- 1Department of Surgery, Faculty of Medicine, Instituto de Investigación Biomédica de Salamanca (IBSAL), Universidad de Salamanca, Salamanca, Spain
- 2Department of Medicine and Medical Specialties, Faculty of Health Sciences, Universidad Alcalá de Henares, Madrid, Spain
- 3Department of Dentistry, Universidad Federico Henríquez y Carvajal, Santo Domingo, Dominican Republic
- 4Faculty of Dentistry, Universidad Alfonso X El Sabio, Madrid, Spain
Introduction: Rough titanium surfaces biofunctionalised by osteogenic proteins, such as BMP-2, have been shown to accelerate the osseointegration process and reduce waiting times for prosthetic loading. The preclinical study presented here compared the bone in contact with the implant and bone neoformation and density between titanium (Ti) implants with a conventional etched surface (SLA type) and others treated with carboxyethylphosphonic acid (CEPA) and bone morphogenetic protein 2 (BMP-2), after 4 weeks of implantation in the tibia of a minipig model.
Methods: Sixteen implants (eight experimental and eight control) of Ti-Al16-V4 with a tapered screw design and internal hexagonal connection were randomly inserted into the tibiae of four minipigs, four in each tibia. The experimental implants were treated with CEPA and BMP-2 and sterilised with gamma radiation (25 KG). The insertion torque was 40 N and primary stability was measured with the Osstell® device (ISQ 64 ± 2.6). Five bone parameters were evaluated: bone in contact with the implant (BIC), bone in contact with the corrected implant (BICc), new bone formation (BV/TV), bone density between threads (BAI/TA) and peri-implant bone density (BAP/TA). A histomorphometric study was performed and the samples were digitised with Adobe Photoshop Cs6. Statistical analysis of the variables was performed using SAS 9.4.
Results: After a period of 4 weeks, no significant clinical signs were observed and all implants were integrated. Light microscopy of the experimental group revealed an ICB with no signs of fiber tissue, but with areas of ectopic new bone in the medullary space. Statistical analysis showed significant results for BIC and BICc (p = 0.0001 and p = 0.001, respectively). No statistical signification was found for the other parameters evaluated.
Conclusion: Despite the limitations of this study, our results demonstrated that dental implant surfaces treated with CEPA and BMP-2 improve their biological response to osseointegration.
1 Introduction
Since Per-Ingvar Brånemark in 1969 demonstrated the long-term success of Ti dental implants and André Schroeder in 1976 introduced the rough surface, both macro- and micro design have undergone profound transformations (Brånemark et al., 1969; Schroeder et al., 1976).
In 1990 (Wilke et al., 1990) published the first in vivo study on acid-etched sandblasted implants (SLA). Subsequently, multiple investigations have studied its biological properties (Cochran et al., 1998; Buser et al., 1999; Cochran et al., 2002; Li et al., 2002).
Ti and its alloys, despite their good biocompatibility, require long periods of time to ensure their osseointegration. To accelerate and ensure their osseointegration, different surface coating methods have been used, such as hydroxyapatite (HA) or calcium phosphate (Ca/P), although these types of coatings have certain limitations and cannot be applied to implants with porous surfaces, nor to those in which it is desired to incorporate biological molecules into the coating (de Groot et al., 2011). In addition, dental implants require biocompatibility with soft tissues, to achieve adhesion of the gingival epithelium and certain antibacterial properties that prevent the formation of biofilms (Hanawa, 2010).
The acid etching process, besides increasing roughness and cleaning the surface, it has been demonstrated that this type of etching increases cell adhesion and bone neoformation, improving osseointegration (Cho and Park, 2003; Stepanovska et al., 2020). Some studies have shown that dual acid treatments will improve osseointegration, since the surface achieved will largely mimic the bone structure (Jang et al., 2017; Giner et al., 2018).
Phosphonic acids are organophosphorus compounds, poorly soluble in water, which have been used, due to their bioactive properties, for surface functionalization, in particular to immobilize organic molecules on the surface of metal oxides such as Ti oxide (TiO2) (Zeininger et al., 2016; Sevrai et al., 2017). Some investigations have used phosphonic acid molecules for the formation of self-assembled monolayers (SAM) on the Ti surface (Gawalt et al., 2001; Adden et al., 2006) that would facilitate the immobilization of bioactive molecules, such as BMP-2, with the capacity to differentiate a good number of mesenchymal cells into osteoblasts and, therefore, generate increased bone growth at the local level (Hoffmann et al., 2001). BMP-2 is considered a potent osteogenic growth factor, capable of promoting cell differentiation, inducing bone healing by recruiting bone-forming cells and providing biomaterials with enhanced osteoinductive capacity (Chen et al., 2004). Currently, it has two FDA-approved indications: treatment of unconsolidated tibial fractures and treatment of open tibial fractures treated with intramedullary fixations (Ronga et al., 2013). Some studies have evaluated its osteoinductive properties in critical defects in different experimental models, reporting high osteoblastic activity and physiological bone regeneration at the experimental sites (von Walter et al., 2008; Kolk et al., 2019). In addition, it has been described that it would act as an accelerator of osteoblastic differentiation, giving rise to a new bone matrix, which would include mineral precursors and collagen (de Queiroz Fernandes et al., 2018). All this demonstrates that BMP-2 can potentially be applied in peri-implant bone regeneration and that it would have the capacity to reduce waiting times, while guaranteeing osseointegration.
The objective of our research was to analyze and compare the effect of carboxyethylphosphonic acid (HO2C-CR1H-CR2H-PO3H2) (CEPA) and bone morphogenetic protein (BMP-2) on the osseointegration of dental implants placed in an experimental animal model, based on the null hypothesis (H0) that there are no differences in the histomorphometric parameters related to osseointegration between dental implants with surfaces treated with CEPA and BMP-2 and implants with SLA surfaces.
2 Material and methods
2.1 Study design; implants design; study animals
The study protocol consisted in randomly inserting 16 conical implants (four in each tibia of each animal), manufactured with grade IV Ti (90wt% Ti, 6wt% Al and 4wt% V) of 4 mm Ø × 10 mm length, with internal connection hexagon and conventional treatment of the implant surface, by coarse-grained sandblasting and acid etching (SLA), in the tibiae of 4 minipigs. Two study groups were established, with 8 implants in each group: the experimental group with CEPA-treated implants functionalized with BMP-2 and the control group with SLA-surfaced implants. The implants of the experimental group received surface treatment by CEPA (Figure 1.) and BMP-2, according to the method proposed by Aresti et al. (2021). For this purpose, they were subjected to a dilution of 50 mL of tetrahydrofuran (THF) (Uvasol®, Madrid, Spain) and 55 mg of CEPA for 1 day at 76°C. Subsequently, CEPA was activated with a solution of 5 mL of distilled H2O, 175 mL of ethyl-3-(3-dimethylaminopropyl) carboxyamide (EDC) and 54 mg of N-hydroxysulfamide (NHS) for 15 min at room temperature. The pH stability (pH 7) was checked using a pH-meter (MP230, Mettler Toledo®, Barcelona, Spain) during the whole process. EDC activates the carboxyl and amine groups, reacting with the carboxyl group to form an O-acylisourea intermediate; however, if it does not react with the amine, it hydrolyzes and regenerates the carboxyl group, thus incorporating NHS. In the presence of NHS, EDC can be used to convert the carboxyl groups to amine-reactive NHS esters by activating CEPA with EDC and NHS to react with the amino groups of BMP-2. Once the carboxyl groups were activated, 20 mg of BMP-2 was incubated for 1 h at 37°C. To remove impurities, the implants were exposed to ultrasonic waves and packed in laminar flow cabinets in sterile atmosphere; they were sterilized by 25 KGy gamma radiation. The implants were concealed from the operator by means of a depistage wrap.
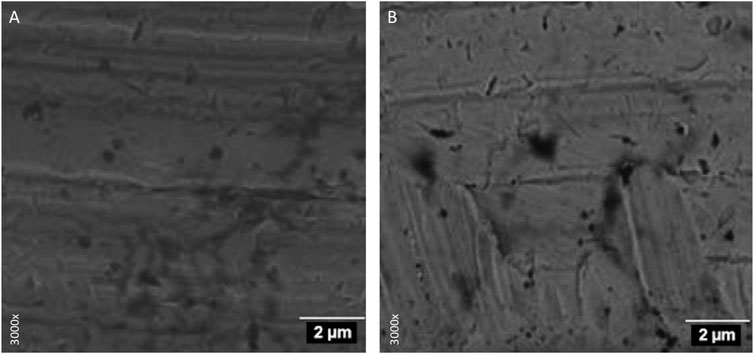
FIGURE 1. Electron microscopy. Implant platform surface: (A) belonging to the control group and (B) treated with carboxylphosphonic acid.
Four Landrace Large White minipigs between 18 and 20 weeks of age were selected for the study in accordance with the European Committee for Standardization guidelines for bone tissue studies. The randomized experimental investigation was approved on 31 January 2013 by the Animal Experimentation Ethics Committee (CEEA) of the Hospital Universitario Puerta de Hierro Majadahonda (Madrid, Spain) and the Instituto de Investigación Puerta de Hierro Majadahonda, with CEEA Code: 017/2013. The study was conducted in accordance with the ethical principles of the ARRIVE guidelines on animal experimentation.
2.2 Surgeries; euthanasia procedure
General anesthesia, performed by a specialized veterinarian, was induced with 0.2–0.4 mg/kg propofol intravenously (Diprivan®, AstraZeneca, Cambridge, United Kingdom), using a 20 G needle and epidural anesthesia with bupivacaine (Bupinex®, Richmond Vet Pharma, Buenos Aires, Argentina) and fentanyl (Fentanyl®, Kilab, Buenos Aires, Argentina). In addition, local anesthesia was infiltrated in the surgical area with 4% articaine and epinephrine 1:200,000 (Ultracain®, Normon, Madrid, Spain). A N°7 endotracheal tube with a balloon cuff was placed and connected to a circular anesthesia circuit (Leon Plus, Heinen&Löwenstein, Bad Ems, Germany). Multimodal analgesia was used during the study, using medetomidine 0.01 mg/kg (Medetor®, Virbac, Carros, France), ketamine 5.0 mg/kg (Ketonal 50®, Richmond Vet Pharma, Buenos Aires, Argentina), midazolam 0.2 mg/kg (Dormicum®, Roche S.A., Basel, Switzerland) and atropine 0.02 mg/kg (Atropina®, Pharmavet, Bogotá, Colombia).
Next, after making an incision in the anterior aspect of the tibia (Figure 2A), a drilling sequence was performed, according to the implant manufacturer’s recommendations, using a micromotor (AM-25 E RM, W&H, Bürmoos, Austria) and a 20:1 reduction contra-angle handpiece (WS-75 LG, W&H, Bürmoos, Austria) with profusion of saline (Vitulia® 0.9%, Barcelona, Spain). Subsequently, four implants, with their corresponding locking screws, were placed in the tibial crest of each animal (Figures 2B, C), using the randomization program Surgimplant IPX, (Galimplant, Sarria, Lugo, Spain). The placement torque was 40N with a primary stability ISQ 64 ± 2.6 (Osstell® device, Ostell/Integration Diagnostics, Gothenburg, Sweden) (Figure 2D).
The incisions were closed by simple stitches in two planes: in the deep plane a polyglactin rapid resorbable suture (5/0) was used and in the superficial plane a non-resorbable braided silk (3/0) was used (Figures 2E, F).
The animals were euthanized randomly, 4 weeks after surgery, by overdose of sodium pentobarbital, under premedication administered intramuscularly, with Zolacepam-Tiletamine-Medetomidine (Zoletil 5 mg/kg, Medetonin 0.1 mg/kg).
2.3 Histomorphometric analysis
Implant-bearing tibiae were dissected and extracted and fixed in 10% buffered formalin solution for 2 weeks to allow histomorphometric processing. Subsequently, bone-implant fragments were extracted with an oscillating saw in 16 mm thick serial sagittal sections and dehydrated in increasing alcohol solutions (80, 96%, and 100%) for 3 days. The samples were embedded in glycolmethacrylate (GMA; 2-hydroxyethyl methacrylate, HEMA, JB-4; JB-4 Plus) (Technovit 7200 VLC, Heraeus Kulzer, Wehrheim, Germany). Finally, they were sectioned into 50 µm thick slices, stained with the Levai Laczko staining technique (Kuchler et al., 2013) and examined with light optical microscopy (BX51, Olympus, Tokyo, Japan) by an experienced pathologist blinded to the randomization of the study groups. In addition, histological images were processed, digitized and loaded into a computer program (Adobe Photoshop Cs6, San Jose, CA, USA; Cell Sens Dimensions, Olympus, Tokyo, Japan) to evaluate, on a percentage basis, 5 parameters, within an area of interest (AOI) of 5 mm × 5 mm. For the calculation of densities, regions of interest (ROI) were established with a thickness of 300 microns, drawn parallel to the peak of the implant threads (Figures 3A, B). The Adobe Photoshop Cs6 program encoded in a digitizer tablet, the bone surrounding the implants, in yellow color the new bone and in pink color the old bone (Figure 3C). The parameters evaluated were:
- Bone-to-implant contact (BIC): percentage of the dental implant surface in contact with the surrounding bone.
- Corrected bone-to-implant contact (BICc): length of bone in contact with the surface of the dental implant, excluding regions not covered by bone.
- New bone formation (BV/TV): area of new bone formed after dental implant placement.
- Bone density between threads (BAI/TA): area of threads covered by surrounding bone.
- Peri-implant bone density (BAP/TA): area of bone growing along the implant.
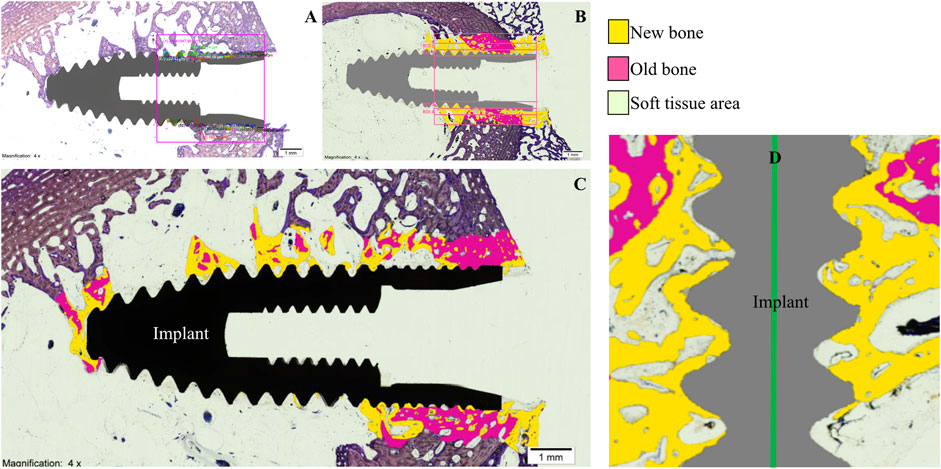
FIGURE 3. (A), area of interest; (B), regions of interest; (C), digitized image; (D), new bone in contact with the implant surface.
2.4 Statistical test
Statistical analysis of all variables was performed with SAS 9.4 (SAS Institute Inc., Cary, NC, United States). Descriptive statistics were expressed as means, medians and standard deviations (SD) for quantitative variables. Comparative analysis was performed by comparing the histomorphometric parameters BIC (%), BICc (%), BV/TV (%), BAI/TA (%) and BAP/TA (%) between the SLA and BMP-2 study groups, using Student’s t-test and the nonparametric Mann-Whitney test. Shapiro-Wilk test was used to contrast the normality of data. Statistical significance was set at p < 0.05.
3 Results
3.1 General clinical observations
Sixteen 4 mm Ø x 10 mm long implants were placed in the tibial crest of 4 minipigs. All animals recovered well and after 4 weeks, at the end of the study, wound healing was uneventful, with no signs of infection, inflammation or significant subcutaneous seroma; keloid scarring was only observed in 2 of the animals used. All implants were integrated, so a total of 16 implants were available for evaluation.
3.2 Histomorphometry
Histological analysis by light microscopy of the longitudinal sections of the samples from the experimental group revealed a BIC with interrupted medullary spaces at the bone-implant interface, but no signs of fibrous tissue (Figure 3D). The images with the implant in black show the longitudinal section of the specimen before processing by the software. The frames with the implant in gray distinguish, using the software, the areas of old bone (in pink), the areas of new bone (in yellow) and the areas of soft tissue (colorless) (Figure 3C). The experimental implants, unlike the control group, presented more areas of ectopic new bone in the medullary space (Figure 4).
3.3 Statistical results
Using a 95% confidence interval, with a significance level <0.05, the means, medians and SD were evaluated. Student’s t-test and the nonparametric Mann-Whitney test showed statistically significant differences between groups for the variables BIC (SLA 34.001% ± 9.921% and BMP-2 60.807% ± 10.419%; p = 0.0001) and BICc (SLA 43.085% ± 10.766% and BMP-2 64.070% ± 8.754%; p = 0.001). Both variables presented a normal distribution of data (Tables 1, 2; Figures 5, 6). The variables BV/TV, BAI/TA and BAP/TA did not show statistical significance between the study groups (p = 0.420, p = 0.644 and p = 0.286, respectively) (Table 3).
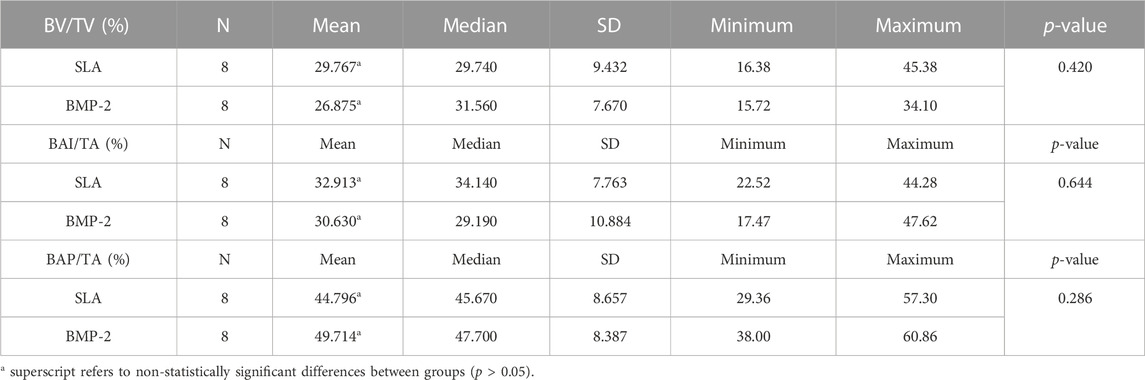
TABLE 3. Descriptive statistics of BV/TV (%), BAI/TA (%) and BAP/TA (%) histomorphometric parameters.
4 Discussion
This study compared the effect on osseointegration between implants treated with carboxyethylphosphonic acid and BMP-2 and implants with conventional SLA surface in an extraoral minipig tibia model. The results showed that the experimental implants had significantly higher osseointegration parameters (BIC and BICc) than the implants with SLA surface, although the BV/TV, BAI/TA and BAP/TA values did not differ from the control group.
The ability of phosphonic acids to immobilize bioactive organic molecules on the surface of metal oxides, such as TiO2, confers them specific qualities that make them suitable for the fixation of osteogenic proteins such as BMP-2; moreover, nanotechnology makes possible the self-assembly of monolayers, by means of affordable processes and without the need to resort to multiple and complex techniques (Kumar et al., 2020). A study by Lan et al. (2020) observed that TiO2 surfaces modified with phosphonic acids had an improved hydrophilic surface that would favor biocompatibility, bone healing and osseointegration. In addition, it has been shown that surface treatment with CEPA increases surface roughness (24) and that the surface texture of an implant strongly influences the biomechanical attachment of Ti to bone (Rabel et al., 2020; Rabel et al., 2021).
The application of BMP-2 on Ti surfaces to stimulate local bone formation has been investigated in ectopic and orthotopic rodent and canine models (Liu et al., 2005; Wikesjö et al., 2008a; Geuze et al., 2012). Hall et al. (2007) observed significant bone formation around porous TiO2 discs coated with BMP-2 at 2 weeks after implantation, proportional to surface porosity, due to increased retention of the protein on the implant surface. Similarly, Liu et al. (2007a) observed that the osteoconductivity of the implant was severely compromised when the protein was directly adsorbed by the untreated surfaces and it has been shown that osteogenic molecules are better adsorbed by the treated surfaces, due to the slow release as the inorganic layer undergoes a degradation process (Liu et al., 2005). Coinciding with these results, our investigation found a significant difference in the BIC in experimental implants treated with BMP-2, compared to the implants of the control group with SLA surface. Liu et al. (2007b) based the osteoinductive efficacy of BMP-2 coatings on the different modes of protein release, highlighting the suitability of surfaces that mimic the mineralized bone matrix and demonstrating that the efficacy of BMP-2 would be directly related to its different initial release, depending on surface roughness. On the other hand, our study found no differences in peri-implant bone density in the experimental implants with biofunctionalized surfaces with respect to the control group, and in this aspect, (Decker et al., 2010) observed that high concentrations of BMP-2 on porous TiO2 implants, after a healing period of 8 weeks, gave rise to immature bone formations and decreased bone density, while low concentrations generated better bone maturation. However, there are discrepancies among researchers; studies in non-human primates, on the contrary, observed enlarged peri-implant bone remodeling zones with increasing concentrations of BMP-2 (Wikesjö et al., 2008b); moreover, we have not found unanimity in this regard on the ideal amounts of BMP-2 for implant biofunctionalization.
Nevertheless, we believe that the differences in bone density and new bone formation, could be attributed to the release kinetics of the implant surfaces and other unknown reasons.
In our research we observed that a high percentage of the samples analyzed (78%) showed ectopic bone formations in the medullary space, and in this regard, (Liu et al., 2007a) highlighted that BMP-2 has a higher osteogenic efficacy when incorporated into a carrier that mimics the mineralized bone matrix, such as the dual-etch surfaces in our study, and that it would decrease its osteogenic efficacy when incorporated on polished Ti surfaces.
The results of our study, although partially, rejected the H0, which assumed that there were no differences in histomorphometric parameters related to osseointegration between dental implants with conventional surfaces and those treated with CEPA and BMP-2, however, we found a series of limitations that we wish to highlight: on the one hand, the small sample size; on the other hand, our experimental model, although considered a predictive model and validated by numerous studies for the investigation of bone regeneration, has the disadvantage of being an orthotopic model with the disadvantages that this entails in terms of bone remodeling. Another question we wish to raise is the quantity and stability of the protein on the textured surface of the Ti and the time required for its effectiveness.
Therefore, we consider that more preclinical studies, appropriately designed and coincident in the methodology, investigating the osteoinductive properties of this protein, in relation to implant osseointegration would be justified and necessary.
5 Conclusion
Within the limitations of this study, our results demonstrated that porous surfaces of dental implants treated with CEPA and BMP-2 enhance the biological response. BMP-2 concentrations could influence bone density and bone formation.
Data availability statement
The original contributions presented in the study are included in the article/Supplementary Material, further inquiries can be directed to the corresponding author.
Ethics statement
The animal study was reviewed and approved by CEEA Code: 017/2013.
Author contributions
NL-V and JA contributed to conception and design of the study. CR organized the database. NL-V performed the statistical analysis. NL-V and JA wrote sections of the manuscript. JMA supervised the manuscript. All authors contributed to the article and approved the submitted version.
Conflict of interest
The authors declare that the research was conducted in the absence of any commercial or financial relationships that could be construed as a potential conflict of interest.
Publisher’s note
All claims expressed in this article are solely those of the authors and do not necessarily represent those of their affiliated organizations, or those of the publisher, the editors and the reviewers. Any product that may be evaluated in this article, or claim that may be made by its manufacturer, is not guaranteed or endorsed by the publisher.
References
Adden, N., Gamble, L. J., Castner, D. G., Hoffmann, A., Gross, G., and Menzel, H. (2006). Phosphonic acid monolayers for binding of bioactive molecules to titanium surfaces. Langmuir 22, 8197–8204. doi:10.1021/la060754c
Aresti, A., Aragoneses, J., López-Valverde, N., Suárez, A., and Aragoneses, J. M. (2021). Effectiveness of biofunctionalization of titanium surfaces with phosphonic acid. Biomedicines 9, 1663. doi:10.3390/biomedicines9111663
Brånemark, P. I., Adell, R., Breine, U., Hansson, B. O., Lindström, J., and Ohlsson, A. (1969). Intra-osseous anchorage of dental prostheses. Scand. J. Plast. Reconstr. Surg. 3, 19–34. doi:10.3109/02844317009038440
Buser, D., Nydegger, T., Oxland, T., Cochran, D. L., Schenk, R. K., Hirt, H. P., et al. (1999). Interface shear strength of titanium implants with a sandblasted and acid-etched surface: A biomechanical study in the maxilla of miniature pigs. J. Biomed. Mater Res. 45, 75–83. doi:10.1002/(sici)1097-4636(199905)45:2<75::aid-jbm1>3.0.co;2-p
Chen, D., Zhao, M., and Mundy, G. R. (2004). Bone morphogenetic proteins. Growth factors. 22, 233–241. doi:10.1080/08977190412331279890
Cho, S. A., and Park, K. T. (2003). The removal torque of titanium screw inserted in rabbit tibia treated by dual acid etching. Biomaterials 24, 3611–3617. doi:10.1016/s0142-9612(03)00218-7
Cochran, D. L., Buser, D., ten Bruggenkate, C. M., Weingart, D., Taylor, T. M., Bernard, J. P., et al. (2002). The use of reduced healing times on ITI® implants with a sandblasted and acid-etched (SLA) surface:: Early results from clinical trials on ITI® SLA implants. Clin. Oral Implants Res. 13, 144–153. doi:10.1034/j.1600-0501.2002.130204.x
Cochran, D. L., Schenk, R. K., Lussi, A., Higginbottom, F. L., and Buser, D. (1998). Bone response to unloaded and loaded titanium implants with a sandblasted and acid-etched surface: A histometric study in the canine mandible. J. Biomed. Mater Res. 40, 1–11. doi:10.1002/(sici)1097-4636(199804)40:1<1::aid-jbm1>3.0.co;2-q
de Groot, K., Wen, H. B., Liu, Y., Layrolle, P., and Barrere, F. (2011). Biomimetic coatings on orthopedic implants: A review. Camb. Univ. Pressp 2011, 109–116. doi:10.1557/PROC-599-109
de Queiroz Fernandes, J., de Lima, V. N., Bonardi, J. P., Filho, O. M., and Queiroz, S. B. F. (2018). Bone regeneration with recombinant human bone morphogenetic protein 2: A systematic review. J. Maxillofac. Oral Surg. 17, 13–18. doi:10.1007/s12663-016-0988-1
Decker, J. F., Lee, J., Cortella, C. A., Polimeni, G., Rohrer, M. D., Wozney, J. M., et al. (2010). Evaluation of implants coated with recombinant human bone morphogenetic protein-2 and vacuum-dried using the critical-size supraalveolar peri-implant defect model in dogs. J. Periodontol. 81, 1839–1849. doi:10.1902/jop.2010.100220
Gawalt, E. S., Avaltroni, M., Koch, N., and Schwartz, J. (2001). Self-assembly and bonding of alkanephosphonic acids on the native oxide surface of titanium. Langmuir 7, 5736–5738. doi:10.1021/la010649x
Geuze, R. E., Theyse, L. F., Kempen, D. H., Hazewinkel, H. A., Kraak, H. Y., Oner, F. C., et al. (2012). A differential effect of bone morphogenetic protein-2 and vascular endothelial growth factor release timing on osteogenesis at ectopic and orthotopic sites in a large-animal model. Tissue Eng. Part A 18, 2052–2062. doi:10.1089/ten.TEA.2011.0560
Giner, L., Mercadé, M., Torrent, S., Punset, M., Pérez, R. A., Delgado, L. M., et al. (2018). Double acid etching treatment of dental implants for enhanced biological properties. J. Appl. Biomater. Funct. Mater 16, 83–89. doi:10.5301/jabfm.5000376
Hall, J., Sorensen, R. G., Wozney, J. M., and Wikesjö, U. M. (2007). Bone formation at rhBMP-2-coated titanium implants in the rat ectopic model. J. Clin. Periodontol. 34, 444–451. doi:10.1111/j.1600-051X.2007.01064.x
Hanawa, T. (2010). Biofunctionalization of titanium for dental implant. Jpn. Dent. Sci. Rev. 46, 93–101. doi:10.1016/j.jdsr.2009.11.001
Hoffmann, A., Weich, H. A., Gross, G., and Hillmann, G. (2001). Perspectives in the biological function, the technical and therapeutic application of bone morphogenetic proteins. Appl. Microbiol. Biotechnol. 57, 294–308. doi:10.1007/s002530100746
Jang, T. S., Jung, H. D., Kim, S., Moon, B. S., Baek, J., Park, C., et al. (2017). Multiscale porous titanium surfaces via a two-step etching process for improved mechanical and biological performance. Biomed. Mater 12, 025008. doi:10.1088/1748-605X/aa5d74
Kolk, A., Boskov, M., Haidari, S., Tischer, T., van Griensven, M., Bissinger, O., et al. (2019). Comparative analysis of bone regeneration behavior using recombinant human BMP-2 versus plasmid DNA of BMP-2. J. Biomed. Mater Res. A 107, 163–173. doi:10.1002/jbm.a.36545
Kuchler, U., Luvizuto, E. R., Muñoz, F., Hofbauer, J., Watzek, G., and Gruber, R. (2013). Bone healing around titanium implants in two rat colitis models. Clin. Oral Implants Res. 24, 224–229. doi:10.1111/j.1600-0501.2012.02454.x
Kumar, S. M., Balakrishnan, P. K., Hedge, C., and Dandekeri, S. (2020). Self-assembled monolayer- A nano surface modification. J. Evol. Med. Dent. Sci. 9, 1608–1612. doi:10.14260/jemds/2020/351
Lan, W. C., Huang, T. S., Cho, Y. C., Huang, Y. T., Walinski, C. J., Chiang, P. C., et al. (2020). The potential of a nanostructured titanium oxide layer with self-assembled monolayers for biomedical applications: Surface properties and biomechanical behaviors. Appl. Sci. 10, 590. doi:10.3390/app10020590
Li, D., Ferguson, S. J., Beutler, T., Cochran, D. L., Sittig, C., Hirt, H. P., et al. (2002). Biomechanical comparison of the sandblasted and acid-etched and the machined and acid-etched titanium surface for dental implants. J. Biomed. Mater Res. 60, 325–332. doi:10.1002/jbm.10063
Liu, Y., de Groot, K., and Hunziker, E. B. (2005). BMP-2 liberated from biomimetic implant coatings induces and sustains direct ossification in an ectopic rat model. Bone 36, 745–757. doi:10.1016/j.bone.2005.02.005
Liu, Y., Enggist, L., Kuffer, A. F., Buser, D., and Hunziker, E. B. (2007b). The influence of BMP-2 and its mode of delivery on the osteoconductivity of implant surfaces during the early phase of osseointegration. Biomaterials 28, 2677–2686. doi:10.1016/j.biomaterials.2007.02.003
Liu, Y., Huse, R. O., de Groot, K., Buser, D., and Hunziker, E. B. (2007a). Delivery mode and efficacy of BMP-2 in association with implants. J. Dent. Res. 86, 84–89. doi:10.1177/154405910708600114
Rabel, K., Kohal, R. J., Steinberg, T., Rolauffs, B., Adolfsson, E., and Altmann, B. (2021). Human osteoblast and fibroblast response to oral implant biomaterials functionalized with non-thermal oxygen plasma. Sci. Rep. 11, 17302. doi:10.1038/s41598-021-96526-x
Rabel, K., Kohal, R. J., Steinberg, T., Tomakidi, P., Rolauffs, B., Adolfsson, E., et al. (2020). Controlling osteoblast morphology and proliferation via surface micro-topographies of implant biomaterials. Sci. Rep. 10, 12810. doi:10.1038/s41598-020-69685-6
Ronga, M., Fagetti, A., Canton, G., Paiusco, E., Surace, M. F., and Cherubino, P. (2013). Clinical applications of growth factors in bone injuries: Experience with BMPs. Injury 44 (1), S34–S39. doi:10.1016/S0020-1383(13)70008-1
Schroeder, A., Pohler, O., and Sutter, F. (1976). Gewebsreaktion auf ein Titan Hohlzylinderimplantat mit Titan-Spritzschichtoberfläche [Tissue reaction to an implant of a titanium hollow cylinder with a titanium surface spray layer]. SSO Schweiz Monatsschr Zahnheilkd 86, 713–727.
Sevrai, C. M., Berchel, M., Couthon, H., and Jaffrès, P. A. (2017). Phosphonic acid: Preparation and applications. Beilstein J. Org. Chem. 13, 2186–2213. doi:10.3762/bjoc.13.219
Stepanovska, J., Matejka, R., Rosina, J., Bacakova, L., and Kolarova, H. (2020). Treatments for enhancing the biocompatibility of titanium implants. Biomed. Pap. Med. Fac. Univ. Palacky. Olomouc Czech Repub. 164, 23–33. doi:10.5507/bp.2019.062
von Walter, M., Herren, C., Gensior, T. J., Steffens, G. C., Hermanns-Sachweh, B., Jahnen-Dechent, W., et al. (2008). Biomimetic modification of the TiO(2)/glass composite Ecopore with heparinized collagen and the osteoinductive factor BMP-2. Acta Biomater. 4, 997–1004. doi:10.1016/j.actbio.2008.01.020
Wikesjö, U. M., Huang, Y. H., Xiropaidis, A. V., Sorensen, R. G., Rohrer, M. D., Prasad, H. S., et al. (2008b). Bone formation at recombinant human bone morphogenetic protein-2-coated titanium implants in the posterior maxilla (Type IV bone) in non-human primates. J. Clin. Periodontol. 35, 992–1000. doi:10.1111/j.1600-051X.2008.01322.x
Wikesjö, U. M., Xiropaidis, A. V., Qahash, M., Lim, W. H., Sorensen, R. G., Rohrer, M. D., et al. (2008a). Bone formation at recombinant human bone morphogenetic protein-2-coated titanium implants in the posterior mandible (Type II bone) in dogs. J. Clin. Periodontol. 35, 985–991. doi:10.1111/j.1600-051X.2008.01318.x
Wilke, H. J., Claes, L., and Steinemann, S. G. (1990). The influence of various titanium surfaces on the interface shear strenght between implants and bone. Amsterdam: Elsevier Science Publishers, 309–314.
Keywords: dental implants, carboxyethylphosphonic acid, BMP-2, osseointegration, minipig model
Citation: López-Valverde N, Aragoneses J, Rodríguez C and Aragoneses JM (2023) Effect on osseointegration of dental implants treated with carboxyethylphosphonic acid and functionalized with BMP-2: preliminary study on a minipig model. Front. Bioeng. Biotechnol. 11:1244667. doi: 10.3389/fbioe.2023.1244667
Received: 22 June 2023; Accepted: 19 July 2023;
Published: 27 July 2023.
Edited by:
Tiago Borges, Catholic University of Portugal, PortugalReviewed by:
Danilo Fernandes, Catholic University of Portugal, PortugalBruno Almeida, Portuguese Catholic University, Portugal
Copyright © 2023 López-Valverde, Aragoneses, Rodríguez and Aragoneses. This is an open-access article distributed under the terms of the Creative Commons Attribution License (CC BY). The use, distribution or reproduction in other forums is permitted, provided the original author(s) and the copyright owner(s) are credited and that the original publication in this journal is cited, in accordance with accepted academic practice. No use, distribution or reproduction is permitted which does not comply with these terms.
*Correspondence: Nansi López-Valverde, nlovalher@usal.es