- 1Department of Hepatobiliary and Pancreatic Surgery, Hainan General Hospital (Hainan Affiliated Hospital of Hainan Medical University), Haikou, China
- 2Department of Gastrointestinal Surgery, Central South University Xiangya School of Medicine Affiliated Haikou Hospital, Haikou, China
Ubiquitin is a small protein that can be added onto target protein for inducing target degradation, thereby modulating the activity and stability of protein. Relatively, deubiquitinases (DUBs), a class catalase that can remove ubiquitin from substrate protein, provide a positive regulation of the protein amount at transcription level, post-translational modification, protein interaction, etc. The reversible and dynamic ubiquitination-deubiquitination process plays an essential role in maintaining protein homeostasis, which is critical to almost all the biological processes. Therefore, the metabolic dysregulation of deubiquitinases often lead to serious consequences, including the growth and metastasis of tumors. Accordingly, deubiquitinases can be served as key drug targets for the treatment of tumors. The small molecule inhibitors targeting deubiquitinases has become one of the hot spots of anti-tumor drug research areas. This review concentrated on the function and mechanism of deubiquitinase system in the proliferation, apoptosis, metastasis and autophagy of tumor cells. The research status of small molecule inhibitors of specific deubiquitinases in tumor treatment is introduced, aiming to provide reference for the development of clinical targeted drugs.
1 Introduction
The synthesis and degradation of organics are the essential processes for maintaining the homeostasis of biological metabolism. The Ubiquitin, a small protein (76-nt) extensively distributed within eukaryotes, can bind to substrate proteins through the ubiquitination process, and then induce its degradation via ubiquitin-proteasome system, which represents an important endogenous protein degradation pathway (Suresh et al., 2016). Ubiquitination, a critical proteins’ post-translational modification pathway, is mainly completed via three proteases. First, the ubiquitin-activating enzyme E1 initiates the ubiquitination process by activating ubiquitin in dependence on ATP. Then, the activated ubiquitin is transferred onto the ubiquitin-binding enzyme E2. Subsequently, the target protein is recognized with the cooperation of ubiquitin ligase E3. Therefore, ubiquitin can bind to the target protein’s Lys residue, thereby triggering a series of enzymatic reactions and eventually inducing hydrolysis of target protein by protease (Skaar et al., 2014). The ubiquitination process can be divided into three forms depending on the amount of the ubiquitin involved, namely, mono ubiquitination, multiubiquitination and polyubiquitination. Of them, mono ubiquitination only adds one individual ubiquitin onto the lysine residue of substrate protein, while multiubiquitination can add multiple ubiquitins onto several different lysine residues in one substrate. If multiple ubiquitin monomers link to each other through different Lys residues (Lys6, Lys11, Lys27, Lys29, Lys33, Lys48 and Lys63) or Met1 residues, the target protein can form a polyubiquitination chain (Komander and Rape, 2012).
Ubiquitination is a reversible dynamic process because deubiquitinases (DUBs) can hydrolyze the peptide bond of glycine at position 76 of ubiquitin, thereby removing ubiquitin from the substrate protein (Amerik and Hochstrasser, 2004). Deubiquitinase regulates protein function by mediating the deubiquitination of substrate proteins, and participates in various life activities of cells. During the process of tumor occurrence and development, there are many important tumor suppressor or cancer-promoting proteins associated with tumors that are regulated by deubiquitinase. The DNA damage repair, apoptosis, autophagy, and other cellular processes will be impacted by abnormal expression and mutation of deubiquitinase, which will regulate the growth, invasion, and metastasis of tumor cells. The dynamic balance between ubiquitination and deubiquitination maintains the healthy growth of cells. The ubiquitination and deubiquitination processes are shown in Figure 1.
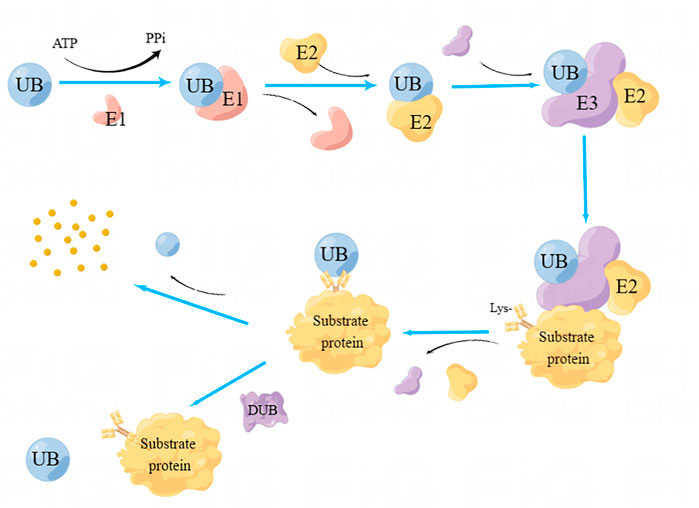
FIGURE 1. Process of Ubiquitination and deubiquitination. Ubiquitin-activating enzyme E1 initiates the ubiquitination process by activating ubiquitin in dependence on ATP. Subsequently, the activated ubiquitin is transferred onto the ubiquitin-binding enzyme E2. Subsequently, the target protein is recognized with the cooperation of ubiquitin ligase E3. As a result, ubiquitin can bind to the target protein’s Lys residue, thereby triggering a series of enzymatic reactions and eventually inducing hydrolysis of target protein by protease. DUBs can hydrolyze the peptide bond of ubiquitin, separate ubiquitin from the substrate protein, and induce deubiquitination.
Up to now, over 4 90 DUBs from seven subfamilies (Clague et al., 2019) have been discovered, including ubiquitin-specific proteases (USP), ubiquitin C-terminal hydrolases (UCH), ovarian tumor proteases (OTU), MachadoJosephin domain proteases (MJD), zinc-finger containing ubiquitin peptidase (ZUP1), Motif Interacting with Ub-containing novel DUB family (MINDY), as well as Jab1/Mov34/MPN + protease (JAMM) family includes zinc-binding metalloproteases (Suresh et al., 2016; Lange et al., 2022). DUBs-mediated deubiquitination has a complicated mechanism. DUBs can not only remove ubiquitin, but also promote the process of ubiquitin by recycling ubiquitination molecules, proofreading of ubiquitination, along with decomposition of ubiquitination inhibitors (Sowa et al., 2009). Deubiquitination is closely related to various cellular biological processes, and plays a vital role in the growth and metastasis of various tumors, including liver cancer, breast cancer (BC), colorectal cancer (CRC) and lung cancer, etc (Lange et al., 2022). At present, DUBs-targeting small-molecule inhibitors are becoming the hotspot anticancer therapeutic agents. Previous reviews on DUBs mainly introduced the molecular mechanism of one specific enzyme (Tao et al., 2022; Oikawa et al., 2023). This review aimed to introduce the effects of DUBs on tumor progression, and the impacts of DUBs on tumor cell growth, apoptosis, metastasis and autophagy, as well as the current research situation and development of DUBs inhibitors.
2 Effect of DUBs on tumor cell proliferation
A variety of DUBs have critical effects on the proliferation of different cancer cells (Liu et al., 2022). Typically, p53 is defined as a tumor suppressor. It can induce cell cycle arrest, apoptosis and senescence under the pressure of gene damage and carcinogenesis in order to maintain the integrity of the genome (Kastenhuber and Lowe, 2017; Uehara and Tanaka, 2018). In addition, it also can induce the proliferation, invasion, metastasis and immune escape of invasive tumor cell (Alexandrova et al., 2015; Bates, 2016; Cortez et al., 2016). USP7 can keep p53 expression level through deubiquitination (Tavana and Gu, 2017), thereby inhibiting the proliferation of tumor cells (Becker et al., 2008). In liver cancer cells, USP7 can be recruited by scaffold protein Abraxas brother 1 (ABRO1) to induce p53 deubiquitination. It has been confirmed to have the ability to inhibit the proliferation and colony formation of tumor cells in vitro (Zhang et al., 2014). USP7 also can deubiquitinate Mdm2, a p53 chaperone molecule and inhibitor, thus inhibiting its degradation (Cummins et al., 2004; Xia et al., 2021). In contrast, miR-205 that can inhibit the expression of USP7 can downregulate p53 and its downstream target protein, thereby promoting cell proliferation (Zhu et al., 2015).
USP21 can catalyze the deubiquitination of MEK2, thereby activating the ERK pathway, inducing cell proliferation and promoting tumor growth (Li et al., 2018). It also can deubiquitinize forkhead box M1 (FOXM1, a transcriptional factor), which plays an important role in the mitotic cycle of cells (Laoukili et al., 2005). In BC cells, loss of USP21 can arrest cell cycle and suppress cell growth (Peng et al., 2016), which are possibly through the regulation of FOXM1 (Arceci et al., 2019; Li et al., 2022).
Androgen receptor (AR) can suppress tumor cell proliferation through suppressing ERα signaling of ER-positive BC, thereby promoting ER-negative BC cell growth (Peters et al., 2009; Cochrane et al., 2014; Mina et al., 2017; Hou et al., 2021). USP14 can inhibit AR degradation by deubiquitination. Therefore, USP14 inhibitors suppress cell proliferation by causing the arrest of the cell cycle of AR-positive BC cells (Liao et al., 2018). Moreover, USP14 can activate phosphatidylinositol-3 kinase (PI3K) to induce cell growth via Wnt/β-catenin pathway (Huang et al., 2015; Zhang et al., 2020a), which also can contribute to the occurrence of drug resistance in CRC cells using USP22 (Miao et al., 2022). USP22 can deubiquitinize CCND1 protein and increase its expression level, thereby promoting the proliferation of CRC cells (Gennaro et al., 2018). In addition, it has also been shown that PSMD14 can promote cell growth in vitro and tumor development in vivo (Lv et al., 2020).
The expression of USP14, USP21 and PSMD14 significantly increases within tumor tissue from liver cancer patients, which is negatively correlated with survival rate (Huang et al., 2015; Li et al., 2018; Lv et al., 2020). In prostate cancer, USP7, USP14, and USP22 can promote cell proliferation via AR, while USP16 inhibits cell proliferation and colony formation (Schrecengost et al., 2014; Liao et al., 2017; Ge et al., 2021). In addition, OTUD6A is reported to upregulate dynamin-related protein 1 (DRP1) by deubiquitination, thus promoting CRC cell proliferation (Shi et al., 2020). USP43 can enhance CRC cell growth by inducing the deubiquitination of Zinc finger E-box-binding homeobox 1 (ZEB1) protein, which is closely associated with tumor cell proliferation, invasion, migration and expression of EMT-related biomarkers. (Ye et al., 2021). In pancreatic cancer and non-small cell lung cancer (NSCLC), various DUBs have also been found to be highly expressed, including OTUD3, USP5, USP17, USP21, USP26, and USP28, which usually predicts dismal prognostic outcome (Lu et al., 2018; Wang et al., 2018; Du et al., 2019; Hou et al., 2019; Hu et al., 2019; Li et al., 2020a; Zhang et al., 2020b). The mechanism of DUB promoting tumor cell proliferation is shown in Figure 2.
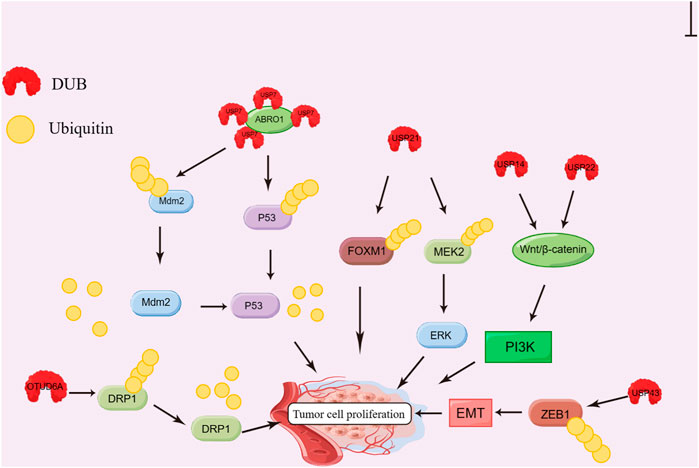
FIGURE 2. Effect of DUBs on tumor cell proliferation. ABRO1 recruits USP7 and induces deubiquitination of Mdm2 and P53; USP21 induces FOXM1 and MEK2 deubiquitination; USP14 and USP22 can activate PI3K via the Wnt/β-catenin pathway. USP43 inhibits the degradation of ZEB1 protein by deubiquitination; OTUD6A can stabilize DRP1 by deubiquitination; All the processes promote the proliferation of tumor cells.
3 Effect of DUBs on tumor cell apoptosis
MYC makes a vital effect on tumor cell growth and apoptosis. It can be classified into three types, c-MYC, N-MYC and L-MYC. Ubiquitination is a predominant pathway for MYC degradation (Chen et al., 2018a; Yoshida, 2018). Both USP28 and USP36 can bind to c-MYC in cells, and deubiquitinize c-MYC, thereby modulating cancer cell growth and apoptosis (Popov et al., 2007; Sun et al., 2015). USP13 can antagonize ubiquitin ligase FBXL14 mediated ubiquitination to stabilize c-MYC, finally maintaining tumorigenicity and self-renewal of germline stem cells (Fang et al., 2017). Moreover, USP13 can deubiquitinize myeloid-cell leukemia 1 (MCL1, an anti-apoptotic protein). Therefore, if USP13 inhibition reduces the protein level of MCL1, it significantly elevates cancer cell apoptosis. Pannem et al. discovered CYLD expression was negatively related to the expression levels of proliferation markers Ki-67 and c-MYC, as evidenced by tissue microarray and immunohistochemistry (IHC) analysis of human liver cancer samples. On the contrary, CYLD silencing can induce tumor cell growth (Pannem et al., 2014).
For AR-positive BC cells, USP14 can deubiquitinize the anti-apoptosis effect of AR, and inhibiting USP14 can induce the cleavage of polyadenosine diphosphate ribose polymerase 1 and reduce BCL2 protein level, thereby promoting apoptosis (Liao et al., 2018). However, PSMD14 shows the opposite effect (Luo et al., 2017). In CRC cells, various DUBs are tightly associated with apoptotic proteins. For instance, USP22 can regulate cell apoptosis by regulating mTOR (Kosinsky et al., 2020), while USP1 can inhibit the degradation of myeloid-cell leukemia 1 (MCL1) and B-cell lymphoma 2 (BCL2), both of which are known as anti-apoptotic proteins (Xu et al., 2019). Other studies have found mutations of USP17 or OTUD1 gene within pancreatic cancer cells. This leads to expression of yes-associated protein 1 (YAP) and the decrease of nuclear factor erythroid 2-related factor 2 (NRF2), eventually regulating cell apoptosis (Chen et al., 2020; Grattarola et al., 2021). DUBs related to DNA damage repair have been reported to be associated with apoptosis in NSCLC tissues. For example, USP35 can reduce the endoplasmic reticulum stress-induced apoptosis by stabilizing ribosomal binding protein 1 (RRBP1) (Garcia-Santisteban et al., 2013; Wang et al., 2022). UCHL1 and WSP9X can regulate cell apoptosis by inhibiting p53 degradation in HCC tissues (Yu et al., 2008; Liu et al., 2015).
4 Effect of DUBs on tumor cell metastasis
Metastasis, a typical characteristic of cancer that causes high mortality, can also be affected by DUBs. For example, OTUB1 can promote the progression, metastasis and invasion of gastric cancer (GC), prostate cancer and colon cancer (Zhou et al., 2014; Iglesias-Gato et al., 2015; Weng et al., 2016; Zhou et al., 2018). Relatively, OTUD1 also can inhibit tumor metastasis by preventing the degradation of SMAD family member 7 (SMDA7), which can inhibit tumor metastasis by blocking TGF-β signaling pathway (Zhang et al., 2017). In addition, OTUB3 exhibits different biological functions in various tumors. In BC tissues, OTUD3 knockout can activate Akt pathway, inducing cell transformation and tumor metastasis (Yuan et al., 2015). However, in lung cancer tissues, its expression is upregulated, which predicts dismal prognostic outcome (Du et al., 2019; Zhang et al., 2020a). The underlying mechanism is that OTUD3 induces the deubiquitination of glucose-regulated protein 78 (GRP78), and subsequently promotes tumor proliferation and metastasis (Qian et al., 2017; Shen et al., 2017).
Many ubiquitin-specific protease family members are also connected with tumor metastasis. USP4 is a vital cell pathway regulator, which is involved in p53 regulation, TGF-β response and NF-κB signal transduction, and makes a critical effect on cancer genesis and progression (Clerici et al., 2014; Qiu et al., 2018; Zhang et al., 2020b). In CRC tissues, USP4 has been found to stabilize PRL-3 by deubiquitinating PRL-3, activating PI3K/AKT pathway and down-regulating E-cadherin, thereby promoting tumor proliferation and metastasis (Xing et al., 2016). In lung cancer tissues, USP4 can stabilize Twist1 to promote tumor cell stemness (Li et al., 2020b). In addition, USP9X can regulate EMT, while USP37 can stabilize c-MYC by the deubiquitination of USP21 and YY1, aiming to promote the metastasis of lung cancer cells (Pan et al., 2015; Cai et al., 2019; Xu et al., 2020). In conclusion, DUBs are closely related to the metastasis of a variety of tumors, and the treatment of targeted DUBs is expected to inhibit tumor metastasis.
5 Effect of DUBs on tumor autophagy
In eukaryotes, autophagy is a highly conserved lysosomal degradation pathway that recognizes and degrades dysfunctional organelles, macromolecular complexes, as well as intracellular bacteria and other foreign bodies. Moreover, it generates energy recycling, and makes a critical effect on maintaining homeostasis of cells, tissues, and organisms (Li et al., 2020c). Autophagy has been found to be related to many disorders including microbial infection, Alzheimer’s disease (AD), cancer progression and drug resistance (Qiu et al., 2022). Nevertheless, the relation between autophagy and tumors is extremely complicated and controversial. Autophagy can protect tumor cells through recycling degraded products and removing injured organelles. However, excess autophagy may paradoxically cause “type II programmed cell death” or “autophagic cell death” in cancer cells (Chen et al., 2018b; Qiu et al., 2022). Autophagy resulted from drug treatment and metabolism has shown a dual-effect on cancer resistance. In a word, it not only causes apoptosis but also promotes cell survival (Yu et al., 2019). Therefore, how to use autophagy appropriately in cancer drug therapy is of particular importance.
Ubiquitination, as an important post-translational modification, participates in several autophagy stages (Grumati and Dikic, 2018). Accordingly, DUBs also have key regulatory effects on autophagy by targeting the ubiquitinated autophagic regulatory components or autophagic substrates (Magraoui et al., 2015).
Mammalian target of rapamycin complex 1 (mTORC1) and ULK1/2 kinase complex are key complexes that induce autophagy. DEPTOR is a mTORC1 inhibitor that can regulate autophagy (Zhao et al., 2011). OTUB1 can directly bind to DEPTOR through the N-terminal domain, which can thereby enable the deubiquitination of DEPTOR, increasing the DEPTOR expression level and inhibiting the activity of mTORC1 (Zhao et al., 2018). USP1, USP20 and USP24 can interact with ULK1 to catalyze its deubiquitination and protect it against degradation, eventually inducing autophagy (Nazio et al., 2016; Kim et al., 2018; Raimondi et al., 2019; Thayer et al., 2020). USP30 and USP33, which are localized in the mitochondrial outer membrane, can interact with the E3 ubiquitin ligase Parkin, thereby negatively regulating mitophagy (Bingol et al., 2014; Michel et al., 2017; Niu et al., 2020; Zhang et al., 2022a). In addition, USP15 and USP35 have also been found to play a negative regulation role in mitophagy (Cornelissen et al., 2014; Wang et al., 2015). By contrast, USP8 can positively regulate mitophagy through specifically eliminating K6 ubiquitin chain on Parkin. Silencing USP8 can prevent the recruitment of Parkin into the depolarized mitochondria, thereby inhibiting Parkin-mediated mitophagy (Durcan et al., 2014; Durcan and Fon, 2015). The targeted proteins and regulatory mechanisms of DUBs are presented in Table 1.
6 Small-molecule inhibitors of DUBs
Due to the extensive involvement of DUBs in tumour proliferation, apoptosis and metastasis, numerous small molecule inhibitors of DUBs have been identified as tumor drugs. At present, most inhibitors of DUBs are still in the research stage. VLX1570, a USP14/UCHL5 dual inhibitor, was the first DUB inhibitor investigated clinically in 2015. However, its investigation was terminated in the clinical trial stage due to its dose-limiting toxic effect (Wang et al., 2016; Schauer et al., 2020). Most of these DUBs inhibitors for cancer therapy are targeting USP family members, and non-USP family inhibitors need to be further investigated. P22077 can selectively downregulate USP7, USP10 and USP47, thereby effectively inhibiting the cell proliferation of neuroblastoma and T-cell acute lymphoblastic leukemia (ALL), and inducing apoptosis (Fan et al., 2013; Shan et al., 2018; Gu et al., 2022). In addition, it can suppress the metastasis of melanoma cells (Vishnoi et al., 2018). B-AP15 contributes to the selective inhibition of USP4 and UCHL5, and restrains the cell proliferation of various cancers, including lung cancer, CRC, BC, myeloma, prostate cancer, and liver cancer (D'Arcy et al., 2011; Tian et al., 2014; Cai et al., 2017; Ding et al., 2018). As the s, P5091 is also an elective dual-inhibitor of USP7 and USP47 that can suppress CRC cell growth and induces their apoptosis in vitro (An et al., 2017). In addition, it can hinder the proliferation of ovarian cancer cells and promote the apoptosis. The effect is more effective on ovarian cancer than wild-type p53 (Wang et al., 2017a; An et al., 2017).
Focusing on autophagy that makes a critical effect on tumor development, researchers have successively identified and screened various specific small-molecule inhibitors of autophagy-related DUBs, including USP1-UAF1 complex inhibitors GW7647 and Pimozide, which can successfully reverse NSCLC cell resistance to chemotherapeutic drugs (Chen et al., 2011). Small-molecule inhibitor Spautin-1 can increase the ubiquitination level of Beclin1 subunit in VPS34-PI3K kinase complex and promote its degradation by suppressing the activities of USP10 and USP13, thus preventing the process of cell autophagy. The combination of DUBs inhibitors and anticancer drugs can enhance the sensitivity of tumor cells to drugs (Shao et al., 2014; Schott et al., 2018; Yeo et al., 2018; Dong et al., 2019). The treatment of H1299 lung cancer cells with LDN-57444, a small-molecule inhibitor targeting UCH-L1, significantly decreased the proliferation rate of tumor cells (Liu et al., 2003). WP1130 can partially selectively inhibit DUBs, which can directly inhibit deubiquitin activities of USP5, USP9X, USP14, UCH37, and UCH-L1, resist the proliferation and promote the apoptosis of various tumor cells (Wang et al., 2017b; Kim et al., 2019). Deubiquitinase inhibitors were shown in Table 2.
7 Conclusion
Over the past decades, the structure, function, role, associated mechanism, and the relationship with diseases of DUBs have been investigated. It has been known that DUBs are closely associated with cancer genesis and progression, which have become the new hotspots in tumor treatment. DUBs are extensively involved in various processes of tumor development. They can regulate the levels of proteins, including the “undrugable” targets that are not sensitive to traditional targeted therapies. Therefore, DUBs have a broad prospect as the tumor therapeutic targets. However, most of the DUBs have poor specificity. One DUB can regulate multiple substrates, while one substrate also can be regulated by multiple DUBs. In addition, there are dynamic changes between ubiquitin ligases and DUBs. Therefore, in various types of tumors, DUBs show a dual role of promotion and suppression. The original methods and techniques for screening inhibitors are also associated with some shortcomings such as poor biocompatibility and high off-target rate (Harrigan et al., 2018), causing difficulties in the clinical translation of DUBs inhibitors. With significant improvements in biochemical analysis and screening techniques, an increasing number of small-molecule DUBs inhibitors have been discovered. Transforming small-molecule inhibitors into specific probes to explore the downstream regulatory mechanisms of DUBs can provide a certain foundation for the clinical evaluation of drug-like molecules, and also provide various tools for further analysis of related regulatory processes, biochemical mechanisms, and drug efficacy evaluation in disease models. We believe that DUBs and their inhibitors have a bright future in the field of cancer therapy, and more exciting results will emerge in the future, promoting them to eventually become the reliable clinical targets for cancer therapy.
Author contributions
X-WL, S-ZW, and BL: paper writing and data collection; J-CC, ZC, F-RC, and XL: data collection; HL and J-CW: supervision and paper revision. All authors contributed to the article and approved the submitted version.
Funding
This work is supported by Natural Science Foundation of Hainan Province (Grant number: 823MS167), and Hainan Province Science and Technology Special Fund (Grant number: ZDYF2022SHFZ283).
Acknowledgments
Our thanks should go to The team from School of Biomedical Engineering of Hainan University.
Conflict of interest
The authors declare that the research was conducted in the absence of any commercial or financial relationships that could be construed as a potential conflict of interest.
Publisher’s note
All claims expressed in this article are solely those of the authors and do not necessarily represent those of their affiliated organizations, or those of the publisher, the editors and the reviewers. Any product that may be evaluated in this article, or claim that may be made by its manufacturer, is not guaranteed or endorsed by the publisher.
References
Alexandrova, E. M., Yallowitz, A. R., Li, D., Xu, S., Schulz, R., Proia, D. A., et al. (2015). Improving survival by exploiting tumour dependence on stabilized mutant p53 for treatment. Nature 523 (7560), 352–356. doi:10.1038/nature14430
Amerik, A. Y., and Hochstrasser, M. (2004). Mechanism and function of deubiquitinating enzymes. Biochim. Biophys. Acta 1695 (1-3), 189–207. doi:10.1016/j.bbamcr.2004.10.003
An, T., Gong, Y., Li, X., Kong, L., Ma, P., Gong, L., et al. (2017). USP7 inhibitor P5091 inhibits Wnt signaling and colorectal tumor growth. Biochem. Pharmacol. 131, 29–39. doi:10.1016/j.bcp.2017.02.011
Arceci, A., Bonacci, T., Wang, X., Stewart, K., Damrauer, J. S., Hoadley, K. A., et al. (2019). FOXM1 deubiquitination by USP21 regulates cell cycle progression and paclitaxel sensitivity in basal-like breast cancer. Cell Rep. 26 (11), 3076–3086.e6. doi:10.1016/j.celrep.2019.02.054
Bates, S. E. (2016). Endocrine cancers: Defying the paradigms. Clin. Cancer Res. 22 (20), 4980. doi:10.1158/1078-0432.ccr-16-0366
Becker, K., Marchenko, N. D., Palacios, G., and Moll, U. M. (2008). A role of HAUSP in tumor suppression in a human colon carcinoma xenograft model. Cell Cycle 7 (9), 1205–1213. doi:10.4161/cc.7.9.5756
Bingol, B., Tea, J. S., Phu, L., Reichelt, M., Bakalarski, C. E., Song, Q., et al. (2014). The mitochondrial deubiquitinase USP30 opposes parkin-mediated mitophagy. Nature 510 (7505), 370–375. doi:10.1038/nature13418
Cai, J., Li, M., Wang, X., Li, L., Li, Q., Hou, Z., et al. (2019). USP37 promotes lung cancer cell migration by stabilizing snail protein via deubiquitination. Front. Genet. 10, 1324. doi:10.3389/fgene.2019.01324
Cai, J., Xia, X., Liao, Y., Liu, N., Guo, Z., Chen, J., et al. (2017). A novel deubiquitinase inhibitor b-AP15 triggers apoptosis in both androgen receptor-dependent and -independent prostate cancers. Oncotarget 8 (38), 63232–63246. doi:10.18632/oncotarget.18774
Chen, H., Liu, H., and Qing, G. (2018). Targeting oncogenic Myc as a strategy for cancer treatment. Signal Transduct. Target Ther. 3, 5. doi:10.1038/s41392-018-0008-7
Chen, H., Zhu, X., Sun, R., Ma, P., Zhang, E., Wang, Z., et al. (2020). Ubiquitin-specific protease 7 is a druggable target that is essential for pancreatic cancer growth and chemoresistance. Invest. New Drugs 38 (6), 1707–1716. doi:10.1007/s10637-020-00951-0
Chen, J., Dexheimer, T. S., Ai, Y., Liang, Q., Villamil, M., Inglese, J., et al. (2011). Selective and cell-active inhibitors of the USP1/UAF1 deubiquitinase complex reverse cisplatin resistance in non-small cell lung cancer cells. Chem. Biol. 18 (11), 1390–1400. doi:10.1016/j.chembiol.2011.08.014
Chen, K., Dai, H., Yuan, J., Chen, J., Lin, L., Zhang, W., et al. (2018). Optineurin-mediated mitophagy protects renal tubular epithelial cells against accelerated senescence in diabetic nephropathy. Cell Death Dis. 9 (2), 105. doi:10.1038/s41419-017-0127-z
Clague, M. J., Urbe, S., and Komander, D. (2019). Breaking the chains: Deubiquitylating enzyme specificity begets function. Nat. Rev. Mol. Cell Biol. 20 (6), 338–352. doi:10.1038/s41580-019-0099-1
Clerici, M., Luna-Vargas, M. P., Faesen, A. C., and Sixma, T. K. (2014). The DUSP–Ubl domain of USP4 enhances its catalytic efficiency by promoting ubiquitin exchange. Nat. Commun. 5, 5399. doi:10.1038/ncomms6399
Cochrane, D. R., Bernales, S., Jacobsen, B. M., Cittelly, D. M., Howe, E. N., D’Amato, N. C., et al. (2014). Role of the androgen receptor in breast cancer and preclinical analysis of enzalutamide. Breast Cancer Res. 16 (1), R7. doi:10.1186/bcr3599
Cornelissen, T., Haddad, D., Wauters, F., Van Humbeeck, C., Mandemakers, W., Koentjoro, B., et al. (2014). The deubiquitinase USP15 antagonizes Parkin-mediated mitochondrial ubiquitination and mitophagy. Hum. Mol. Genet. 23 (19), 5227–5242. doi:10.1093/hmg/ddu244
Cortez, M. A., Ivan, C., Valdecanas, D., Wang, X., Peltier, H. J., Ye, Y., et al. (2016). PDL1 Regulation by p53 via miR-34. J. Natl. Cancer Inst. 108 (1), djv303. doi:10.1093/jnci/djv303
Cummins, J. M., Rago, C., Kohli, M., Kinzler, K. W., Lengauer, C., and Vogelstein, B. (2004). Tumour suppression: Disruption of HAUSP gene stabilizes p53[J]. Nature 428 (6982), 1–2. doi:10.1038/nature02501
D'Arcy, P., Brnjic, S., Olofsson, M. H., Fryknäs, M., Lindsten, K., De Cesare, M., et al. (2011). Inhibition of proteasome deubiquitinating activity as a new cancer therapy. Nat. Med. 17 (12), 1636–1640. doi:10.1038/nm.2536
Ding, Y., Chen, X., Wang, B., Yu, B., and Ge, J. (2018). Deubiquitinase inhibitor b-AP15 activates endoplasmic reticulum (ER) stress and inhibits Wnt/Notch1 signaling pathway leading to the reduction of cell survival in hepatocellular carcinoma cells. Eur. J. Pharmacol. 825, 10–18. doi:10.1016/j.ejphar.2018.02.020
Dong, K., Chen, X., Xie, L., Yu, L., Shen, M., Wang, Y., et al. (2019). Spautin-A41 attenuates cerulein-induced acute pancreatitis through inhibition of dysregulated autophagy. Biol. Pharm. Bull. 42 (11), 1789–1798. doi:10.1248/bpb.b19-00132
Du, T., Li, H., Fan, Y., Yuan, L., Guo, X., Zhu, Q., et al. (2019). The deubiquitylase OTUD3 stabilizes GRP78 and promotes lung tumorigenesis. Nat. Commun. 10 (1), 2914. doi:10.1038/s41467-019-10824-7
Durcan, T. M., and Fon, E. A. (2015). USP8 and PARK2/parkin-mediated mitophagy. Autophagy 11 (2), 428–429. doi:10.1080/15548627.2015.1009794
Durcan, T. M., Tang, M. Y., Perusse, J. R., Dashti, E. A., Aguileta, M. A., McLelland, G., et al. (2014). USP 8 regulates mitophagy by removing K 6-linked ubiquitin conjugates from parkin. EMBO J. 33 (21), 2473–2491. doi:10.15252/embj.201489729
Fan, Y. H., Cheng, J., Vasudevan, S. A., Dou, J., Zhang, H., Patel, R. H., et al. (2013). USP7 inhibitor P22077 inhibits neuroblastoma growth via inducing p53-mediated apoptosis. Cell Death Dis. 4 (10), e867. doi:10.1038/cddis.2013.400
Fang, X., Zhou, W., Wu, Q., Huang, Z., Shi, Y., Yang, K., et al. (2017). Deubiquitinase USP13 maintains glioblastoma stem cells by antagonizing FBXL14-mediated Myc ubiquitination. J. Exp. Med. 214 (1), 245–267. doi:10.1084/jem.20151673
Garcia-Santisteban, I., Peters, G. J., Giovannetti, E., and Rodríguez, J. A. (2013). USP1 deubiquitinase: Cellular functions, regulatory mechanisms and emerging potential as target in cancer therapy. Mol. Cancer 12, 91. doi:10.1186/1476-4598-12-91
Ge, J., Yu, W., Li, J., Ma, H., Wang, P., Zhou, Y., et al. (2021). USP16 regulates castration-resistant prostate cancer cell proliferation by deubiquitinating and stablizing c-Myc. J. Exp. Clin. Cancer Res. 40 (1), 59. doi:10.1186/s13046-021-01843-8
Gennaro, V. J., Stanek, T. J., Peck, A. R., Sun, Y., Wang, F., Qie, S., et al. (2018). Control of CCND1 ubiquitylation by the catalytic SAGA subunit USP22 is essential for cell cycle progression through G1 in cancer cells. Proc. Natl. Acad. Sci. U. S. A. 115 (40), E9298–E9307. doi:10.1073/pnas.1807704115
Grattarola, M., Cucci, M. A., Roetto, A., Dianzani, C., Barrera, G., and Pizzimenti, S. (2021). Post-translational down-regulation of Nrf2 and YAP proteins, by targeting deubiquitinases, reduces growth and chemoresistance in pancreatic cancer cells. Free Radic. Biol. Med. 174, 202–210. doi:10.1016/j.freeradbiomed.2021.08.006
Grumati, P., and Dikic, I. (2018). Ubiquitin signaling and autophagy. J. Biol. Chem. 293 (15), 5404–5413. doi:10.1074/jbc.tm117.000117
Gu, H., Shi, X., Liu, C., Wang, C., Sui, N., Zhao, Y., et al. (2019). USP8 maintains embryonic stem cell stemness via deubiquitination of EPG5. Nat. Commun. 10 (1), 1465. doi:10.1038/s41467-019-09430-4
Gu, Y. H., Ren, K. W., Wang, Y., Wang, S. H., Yu, X. H., Xu, L. W., et al. (2022). Administration of USP7 inhibitor P22077 inhibited cardiac hypertrophy and remodeling in Ang II-induced hypertensive mice. Front. Pharmacol. 13, 1021361. doi:10.3389/fphar.2022.1021361
Harrigan, J. A., Jacq, X., Martin, N. M., and Jackson, S. P. (2018). Deubiquitylating enzymes and drug discovery: Emerging opportunities. Nat. Rev. Drug Discov. 17 (1), 57–78. doi:10.1038/nrd.2017.152
Hou, J., Ye, X., Wang, Y., and Li, C. (2021). Stratification of estrogen receptor-negative breast cancer patients by integrating the somatic mutations and transcriptomic data. Front. Genet. 12, 610087. doi:10.3389/fgene.2021.610087
Hou, P., Ma, X., Zhang, Q., Wu, C. J., Liao, W., Li, J., et al. (2019). USP21 deubiquitinase promotes pancreas cancer cell stemness via Wnt pathway activation. Genes Dev. 33 (19-20), 1361–1366. doi:10.1101/gad.326314.119
Hu, B., Deng, T., Ma, H., Liu, Y., Feng, P., Wei, D., et al. (2019). Deubiquitinase DUB3 regulates cell cycle progression via stabilizing cyclin A for proliferation of non-small cell lung cancer cells. Cells 8 (4), 297. doi:10.3390/cells8040297
Huang, G., Li, L., and Zhou, W. (2015). USP14 activation promotes tumor progression in hepatocellular carcinoma. Oncol. Rep. 34 (6), 2917–2924. doi:10.3892/or.2015.4296
Iglesias-Gato, D., Chuan, Y. C., Jiang, N., Svensson, C., Bao, J., Paul, I., et al. (2015). OTUB1 de-ubiquitinating enzyme promotes prostate cancer cell invasion in vitro and tumorigenesis in vivo[J]. Mol. Cancer 14 (1), 8. doi:10.1186/s12943-014-0280-2
Jin, S., Tian, S., Chen, Y., Zhang, C., Xie, W., Xia, X., et al. (2016). USP19 modulates autophagy and antiviral immune responses by deubiquitinating Beclin-1. EMBO J. 35 (8), 866–880. doi:10.15252/embj.201593596
Kastenhuber, E. R., and Lowe, S. W. (2017). Putting p53 in context. Cell 170 (6), 1062–1078. doi:10.1016/j.cell.2017.08.028
Kim, J. H., Seo, D., Kim, S. J., Choi, D. W., Park, J. S., Ha, J., et al. (2018). The deubiquitinating enzyme USP20 stabilizes ULK1 and promotes autophagy initiation. EMBO Rep. 19 (4), e44378. doi:10.15252/embr.201744378
Kim, S., Woo, S. M., Min, K. J., Seo, S., Lee, T. J., Kubatka, P., et al. (2019). WP1130 enhances TRAIL-induced apoptosis through USP9X-dependent miR-708-mediated downregulation of c-FLIP. Cancers (Basel) 11 (3), 344. doi:10.3390/cancers11030344
Komander, D., and Rape, M. (2012). The ubiquitin code. Annu. Rev. Biochem. 81, 203–229. doi:10.1146/annurev-biochem-060310-170328
Kosinsky, R. L., Zerche, M., Saul, D., Wang, X., Wohn, L., Wegwitz, F., et al. (2020). USP22 exerts tumor-suppressive functions in colorectal cancer by decreasing mTOR activity. Cell Death Differ. 27 (4), 1328–1340. doi:10.1038/s41418-019-0420-8
Lange, S. M., Armstrong, L. A., and Kulathu, Y. (2022). Deubiquitinases: From mechanisms to their inhibition by small molecules. Mol. Cell 82 (1), 15–29. doi:10.1016/j.molcel.2021.10.027
Laoukili, J., Kooistra, M. R., Bras, A., Kauw, J., Kerkhoven, R. M., Morrison, A., et al. (2005). FoxM1 is required for execution of the mitotic programme and chromosome stability. Nat. Cell Biol. 7 (2), 126–136. doi:10.1038/ncb1217
Li, F., Hu, Q., He, T., Xu, J., Yi, Y., Xie, S., et al. (2020). The deubiquitinase USP4 stabilizes Twist1 protein to promote lung cancer cell stemness. Cancers (Basel) 12 (6), 1582. doi:10.3390/cancers12061582
Li, J., Li, H., Zhu, W., Zhou, B., Ying, J., Wu, J., et al. (2020). Deubiquitinase inhibitor degrasyn suppresses metastasis by targeting USP5-WT1-E-cadherin signalling pathway in pancreatic ductal adenocarcinoma. J. Cell Mol. Med. 24 (2), 1370–1382. doi:10.1111/jcmm.14813
Li, W., Cui, K., Prochownik, E. V., and Li, Y. (2018). The deubiquitinase USP21 stabilizes MEK2 to promote tumor growth. Cell Death Dis. 9 (5), 482. doi:10.1038/s41419-018-0523-z
Li, X., He, S., and Ma, B. (2020). Autophagy and autophagy-related proteins in cancer. Mol. Cancer 19 (1), 12. doi:10.1186/s12943-020-1138-4
Li, Z., Liu, X., Yu, H., Wang, S., Zhao, S., and Jiang, G. (2022). USP21 regulates Hippo signaling to promote radioresistance by deubiquitinating FOXM1 in cervical cancer. Hum. Cell 35 (1), 333–347. doi:10.1007/s13577-021-00650-9
Liao, Y., Liu, N., Hua, X., Cai, J., Xia, X., Wang, X., et al. (2017). Proteasome-associated deubiquitinase ubiquitin-specific protease 14 regulates prostate cancer proliferation by deubiquitinating and stabilizing androgen receptor. Cell Death Dis. 8 (2), e2585. doi:10.1038/cddis.2016.477
Liao, Y., Xia, X., Liu, N., Cai, J., Guo, Z., Li, Y., et al. (2018). Growth arrest and apoptosis induction in androgen receptor-positive human breast cancer cells by inhibition of USP14-mediated androgen receptor deubiquitination. Oncogene 37 (14), 1896–1910. doi:10.1038/s41388-017-0069-z
Liu, H., Chen, W., Liang, C., Chen, B. W., Zhi, X., Zhang, S., et al. (2015). WP1130 increases doxorubicin sensitivity in hepatocellular carcinoma cells through usp9x-dependent p53 degradation. Cancer Lett. 361 (2), 218–225. doi:10.1016/j.canlet.2015.03.001
Liu, J., Leung, C. T., Liang, L., Wang, Y., Chen, J., Lai, K. P., et al. (2022). Deubiquitinases in cancers: Aspects of proliferation, metastasis, and apoptosis. J. Cancers (Basel) 14 (14), 3547. doi:10.3390/cancers14143547
Liu, Y., Lashuel, H. A., Choi, S., Xing, X., Case, A., Ni, J., et al. (2003). Discovery of inhibitors that elucidate the role of UCH-L1 activity in the H1299 lung cancer cell line. Chem. Biol. 10 (9), 837–846. doi:10.1016/j.chembiol.2003.08.010
Lu, C. H., Yeh, D. W., Lai, C. Y., Liu, Y. L., Huang, L. R., Lee, A. Y. L., et al. (2018). USP17 mediates macrophage-promoted inflammation and stemness in lung cancer cells by regulating TRAF2/TRAF3 complex formation. Oncogene 37 (49), 6327–6340. doi:10.1038/s41388-018-0411-0
Luo, G., Hu, N., Xia, X., Zhou, J., and Ye, C. (2017). RPN11 deubiquitinase promotes proliferation and migration of breast cancer cells. Mol. Med. Rep. 16 (1), 331–338. doi:10.3892/mmr.2017.6587
Lv, J., Zhang, S., Wu, H., Lu, J., Lu, Y., Wang, F., et al. (2020). Deubiquitinase PSMD14 enhances hepatocellular carcinoma growth and metastasis by stabilizing GRB2. Cancer Lett. 469, 22–34. doi:10.1016/j.canlet.2019.10.025
Magraoui, F. E., Reidick, C., Meyer, H. E., and Platta, H. (2015). Autophagy-related deubiquitinating enzymes involved in health and disease. Cells 4 (4), 596–621. doi:10.3390/cells4040596
Miao, D., Wang, Y., Jia, Y., Tong, J., Jiang, S., and Liu, L. (2022). ZRANB1 enhances stem-cell-like features and accelerates tumor progression by regulating Sox9-mediated USP22/Wnt/β-catenin pathway in colorectal cancer. Cell Signal 90, 110200. doi:10.1016/j.cellsig.2021.110200
Michel, M. A., Swatek, K. N., Hospenthal, M. K., and Komander, D. (2017). Ubiquitin linkage-specific affimers reveal insights into K6-linked ubiquitin signaling. Mol. Cell 68 (1), 233–246.e5. doi:10.1016/j.molcel.2017.08.020
Min, Y., Lee, S., Kim, M. J., Chun, E., and Lee, K. Y. (2017). Ubiquitin-specific protease 14 negatively regulates toll-like receptor 4-mediated signaling and autophagy induction by inhibiting ubiquitination of TAK1-binding protein 2 and Beclin 1. Front. Immunol. 8, 1827. doi:10.3389/fimmu.2017.01827
Mina, A., Yoder, R., and Sharma, P. (2017). Targeting the androgen receptor in triple-negative breast cancer: Current perspectives. Onco Targets Ther. 10, 4675–4685. doi:10.2147/ott.s126051
Nazio, F., Carinci, M., Valacca, C., Bielli, P., Strappazzon, F., Antonioli, M., et al. (2016). Fine-tuning of ULK1 mRNA and protein levels is required for autophagy oscillation. J. Cell Biol. 215 (6), 841–856. doi:10.1083/jcb.201605089
Niu, K., Fang, H., Chen, Z., Zhu, Y., Tan, Q., Wei, D., et al. (2020). USP33 deubiquitinates PRKN/parkin and antagonizes its role in mitophagy. Autophagy 16 (4), 724–734. doi:10.1080/15548627.2019.1656957
Oikawa, D., Shimizu, K., and Tokunaga, F. (2023). Pleiotropic roles of a KEAP1-associated deubiquitinase, OTUD1. Antioxidants (Basel) 12 (2), 350. doi:10.3390/antiox12020350
Pan, J., Deng, Q., Jiang, C., Wang, X., Niu, T., Li, H., et al. (2015). USP37 directly deubiquitinates and stabilizes c-Myc in lung cancer. Oncogene 34 (30), 3957–3967. doi:10.1038/onc.2014.327
Pannem, R. R., Dorn, C., Ahlqvist, K., Bosserhoff, A. K., Hellerbrand, C., and Massoumi, R. (2014). CYLD controls c-MYC expression through the JNK-dependent signaling pathway in hepatocellular carcinoma. Carcinogenesis 35 (2), 461–468. doi:10.1093/carcin/bgt335
Peng, L., Hu, Y., Chen, D., Linghu, R., Wang, Y., Kou, X., et al. (2016). Ubiquitin specific protease 21 upregulation in breast cancer promotes cell tumorigenic capability and is associated with the NOD-like receptor signaling pathway. Oncol. Lett. 12 (6), 4531–4537. doi:10.3892/ol.2016.5263
Peters, A. A., Buchanan, G., Ricciardelli, C., Bianco-Miotto, T., Centenera, M. M., Harris, J. M., et al. (2009). Androgen receptor inhibits estrogen receptor-α activity and is prognostic in breast cancer. Cancer Res. 69 (15), 6131–6140. doi:10.1158/0008-5472.can-09-0452
Popov, N., Wanzel, M., Madiredjo, M., Zhang, D., Beijersbergen, R., Bernards, R., et al. (2007). The ubiquitin-specific protease USP28 is required for MYC stability. Nat. Cell Biol. 9 (7), 765–774. doi:10.1038/ncb1601
Qian, Y., Wong, C. C., Xu, J., Chen, H., Zhang, Y., Kang, W., et al. (2017). Sodium Channel subunit SCNN1B suppresses gastric cancer growth and metastasis via GRP78 degradation. Cancer Res. 77 (8), 1968–1982. doi:10.1158/0008-5472.can-16-1595
Qiu, C., Liu, Y., Mei, Y., Zou, M., Zhao, Z., et al. (2018). Ubiquitin-Specific protease 4 promotes metastasis of hepatocellular carcinoma by increasing TGF-β signaling-induced epithelial-mesenchymal transition. Aging Albany NY 10 (10), 2783–2799. doi:10.18632/aging.101587
Qiu, Y., Wang, J., Li, H., Yang, B., He, Q., et al. (2022). Emerging views of OPTN (optineurin) function in the autophagic process associated with disease. Autophagy 18 (1), 73–85. doi:10.1080/15548627.2021.1908722
Raimondi, M., Cesselli, D., Di Loreto, C., La Marra, F., Schneider, C., and Demarchi, F. (2019). USP1 (ubiquitin specific peptidase 1) targets ULK1 and regulates its cellular compartmentalization and autophagy. Autophagy 15 (4), 613–630. doi:10.1080/15548627.2018.1535291
Schauer, N. J., Magin, R. S., Liu, X., Doherty, L. M., and Buhrlage, S. J. (2020). Advances in discovering deubiquitinating enzyme (DUB) inhibitors. J. Med. Chem. 63 (6), 2731–2750. doi:10.1021/acs.jmedchem.9b01138
Schott, C. R., Ludwig, L., Mutsaers, A. J., et al. (2018). The autophagy inhibitor spautin-1, either alone or combined with doxorubicin, decreases cell survival and colony formation in canine appendicular osteosarcoma cells[J]. PLoS One 13 (10), e206427. doi:10.1371/journal.pone.0206427
Schrecengost, R. S., Dean, J. L., Goodwin, J. F., Schiewer, M. J., Urban, M. W., Stanek, T. J., et al. (2014). USP22 regulates oncogenic signaling pathways to drive lethal cancer progression. Cancer Res. 74 (1), 272–286. doi:10.1158/0008-5472.can-13-1954
Shan, H., Li, X., Xiao, X., Dai, Y., Huang, J., Song, J., et al. (2018). USP7 deubiquitinates and stabilizes NOTCH1 in T-cell acute lymphoblastic leukemia. Signal Transduct. Target Ther. 3, 29. doi:10.1038/s41392-018-0028-3
Shao, S., Li, S., Qin, Y., Wang, X., Yang, Y., Bai, H., et al. (2014). Spautin-1, a novel autophagy inhibitor, enhances imatinib-induced apoptosis in chronic myeloid leukemia. Int. J. Oncol. 44 (5), 1661–1668. doi:10.3892/ijo.2014.2313
Shen, J., Ha, D. P., Zhu, G., Rangel, D. F., Kobielak, A., Gill, P. S., et al. (2017). GRP78 haploinsufficiency suppresses acinar-to-ductal metaplasia, signaling, and mutant Kras-driven pancreatic tumorigenesis in mice. Proc. Natl. Acad. Sci. U. S. A. 114 (20), E4020–E4029. doi:10.1073/pnas.1616060114
Shi, L., Liu, J., Peng, Y., Zhang, J., Dai, X., Zhang, S., et al. (2020). Deubiquitinase OTUD6A promotes proliferation of cancer cells via regulating Drp1 stability and mitochondrial fission. Mol. Oncol. 14 (12), 3169–3183. doi:10.1002/1878-0261.12825
Simicek, M., Lievens, S., Laga, M., Guzenko, D., Aushev, V. N., Kalev, P., et al. (2013). The deubiquitylase USP33 discriminates between RALB functions in autophagy and innate immune response. Nat. Cell Biol. 15 (10), 1220–1230. doi:10.1038/ncb2847
Skaar, J. R., Pagan, J. K., and Pagano, M. (2014). SCF ubiquitin ligase-targeted therapies. Nat. Rev. Drug Discov. 13 (12), 889–903. doi:10.1038/nrd4432
Sowa, M. E., Bennett, E. J., Gygi, S. P., and Harper, J. W. (2009). Defining the human deubiquitinating enzyme interaction landscape. Cell 138 (2), 389–403. doi:10.1016/j.cell.2009.04.042
Sun, X. X., He, X., Yin, L., Komada, M., Sears, R. C., and Dai, M. S. (2015). The nucleolar ubiquitin-specific protease USP36 deubiquitinates and stabilizes c-Myc. Proc. Natl. Acad. Sci. U. S. A. 112 (12), 3734–3739. doi:10.1073/pnas.1411713112
Suresh, B., Lee, J., Kim, K. S., and Ramakrishna, S. (2016). The importance of ubiquitination and deubiquitination in cellular reprogramming. Stem Cells Int. 2016, 6705927. doi:10.1155/2016/6705927
Tao, L., Liu, X., Jiang, X., Zhang, K., Wang, Y., Li, X., et al. (2022). USP10 as a potential therapeutic target in human cancers. Genes (Basel) 13 (5), 831. doi:10.3390/genes13050831
Tavana, O., and Gu, W. (2017). Modulation of the p53/MDM2 interplay by HAUSP inhibitors. J. Mol. Cell Biol. 9 (1), 45–52. doi:10.1093/jmcb/mjw049
Thayer, J. A., Awad, O., Hegdekar, N., Sarkar, C., Tesfay, H., Burt, C., et al. (2020). The PARK10 gene USP24 is a negative regulator of autophagy and ULK1 protein stability. Autophagy 16 (1), 140–153. doi:10.1080/15548627.2019.1598754
Tian, Z., D'Arcy, P., Wang, X., Ray, A., Tai, Y. T., Hu, Y., et al. (2014). A novel small molecule inhibitor of deubiquitylating enzyme USP14 and UCHL5 induces apoptosis in multiple myeloma and overcomes bortezomib resistance. Blood 123 (5), 706–716. doi:10.1182/blood-2013-05-500033
Uehara, I., and Tanaka, N. (2018). Role of p53 in the regulation of the inflammatory tumor microenvironment and tumor suppression. Cancers (Basel) 10 (7), 219. doi:10.3390/cancers10070219
Vishnoi, M., Boral, D., Liu, H., Sprouse, M. L., Yin, W., Goswami-Sewell, D., et al. (2018). Targeting USP7 identifies a metastasis-competent state within bone marrow-resident melanoma CTCs. J. Cancer Res. 78 (18), 5349–5362. doi:10.1158/0008-5472.can-18-0644
Wang, M., Zhang, Y., Wang, T., Zhang, J., Zhou, Z., Sun, Y., et al. (2017). The USP7 inhibitor P5091 induces cell death in ovarian cancers with different P53 status. Cell Physiol. Biochem. 43 (5), 1755–1766. doi:10.1159/000484062
Wang, S., Juan, J., Zhang, Z., Du, Y., Xu, Y., Tong, J., et al. (2017). Inhibition of the deubiquitinase USP5 leads to c-Maf protein degradation and myeloma cell apoptosis. Cell Death Dis. 8 (9), e3058. doi:10.1038/cddis.2017.450
Wang, W., Wang, M., Xiao, Y., Wang, Y., Guo, L., et al. (2022). USP35 mitigates endoplasmic reticulum stress-induced apoptosis by stabilizing RRBP1 in non-small cell lung cancer. Mol. Oncol. 16 (7), 1572–1590. doi:10.1002/1878-0261.13112
Wang, X., Liu, Z., Zhang, L., Yang, Z., Chen, X., Luo, J., et al. (2018). Targeting deubiquitinase USP28 for cancer therapy. Cell Death Dis. 9 (2), 186. doi:10.1038/s41419-017-0208-z
Wang, X., Mazurkiewicz, M., Hillert, E. K., Olofsson, M. H., Pierrou, S., Hillertz, P., et al. (2016). The proteasome deubiquitinase inhibitor VLX1570 shows selectivity for ubiquitin-specific protease-14 and induces apoptosis of multiple myeloma cells. Sci. Rep. 6, 26979. doi:10.1038/srep26979
Wang, Y., Serricchio, M., Jauregui, M., Shanbhag, R., Stoltz, T., Di Paolo, C. T., et al. (2015). Deubiquitinating enzymes regulate PARK2-mediated mitophagy. Autophagy 11 (4), 595–606. doi:10.1080/15548627.2015.1034408
Weng, W., Zhang, Q., Xu, M., Wu, Y., Zhang, M., Shen, C., et al. (2016). OTUB1 promotes tumor invasion and predicts a poor prognosis in gastric adenocarcinoma. Am. J. Transl. Res. 8 (5), 2234–2244.
Xia, X., Huang, C., Liao, Y., Liu, Y., He, J., Shao, Z., et al. (2021). The deubiquitinating enzyme USP15 stabilizes ERα and promotes breast cancer progression. Cell Death Dis. 12 (4), 329. doi:10.1038/s41419-021-03607-w
Xing, C., Lu, X. X., Guo, P. D., Shen, T., Zhang, S., He, X. S., et al. (2016). Ubiquitin-specific protease 4-mediated deubiquitination and stabilization of PRL-3 is required for potentiating colorectal oncogenesis. Cancer Res. 76 (1), 83–95. doi:10.1158/0008-5472.can-14-3595
Xu, P., Xiao, H., Yang, Q., Hu, R., Jiang, L., Bi, R., et al. (2020). The USP21/YY1/SNHG16 axis contributes to tumor proliferation, migration, and invasion of non-small-cell lung cancer. Exp. Mol. Med. 52 (1), 41–55. doi:10.1038/s12276-019-0356-6
Xu, X., Li, S., Cui, X., Han, K., Wang, J., Hou, X., et al. (2019). Inhibition of ubiquitin specific protease 1 sensitizes colorectal cancer cells to DNA-damaging chemotherapeutics. Front. Oncol. 9, 1406. doi:10.3389/fonc.2019.01406
Ye, D. X., Wang, S. S., Huang, Y., and Chi, P. (2021). USP43 directly regulates ZEB1 protein, mediating proliferation and metastasis of colorectal cancer. J. Cancer 12 (2), 404–416. doi:10.7150/jca.48056
Yeo, S. K., Paul, R., Haas, M., Wang, C., and Guan, J. L. (2018). Improved efficacy of mitochondrial disrupting agents upon inhibition of autophagy in a mouse model of BRCA1-deficient breast cancer. Autophagy 14 (7), 1214–1225. doi:10.1080/15548627.2018.1460010
Yoshida, G. J. (2018). Emerging roles of Myc in stem cell biology and novel tumor therapies. J. Exp. Clin. Cancer Res. 37 (1), 173. doi:10.1186/s13046-018-0835-y
Yu, J., Tao, Q., Cheung, K. F., Jin, H., Poon, F. F., Wang, X., et al. (2008). Epigenetic identification of ubiquitin carboxyl-terminal hydrolase L1 as a functional tumor suppressor and biomarker for hepatocellular carcinoma and other digestive tumors. Hepatology 48 (2), 508–518. doi:10.1002/hep.22343
Yu, Z., Li, Y., Han, T., and Liu, Z. (2019). Demethylation of the HACE1 gene promoter inhibits the proliferation of human liver cancer cells. Oncol. Lett. 17 (5), 4361–4368. doi:10.3892/ol.2019.10139
Yuan, L., Lv, Y., Li, H., Gao, H., Song, S., Zhang, Y., et al. (2015). Deubiquitylase OTUD3 regulates PTEN stability and suppresses tumorigenesis. Nat. Cell Biol. 17 (9), 1169–1181. doi:10.1038/ncb3218
Zhang, J., Cao, M., Dong, J., Li, C., Xu, W., Zhan, Y., et al. (2014). ABRO1 suppresses tumourigenesis and regulates the DNA damage response by stabilizing p53. Nat. Commun. 5, 5059. doi:10.1038/ncomms6059
Zhang, P., Li, C., Li, H., Yuan, L., Dai, H., Peng, Z., et al. (2020). Ubiquitin ligase CHIP regulates OTUD3 stability and suppresses tumour metastasis in lung cancer. Cell Death Differ. 27 (11), 3177–3195. doi:10.1038/s41418-020-0571-7
Zhang, R., Ozgen, S., Luo, H., Krigman, J., Zhao, Y., Xin, G., et al. (2022a). The mitochondrial deubiquitinase USP30 regulates AKT/mTOR signaling. Front. Pharmacol. 13, 816551. doi:10.3389/fphar.2022.816551
Zhang, Y., Jia, J., Jin, W., Cao, J., Fu, T., Ma, D., et al. (2020b). Lidocaine inhibits the proliferation and invasion of hepatocellular carcinoma by downregulating USP14 induced PI3K/Akt pathway. Pathol. Res. Pract. 216 (8), 152963. doi:10.1016/j.prp.2020.152963
Zhang, Z., Fan, Y., Xie, F., Zhou, H., Jin, K., Shao, L., et al. (2017). Breast cancer metastasis suppressor OTUD1 deubiquitinates SMAD7. Nat. Commun. 8 (1), 2116. doi:10.1038/s41467-017-02029-7
Zhao, L., Wang, X., Yu, Y., Deng, L., Chen, L., Peng, X., et al. (2018). OTUB1 protein suppresses mTOR complex 1 (mTORC1) activity by deubiquitinating the mTORC1 inhibitor DEPTOR. J. Biol. Chem. 293 (13), 4883–4892. doi:10.1074/jbc.m117.809533
Zhao, Y., Xiong, X., and Sun, Y. (2011). DEPTOR, an mTOR inhibitor, is a physiological substrate of SCFβTrCP E3 Ubiquitin ligase and regulates survival and autophagy. Mol. Cell 44 (2), 304–316. doi:10.1016/j.molcel.2011.08.029
Zhou, H., Liu, Y., Zhu, R., Ding, F., Cao, X., Lin, D., et al. (2018). OTUB1 promotes esophageal squamous cell carcinoma metastasis through modulating Snail stability. Oncogene 37 (25), 3356–3368. doi:10.1038/s41388-018-0224-1
Zhou, Y., Wu, J., Fu, X., Du, W., Zhou, L., Meng, X., et al. (2014). OTUB1 promotes metastasis and serves as a marker of poor prognosis in colorectal cancer. Mol. Cancer 13, 258. doi:10.1186/1476-4598-13-258
Keywords: deubiquitinase, deubiquitination, tumor treatment, deubiquitinase inhibitor, cancer
Citation: Liang X-W, Wang S-Z, Liu B, Chen J-C, Cao Z, Chu F-R, Lin X, Liu H and Wu J-C (2023) A review of deubiquitinases and thier roles in tumorigenesis and development. Front. Bioeng. Biotechnol. 11:1204472. doi: 10.3389/fbioe.2023.1204472
Received: 12 April 2023; Accepted: 03 May 2023;
Published: 12 May 2023.
Edited by:
Ru Huang, Hainan University, ChinaReviewed by:
Zhijin Fan, Sun Yat-sen University, ChinaHaocai Chang, South China Normal University, China
Jinshun Xu, Sichuan Cancer Hospital, China
Xiaohai Song, Sichuan University, China
Hua-Yang Pang, Chongqing University, China
Copyright © 2023 Liang, Wang, Liu, Chen, Cao, Chu, Lin, Liu and Wu. This is an open-access article distributed under the terms of the Creative Commons Attribution License (CC BY). The use, distribution or reproduction in other forums is permitted, provided the original author(s) and the copyright owner(s) are credited and that the original publication in this journal is cited, in accordance with accepted academic practice. No use, distribution or reproduction is permitted which does not comply with these terms.
*Correspondence: Hui Liu, huihui25808@163.com; Jin-Cai Wu, wujincai021@126.com
†These authors have contributed equally to this work and share first authorship