction with substances in the growth medium, which also serves as a pathway for N2O conversion (Liu et al., 2017). Previous studies may have underestimated N2O emissions caused by non-biological pathways.
The nitrification of AOB is achieved by the membrane-bound enzyme ammonia monooxygenase (AMO) oxidizing ammonia (NH3) to produce NH2OH, which is then mediated by the periplasmic enzyme hydroxylamine dehydrogenase (HAO) to produce nitrite. The acidic conditions mentioned earlier (pH < 5) can induce partial inhibition of HAO, and the released NO can be further reduced to N2O, thereby enhancing the NH2OH oxidation pathway of N2O (Jiang and Bakken, 1999).
There remains a research gap regarding the N2O emission contribution of AOA in BNR of WWTPs. It is known that both AOA and AOB increase in high ammonium states in soil ecosystems, while AOA dominates in low ammonium states (Hink et al., 2018). The advantages of AOA can be demonstrated under conditions of low ammonium, hypoxia, long SRT, and high temperature, indicating that AOA may promote the generation of N2O to a certain extent under these conditions (Wu et al., 2020). However, currently only Castellano-Hinojosa et al. (2018) have reported a negative correlation between AOA abundance and N2O emissions in four aerobic sludge wastewater treatment plants in Spain. Therefore, they believe that AOA is unlikely to make a significant contribution to N2O generation.
For nitrogen removal systems with integrated functions of autotrophic and heterotrophic microorganisms, denitrification is the main contributor to N2O emissions. For example, in the SBBR reactor for treating low C/N (=0∼1) wastewater, N2O emissions from hydroxylamine oxidation, AOB denitrification, and heterotrophic denitrification accounted for 5.4∼7.6%, 45.2∼60.8% and 33.8∼47.2% of the total N2O emissions, respectively. In reactors with varying C/N ratios, the contribution of denitrification to N2O production ranged from 90% to 96%. And with the decreases of the C/N ratios, the total amount of N2O in denitrification process increased (Domingo-Felez et al., 2017; Yan et al., 2019; Fang et al., 2020). However, NH2OH oxidation and AOB denitrification pathways are primarily found in the PN process (Ni and Yuan, 2015). In the aerobic stage, N2O generated by NH2OH oxidation accounts for 65% of the total N2O, and the N2O generated by AOB denitrification in the later stage is nearly identical to that generated by NH2OH oxidation (Rathnayake et al., 2013). In addition, N2O emissions from non-biological pathways account for 1.1% of the TN load (Soler-Jofra et al., 2016; Liu et al., 2018).
In the partial nitrification-anammox (PN/A) process, which enhances denitrification performance, anammox process also emits a small amount of N2O in addition to the contribution of PN. The most probable N2O emission path involves heterotrophic denitrification in anammox particles (Okabe et al., 2011). The research showed that in the primary PN/A particle reactor, 70% of N2O emissions occurred in the aerobic surface area dominated by AOB, and 30% occurred in the anoxic area dominated by anammox, and NH2OH oxidation and AOB denitrification had a similar proportion of contributions to N2O emissions from AOB related pathways (Ali et al., 2016). In the full-size two-stage PN/A reactor, N2O emissions in PN stage were high (1.2% of TN load), and the emission source could be located in HD (Hausherr et al., 2022). Due to the fact that the majority of N2O (approximately 97.5%) was emitted by PN units, the emissions of N2O from anammox could be almost negligible (Desloover et al., 2011; Okabe et al., 2011) (Figure 1B). In addition, for the monopolar anaerobic nitrogen removal process dominated by Anammox, the batch experiment of 15N isotope tracing and specific inhibitors demonstrated that when nitrite was the main nitrogen source, the N2O emissions of HD and AOB denitrification were 64% and 36%, respectively (Li et al., 2017).
Under aerobic conditions, little research is available on enzyme-based N2O metabolism. The primary metabolic pathway of N2O is HD, with the majority of escaping N2O originating from AOB. This indicates that the rate of N2O consumption by HB is lower than that of N2O production by AOB, which is the main reason for N2O emission from aerobic phase (Yang et al., 2021). In SBR with intermittent aeration, it is also found that AOB denitrification is the main way to produce N2O, and HD is the sink of N2O (Liu et al., 2021a).
Due to the unclear correlation between microbial community abundance and N2O generation contribution, it is challenging to make a unified and clear judgment on the N2O generation mechanism under different denitrification processes for urban wastewater with low C/N ratios. Future research needs to start from multiple factors such as microbial community structure, N2O metabolic characteristics, enzyme activity, and more in-depth consideration of various N2O production pathways and their representative microbial contributions, in order to lay a solid scientific foundation for proposing N2O emission reduction strategies for low C/N wastewater treatment. In terms of methodological applications, emerging single cell metabolic phenotypes can be considered, whose single cell Raman spectroscopy (SCRS) can identify and select individual cells or functional bacterial populations (Jones et al., 2019; Liu et al., 2022), enabling in situ functional analysis. The key relationship between phenotypic heterogeneity and plasticity of PAO populations in enhanced biological phosphorus removal (EBPR) systems and the stability of the EBPR process has been investigated using SCRS, which cannot be solely determined through phylogenetic analysis (Li et al., 2018). Practical experience has shown that SCRS can be widely applied in the fields of biology and environment, addressing key limitations related to omics-centered environmental ecological research methods, such as a lack of cell-level resolution and limited capacity to infer gene functional relationships, especially for highly diverse and featureless ecosystems (Wang et al., 2020). If relevant cutting-edge technologies are employed to directly correspond different bacterial communities and their N2O production and reduction functions, it could provide a better understanding of the N2O metabolism mechanism in BNR systems.
The traditional BNR process, represented by complete nitrification/denitrification and PN-D, has been widely used in WWTPs. For the treatment of urban wastewater with low C/N, most efforts still focus on upgrading and renovating existing WWTPs. Therefore, it is particularly important to optimize emission reduction measures based on the N2O emission characteristics of low C/N wastewater for practical applications.
For the denitrification process in SBR reactors, the N2O production characteristics of microbial systems established on different carbon sources vary. Lack of oxygen inhibits the production of NAR in the methanol carbon source system, leading to reduced N2O production in the anoxic and subsequent aerobic stages. However, in a carbon source system where ethanol is used, NAR is more resistant to oxygen limitations. The downstream N2OR is more sensitive to hypoxia and inhibition than other denitrification enzymes (Korner and Zumft, 1989), which makes N2O generated and accumulated in the subsequent aerobic stage. The above differences may be related to the distinct composition of microbial communities established by methanol and ethanol. Therefore, in the practical operation of WWTPs, strict control of ethanol addition to the anoxic zone is required to minimize the production and discharge of N2O in the downstream aerobic zone. For the modified Ludzak Ettinger (MLE) processes dominated by denitrification, changing the carbon source from methanol to acetate can reduce the N2O conversion rate from 3.0% to 1.0%, and the N2O reduction rate of acetate biomass is higher than that of methanol biomass (Table 2). The disparity in N2O reduction rate could be attributed to different bacterial communities enriched with different carbon sources (Song et al., 2015). Then some scholars used methanol, sodium acetate and glucose as external carbon sources to optimize C/N of denitrification biofilter. Through comprehensive comparison, it is also confirmed that sodium acetate is more suitable as an external carbon source (Xu et al., 2018).
TABLE 2. Optimization strategies for N2O emission reduction operation of various BNR processes for low C/N wastewater treatment.
Compared with one-time dosing, incremental addition of carbon sources enhanced nitrogen removal efficiency and could serve as a strategy for highly automated wastewater treatment systems to reduce N2O emissions (Chai et al., 2019). Furthermore, within the cyclic activated sludge system (CASS), consistent feeding can improve denitrification efficiency and decrease N2O emissions as compared to intermittent feeding. The carbon source in the continuous feeding alleviates the electronic competition between denitrification reductases in the non-aeration stage (Richardson et al., 2009). From a microbial community perspective, the continuous feeding system exhibits a high abundance of N2O reducing bacteria within the denitrification bacteria (Liang et al., 2015). There are significant differences in N2O emission reduction strategies among the different processes mentioned above, therefore, when designing emission reduction plans, it is necessary to optimize the design by categorizing the processes.
This indicates that the substitution and addition strategy of additional carbon sources can improve the C/N ratio of wastewater, thereby enhancing denitrification efficiency while reducing N2O. It is a straightforward and more cost-effective strategy for reducing emissions in WWTPs.
In the combined denitrification process of CANON and denitrification, an appropriate carbon source (C/N = 1) could decrease the total N2O production by 16.7% compared to C/N = 0. This was due to the inhibition of the AOB denitrification process that consumed NO2−-N, which accounted for over 94.5% of N2O emissions (Yan et al., 2019). Based on the N2O emissions of wastewater with low C/N from various processes in Chapter 2, there are significant differences in carbon source dosage and C/N ratio for different processes to achieve emission reduction effects (Figure 4A). For the CANON system, primarily composed of autotrophic microorganisms, adding a small amount of carbon source with C/N ratio of 1 could achieve a significant reduction in N2O emission. If a large amount of carbon source (C/N > 1) is added, the heterotrophic NOB activity cannot be inhibited, disrupting the stability of the system (Zhang et al., 2017; Yan et al., 2019). Specifically, due to the predominance of autotrophic denitrification bacteria in this process, the main carbon source required is IC. Considering the weak inhibitory effect of IC/N on AOB activity and the strong inhibitory effect on anammox activity, some studies have shown that using influent with a C/N range of 1.2 to 1.5–2.0 in CANON can achieve stable denitrification effects (Zhang X J et al., 2016; Yue et al., 2018). This indirectly indicates that the CANON process is suitable for treating low C/N wastewater, but the relationship between N2O emissions and IC concentration requires further exploration.
FIGURE 4. Optimization of process strategies for reducing N2O emissions in low C/N wastewater. (A) Carbon source regulation; (B) Aeration; (C) Internal recycle rate; (D) Weak electrical stimulation.
In the CANDO that also eliminates NO2−, when the COD/N ratio increases to 5, the N2O yield decreases and the nitrogen removal rate increases compared to C/N = 3 or 4 (Weissbach et al., 2018). Considering the reduction of nitrogen removal efficiency, enzyme activity, and N2O emissions, it is more feasible to maintain the influent C/N of A/O SBR at around 6.5 (Yang et al., 2021). Similarly, in another anoxic aerobic BNR experiment, step feeding and additional carbon sources allow AOB (Nitrosomonas) to mitigate the denitrification effect of nitrifying bacteria, thereby reducing the N2O conversion rate by 66.6% and 12.0%, respectively, indicating that these two methods are effective in reducing N2O emissions during sewage treatment (Hu et al., 2013b).
In addition, for the system that simultaneously removes nitrogen and phosphorus, setting the C/N ratio to 4 will result in a minimum N2O conversion rate and an ideal nitrogen removal efficiency of 98.3%, but only 27.44% phosphorus can be removed. The phosphorus removal efficiency will reach the optimal value of 82.79% when C/N = 3. This also inspires future research on actual wastewater denitrification to consider the synergistic effects of multiple pollutants removal and N2O emission reduction (Ge et al., 2018).
In the BNR process, the optimization of operating parameters is the key to reducing N2O emissions. In the treatment of low C/N (=6) wastewater, compared to continuous aeration, intermittent aeration had a higher TN removal efficiency (93.5% on average), and the N2O emission factor decreased from 0.42% of continuous aeration to 0.19%, which promoted PN-D. Among them, the complete ammonia oxidizer (comammox) was significantly enriched during intermittent aeration, and the quantitative results showed that their gene abundance reached 24.7%; The abundance of AOB bacteria significantly decreased (Liu et al., 2021a). Comammox lacks NO reductase, and non-biotransformation emits much less N2O than AOB, showcasing its potential for reducing N2O emissions (Liu et al., 2017; Kits et al., 2019). Conversely, during the operation of the PN/A process, a transition from low aeration (or hypoxia) to high aeration will quickly increase the N2O emission rate, while increased continuous aeration will reduce the emission rate, proving that the continuous aeration strategy is effective in reducing N2O emissions in PN/A (Figure 4B) (Castro-Barros et al., 2015).
In the A2/O process, for actual domestic wastewater with low C/N (=4.4), when the internal circulation ratio of the process is reduced from 300% to 100%, the production of N2O increases from 9.81 × 10−2 mg/L reduced to 3.47 × 10−2 mg/L (Figure 4C). The primary reduction occurs in the form of N2O produced by denitrification in anoxic section. This phenomenon is due to the reduction of the volume of internal circulating liquid and the reduction of nitrate substrate and oxygen that can be used for denitrification. As mentioned in Chapter 4.1, N2OR is more sensitive to oxygen, thus, enhancing the activity of N2OR entering the anoxic zone, making it more conducive to the denitrification process of reducing N2O to N2. Experimental evidence indicated that the copy number of nosZ gene increased as the internal circulation ratio decreased from 300% to 100% (Yan et al., 2016).
In addition, electrical stimulation can optimize microbial population structure and enhance microbial autotrophic denitrification (Figure 4D). At the same time, the activity of NAR and NIR is increased to promote denitrification, so as to improve the removal efficiency of NO3− and reduce the accumulation of N2O. The removal rate of nitrate and TN can be increased by 20%, and the production of intermediate greenhouse gas N2O can be reduced by 62.6% when weak electric stimulation (0.2V) is added to the denitrification process of influent C/N = 3 (Dong et al., 2022).
This research suggests that future N2O emission reduction strategies can be developed based on N2O metabolism mechanisms, such as identifying operating conditions that promote N2OR activity or nosZ transcription initiation.
At present, more studies on BNR and N2O emission reduction only investigate the effects of individual parameters on emissions, making it difficult to compare the specific effects and overall impacts of various operating conditions. In light of this limitation, researchers have employed Plackett Burman (PB) multi-factor experimental design and response surface methodology (RSM) to explore emission reduction strategies for N2O in nitrifying granular sludge systems. The analysis revealed that at a temperature of 22.3°C, the pH value of 7.1, and the aeration rate of 0.20 m3/h, the N2O emission during the denitrification process is minimal. The predicted results were confirmed using wastewater with COD/NH4+ = 5/3 (Liu et al., 2016).
In the complete nitrification/denitrification process, the N2O emission reduction effect in the BNR process related to oxidation ditch is very significant. In Carrousel oxidation ditch and related pre-anaerobic processes, for influent with C/N ratios of 2.57–5, N2O emissions were as low as 0.027%–0.14% of influent nitrogen (Table 3), which was significantly lower than in other complete nitrification and denitrification processes (Ren et al., 2013; Zheng et al., 2015), of which approximately 90% is attributed to nitrification and denitrification. And research on the impact of COD/N on its N2O emission characteristics showed that at lower COD/N ratio of 5, the N2O emission factor could reach a maximum value of 0.142%, which was higher than the 0.055% at a COD/N ratio of 7 (Zheng et al., 2021). Even though low C/N inflow can promote N2O emissions, the oxidation ditch system still plays a significant role in reducing emissions.
The primary reason why this process can reduce N2O emissions is that it can enrich the denitrification bacteria and NOB with a high abundance, which have lower N2O emissions (Zheng et al., 2015). However, further evaluation is necessary to determine the emission reduction potential of the process based on the actual operating mode. When the normal operation of the oxidation ditch system is impacted by ammonia overload or aeration failure, the production of N2O could significantly increase. Further optimization of operating conditions could also impact N2O emissions from the pilot oxidation ditch. The study found that properly extending the SRT to 25 days or immobilizing the aerobic denitrification bacteria PCN-1 on the polyurethane biological carrier to biologically strengthen the oxidation ditch can enrich more comammox belonging to Nitrospira in the system, effectively avoid the accumulation of NO2−, and the system will also express more abundant N2O reductase to achieve N2O emission reduction (Zhou et al., 2019; Tian et al., 2021).
Biofilm systems can improve the efficiency of BNR, thereby reducing N2O emissions. For example, in a pilot-scale UCT (University of Cape Town) MBR reactor, when treating wastewater with a C/N ratio of 5, the average N2O discharge amounts to 0.5% of the influent nitrogen. The enhanced nitrogen removal efficiency of biofilm may be attributed to the coexistence of suspended and attached biomass, as well as the increased richness and diversity of biological communities, which enhance the nitrification and denitrification performance (Eldyasti et al., 2010; Mannina et al., 2018a; Sun et al., 2019). N2O can be produced during the oxidation of hydroxylamine and the reduction of nitrite. Due to the mediation and strong influence of microorganisms diffusing within and outside the biofilm on the process area, the thickness of the biofilm has a certain contribution to N2O emissions. In the denitrification fluidized bed bioreactor (DFBBR), at a COD/N ratio of 5, the N2O conversion rate of the DFBBR system with a biofilm thickness of 680 μm was 0.53% of the total influent nitrogen load, and when the biofilm thickness was 230 μm, the N2O conversion rate increased to 0.95%. The sevenfold increase in the concentration of liquid nitrite indicated that the increase in emissions was due to the limited reduction rate of NO3− to NO2− in thinner biofilms. Therefore, increasing the thickness of the biofilm can reduce N2O emissions in granular biofilm processes (Eldyasti et al., 2014).
The biofilm system also promotes the formation of flocs within the system. The flocs of the denitrifying biofilm/flocs system can effectively reduce the total N2O accumulation by 32%. The flocs also promote a high proportion of electron distribution to N2OR, indicating that the flocs have strong N2O reduction ability (Liu Y R et al., 2023).
In addition, incorporating anoxic carrier biofilm in to actual WWTPs can facilitate in-situ enrichment of anammox and enhance the nitrogen removal efficiency of urban WWTPs. This process has been studied for treating urban wastewater with COD/N ratios ranging from 1.2 to 7.9. The proportion of N2O emissions (liquid and gaseous) from the anoxic zone to nitrogen loss is less than 0.08%. With the extension of reaction time, the proportion of N2O emissions to nitrogen loss further decreased to <0.02%, and the N2O conversion rate was significantly lower than the traditional denitrification process summarized in this article. This indicated that the addition of anammox could help reduce N2O emissions in BNR (Li et al., 2019).
Based on Section 2.3, it can be seen that the average N2O emission level of anammox related processes in treating urban wastewater is lower than that of traditional BNR processes. However, the combination of processes and operating conditions also significantly influence the N2O conversion rate. In combined processes, such as PN/A and partial denitrification/anammox (PD/A), N2O accumulation is more significant. When the two-stage PN/A process was employed to treat high ammonium synthesis wastewater or low C/N mainstream wastewater, the comprehensive N2O conversion rate ranged from 4.1% to 6.6%, with most of the emissions being contributed by the PN unit (Desloover et al., 2011; Okabe et al., 2011; Li L et al., 2020). The comprehensive discharge of unipolar PN/A when treating urban wastewater accounted for 0.35%–2.00% of the influent nitrogen load, thereby enhancing the N2O emission reduction performance to some extent (Ali et al., 2016; Yang et al., 2016; Zhou et al., 2020).
Summarizing multiple studies, it has been observed that the PD/A process can achieve stable denitrification of mainstream wastewater with C/N ranging from 1.77 to 3.4 (Zhang et al., 2019). Comparing this process reveals that the maximum cumulative amount of N2O is 2.4% of influent nitrogen, which is lower than the traditional BNR or two-stage PN/A process (Du et al., 2020; Gao et al., 2022). The PN/A or PD/A combination process can help reduce N2O emissions during BNR of low C/N wastewater.
Although there are anammox, nitrifying bacteria and denitrification bacteria in the unipolar anammox system, the abundance of anammox is the highest, and the theoretical N2O emission value is low. The experiment showed that when treating wastewater with a C/N of 0.3∼1.4, the production of N2O was directly proportional to the filtration rate, with the N2O emission factor increasing from 0.012% at 1.0 m/h to 0.496% at 3.0 m/h. When the filtration rate was 1.5 m/h, both the removal rates of NH4+-N and NO2−-N reached 99%, and the N2O concentration was minimal. These conditions can be considered as the optimal reference for the process (Li et al., 2022).
Iron based materials enhancing BNR are considered one of the potential methods for effectively treating low C/N ratio wastewater. And feammox is a novel BNR process that combines anammox with Fe(III) reduction. Feammox can utilize Fe (III) instead of NO2−- N as the electron acceptor to reduce NH4+-N to N2, NO2−-N and NO3−-N through microorganisms (Peng et al., 2021). Therefore, the theoretical avoidance of N2O producing from denitrification is achieved. The feammox process has been demonstrated to be applicable for low C/N wastewater treatment. The feammox process operating in the Multistage Feammox Bioreactor (MSFB) can effectively treat actual anaerobic digestion (AD) wastewater. When the C/N ratio was 2.5, the AD effluent performance of the reactor exhibited the best, with a TN removal rate of 99% (Nguyen et al., 2023).
During the batch test of feammox, it was detected that the total N2O production was significantly lower (p < 0.05) than N2, and N2O emissions were beneath the detection limit, thereby confirming the potential to reduce N2O emissions (Zhou et al., 2016). However, in practical wastewater treatment, there remains a dearth of monitoring N2O emissions within the feammox system and the analysis of the impact of iron on N2O metabolic pathways.
In 2006, Islas-Lima S and Raghoebarsing found and obtained the concentration of n-DAMO for the first time. The n-DAMO group can be mainly divided into the DAMO archaea system of ANME-2d and the n-DAMO bacteria within the NC10 phylum. CH4 can serve as the carbon source to facilitate the denitrification process, convertingNO3- to NO2− and NO2− to N2, respectively (Islas-Lima et al., 2004; Raghoebarsing et al., 2006). When co-cultured with anammox, a DAMO-A process can be formed. N-DAMO microorganisms are capable of oxidizing methane and releasing electrons during the reverse process of methane production. These electrons can be utilized as donors for denitrification, ultimately converting CH4 into CO2. Additionally, anammox will transform the generated NO2− and NH4+ into N2 (Haroon et al., 2013). The reaction scheme of the system is as follows:
The coupling process is an autotrophic system, and the functional microbial community n-DAMO and anammox mainly utilize inorganic carbon sources. Therefore, the low COD/N ratio of the influent should not affect the BNR performance, as it minimizes the likelihood of N2O generation through denitrification. Moreover, this process can simultaneously reduce CH4 and N2O emissions, offering a synergistic effects of pollution reduction and carbon reduction, making it a promising choice for future green innovations in WWTPs.
However, limited systematic research exists on the emission and mechanism of N2O within the DAMO-A system. In 2015, it was reported that N2O was undetectable in the reactor on the 53rd, 115th, 199th, and 260th day (data not shown) (Hu et al., 2015). In 2019, researchers developed a new technology in MBfR that integrates PN, anammox, and methane dependent nitrite/nitrate reduction reactions, which can be abbreviated as PNAM process. The average TN removal rate achieved by this process was 98%, with a N2O emission factor of 0.34%, which is more likely related to AOB metabolism (Liu et al., 2019; Nie et al., 2020; Liu et al., 2021b). However, some scholars have pointed out that NC10 bacteria have a potential pathway to reduce NO to N2O, and the conversion rate of N2O is related to the external NO2− concentration and the non-specific oxidation process of NH4+(Nie et al., 2019). Moreover, the genome of the DAMO-A system contains the gene of N2OR enzyme (Figure 5), but the specific role of this enzyme in the in-situ N2O conversion rate of the system has not been explored (Cogert et al., 2019). Therefore, in the future, it is still necessary to further quantify the gas emissions from this process in urban mainstream wastewater treatment, determine the N2O emission factors and its metabolic pathway of DAMO-A related BNR processes, and evaluate their comprehensive GHG emission reduction effects of eliminating CH4 and reducing N2O.
FIGURE 5. Carbon and nitrogen conversion process of DAMO-A. Solid line: a known process, dashed line: an unproven and possibly existing process.
Recycling Sludge-derived hydrochar (SDHC) enhances the denitrification effect of secondary effluent from WWTPs with low C/N and reduce N2O emissions. When C/N ranges from 3.0 to 3.2, the nitrogen removal rate (NRR) in the enhanced denitrification process DN-SDHC is 3.6 times higher than that of denitrification alone (DN). The high conductivity of SDHC accelerates the extracellular electron transfer from the carbon source to denitrification bacteria. SDHC also promotes a significant increase in the nosZ gene encoding N2OR, which is beneficial for reducing N2O accumulation (Hao et al., 2022).
Aerobic environments are usually not conducive to reducing N2O emissions in WWTPs. Researchers have isolated a new strain Pseudomonas sp. YR02 that can reduce N2O under aerobic conditions. The successful amplification of four denitrification genes proved its complete denitrification ability. YR02 exhibited excellent performance in treating wastewater with high ammonia nitrogen and dissolved N2O. Although the strain achieves maximum inorganic nitrogen (IN) removal efficiency (>98%) under higher C/N conditions (C/N = 15), it was more conducive at reducing N2O emissions when the C/N ratio is 5. YR02 could reduce N2O emissions by 98.7% and increase NRE by 32% in WWTPs, demonstrating its potential for alleviating N2O emissions (Wang et al., 2023).
Pseudomonas sp. GZWN4, another species of the same genus, exhibits excellent aerobic denitrification performance across a wide range of C/N ratios for 5 to 20. This strain was isolated from seaweed aquaculture wastewater and is suitable for enhancing the treatment of ordinary or saline aquaculture water. GZWN4 carries the nosZ gene, indicating its ability to reduce N2O (Su et al., 2021). In addition, Achromobacter sp. HNDS-1 and Enterobacter sp. HNDS-6possess Nxr, narG, nirK, norB, and nosZ genes involved in the denitrification pathway, allowing them to effectively remove mixed nitrogen under C/N = 5 conditions. However, the N2O emission reduction effects of these three strains in actual sewage treatment have not been evaluated (Liu X et al., 2023).
For environments with low C/N ratios, Bacillus thuringiensis strain WXN-23, isolated from aquaculture filtrate, has stronger adaptability. Batch tests showed that the bacterium can achieve a TN removal rate of 95.996% at a C/N ratio of 5.91. Moreover, it has a relatively complete nitrification and denitrification pathway (NH4+-N→NH2OH→NO2−-N→NO3−-N→NO2−-N→NO→N2O→N2) (Xu et al., 2021), which promotes N2O reduction. Therefore, strain WXN-23 holds potential as a powerful strain for enhancing the green process in low C/N wastewater treatment.
Adding new bacterial strains to existing processes to enhance BNR performance has emerged as a research hotspot in recent years. Many new strains are isolated from environmental systems, sludge, or sewage treatment facilities and optimized for cultivation conditions. Before applying new bacterial strains, it is essential to assess their safety. There have been reports indicating that certain bacteria can produce hemolysin, which can cause toxic effects like cell membrane damage and lysis (Mogrovejo et al., 2020; Su et al., 2021). The presence of hemolysin production in a strain can serve as a crucial criterion for evaluating its safety.
However, if we want to apply this approach to enhance the process of sewage treatment plants and solve the problem of N2O emission reduction in low C/N wastewater, more in-depth experiments and research are still needed. For example, how to better maintain the strain in the system without loss with effluent. In actual sewage, there is a presence of diverse organisms, including predatory protozoa and phage, which can hinder the survival of introduced strains and potentially lead to the failure of bioaugmentation (Ma et al., 2022). To address this challenge, it is possible to select specific strains with BNR capabilities, such as the denitrifying strain Alcaligenes aquatilis AS1 (Cao et al., 2023). These strains can help enhance the interaction within microbial networks and promote the stability of microbial communities. Another approach to ensure the survival of selected strains involves immobilizing them using gel particles or other immobilized redox mediator granules (IRMG), which prevents competition-induced destruction. The application of this biological immobilization technology can enhance the survival capacity of the strains (Han et al., 2021; Shi et al., 2022; Sun et al., 2023). It is also important that the majority of aerobic denitrification strains thrive in environments with an ample carbon source. Therefore, when evaluating the trade-off between NRE and the reduction of N2O emissions in low C/N environments, this factor must be taken into account.
Based on the characteristics of biological nitrogen removal (BNR) processes, such as complete nitrification/denitrification, partial nitrification/denitrification, and anammox, it has been observed that treating domestic wastewater with a generally low C/N ratio leads to higher N2O emissions. The insufficient carbon sources and a low carbon loading rate in low C/N wastewater intensify the competition among denitrification enzymes, affecting the production and consumption of N2O and resulting in its accumulation. Additionally, the limitation of inorganic carbon (IC) in autotrophic nitrification/denitrification systems restricts the oxidation activity of ammonia-oxidizing bacteria (AOB) towards NH4+ and contributes to increased N2O emissions. To effectively reduce N2O emissions in low C/N wastewater, adjusting the type or dosage of the carbon source has proven to be effective. Furthermore, during the upgrade process of sewage treatment plants, comprehensive optimization of aeration methods, internal circulation ratio, and other conditions, as well as the implementation of oxidation ditch systems and biofilm systems, can be implemented to reduce N2O emissions. The development of new BNR processes, particularly autotrophic anammox-related processes such as unipolar PN/A, PD/A, unipolar Anammox, and Feammox, shows promise in reducing the demand for organic carbon and achieving deep denitrification and N2O emission reduction in low C/N wastewater. Notably, the DAMO-A system can utilize CH4 while reducing N2O production. However, there is still a lack of systematic research on the emission factors and metabolic mechanisms of N2O in these processes, which would provide theoretical support for emission reduction.
The perspective of this review focuses on N2O emission reduction through BNR of low C/N wastewater. We have proposed some directions and suggestions to enhance our understanding and develop solutions for mitigating N2O emissions in low C/N wastewater:
• Develop N2O emission evaluation indicators for WWTPs or BNR processes. This includes measuring the N2O conversion rate of the process and establishing standardized measurement methods for different nitrogen-based unit conversion rates. Additionally scientific methods should be established to compare the effectiveness of N2O emission reduction strategies across different processes.
• Explore the application of emerging methodologies such as SCRS in studying microbial N2O metabolism. This approach can help analyze the N2O production and reduction functions of individual functional microbial communities in complex microbial systems involved in BNR. It is advisable to consider multiple factors such as microbial community structure, N2O metabolic characteristics, the relationship between electricity competition caused by carbon source limitation and enzyme activity. More in-depth consideration of various N2O production pathways and the contributions of representative microorganisms should be formed to address the limitations of N2O metabolism research in low C/N wastewater treatment.
• When proposing N2O emission reduction strategies, more consideration should be given to the economic and cost-effectiveness of measures such as adding carbon sources and changing feeding methods. These measures should not only provide environmental benefits but also be evaluated in terms of emission reduction scenarios, such as carbon footprint. Conducting a comprehensive review of emission reduction plans will facilitate their practical application.
• Further investigation into novel processes, such as the unipolar and two-stage systems driven by Anammox, is warranted. Of particular interest is the DAMO-A process, which has the potential to simultaneously reduce CH4 and N2O emissions. This process mainly focuses on autotrophic DAMO microorganisms and Anammox bacteria. In the future, rapid enrichment methods need to be developed to explore their N2O emission levels and mechanisms as application support.
In summary, the scientific issues addressed in this article are of great significance in the context of global warming. As an essential aspect of sustainable social development, sewage treatment must strive to achieve the objective of coordinated pollution reduction and carbon reduction. For urban wastewater that has large displacement, we need to pay attention to the powerful influencing factors of its low C/N ratio, and further analyze the impact and mechanism of this characteristic on N2O emissions in the BNR process. Research should not be limited solely to monitoring emissions on a macro-scale. In order to address this challenging issue, it is necessary to develop more robust functional analysis methods, surpass the limitations posed by the complexity of environmental microbial systems and various BNR processes, and expand future research in this direction.
YX, CJ, and SX contributed to conception and design of the study. YX and BK organized the database. YX wrote the first draft of the manuscript. CJ, BK, and SX added some content to the manuscript. CJ and XZ revised it critically for important intellectual content. XZ provide approval for publication of the content. All authors contributed to the article and approved the submitted version.
This research was supported by the National Natural Science Foundation of China (No. 42177099 and 91951108), the Knowledge Innovation Program of Shenzhen (JSGG20191129112812329), the National Natural Science Foundation of China (No. 21976197), and the CAS International Partnership Program (No. 121311KYSB20200017), Provincial science and technology innovative program for carbon peak and carbon neutrality of Jiangsu of China (BE2022422), Jiangsu North Science and Technology project (SZ-YC202118).
Author BK was employed by Sheyang Lexin Agricultural Development Co., Ltd.
The remaining authors declare that the research was conducted in the absence of any commercial or financial relationships that could be construed as a potential conflict of interest.
All claims expressed in this article are solely those of the authors and do not necessarily represent those of their affiliated organizations, or those of the publisher, the editors and the reviewers. Any product that may be evaluated in this article, or claim that may be made by its manufacturer, is not guaranteed or endorsed by the publisher.
Ali, M., Rathnayake, R. M. L. D., Zhang, L., Ishii, S., Kindaichi, T., Satoh, H., et al. (2016). Source identification of nitrous oxide emission pathways from a single-stage nitritation-anammox granular reactor. WATER Res. 102, 147–157. doi:10.1016/j.watres.2016.06.034
Alinsafi, A., Adouani, N., Beline, F., Lendormi, T., Limousy, L., and Sire, O. (2008). Nitrite effect on nitrous oxide emission from denitrifying activated sludge. Process Biochem. 43 (6), 683–689. doi:10.1016/j.procbio.2008.02.008
Bock, E., Schmidt, I., Stuven, R., and Zart, D. (1995). NITROGEN LOSS CAUSED BY DENITRIFYING NITROSOMONAS CELLS USING AMMONIUM OR HYDROGEN AS ELECTRON-DONORS AND NITRITE AS ELECTRON-ACCEPTOR. ARCHIVES Microbiol. 163 (1), 16–20. doi:10.1007/bf00262198
Bodik, I., Blstakova, A., Sedlacek, S., and Hutnan, M. (2009). Biodiesel waste as source of organic carbon for municipal WWTP denitrification. Bioresour. Technol. 100 (8), 2452–2456. doi:10.1016/j.biortech.2008.11.050
Bogner, J., Pipatti, R., Hashimoto, S., Diaz, C., Mareckova, K., Diaz, L., et al. (2008). Mitigation of global greenhouse gas emissions from waste: conclusions and strategies from the intergovernmental panel on climate change (IPCC) fourth assessment report. Working group III (mitigation). Waste Manag. Res. 26 (1), 11–32. doi:10.1177/0734242x07088433
Cao, X. H., Hu, C. C., Sun, X. Y., Zhang, L., Wang, H. Z., Dong, L., et al. (2023). Long-term stability of reactor microbiome through bioaugmentation with Alcaligenes aquatilis AS1 promotes nitrogen removal of piggery wastewater. J. Environ. Manag. 330, 117146. ARTN 117146. doi:10.1016/j.jenvman.2022.117146
Caspi, R., Altman, T., Dreher, K., Fulcher, C. A., Subhraveti, P., Keseler, I. M., et al. (2012). The MetaCyc database of metabolic pathways and enzymes and the BioCyc collection of pathway/genome databases. NUCLEIC ACIDS Res. 40 (D1), D742–D753. doi:10.1093/nar/gkr1014
Castellano-Hinojosa, A., Maza-Marquez, P., Melero-Rubio, Y., Gonzalez-Lopez, J., and Rodelas, B. (2018). Linking nitrous oxide emissions to population dynamics of nitrifying and denitrifying prokaryotes in four full-scale wastewater treatment plants. Chemosphere 200, 57–66. doi:10.1016/j.chemosphere.2018.02.102
Castro-Barros, C. M., Daelman, M. R. J., Mampaey, K. E., van Loosdrecht, M. C. M., and Volcke, E. I. P. (2015). Effect of aeration regime on N2O emission from partial nitritation-anammox in a full-scale granular sludge reactor. WATER Res. 68, 793–803. doi:10.1016/j.watres.2014.10.056
Chai, H. X., Deng, S. P., Zhou, X. Y., Su, C. R., Xiang, Y., Yang, Y., et al. (2019). Nitrous oxide emission mitigation during low-carbon source wastewater treatment: effect of external carbon source supply strategy. Environ. Sci. Pollut. Res. 26 (22), 23095–23107. doi:10.1007/s11356-019-05516-0
Chandran, K., Stein, L. Y., Klotz, M. G., and van Loosdrecht, M. C. M. (2011). Nitrous oxide production by lithotrophic ammonia-oxidizing bacteria and implications for engineered nitrogen-removal systems. Biochem. Soc. Trans. 39, 1832–1837. doi:10.1042/BST20110717
Chung, Y. C., and Chung, M. S. (2000). BNP test to evaluate the influence of C/N ratio on N2O production in biological denitrification. Water Sci. Technol. 42 (3-4), 23–27. doi:10.2166/wst.2000.0354
Cogert, K. I., Ziels, R. M., and Winkler, M. K. H. (2019). Reducing cost and environmental impact of wastewater treatment with denitrifying methanotrophs, anammox, and mainstream anaerobic treatment. Environ. Sci. Technol. 53 (21), 12935–12944. doi:10.1021/acs.est.9b04764
Colliver, B. B., and Stephenson, T. (2000). Production of nitrogen oxide and dinitrogen oxide by autotrophic nitrifiers. Biotechnol. Adv. 18 (3), 219–232. doi:10.1016/S0734-9750(00)00035-5
Conthe, M., Wittorf, L., Kuenen, J. G., Kleerebezem, R., van Loosdrecht, M. C. M., and Hallin, S. (2018). Life on N2O: deciphering the ecophysiology of N2O respiring bacterial communities in a continuous culture. Isme J. 12 (4), 1142–1153. doi:10.1038/s41396-018-0063-7
de Graaff, M. S., Zeeman, G., Temmink, H., van Loosdrecht, M. C. M., and Buisman, C. J. N. (2010). Long term partial nitritation of anaerobically treated black water and the emission of nitrous oxide. WATER Res. 44 (7), 2171–2178. doi:10.1016/j.watres.2009.12.039
Desloover, J., De Clippeleir, H., Boeckx, P., Du Laing, G., Colsen, J., Verstraete, W., et al. (2011). Floc-based sequential partial nitritation and anammox at full scale with contrasting N2O emissions. WATER Res. 45 (9), 2811–2821. doi:10.1016/j.watres.2011.02.028
Domingo-Felez, C., Mutlu, A. G., Jensen, M. M., and Smets, B. F. (2014). Aeration strategies to mitigate nitrous oxide emissions from single-stage nitritation/anammox reactors. Environ. Sci. Technol. 48 (15), 8679–8687. doi:10.1021/es501819n
Domingo-Felez, C., Pellicer-Nacher, C., Petersen, M. S., Jensen, M. M., Plosz, B. G., and Smets, B. F. (2017). Heterotrophs are key contributors to nitrous oxide production in activated sludge under low C-to-N ratios during nitrification-batch experiments and modeling. Biotechnol. Bioeng. 114 (1), 132–140. doi:10.1002/bit.26062
Dong, X. Y., Liu, H. B., Long, S. P., Xu, S. Y., and Lichtfouse, E. (2022). Weak electrical stimulation on biological denitrification: insights from the denitrifying enzymes. Sci. TOTAL Environ. 806, 150926. ARTN 150926. doi:10.1016/j.scitotenv.2021.150926
Du, R., Cao, S. B., Zhang, H. Y., Li, X. C., and Peng, Y. Z. (2020). Flexible nitrite supply alternative for mainstream anammox: advances in enhancing process stability. Environ. Sci. Technol. 54 (10), 6353–6364. doi:10.1021/acs.est.9b06265
Edenhofer, O., Pichs-Madruga, R., and Sokona, Y. (2014). “Climate change 2014 mitigation of climate change working group III contribution to the fifth assessment report of the intergovernmental panel on climate change preface,” in Climate change 2014: mitigation of climate change (Cambridge University Press). Ix-Xi. <Go to ISI>://WOS:000372635100002. doi:10.1017/CBO9781107415416
Eldyasti, A., Chowdhury, N., Nakhla, G., and Zhu, J. (2010). Biological nutrient removal from leachate using a pilot liquid-solid circulating fluidized bed bioreactor (LSCFB). J. Hazard. Mater. 181 (1-3), 289–297. doi:10.1016/j.jhazmat.2010.05.010
Eldyasti, A., Nakhla, G., and Zhu, J. (2014). Influence of biofilm thickness on nitrous oxide (N2O) emissions from denitrifying fluidized bed bioreactors (DFBBRs). J. Biotechnol. 192, 281–290. doi:10.1016/j.jbiotec.2014.10.008
Fang, F., Li, K., Guo, J. S., Wang, H., Zhang, P., and Yan, P. (2020). New insights into nitrous oxide emissions in a single-stage CANON process coupled with denitrification: thermodynamics and nitrogen transformation. Water Sci. Technol. 82 (1), 157–169. doi:10.2166/wst.2020.344
Gabarro, J., Gonzalez-Carcamo, P., Ruscalleda, M., Ganigue, R., Gich, F., Balaguer, M. D., et al. (2014). Anoxic phases are the main N2O contributor in partial nitritation reactors treating high nitrogen loads with alternate aeration. Bioresour. Technol. 163, 92–99. doi:10.1016/j.biortech.2014.04.019
Gao, J. L., Wang, R., Li, Y. L., Huang, H. M., Su, X. M., An, Z. J., et al. (2022). Effect of aeration modes on nitrogen removal and N2O emission in the partial nitrification and denitrification process for landfill leachate treatment. Sci. TOTAL Environ. 853. ARTN 158424. doi:10.1016/j.scitotenv.2022.158424
Ge, G. H., Zhao, J. Q., Li, X. L., Ding, X. Q., Chen, A. X., Chen, Y., et al. (2018). Author Correction: effects of influent COD/N ratios on nitrous oxide emission in a sequencing biofilm batch reactor for simultaneous nitrogen and phosphorus removal. Sci. Rep. 8, 17646. ARTN 17646. doi:10.1038/s41598-018-36019-6
Han, H., Li, J., Zhang, J., Peng, Y. Z., Li, Y., Zhang, K., et al. (2021). Enhancing the treatment performance of partial denitrification/Anammox process at high nitrogen load: effects of immobilized strain HFQ8C/Non the sludge characteristics. Bioresour. Technol. 341, 125870. ARTN 125870. doi:10.1016/j.biortech.2021.125870
Hanaki, K., Hong, Z., and Matsuo, T. (1992). PRODUCTION OF NITROUS-OXIDE GAS DURING DENITRIFICATION OF WASTE-WATER. Water Sci. Technol. 26 (5-6), 1027–1036. doi:10.2166/wst.1992.0544
Hao, M. X., Chen, H., He, Y. L., Wang, X. N., Zhang, Y., Lao, H. B. A., et al. (2022). Recycling sludge-derived hydrochar to facilitate advanced denitrification of secondary effluent: role of extracellular electron transfer. Chemosphere 291, 132683. ARTN 132683. doi:10.1016/j.chemosphere.2021.132683
Hao, X. D., Liu, R. B., and Huang, X. (2015). Evaluation of the potential for operating carbon neutral WWTPs in China. WATER Res. 87, 424–431. doi:10.1016/j.watres.2015.05.050
Haroon, M. F., Hu, S. H., Shi, Y., Imelfort, M., Keller, J., Hugenholtz, P., et al. (2013). Anaerobic oxidation of methane coupled to nitrate reduction in a novel archaeal lineage. NATURE 500 (7464), 567–570. doi:10.1038/nature12375
Hausherr, D., Niederdorfer, R., Burgmann, H., Lehmann, M. F., Magyar, P., Mohn, J., et al. (2022). Successful year-round mainstream partial nitritation anammox: assessment of effluent quality, performance and N2O emissions. WATER Res. X 16, 100145. Article 100145. doi:10.1016/j.wroa.2022.100145
Horstmeyer, N., Weißbach, M., Koch, K., and Drewes, J. E. (2017). A novel concept to integrate energy recovery into potable water reuse treatment schemes. J. Water Reuse Desalination 8 (4), 455–467. doi:10.2166/wrd.2017.051
Hu, S. H., Zeng, R. J., Haroon, M. F., Keller, J., Lant, P. A., Tyson, G. W., et al. (2015). A laboratory investigation of interactions between denitrifying anaerobic methane oxidation (DAMO) and anammox processes in anoxic environments. Sci. Rep. 5, 8706. Article 8706. doi:10.1038/srep08706
Hu, Z., Zhang, J., Li, S. P., and Xie, H. J. (2013a). Impact of carbon source on nitrous oxide emission from anoxic/oxic biological nitrogen removal process and identification of its emission sources. Environ. Sci. Pollut. Res. 20 (2), 1059–1069. doi:10.1007/s11356-012-1018-6
Hu, Z., Zhang, J., Xie, H., Liang, S., and Li, S. (2013b). Minimization of nitrous oxide emission from anoxic-oxic biological nitrogen removal process: effect of influent COD/NH4+ ratio and feeding strategy. J. Biosci. Bioeng. 115 (3), 272–278. doi:10.1016/j.jbiosc.2012.09.016
Hu, Z. F., Liu, J. Y., Zheng, W. Y., Li, D. S., Liu, Y. H., and Yao, H. (2019). Highly-efficient nitrogen removal from domestic wastewater based on enriched aerobic/anoxic biological filters and functional microbial community characteristics. J. Clean. Prod. 238, 117867. ARTN 117867. doi:10.1016/j.jclepro.2019.117867
Hwang, S., Jang, K., Jang, H., Song, J. Y., and Bae, W. (2006). Factors affecting nitrous oxide production: a comparison of biological nitrogen removal processes with partial and complete nitrification. Biodegradation 17 (1), 19–29. doi:10.1007/s10532-005-2701-9
Islas-Lima, S., Thalasso, F., and Gomez-Hernandez, J. (2004). Evidence of anoxic methane oxidation coupled to denitrification. WATER Res. 38 (1), 13–16. doi:10.1016/j.watres.2003.08.024
Itokawa, H., Hanaki, K., and Matsuo, T. (2001). Nitrous oxide production in high-loading biological nitrogen removal process under low COD/N ratio condition. WATER Res. 35 (3), 657–664. doi:10.1016/S0043-1354(00)00309-2
Jetten, M. S. M., Logemann, S., Muyzer, G., Robertson, L. A., deVries, S., vanLoosdrecht, M. C. M., et al. (1997). Novel principles in the microbial conversion of nitrogen compounds. Ant. Van Leeuwenhoek Int. J. General Mol. Microbiol. 71 (1-2), 75–93. doi:10.1023/A:1000150219937
Jiang, Q. Q., and Bakken, L. R. (1999). Nitrous oxide production and methane oxidation by different ammonia-oxidizing bacteria. Appl. Environ. Microbiol. 65 (6), 2679–2684. doi:10.1128/aem.65.6.2679-2684.1999
Jin, L. Y., Zhang, G. M., and Tian, H. F. (2014). Current state of sewage treatment in China. WATER Res. 66, 85–98. doi:10.1016/j.watres.2014.08.014
Jones, R. R., Hooper, D. C., Zhang, L. W., Wolverson, D., and Valev, V. K. (2019). Raman techniques: fundamentals and Frontiers. Nanoscale Res. Lett. 14, 231. ARTN 231. doi:10.1186/s11671-019-3039-2
Joss, A., Salzgeber, D., Eugster, J., Konig, R., Rottermann, K., Burger, S., et al. (2009). Full-scale nitrogen removal from digester liquid with partial nitritation and anammox in one SBR. Environ. Sci. Technol. 43 (14), 5301–5306. doi:10.1021/es900107w
Kampschreur, M. J., van der Star, W. R. L., Wielders, H. A., Mulder, J. W., Jetten, M. S. M., and van Loosdrecht, M. C. M. (2008). Dynamics of nitric oxide and nitrous oxide emission during full-scale reject water treatment. WATER Res. 42 (3), 812–826. doi:10.1016/j.watres.2007.08.022
Kim, S. W., Miyahara, M., Fushinobu, S., Wakagi, T., and Shoun, H. (2010). Nitrous oxide emission from nitrifying activated sludge dependent on denitrification by ammonia-oxidizing bacteria. Bioresour. Technol. 101 (11), 3958–3963. doi:10.1016/j.biortech.2010.01.030
Kim, T. H., Lee, M. J., Jang, H. S., Min, B. K., Yoo, G. Y., and Hwang, S. J. (2011). Characteristics of N2O release from fluidized media type BNR processes and identification of N2O sources. Desalination Water Treat. 28 (1-3), 378–384. doi:10.5004/dwt.2011.2910
Kishida, N., Kim, J. H., Kimochi, Y., Nishimura, O., Sasaki, H., and Sudo, R. (2004). Effect of C/N ratio on nitrous oxide emission from swine wastewater treatment process. Water Sci. Technol. 49 (5-6), 359–371. doi:10.2166/wst.2004.0775
Kits, K. D., Jung, M. Y., Vierheilig, J., Pjevac, P., Sedlacek, C. J., Liu, S. R., et al. (2019). Low yield and abiotic origin of N2O formed by the complete nitrifier Nitrospira inopinata. Nat. Commun. 10, 1836. ARTN 1836. doi:10.1038/s41467-019-09790-x
Korner, H., and Zumft, W. G. (1989). EXPRESSION OF DENITRIFICATION ENZYMES IN RESPONSE TO THE DISSOLVED-OXYGEN LEVEL AND RESPIRATORY SUBSTRATE IN CONTINUOUS CULTURE OF PSEUDOMONAS-STUTZERI. Appl. Environ. Microbiol. 55 (7), 1670–1676. doi:10.1128/aem.55.7.1670-1676.1989
Kozlowski, J. A., Kits, K. D., and Stein, L. Y. (2016a). Comparison of nitrogen oxide metabolism among diverse ammonia-oxidizing bacteria. Front. Microbiol. 7, 1090. ARTN 1090. doi:10.3389/fmicb.2016.01090
Kozlowski, J. A., Kits, K. D., and Stein, L. Y. (2016b). Genome sequence of Nitrosomonas communis strain Nm2, a mesophilic ammonia-oxidizing bacterium isolated from mediterranean soil. Genome Announc. 4 (1), e01541-15. ARTN e01541-15. doi:10.1128/genomeA.01541-15
Kozlowski, J. A., Price, J., and Stein, L. Y. (2014). Revision of N2O-producing pathways in the ammonia-oxidizing bacterium Nitrosomonas europaea ATCC 19718. Appl. Environ. Microbiol. 80 (16), 4930–4935. doi:10.1128/Aem.01061-14
Law, Y. Y., Ye, L., Pan, Y. T., and Yuan, Z. G. (2012). Nitrous oxide emissions from wastewater treatment processes. PHILOSOPHICAL Trans. R. Soc. B-BIOLOGICAL Sci. 367 (1593), 1265–1277. doi:10.1098/rstb.2011.0317
Lee, Y. Y., Choi, H., and Cho, K. S. (2019). Effects of carbon source, C/N ratio, nitrate, temperature, and pH on N2O emission and functional denitrifying genes during heterotrophic denitrification. J. Environ. Sci. Health Part a-Toxic/Hazardous Subst. Environ. Eng. 54 (1), 16–29. doi:10.1080/10934529.2018.1503903
Li, J. M., Liu, X. H., Gu, P. C., Cui, B., Yang, Q., and Zeng, W. (2022). N2O production and emission pathways in anammox biofilter for treating wastewater with low nitrogen concentrations. Sci. TOTAL Environ. 852, 158282. ARTN 158282. doi:10.1016/j.scitotenv.2022.158282
Li, J. W., Peng, Y. Z., Zhang, L., Gao, R. T., Yang, L., Liu, Q. Y., et al. (2020). Enhanced nitrogen removal assisted by mainstream partial-anammox from real sewage in a continuous flow A(2)/O reactor. Chem. Eng. J. 400, 125893. ARTN 125893. doi:10.1016/j.cej.2020.125893
Li, J. W., Peng, Y. Z., Zhang, L., Liu, J. J., Wang, X. D., Gao, R. T., et al. (2019). Quantify the contribution of anammox for enhanced nitrogen removal through metagenomic analysis and mass balance in an anoxic moving bed biofilm reactor. WATER Res. 160, 178–187. doi:10.1016/j.watres.2019.05.070
Li, K., Fang, F., Wang, H., Wang, C., Chen, Y. P., Guo, J. S., et al. (2017). Pathways of N removal and N2O emission from a one-stage autotrophic N removal process under anaerobic conditions. Sci. Rep. 7, 42072. Article 42072. doi:10.1038/srep42072
Li, L., Ling, Y., Wang, H. Y., Chu, Z. S., Yan, G. K., Li, Z. W., et al. (2020). N2O emission in partial nitritation-anammox process. Chin. Chem. Lett. 31 (1), 28–38. doi:10.1016/j.cclet.2019.06.035
Li, Y. Y., Cope, H. A., Rahman, S. M., Li, G. Y., Nielsen, P. H., Elfick, A., et al. (2018). Toward better understanding of EBPR systems via linking Raman-based phenotypic profiling with phylogenetic diversity. Environ. Sci. Technol. 52 (15), 8596–8606. doi:10.1021/acs.est.8b01388
Liang, W. H., Yu, C., Ren, H. Q., Geng, J. J., Ding, L. L., and Xu, K. (2015). Minimization of nitrous oxide emission from CASS process treating low carbon source domestic wastewater: effect of feeding strategy and aeration rate. Bioresour. Technol. 198, 172–180. doi:10.1016/j.biortech.2015.08.075
Liu, R. T., Wang, X. H., Zhang, Y., Wang, M. Y., Gao, M. M., and Wang, S. G. (2016). Optimization of operation conditions for the mitigation of nitrous oxide (N2O) emissions from aerobic nitrifying granular sludge system. Environ. Sci. Pollut. Res. 23 (10), 9518–9528. doi:10.1007/s11356-016-6178-3
Liu, S. R., Han, P., Hink, L., Prosser, J. I., Wagner, M., and Bruggemann, N. (2017). Abiotic conversion of extracellular NH2OH contributes to N2O emission during ammonia oxidation. Environ. Sci. Technol. 51 (22), 13122–13132. doi:10.1021/acs.est.7b02360
Liu, T., Hu, S. H., Yuan, Z. G., and Guo, J. H. (2019). High-level nitrogen removal by simultaneous partial nitritation, anammox and nitrite/nitrate-dependent anaerobic methane oxidation. WATER Res. 166, 115057. Article 115057. doi:10.1016/j.watres.2019.115057
Liu, T., Liu, S. F., He, S. S., Tian, Z. C., and Zheng, M. S. (2021a). Minimization of N2O emission through intermittent aeration in a sequencing batch reactor (SBR): main behavior and mechanism. Water 13 (2), 210. ARTN 210. doi:10.3390/w13020210
Liu, T., Lu, Y., Zheng, M., Hu, S. H., Yuan, Z. G., and Guo, J. H. (2021b). Efficient nitrogen removal from mainstream wastewater through coupling Partial Nitritation, Anammox and Methane-dependent nitrite/nitrate reduction (PNAM). WATER Res. 206, 117723. Article 117723. doi:10.1016/j.watres.2021.117723
Liu, X., Zhang, Q., Yang, X., Wu, D., Li, Y., and Di, H. (2023). Isolation and characteristics of two heterotrophic nitrifying and aerobic denitrifying bacteria, Achromobacter sp. strain HNDS-1 and Enterobacter sp. strain HNDS-6. Environ. Res. 220, 115240. doi:10.1016/j.envres.2023.115240
Liu, Y. R., He, Y. Y., Chen, F., Ren, S. Q., Zhao, T. H., Zhu, T. T., et al. (2023). Flocs enhance nitrous oxide reduction capacity in a denitrifying biofilm-based system: mechanism of electron competition. Chem. Eng. J. 455, 140599. ARTN 140599. doi:10.1016/j.cej.2022.140599
Liu, Y. W., Ngo, H. H., Guo, W. S., Peng, L., Chen, X. M., Wang, D. B., et al. (2018). Modeling electron competition among nitrogen oxides reduction and N2O accumulation in hydrogenotrophic denitrification. Biotechnol. Bioeng. 115 (4), 978–988. doi:10.1002/bit.26512
Liu, Z. H., Parida, S., Prasad, R., Pandey, R., Sharma, D., and Barman, I. (2022). Vibrational spectroscopy for decoding cancer microbiota interactions: current evidence and future perspective. Seminars Cancer Biol. 86, 743–752. doi:10.1016/j.semcancer.2021.07.004
Lu, H. J., and Chandran, K. (2010). Factors promoting emissions of nitrous oxide and nitric oxide from denitrifying sequencing batch reactors operated with methanol and ethanol as electron donors. Biotechnol. Bioeng. 106 (3), 390–398. doi:10.1002/bit.22704
Ma, H. L., Zhao, Y. X., Yang, K. C., Wang, Y., Zhang, C. G., and Ji, M. (2022). Application oriented bioaugmentation processes: mechanism, performance improvement and scale-up. Bioresour. Technol. 344, 126192. ARTN 126192. doi:10.1016/j.biortech.2021.126192
Ma, Y., Sundar, S., Park, H., and Chandran, K. (2015). The effect of inorganic carbon on microbial interactions in a biofilm nitritation-anammox process. WATER Res. 70, 246–254. doi:10.1016/j.watres.2014.12.006
Mampaey, K. E., De Kreuk, M. K., van Dongen, U. G. J. M., van Loosdrecht, M. C. M., and Volcke, E. I. P. (2016). Identifying N2O formation and emissions from a full-scale partial nitritation reactor. WATER Res. 88, 575–585. doi:10.1016/j.watres.2015.10.047
Mannina, G., Chandran, K., Capodici, M., Cosenza, A., Di Trapani, D., and van Loosdrecht, M. C. M. (2018a). Greenhouse gas emissions from membrane bioreactors: analysis of a two-year survey on different MBR configurations. Water Sci. Technol. 78 (4), 896–903. doi:10.2166/wst.2018.366
Mannina, G., Ekama, G. A., Capodici, M., Cosenza, A., Di Trapani, D., and Odegaard, H. (2018b). Integrated fixed-film activated sludge membrane bioreactors versus membrane bioreactors for nutrient removal: a comprehensive comparison. J. Environ. Manag. 226, 347–357. doi:10.1016/j.jenvman.2018.08.006
Mannina, G., Ekama, G. A., Capodici, M., Cosenza, A., Di Trapani, D., Odegaard, H., et al. (2018c). Influence of carbon to nitrogen ratio on nitrous oxide emission in an integrated fixed film activated sludge membrane BioReactor plant. J. Clean. Prod. 176, 1078–1090. doi:10.1016/j.jclepro.2017.11.222
Meinhold, J., Filipe, C. D. M., Daigger, G. T., and Isaacs, S. (1999). Characterization of the denitrifying fraction of phosphate accumulating organisms in biological phosphate removal. Water Sci. Technol. 39 (1), 31–42. doi:10.2166/wst.1999.0007
Miao, L., Wang, S. Y., Li, B. K., Cao, T. H., Xue, T. L., and Peng, Y. Z. (2015). Advanced nitrogen removal via nitrite using stored polymers in a modified sequencing batch reactor treating landfill leachate. Bioresour. Technol. 192, 354–360. doi:10.1016/j.biortech.2015.05.013
Mogrovejo, D. C., Perini, L., Gostincar, C., Sepcic, K., Turk, M., Ambrozic-Avgustin, J., et al. (2020). Prevalence of antimicrobial resistance and hemolytic phenotypes in culturable arctic bacteria. Front. Microbiol. 11, 570. ARTN 570. doi:10.3389/fmicb.2020.00570
Nguyen, H. T., Nguyen, L. D., Le, C. P., Hoang, N. D., and Dinh, H. T. (2023). Nitrogen and carbon removal from anaerobic digester effluents with low carbon to nitrogen ratios under feammox conditions. Bioresour. Technol. 371, 128585. ARTN 128585. doi:10.1016/j.biortech.2023.128585
Ni, B. J., and Yuan, Z. G. (2015). Recent advances in mathematical modeling of nitrous oxides emissions from wastewater treatment processes. WATER Res. 87, 336–346. doi:10.1016/j.watres.2015.09.049
Nie, W. B., Xie, G. J., Ding, J., Lu, Y., Liu, B. F., Xing, D. F., et al. (2019). High performance nitrogen removal through integrating denitrifying anaerobic methane oxidation and Anammox: from enrichment to application. Environ. Int. 132, 105107. ARTN. doi:10.1016/j.envint.2019.105107
Nie, W. B., Xie, G. J., Ding, J., Peng, L., Lu, Y., Tan, X., et al. (2020). Operation strategies of n-DAMO and Anammox process based on microbial interactions for high rate nitrogen removal from landfill leachate. Environ. Int. 139, 105596. doi:10.1016/j.envint.2020.105596
Oehmen, A., Zeng, R. J., Yuan, Z. G., and Keller, J. (2005). Anaerobic metabolism of propionate by polyphosphate-accumulating organisms in enhanced biological phosphorus removal systems. Biotechnol. Bioeng. 91 (1), 43–53. doi:10.1002/bit.20480
Okabe, S., Oshiki, M., Takahashi, Y., and Satoh, H. (2011). N2O emission from a partial nitrification-anammox process and identification of a key biological process of N2O emission from anammox granules. WATER Res. 45 (19), 6461–6470. doi:10.1016/j.watres.2011.09.040
Pan, Y. T., Ni, B. J., Bond, P. L., Ye, L., and Yuan, Z. G. (2013a). Electron competition among nitrogen oxides reduction during methanol-utilizing denitrification in wastewater treatment. WATER Res. 47 (10), 3273–3281. doi:10.1016/j.watres.2013.02.054
Pan, Y. T., Ni, B. J., and Yuan, Z. G. (2013b). Modeling electron competition among nitrogen oxides reduction and N2O accumulation in denitrification. Environ. Sci. Technol. 47 (19), 11083–11091. doi:10.1021/es402348n
Park, K. Y., Inamori, Y., Mizuochi, M., and Ahn, K. H. (2000). Emission and control of nitrous oxide from a biological wastewater treatment system with intermittent aeration. J. Biosci. Bioeng. 90 (3), 247–252. doi:10.1016/S1389-1723(00)80077-8
Peng, L., Ni, B. J., Ye, L., and Yuan, Z. G. (2015a). The combined effect of dissolved oxygen and nitrite on N2O production by ammonia oxidizing bacteria in an enriched nitrifying sludge. WATER Res. 73, 29–36. doi:10.1016/j.watres.2015.01.021
Peng, L., Ni, B. J., Ye, L., and Yuan, Z. G. (2015b). N2O production by ammonia oxidizing bacteria in an enriched nitrifying sludge linearly depends on inorganic carbon concentration. WATER Res. 74, 58–66. doi:10.1016/j.watres.2015.02.003
Peng, Y. Y., He, S. B., and Wu, F. (2021). Biochemical processes mediated by iron-based materials in water treatement: enhancing nitrogen and phosphorus removal in low C/N ratio wastewater. Sci. TOTAL Environ. 775, 145137. ARTN 145137. doi:10.1016/j.scitotenv.2021.145137
Pollice, A., Tandoi, V., and Lestingi, C. (2002). Influence of aeration and sludge retention time on ammonium oxidation to nitrite and nitrate. WATER Res. 36 (10), 2541–2546. doi:10.1016/S0043-1354(01)00468-7
Quan, X. C., Zhang, M. C., Lawlor, P. G., Yang, Z. F., and Zhan, X. M. (2012). Nitrous oxide emission and nutrient removal in aerobic granular sludge sequencing batch reactors. WATER Res. 46 (16), 4981–4990. doi:10.1016/j.watres.2012.06.031
Raghoebarsing, A. A., Pol, A., van de Pas-Schoonen, K. T., Smolders, A. J. P., Ettwig, K. F., Rijpstra, W. I. C., et al. (2006). A microbial consortium couples anaerobic methane oxidation to denitrification. NATURE 440 (7086), 918–921. doi:10.1038/nature04617
Rathnayake, R. M. L. D., Song, Y., Tumendelger, A., Oshiki, M., Ishii, S., Satoh, H., et al. (2013). Source identification of nitrous oxide on autotrophic partial nitrification in a granular sludge reactor. WATER Res. 47 (19), 7078–7086. doi:10.1016/j.watres.2013.07.055
Ren, Y. G., Wang, J. H., Li, H. F., Zhang, J., Qi, P. Y., and Hu, Z. (2013). Nitrous oxide and methane emissions from different treatment processes in full-scale municipal wastewater treatment plants. Environ. Technol. 34 (21), 2917–2927. doi:10.1080/09593330.2012.696717
Ren, Y. G., Wang, J. H., Xu, L., Liu, C., Zong, R. Q., Yu, J. L., et al. (2015). Direct emissions of N2O, CO2, and CH4 from A/A/O bioreactor systems: impact of influent C/N ratio. Environ. Sci. Pollut. Res. 22 (11), 8163–8173. doi:10.1007/s11356-015-4408-8
Richardson, D., Felgate, H., Watmough, N., Thomson, A., and Baggs, E. (2009). Mitigating release of the potent greenhouse gas N2O from the nitrogen cycle - could enzymic regulation hold the key? Trends Biotechnol. 27 (7), 388–397. doi:10.1016/j.tibtech.2009.03.009
Schalk-Otte, S., Seviour, R. J., Kuenen, J. G., and Jetten, M. S. M. (2000). Nitrous oxide (N2O) production by Alcaligenes faecalis during feast and famine regimes. WATER Res. 34 (7), 2080–2088. doi:10.1016/S0043-1354(99)00374-7
Schmidt, I., Sliekers, O., Schmid, M., Bock, E., Fuerst, J., Kuenen, J. G., et al. (2003). New concepts of microbial treatment processes for the nitrogen removal in wastewater. Fems Microbiol. Rev. 27 (4), 481–492. doi:10.1016/S0168-6445(03)00039-1
Shi, Y. Q., Hu, Y. Y., Liang, D. H., Wang, G. B., Xie, J. Y., and Zhu, X. Q. (2022). Enhanced denitrification of sewage via bio-microcapsules embedding heterotrophic nitrification-aerobic denitrification bacteria Acinetobacter pittii SY9 and corn coba SY9 and corn cob. Bioresour. Technol. 358, 127260. doi:10.1016/j.biortech.2022.127260
Soler-Jofra, A., Stevens, B., Hoekstra, M., Picioreanu, C., Sorokin, D., van Loosdrecht, M. C. M., et al. (2016). Importance of abiotic hydroxylamine conversion on nitrous oxide emissions during nitritation of reject water. Chem. Eng. J. 287, 720–726. doi:10.1016/j.cej.2015.11.073
Song, K., Harper, W. F., Hori, T., Riya, S., Hosomi, M., and Terada, A. (2015). Impact of carbon sources on nitrous oxide emission and microbial community structure in an anoxic/oxic activated sludge system. Clean Technol. Environ. Policy 17 (8), 2375–2385. doi:10.1007/s10098-015-0979-9
Su, Z. P., Li, Y., Pan, L. Q., He, Z. Y., Dou, L., Liu, L. P., et al. (2021). Nitrogen removal performance, quantitative detection and potential application of a novel aerobic denitrifying strain, Pseudomonas sp. GZWN4 isolated from aquaculture water. Bioprocess Biosyst. Eng. 44 (6), 1237–1251. doi:10.1007/s00449-021-02523-9
Sun, S. C., Cheng, X., and Sun, D. Z. (2013). Emission of N2O from a full-scale sequencing batch reactor wastewater treatment plant: characteristics and influencing factors. Int. Biodeterior. Biodegrad. 85, 545–549. doi:10.1016/j.ibiod.2013.03.034
Sun, S. P., Nacher, C. P. I., Merkey, B., Zhou, Q., Xia, S. Q., Yang, D. H., et al. (2010). Effective biological nitrogen removal treatment processes for domestic wastewaters with low C/N ratios: a review. Environ. Eng. Sci. 27 (2), 111–126. doi:10.1089/ees.2009.0100
Sun, S. S., Liu, J., Zhang, M. P., and He, S. B. (2019). Simultaneous improving nitrogen removal and decreasing greenhouse gas emission with biofilm carriers addition in ecological floating bed. Bioresour. Technol. 292, 121944. ARTN 121944. doi:10.1016/j.biortech.2019.121944
Sun, Y., Chen, Z., Wu, G. X., Wu, Q. Y., Zhang, F., Niu, Z. B., et al. (2016). Characteristics of water quality of municipal wastewater treatment plants in China: implications for resources utilization and management. J. Clean. Prod. 131, 1–9. doi:10.1016/j.jclepro.2016.05.068
Sun, Y., Su, J. F., Ali, A., Huang, T. L., Zhang, S., and Min, Y. T. (2023). Enhanced nitrate and cadmium removal performance at low carbon to nitrogen ratio through immobilized redox mediator granules and functional strains in a bioreactor. Chemosphere 312, 137255. ARTN 137255. doi:10.1016/j.chemosphere.2022.137255
Terada, A., Sugawara, S., Hojo, K., Takeuchi, Y., Riya, S., Harper, W. F., et al. (2017). Hybrid nitrous oxide production from a partial nitrifying bioreactor: hydroxylamine interactions with nitrite. Environ. Sci. Technol. 51 (5), 2748–2756. doi:10.1021/acs.est.6b05521
Tian, Z. C., Zhou, N., You, W. B., He, D., Chang, F., and Zheng, M. S. (2021). Mitigating NO and N2O emissions from a pilot-scale oxidation ditch using bioaugmentation of immobilized aerobic denitrifying bacteria. Bioresour. Technol. 340, 125704. Article 125704. doi:10.1016/j.biortech.2021.125704
Todt, D., and Dorsch, P. (2016). Mechanism leading to N2O production in wastewater treating biofilm systems. Rev. Environ. Sci. Bio-Technology 15 (3), 355–378. doi:10.1007/s11157-016-9401-2
Tseng, C. C., Potter, T. G., and Koopman, B. (1998). Effect of influent chemical oxygen demand to nitrogen ratio on a partial nitrification complete denitrification process. WATER Res. 32 (1), 165–173. doi:10.1016/S0043-1354(97)00195-4
Wang, D. Q., He, P. S., Wang, Z. J., Li, G. Y., Majed, N., and Gu, A. Z. (2020). Advances in single cell Raman spectroscopy technologies for biological and environmental applications. Curr. Opin. Biotechnol. 64, 218–229. doi:10.1016/j.copbio.2020.06.011
Wang, H. C., Jiang, C. C., Wang, X., Xu, S. J., and Zhuang, X. L. (2021). Application of internal carbon source from sewage sludge: a vital measure to improve nitrogen removal efficiency of low C/N wastewater. Water 13 (17), 2338. ARTN 2338. doi:10.3390/w13172338
Wang, S., Zhao, J. Q., and Huang, T. (2019). High NO and N2O accumulation during nitrite denitrification in lab-scale sequencing batch reactor: influencing factors and mechanism. Environ. Sci. Pollut. Res. 26 (33), 34377–34387. doi:10.1007/s11356-019-06391-5
Wang, Y. R., Deng, M., Li, B. Q., Li, L., Oon, Y. S., Zhao, X. L., et al. (2023). High nitrous oxide (N2O) greenhouse gas reduction potential of Pseudomonas sp. YR02 under aerobic condition. Bioresour. Technol. 378, 128994. ARTN 128994. doi:10.1016/j.biortech.2023.128994
Weissbach, M., Drewes, J. E., and Koch, K. (2018). Application of the oxidation reduction potential (ORP) for process control and monitoring nitrite in a Coupled Aerobic-anoxic Nitrous Decomposition Operation (CANDO). Chem. Eng. J. 343, 484–491. doi:10.1016/j.cej.2018.03.038
Wu, G. X., Zheng, D. R., and Xing, L. Z. (2014). Nitritation and N2O emission in a denitrification and nitrification two-sludge system treating high ammonium containing wastewater. Water 6 (10), 2978–2992. doi:10.3390/w6102978
Wu, L., Chen, X. M., Wei, W., Liu, Y. W., Wang, D. B., and Ni, B. J. (2020). A critical review on nitrous oxide production by ammonia-oxidizing archaea. Environ. Sci. Technol. 54 (15), 9175–9190. doi:10.1021/acs.est.0c03948
Xu, N., Liao, M., Liang, Y. Q., Guo, J. W., Zhang, Y. H., Xie, X. M., et al. (2021). Biological nitrogen removal capability and pathways analysis of a novel low C/N ratio heterotrophic nitrifying and aerobic denitrifying bacterium (Bacillus thuringiensis strain WXN-23). Environ. Res. 195, 110797. ARTN 110797. doi:10.1016/j.envres.2021.110797
Xu, Y. F., Liu, Y. H., Zhang, W., Wang, Z. Z., and Li, S. M. (2018). Optimization of C/N and carbon types on the denitrification biofilter for advanced wastewater treatment. Desalination Water Treat. 119, 107–117. doi:10.5004/dwt.2018.22430
Yan, P., Li, K., Guo, J. S., Zhu, S. X., Wang, Z. K., and Fang, F. (2019). Toward N2O emission reduction in a single-stage CANON coupled with denitrification: investigation on nitrite simultaneous production and consumption and nitrogen transformation. Chemosphere 228, 485–494. doi:10.1016/j.chemosphere.2019.04.148
Yan, X., Han, Y. P., Li, Q. L., Sun, J. H., and Su, X. F. (2016). Impact of internal recycle ratio on nitrous oxide generation from anaerobic/anoxic/oxic biological nitrogen removal process. Biochem. Eng. J. 106, 11–18. doi:10.1016/j.bej.2015.11.005
Yan, X., Zheng, J. X., Han, Y. P., Liu, J. W., and Sun, J. H. (2017). Effect of influent C/N ratio on N2O emissions from anaerobic/anoxic/oxic biological nitrogen removal processes. Environ. Sci. Pollut. Res. 24 (30), 23714–23724. doi:10.1007/s11356-017-0019-x
Yang, J. J., Trela, J., and Plaza, E. (2016). Nitrous oxide emissions from one-step partial nitritation/anammox processes. Water Sci. Technol. 74 (12), 2870–2878. doi:10.2166/wst.2016.454
Yang, R., Yuan, L. J., Wang, R., He, Z. X., and Chen, X. (2021). New insight on the regulation of N2O production in aerobic condition: an N2O metabolic perspective based on enzymatic analysis of nitrous oxide reductase. J. Water Process Eng. 41, 102090. ARTN 102090. doi:10.1016/j.jwpe.2021.102090
Yue, X., Yu, G. P., Liu, Z. H., Lu, Y. Q., and Li, Q. H. (2018). Start-up of the completely autotrophic nitrogen removal over nitrite process with a submerged aerated biological filter and the effect of inorganic carbon on nitrogen removal and microbial activity. Bioresour. Technol. 254, 347–352. doi:10.1016/j.biortech.2018.01.107
Zawartka, P., Burchart-Korol, D., and Blaut, A. (2020). Model of carbon footprint assessment for the life cycle of the system of wastewater collection, transport and treatment. Sci. Rep. 10 (1), 5799. ARTN 5799. doi:10.1038/s41598-020-62798-y
Zhang, M., Wang, S. Y., Ji, B., and Liu, Y. (2019). Towards mainstream deammonification of municipal wastewater: partial nitrification-anammox versus partial denitrification-anammox. Sci. TOTAL Environ. 692, 393–401. doi:10.1016/j.scitotenv.2019.07.293
Zhang, X. J., Yu, B. Y., Zhang, N., Zhang, H. J., Wang, C. N., and Zhang, H. Z. (2016). Effect of inorganic carbon on nitrogen removal and microbial communities of CANON process in a membrane bioreactor. Bioresour. Technol. 202, 113–118. doi:10.1016/j.biortech.2015.11.083
Zhang, X. J., Zhang, N., Wang, L. N., Zheng, K. W., Fu, H. Q., Chen, T., et al. (2017). Bioactivity and microbial community structure of nitrite-oxidizing bacteria in five membrane bioreactors operated as CANON process with different C/N ratio. Ecol. Eng. 99, 159–163. doi:10.1016/j.ecoleng.2016.11.049
Zhang, Y., Ji, G., and Wang, R. (2016). Drivers of nitrous oxide accumulation in denitrification biofilters with low carbon:nitrogen ratios. Water Res. 106, 79–85. doi:10.1016/j.watres.2016.09.046
Zhang, Z. M., Yu, Z. D., Dong, J. J., Wang, Z. H., Ma, K., Xu, X. Y., et al. (2018). Stability of aerobic granular sludge under condition of low influent C/N ratio: correlation of sludge property and functional microorganism. Bioresour. Technol. 270, 391–399. doi:10.1016/j.biortech.2018.09.045
Zhao, W., Wang, Y. Y., Liu, S. H., Pan, M. L., Yang, J., and Chen, S. W. (2013). Denitrification activities and N2O production under salt stress with varying COD/N ratios and terminal electron acceptors. Chem. Eng. J. 215, 252–260. doi:10.1016/j.cej.2012.10.084
Zheng, M. S., Tian, Y. H., Liu, T., Ma, T., Li, L., Li, C., et al. (2015). Minimization of nitrous oxide emission in a pilot-scale oxidation ditch: generation, spatial variation and microbial interpretation. Bioresour. Technol. 179, 510–517. doi:10.1016/j.biortech.2014.12.027
Zheng, M. S., Zhou, N., He, S. S., Chang, F., Zhong, J., Xu, S., et al. (2021). Nitrous oxide (N2O) emissions from a pilot-scale oxidation ditch under different COD/N ratios, aeration rates and two shock-load conditions. J. Environ. Manag. 280, 111657. Article 111657. doi:10.1016/j.jenvman.2020.111657
Zhou, G. W., Yang, X. R., Li, H., Marshall, C. W., Zheng, B. X., Yan, Y., et al. (2016). Electron shuttles enhance anaerobic ammonium oxidation coupled to iron(III) reduction. Environ. Sci. Technol. 50 (17), 9298–9307. doi:10.1021/acs.est.6b02077
Zhou, N., Dang, C. Y., Zhao, Z. R., He, S. S., Zheng, M. S., Liu, W., et al. (2019). Role of sludge retention time in mitigation of nitrous oxide emission from a pilot-scale oxidation ditch. Bioresour. Technol. 292, 121961. Article 121961. doi:10.1016/j.biortech.2019.121961
Zhou, X., Song, J. J., Wang, G. L., Yin, Z. Y., Cao, X. W., and Gao, J. F. (2020). Unravelling nitrogen removal and nitrous oxide emission from mainstream integrated nitrification-partial denitrification-anammox for low carbon/nitrogen domestic wastewater. J. Environ. Manag. 270, 110872. ARTN 110872. doi:10.1016/j.jenvman.2020.110872
Keywords: BNR, carbon and nitrogen ratio (C/N ratio), N2O emission, anaerobic ammonium oxidation (anammox), heterotrophic denitrification (HD)
Citation: Xie Y, Jiang C, Kuai B, Xu S and Zhuang X (2023) N2O emission reduction in the biological nitrogen removal process for wastewater with low C/N ratios: mechanisms and strategies. Front. Bioeng. Biotechnol. 11:1247711. doi: 10.3389/fbioe.2023.1247711
Received: 28 June 2023; Accepted: 16 November 2023;
Published: 29 November 2023.
Edited by:
Krist V. Gernaey, Technical University of Denmark, DenmarkReviewed by:
Mingyi Xu, Technical University of Denmark, DenmarkCopyright © 2023 Xie, Jiang, Kuai, Xu and Zhuang. This is an open-access article distributed under the terms of the Creative Commons Attribution License (CC BY). The use, distribution or reproduction in other forums is permitted, provided the original author(s) and the copyright owner(s) are credited and that the original publication in this journal is cited, in accordance with accepted academic practice. No use, distribution or reproduction is permitted which does not comply with these terms.
*Correspondence: Cancan Jiang, Y2NqaWFuZ0ByY2Vlcy5hYy5jbg==; Shengjun Xu, c2p4dUByY2Vlcy5hYy5jbg==
Disclaimer: All claims expressed in this article are solely those of the authors and do not necessarily represent those of their affiliated organizations, or those of the publisher, the editors and the reviewers. Any product that may be evaluated in this article or claim that may be made by its manufacturer is not guaranteed or endorsed by the publisher.
Research integrity at Frontiers
Learn more about the work of our research integrity team to safeguard the quality of each article we publish.