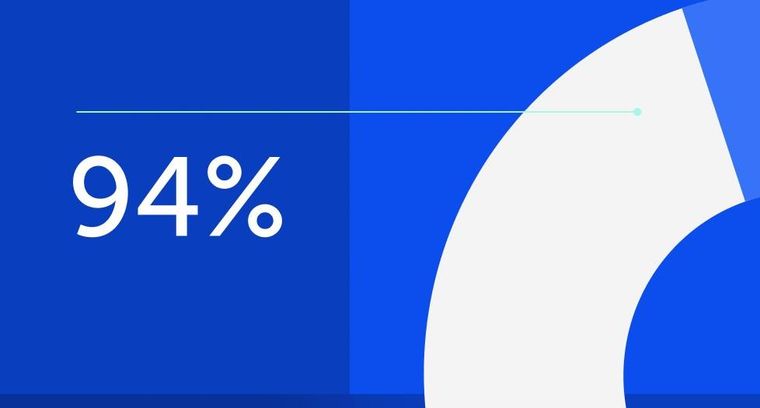
94% of researchers rate our articles as excellent or good
Learn more about the work of our research integrity team to safeguard the quality of each article we publish.
Find out more
OPINION article
Front. Bioeng. Biotechnol., 05 June 2023
Sec. Industrial Biotechnology
Volume 11 - 2023 | https://doi.org/10.3389/fbioe.2023.1210228
This article is part of the Research TopicAlgae Biotechnology as a Promising Solution to Environmental PollutionView all 8 articles
Water is an essential resource for human survival and a pivotal feedstock for industrial production (Song et al., 2022). With the rapid development of industrialization, urbanization, and human population, excessive wastewater (about 380 billion t) would be generated annually across the world (Qadir et al., 2020). Wastewater contains various nutrient elements (e.g., nitrogen, phosphorus, organic compounds, and heavy metals), which can cause water eutrophication if it is released into natural water bodies without treatment (Wan Mahari et al., 2022). Under this situation, a wide range of wastewater treatment plants has been constructed for removing/reducing the nutrients to meet water quality regulations, but the traditional plants have some disadvantages, such as uneven removal efficiency, high installation cost, and diseconomy (Dutta et al., 2021). Especially, large amounts of greenhouse gases, mainly consisting of CO2, methane (CH4), and N2O, will be emitted into the atmosphere during the traditional wastewater treatment process. For example, emissions of greenhouse gases from municipal wastewater treatment plants in Beijing were estimated to be 1 045 611.5 t CO2-eq (tons of CO2-equivalent), with an average emission intensity of 0.603 kg CO2-eq/m3 (Zhou et al., 2022). Thus, greenhouse gases emitted from wastewater treatment plants have become a worldwide issue for the reduction of carbon footprint (Du et al., 2022).
At present, it has been widely recognized that microalgae are a promising renewable resource for wastewater treatment and resource recovery because they not only purify wastewater from different sources, but also use wastewater and CO2 as growth matrix to produce valuable biological products, biomaterials and bioenergy (Song et al., 2022; Srimongkol et al., 2022). Based on previous studies, some key opinions about this topic are summarized and listed as follows. Firstly, microalgae could remove/reduce nutrients, organic pollutants, heavy metals, chemical oxygen demand (COD), and biological oxygen demand (BOD) in wastewater effectively (Song et al., 2022). Secondly, algal biomass cultivated in wastewater could be used as a potential source of precious products that could be utilized in biofuels, cosmetics, pharmaceuticals, and nutraceuticals fields (Li et al., 2021; Lu and Lu, 2022; Lu and Xiao, 2022). Thirdly, the microalgae-driven wastewater treatment could help to realize the integration of wastewater treatment, industrial CO2 sequestration and microalgal biomass valorization, and shift wastewater treatment from linear economy to circular economy (Vaz et al., 2023). However, some limiting factors which may hinder the development of microalgae-driven wastewater treatment systems were neglected by previous studies. Herein, some challenges and potential solutions on the application of microalgae for wastewater treatment and resource recovery were proposed in this paper (Figure 1). It is hoped that the comments and discussions can prevent the overly optimistic attitudes towards the practical application of microalgae in wastewater treatment and resource recovery, and spur investigators to find out practically-feasible solutions to these challenging problems.
FIGURE 1. Challenges and potential solutions of microalgae-based systems for wastewater treatment and resource recovery.
Due to the different production materials and manufacturing technology, wastewater possesses different physicochemical properties. For example, most of nitrogen in silk industry wastewater is in the form of organic compounds, but that in swine manure is in the form of NH4+-N (Deng et al., 2017; Deng et al., 2020). Wastewater collected from different processing units of the silk industry (i.e., cooking cocoon wastewater, reeling wastewater, and frigon wastewater) have different physicochemical characteristics (Deng et al., 2020). Moreover, some toxic compounds, such as pesticides, insecticides, antibiotics, and toxic metals, are present in agricultural wastewater (Wang et al., 2018). Thus, most wastewater need to be pre-treated or diluted before its treatment using microalgae, which accounts a fraction of the treatment cost. The frequently-used pretreatment methods are anaerobic digestion, chemical oxidation, filtration, autoclave, and UV-radiation (Sharmila et al., 2020). In the future, it is urgent to seek for simple and effective pretreatment methods, which could be used to pretreat wastewater in low cost.
Different species of microalgae have different characteristics, such as nutrient removal efficiency, tolerance to toxic compounds, adaptability and cell composition (Lu et al., 2020). For example, López-Sánchez et al. (2022) summarized the maximum biomass concentration, COD removal, total nitrogen removal, and total phosphorus removal from different microalgae species cultivated in primary livestock wastewater as a medium, and pointed out that selection of an algal strain with high performance for wastewater treatment and resource recovery was very important. In future, selection of algal strains with excellent growth characteristics and nutrient removal capacity should be carried out by different methods, such as genetic engineering, adaptive laboratory evolution, and random mutagenesis (Hassanien et al., 2023).
At present, the frequently-used microalgae cultivation facilities have been directly substituted into the field of microalgae-based wastewater treatment. For example, some open systems, such as waste stabilization ponds, raceway reactors, and sophisticated high-rate algal ponds, have been applied in this field because they are relatively inexpensive to build and easy to scale up (Leong et al., 2021). But the open systems have some main disadvantages, such as poor light utilization, evaporative losses, diffusion of CO2 to the atmosphere, contamination by predators, heterotrophs, and the requirement of large areas of land (Cheng et al., 2021). In order to address the above disadvantages, researchers have developed various closed photobioreactors for the treatment of wastewater using microalgae, such as vertical column, tubular, flat plate, membrane, and biofilm-based photobioreactors (Sathinathan et al., 2023). But their installation and maintenance costs are much higher than that of the open ones, suggesting that they are also not suitable facilities for wastewater management. Based on the above discussion, economical, effective, and practical in situ treating facilities have not been designed and constructed for microalgae-based wastewater treatment, indicating that more attentions should be paid to this field in future.
It is well known that microalgae are rich in fatty acids, proteins, carbohydrates, lipids, and various micronutrients (e.g., vitamins, chlorophylls and carotenoids) (Tang et al., 2020). Nowadays, microalgae have been applied in various industrial areas, such as food, feed, pharmaceutical, and cosmetic industries, when they are cultivated in tap water, well water, and other clean water (Okeke et al., 2022). Studies on microalgae as animal feed and human food have been started since the early 1950s (Kusmayadi et al., 2021). In the past decades, microalgal biomass in the forms of capsule, powder, and tablet has merged in the market mainly as health food products (Kusmayadi et al., 2021). In 2018, the Food and Agriculture Organization of the United Nations (FAO) registered 87.0 tons of microalgae cultivation in 11 countries, with 86.6 tons only from China (Ferreira de Oliveira and Bragotto, 2022). However, the algal biomass could not be suitable to produce some typical products (e.g., food, feed, and biofertilizer) when microalgae grow on wastewater due to the exceeding permitted levels of heavy metals, pollutants, and pathogens. As reported previously, cadmium in the microalgal biomass was higher than the threshold established by the European regulation for fertilizing products, and three contaminants of emerging concern (hydrocinnamic acid, caffeine, and bisphenol A) were detected in the microalgae biomass used as biofertilizer (Álvarez-González et al., 2023). Although microalgae could remove pathogenic bacteria effectively from wastewater (Bhatt et al., 2023), Escherichia coli with a number of 400 CFU were found in 1 g algal biomass (Álvarez-González et al., 2023). In the light of above discussion, application scenario of algal biomass obtained from microalgae-based systems for wastewater treatment and resource recovery is restricted. Therefore, more and more application modes (e.g., algal biorefinery) should be investigated in the future.
In recent years, the microalgae-based systems for wastewater treatment and resource recovery have attracted much attention because they could integrate wastewater treatment, industrial CO2 sequestration, and microalgal biomass valorization as a whole system. According to our research experiences and literature review, there are some problems challenging the application of microalgae in wastewater treatment and resource recovery. Firstly, pretreatment of wastewater is complicated when it is treated by using microalgae due to the fact that each type of wastewater has its own characteristics. Secondly, most of algal strains used to treat wastewater have low performances relative to traditional treatment methods. Thirdly, existing treating facilities cannot satisfy the demand for microalgae-based systems for wastewater treatment and resource recovery. Finally, application scenario of algal biomass obtained from the systems is restricted. Thus, there is still a long way to go for wastewater treatment using microalgae due to the above challenges, and the corresponding potential solutions have been recommended in this paper. It is expected that these recommendations could help investigators to find the future trends of this research topic.
LL contributed to data collection and analysis, and writing-original draft preparation; KG contributed to data collection and analysis; MY contributed to writing-original draft preparation; QZ contributed to writing-original draft preparation; MZ contributed to writing-review and editing; XD contributed to writing-review and editing, and manuscript revision. All authors contributed to the article and approved the submitted version.
Jiangsu Provincial Natural Science Foundation of China (BK20221293) Key Research and Development Project of Zhenjiang (SH2022020) Key Laboratory of Ecological Impacts of Hydraulic-projects and Restoration of Aquatic Ecosystem of Ministry of Water Resources (KL-MWR-202105).
The authors declare that the research was conducted in the absence of any commercial or financial relationships that could be construed as a potential conflict of interest.
All claims expressed in this article are solely those of the authors and do not necessarily represent those of their affiliated organizations, or those of the publisher, the editors and the reviewers. Any product that may be evaluated in this article, or claim that may be made by its manufacturer, is not guaranteed or endorsed by the publisher.
Álvarez-González, A., Uggetti, E., Serrano, L., Gorchs, G., Escolà Casas, M., Matamoros, V., et al. (2023). The potential of wastewater grown microalgae for agricultural purposes: Contaminants of emerging concern, heavy metals and pathogens assessment. Environ. Pollut. 324, 121399. doi:10.1016/j.envpol.2023.121399
Bhatt, A., Arora, P., and Prajapati, S. K. (2023). Chlorella pyrenoidosa-mediated removal of pathogenic bacteria from municipal wastewater – multivariate process optimization and application in the real sewage. J. Environ. Chem. Eng. 11, 109494. doi:10.1016/j.jece.2023.109494
Cheng, Y. W., Lim, J. S. M., Chong, C. C., Lam, M. K., Lim, J. W., Tan, I. S., et al. (2021). Unravelling CO2 capture performance of microalgae cultivation and other technologies via comparative carbon balance analysis. J. Environ. Chem. Eng. 9, 106519. doi:10.1016/j.jece.2021.106519
Deng, X., Li, D., Xue, C., Chen, B., Dong, J., Tetteh, P. A., et al. (2020). Cultivation of Chlorella sorokiniana using wastewaters from different processing units of the silk industry for enhancing biomass production and nutrient removal. J. Chem. Techn. Biot. 95, 264–273. doi:10.1002/jctb.6230
Deng, X. Y., Gao, K., Zhang, R. C., Addy, M., Lu, Q., Ren, H. Y., et al. (2017). Growing Chlorella vulgaris on thermophilic anaerobic digestion swine manure for nutrient removal and biomass production. Bioresour. Technol. 243, 417–425. doi:10.1016/j.biortech.2017.06.141
Du, R., Li, C., Liu, Q., Fan, J., and Peng, Y. (2022). A review of enhanced municipal wastewater treatment through energy savings and carbon recovery to reduce discharge and CO2 footprint. Bioresour. Technol. 364, 128135. doi:10.1016/j.biortech.2022.128135
Dutta, D., Arya, S., and Kumar, S. (2021). Industrial wastewater treatment: Current trends, bottlenecks, and best practices. Chemosphere 285, 131245. doi:10.1016/j.chemosphere.2021.131245
Ferreira de Oliveira, A. P., and Bragotto, A. P. A. (2022). Microalgae-based products: Food and public health. Future Foods 6, 100157. doi:10.1016/j.fufo.2022.100157
Hassanien, A., Saadaoui, I., Schipper, K., Al-Marri, S., Dalgamouni, T., Aouida, M., et al. (2023). Genetic engineering to enhance microalgal-based produced water treatment with emphasis on CRISPR/Cas9: A review. Front. Bioeng. Biotechnol. 10, 1104914. doi:10.3389/fbioe.2022.1104914
Kusmayadi, A., Leong, Y. K., Yen, H. W., Huang, C. Y., and Chang, J. S. (2021). Microalgae as sustainable food and feed sources for animals and humans - biotechnological and environmental aspects. Chemosphere 271, 129800. doi:10.1016/j.chemosphere.2021.129800
Leong, Y. K., Huang, C. Y., and Chang, J. S. (2021). Pollution prevention and waste phycoremediation by algal-based wastewater treatment technologies: The applications of high-rate algal ponds (HRAPs) and algal turf scrubber (ATS). J. Environ. Manage. 296, 113193. doi:10.1016/j.jenvman.2021.113193
Li, H., Chen, S., Liao, K., Lu, Q., and Zhou, W. (2021). Microalgae biotechnology as a promising pathway to ecofriendly aquaculture: A state-of-the-art review. J. Chem. Techn. Biot. 96, 837–852. doi:10.1002/jctb.6624
López-Sánchez, A., Silva-Gálvez, A. L., Aguilar-Juárez, Ó., Senés-Guerrero, C., Orozco-Nunnelly, D. A., Carrillo-Nieves, D., et al. (2022). Microalgae-based livestock wastewater treatment (MbWT) as a circular bioeconomy approach: Enhancement of biomass productivity, pollutant removal and high-value compound production. J. Environ. Manage. 308, 114612. doi:10.1016/j.jenvman.2022.114612
Lu, Q., and Lu, Y. (2022). Microalga- and yeast-based astaxanthin production via nutrient recovery from wastewater for aquaculture practice: An emerging technology for sustainable development. J. Chem. Techn. Biot. 97, 3035–3048. doi:10.1002/jctb.7164
Lu, Q., and Xiao, Y. (2022). From manure to high-value fertilizer: The employment of microalgae as a nutrient carrier for sustainable agriculture. Algal Res. 67, 102855. doi:10.1016/j.algal.2022.102855
Lu, W., Asraful Alam, M., Liu, S., Xu, J., and Parra Saldivar, R. (2020). Critical processes and variables in microalgae biomass production coupled with bioremediation of nutrients and CO2 from livestock farms: A review. Sci. Total Environ. 716, 135247. doi:10.1016/j.scitotenv.2019.135247
Okeke, E. S., Ejeromedoghene, O., Okoye, C. O., Ezeorba, T. P. C., Nyaruaba, R., Ikechukwu, C. K., et al. (2022). Microalgae biorefinery: an integrated route for the sustainable production of high-value-added products. Energ. Convers. Manage.: X 16, 100323. doi:10.1016/j.ecmx.2022.100323
Qadir, M., Drechsel, P., Jiménez Cisneros, B., Kim, Y., Pramanik, A., Mehta, P., et al. (2020). Global and regional potential of wastewater as a water, nutrient and energy source. Nat. Resour. Forum. 44, 40–51. doi:10.1111/1477-8947.12187
Sathinathan, P., Parab, H. M., Yusoff, R., Ibrahim, S., Vello, V., and Ngoh, G. C. (2023). Photobioreactor design and parameters essential for algal cultivation using industrial wastewater: A review. Renew. Sust. Energ. Rev. 173, 113096. doi:10.1016/j.rser.2022.113096
Sharmila, V. G., Banu, J. R., Kim, S. H., and Kumar, G. (2020). A review on evaluation of applied pretreatment methods of wastewater towards sustainable H2 generation: Energy efficiency analysis. Int. J. Hydrogen Energ. 45, 8329–8345. doi:10.1016/j.ijhydene.2020.01.081
Song, Y., Wang, L., Qiang, X., Gu, W., Ma, Z., and Wang, G. (2022). The promising way to treat wastewater by microalgae: Approaches, mechanisms, applications and challenges. J. Water Process Eng. 49, 103012. doi:10.1016/j.jwpe.2022.103012
Srimongkol, P., Sangtanoo, P., Songserm, P., Watsuntorn, W., and Karnchanatat, A. (2022). Microalgae-based wastewater treatment for developing economic and environmental sustainability: Current status and future prospects. Front. Bioeng. Biotechnol. 10, 904046. doi:10.3389/fbioe.2022.904046
Tang, D. Y. Y., Khoo, K. S., Chew, K. W., Tao, Y., Ho, S. H., and Show, P. L. (2020). Potential utilization of bioproducts from microalgae for the quality enhancement of natural products. Bioresour. Technol. 304, 122997. doi:10.1016/j.biortech.2020.122997
Vaz, S. A., Badenes, S. M., Pinheiro, H. M., and Martins, R. C. (2023). Recent reports on domestic wastewater treatment using microalgae cultivation: Towards a circular economy. Environ. Technol. Inno. 30, 103107. doi:10.1016/j.eti.2023.103107
Wan Mahari, W. A., Waiho, K., Azwar, E., Fazhan, H., Peng, W., Ishak, S. D., et al. (2022). A state-of-the-art review on producing engineered biochar from shellfish waste and its application in aquaculture wastewater treatment. Chemosphere 288, 132559. doi:10.1016/j.chemosphere.2021.132559
Wang, T., Ai, S., Zhou, Y., Luo, Z., Dai, C., Yang, Y., et al. (2018). Adsorption of agricultural wastewater contaminated with antibiotics, pesticides and toxic metals by functionalized magnetic nanoparticles. J. Environ. Chem. Eng. 6, 6468–6478. doi:10.1016/j.jece.2018.10.014
Keywords: Microalage, wastewater treament, challenges, potential solutions, resource recovery
Citation: Li L, Gao K, Yang M, Zheng Q, Zhang M and Deng X (2023) Challenges and potential solutions of microalgae-based systems for wastewater treatment and resource recovery. Front. Bioeng. Biotechnol. 11:1210228. doi: 10.3389/fbioe.2023.1210228
Received: 22 April 2023; Accepted: 25 May 2023;
Published: 05 June 2023.
Edited by:
Hongli Zheng, Nanchang University, ChinaReviewed by:
Shuhao Huo, Jiangsu University, ChinaCopyright © 2023 Li, Gao, Yang, Zheng, Zhang and Deng. This is an open-access article distributed under the terms of the Creative Commons Attribution License (CC BY). The use, distribution or reproduction in other forums is permitted, provided the original author(s) and the copyright owner(s) are credited and that the original publication in this journal is cited, in accordance with accepted academic practice. No use, distribution or reproduction is permitted which does not comply with these terms.
*Correspondence: Xiangyuan Deng, ZGVuZ3h5MjAwOUAxMjYuY29t
Disclaimer: All claims expressed in this article are solely those of the authors and do not necessarily represent those of their affiliated organizations, or those of the publisher, the editors and the reviewers. Any product that may be evaluated in this article or claim that may be made by its manufacturer is not guaranteed or endorsed by the publisher.
Research integrity at Frontiers
Learn more about the work of our research integrity team to safeguard the quality of each article we publish.