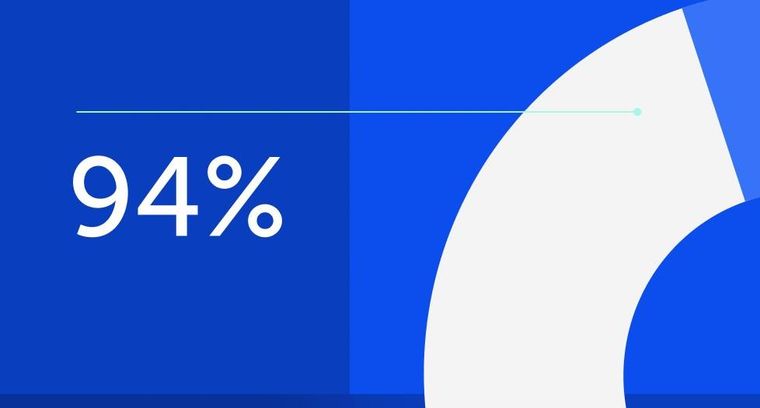
94% of researchers rate our articles as excellent or good
Learn more about the work of our research integrity team to safeguard the quality of each article we publish.
Find out more
REVIEW article
Front. Bioeng. Biotechnol., 21 July 2023
Sec. Biomaterials
Volume 11 - 2023 | https://doi.org/10.3389/fbioe.2023.1191327
This article is part of the Research TopicBioactive Materials for Disease Diagnosis and TherapyView all 17 articles
The new and unique possibilities that nanomaterials offer have greatly impacted biomedicine, from the treatment and diagnosis of diseases, to the specific and optimized delivery of therapeutic agents. Technological advances in the synthesis, characterization, standardization, and therapeutic performance of nanoparticles have enabled the approval of several nanomedicines and novel applications. Discoveries continue to rise exponentially in all disease areas, from cancer to neurodegenerative diseases. In Spain, there is a substantial net of researchers involved in the development of nanodiagnostics and nanomedicines. In this review, we summarize the state of the art of nanotechnology, focusing on nanoparticles, for the treatment of diseases in Spain (2017–2022), and give a perspective on the future trends and direction that nanomedicine research is taking.
Nanoparticles (NPs) are small particles usually around 10–100 nm in size, that can be obtained from a broad class of materials, (Khan et al., 2019), and are classified according to their nature (Figure 1). Materials have different properties at a nanometric scale, such as higher reactivity, singular optical or magnetic properties, among others. These properties can be used as warheads against pathological conditions (Fratila et al., 2019), (Guisasola et al., 2018a). Nanomedicine takes advantage of these unique features, offering a new set of therapeutic and diagnostic tools. For example, in NP-mediated hyperthermia, each NP acts as a heat source, increasing the temperature specifically at localized areas, damaging specific tumor cells in a controlled manner and reducing side effects in healthy tissues (in contrast with the bulk heating of conventional hyperthermia) (Vilaboa et al., 2017), (Sanz et al., 2017). A rise in temperature to 41°C–50°C induces cell death via necrosis and/or apoptosis, especially for the more thermosensitive cancer cells (Bass et al., 1978), (Sapareto and Dewey, 1984). In addition, hyperthermia increases blood irrigation preferentially within tumors (Elming et al., 2019), modifies the extracellular matrix of tumor tissue (Kolosnjaj-Tabi et al., 2017), and activates immunological responses by increasing the surface display of tumor antigens (Lee et al., 2018).
FIGURE 1. Main types of NPs and the therapeutic areas in which they are used in the articles included in this review.
The shape, size, and surface of NPs are important properties to consider when using them for biological applications, since they determine the biocompatibility, biodistribution, cell-targeting, and uptake efficiency. NPs can be surface-modified with different biomolecules, including small proteins, antibodies, aptamers, oligonucleotides, oligosaccharides, polymers, or drugs. Therefore, by selecting suitable molecules, NPs can be tailored for the desired biological applications (Jindal, 2017), (Dolai et al., 2021), (Gatoo et al., 2014). In addition, the nanoformulation protects the cargo from degradation and improves its distribution in physiological media, facilitating oral administration and improving cell entry (Kim et al., 2021). Coating NPs with PEG, shields the surface from aggregation, opsonization, and phagocytosis, prolonging systemic circulation time and reducing their immunogenicity (Shi et al., 2021). Thus, different functionalization strategies make possible to enhance the pharmacokinetic properties of NPs, boosting the efficacy of therapy (Dacoba et al., 2017).
Another fundamental aspect is the protein corona formed on the surface of NPs, which plays a crucial role in the biological identity of NPs as it affects cytotoxicity, body distribution, endocytosis into specific cells, and biodegradation (Stepien et al., 2018), (Garcia-Alvarez and Vallet-Regi, 2021; Fleury et al., 2021). For these reasons, the proper identification and characterization of protein corona are essential in developing NPs-based therapeutics (Ritz et al., 2015; Di Silvio et al., 2018), (Alfranca et al., 2019).
NPs have a significant application as drug delivery systems (Miron-Barroso et al., 2021). Targeted therapies have at least three main advantages: reaching the target site specifically, not affecting other healthy organs, and reducing the dose needed to have the same therapeutic effect to the free drug. Biological barriers such as the blood-brain barrier (BBB) or mucus have a protective nature, hindering the simple diffusion of some therapeutic molecules, and a specific NP-mediated delivery can improve the permeability of therapeutic agents (Mulvihill et al., 2020), (Martin-Rapun et al., 2017). Finally, the possibility to encapsulate more than one drug that could provide synergistic effects showcases NPs versatility for delivery.
A significant example of the success of NPs as carriers is the drug Abraxane®. This nanomedicine, which comprises the chemotherapeutic paclitaxel (PTX) bound to albumin, has been approved for the treatment of metastatic breast cancer, advanced non-small cell lung cancer, late-stage pancreatic cancer, and metastatic triple-negative breast cancer. In several studies, it has increased patient survival and response rate significantly (De Luca et al., 2019).
Moreover, nanotechnology has also acquired a great interest in the immunological field. Vaccines are an extremely effective strategy to prevent several diseases, however their generation can be challenging since it is necessary to finely regulate the immunogenicity and the use of adjuvants as immunostimulatory agents can be critical to produce the desired effect. NPs have importance in vaccine generation because they are easily recognized by immune cells as they have a similar size to pathogens, and molecules can be anchored to their membrane to improve their recognition. In addition, new routes of administration, such as oral and nasal, can be used, and the possibility of multivalency enhances their activity (Miron-Barroso et al., 2021), (Gonzalez-Aramundiz et al., 2018).
Spain has emerged as a prominent player in the field of nanomedicine research in recent years. With numerous cutting-edge research centers and groups dedicated to this field, the country has made significant strides in the development of advanced treatments. Our review aims to provide a comprehensive overview of the latest breakthroughs in nanomedicine treatments in Spain over the past 5 years. By highlighting the capabilities of various research centers and groups, we hope to shed light on the role that Spain plays in advancing this exciting field.
Inorganic NPs encompass the nanoformulations that mainly contain inorganic elements. They include magnetic, metallic, gold, silver NPs and metallic quantum dots, among others, and have been studied as therapeutic systems against various diseases. For example, the plasmonic and optical properties of inorganic NPs have enabled to undertake innovative approaches to treat diseases, serving as improved contrast agents or thermo-photo-induced sources (Jaque et al., 2014), (Kim et al., 2018), (Borzenkov et al., 2019). Their tunable morphological properties and advantageous stability have promoted great promises in their nanomedical use. However, the long-term effects of the administration of these NPs in the human body still needs exhaustive characterization, which has resulted in a slower clinical translation of these NPs in comparison to their organic counterparts (Paul et al., 2020). In this review, recent advances in silica, magnetic, gold, and silver NPs, are summarized. At the end of each section, the studies are summarized in tables and categorized by NP type highlighting their purpose, therapeutic area, agent and functionalization strategy.
Mesoporous silica NPs (MSNs) present several advantages that have qualified them as ideal carriers for drug delivery (Manzano and Vallet-Regi, 2019), (Manzano and Vallet-Regi, 2018), (Iturrioz-Rodriguez et al., 2019). They have large pores (0.6–1 cm3) to encapsulate molecules of different sizes (including proteins), an easily modifiable surface that allows controlling drug loading and release, a very high surface-to-volume ratio, and good biocompatibility (Villegas et al., 2018), (Vallet-Regi et al., 2022). In addition, they can be prepared at different sizes (50–200 nm) and present a large surface area (Vallet-Regi et al., 2017). The group of Vallet-Regí was the first one to report the use of ordered mesoporous silica for drug delivery using the mesoporous material MCM-41 for the controlled release of ibuprofen using a simulated body fluid (Vallet-Regi et al., 2001). After that, tailored strategies have been developed for an efficient and smart delivery of therapeutic molecules, mainly for cancer treatment. For example, MSNs can be tuned to be responsive to a specific pH through an acid-sensitive linker, increasing tumor selectivity and efficacy, (Martinez-Carmona et al., 2018), and they can also be tuned to target specific organelles (Table 1) (Gisbert-Garzaran et al., 2020).
The delivery of the specific agent of MSNs can be controlled in different manners (Vallet-Regi et al., 2022). For example, Poyatos-Racionero et al. prepared MSNs loaded with essential oil components and covered by lactose that functioned as a molecular gate. They explored the properties of these particles loaded with different active agents in cellular and animal models, confirming the potential of this strategy for controlled delivery (Poyatos-Racionero et al., 2021). With regard to toxicity, mesoporous silica rods (MSR) have been less investigated in terms of biodistribution, biocompatibility, and cellular uptake, however in this case they presented improved characteristics when compared to their spherical counterparts in animal models. In this scenario, MSRs were functionalized with magnetic and fluorescent elements for diagnosis and treatment of fibrotic liver diseases (Grzelak et al., 2022).
Jimenez-Falcao et al. brought further the strategy of on-command delivery engineering loaded MSNs with a layer-by-layer supramolecular architecture, each with a specific role. The particles were first functionalized by benzimidazole and β-cyclodextrin gold NPs that act as a pH-sensitive gate. Then, a final coating was performed with glucose oxidase modified with an adamantine moiety linked to the free cyclodextrins. In that manner, these conjugates delivered their cargo upon the addition of glucose and were able to reduce HeLa cell viability (Jimenez-Falcao et al., 2019). A different delivery strategy was developed by Muñoz-Espín et al. to target senescent cells. They used MSNs coated with galacto-oligosaccharides, taking advantage of the high activity of lysosomal β-galactosidase activity in senescent cells. The nanoconjugates showed a preferential accumulation in senescent cells in animal models, improving tumor regression in mice and reducing the side effect of toxic drugs (Munoz-Espin et al., 2018).
To enhance delivery and exploit different ways of cellular entry, Navarro-Palomares et al. took advantage of cytoplasmatic entry of the toxic Shiga protein. They prepared fluorescent MSNs conjugated to a safe fragment of the protein that enabled to deliver the NPs intracellularly by a non-canonical pathway and thus avoiding the endolysosomal entry and its associated degradation (Navarro-Palomares et al., 2021).
Llinas et al. prepared a pH responsive nanosystem to deliver several drugs. First, they developed a nanosystem capable of delivering camptothecin (CPT) and doxorubicin (DOX) (Llinas et al., 2018), and, in a further step, they developed a new system for the delivery of CPT, DOX, and zinc (II) phthalocyanine (Pc). They labelled (Pc-CPT)@MSN-hyd-PEG-hyd-DOX, which sequentially releases DOX, linked on the MSNs surface through a pH-sensitive PEG linker that gradually delivers the Pc-CPT conjugate loaded inside the MSNs. In this manner, they combined chemotherapy and photosensitizers for photodynamic therapy (PDT). Upon irradiation of the samples, the Pc phototoxicity enhances the chemotoxicity of DOX and CPT (Martinez-Edo et al., 2021).
In a further step, self-propelled NPs also enable a more efficient manner to reach their target site without the need for external stimuli. Diez et al. designed MSNs coupled with platinum nanodendrites as a self-propulsion element (Figure 2). The particles were designed with an oligoethylenglycol containing a disulfide element that acts as a gating system that can be opened under specific redox conditions. This is a successful proof of concept of a nanomaterial that can autonomously reach its target and deliver the cargo upon specific and controllable conditions (Diez et al., 2021).
FIGURE 2. Design of Janus Pt-MSN motors. Reprinted from Diez et al. (Diez et al., 2021), and licensed under the Creative Commons Attribution.
Another complicated site for drug delivery are the lungs, which present several pulmonary barriers that prevent the effective delivery of traditional drugs. In this regard, García-Fernández et al. addressed this issue using MSNs (Garcia-Fernandez et al., 2021a), (Garcia-Fernandez et al., 2021b). In this case, the nanostructures were loaded with dexamethasone, which is the standard corticoid used for the treatment of this disease, and decorated with a peptide with a dual objective, targeting TNFR1 receptors and avoiding the release of the cargo. TNFR1 receptors are expressed in pro-inflammatory macrophages, and indeed the particles showed a selective uptake by these cells and released the drug. The conjugates were also effective in animal models, demonstrating lung accumulation and the reduction of the damage.
As seen by now, the versatility of NP development with therapeutic approaches enables infinite combinations of coatings, particle size, shape, and biomolecule incorporation. In addition, one can combine several NPs into new assemblies that enable to take advantage of several properties simultaneously (Redolfi Riva et al., 2017). When NPs display two or more physical properties divided on their surface, they are called Janus particles, due to their asymmetric geometry. They can also be prepared with the combination of different kinds of NPs that enable them to take advantage of both materials, and even unlock undiscovered synergistic effects. Mayo et al. developed mesoporous silica-gold Janus NPs linked to glutathione reductase. These assemblies resulted highly efficient in delivering their cargo when presented with NADPH and glutathione disulfide as triggers (Mayol et al., 2021).
Similarly, Guisasola et al. developed innovative MSNs loaded with iron oxide NPs coated with a polymer sensitive to temperature. Upon the application of alternating magnetic fields (AMF), DOX was effectively released in animal models without increasing global tissue temperature, provoking a synergistic effect of drug and hyperthermia antitumoral activities (Guisasola et al., 2018b). In another example of Janus-type MSNs, Lopez et al. designed particles selective for tumor cells that, once internalized, specifically targeted mitochondria organelles, showcasing the utility of this strategy (Lopez et al., 2017).
Bacterial resistance to common antimicrobials is growing, and many efforts are being dedicated to the development of new antibiotic tools. Biofilm formation is especially problematic because it requires much higher antibiotic doses. MSNs can be designed to deliver antimicrobials in a localized and efficient way (Bernardos et al., 2019), (Vallet-Regi et al., 2019), (Colilla and Vallet-Regi, 2020). This approach has been used in MSNs functionalized with concanavalin A, which promotes the internalization of the NPs into the biofilm matrix to deliver levofloxacin (Martinez-Carmona et al., 2019). Also, in a sophisticated example, the release of levofloxacin and nitric oxide in biofilms was enhanced by near-infrared (NIR) irradiation using core-shell Au-MSN NPs (García et al., 2021). Aguilera-Correa et al. developed gelatin/colistin coated MSNs to treat osteomyelitis, a bone infection with poor prognosis. The functional coating prevented premature antibiotic release and induced biofilm disaggregation, showing the potential of these NPs to treat bone infections (Aguilera-Correa et al., 2022a). Finally, Montalvo-Quirós et al. explored the antimicrobial activity of MSNs loaded with silver bromide NPs and silver NPs with a mesoporous silica shell, confirming the great potential of MSNs for this application (Montalvo-Quiros et al., 2021).
Another prominent field of nanomedicine is the use of MSNs in bone regeneration. Mora-Raimundo et al. engineered particles to simultaneously deliver siRNA, to silence genes that inhibit osteoblasts differentiation, and osteostatin, which stimulates bone regeneration in animal models, taking advantage of the high loading capacity of MSNs (Mora-Raimundo et al., 2019). Further, Arcos et al. developed a methodology to inject MSNs loaded with an antiosteoporotic drug, ipriflavone, for the first time in rabbits, by suspending them in a hyaluronic acid hydrogel. The particles induced osteogenesis and bone repair (Arcos et al., 2022). In the same field, Casarrubios et al. decided to take advantage of the essential role that angiogenesis plays in vascularization and tissue regeneration. They loaded mesoporous nanospheres with ipriflavone, showing its release in endothelial cells by the increase of VEGFR2 expression indicating angiogenesis (Casarrubios et al., 2021). In another publication, the authors explored the potential of ipriflavone MSNs for periodontal treatment. They confirmed the clathrin-mediated entrance of the NPs, showing an osteogenesis activity (Casarrubios et al., 2020).
An original manner to deliver NPs was developed by Iturrioz-Rodriguez et al. that coated silica NPs with carbon nanotubes that enabled a cytoplasmic delivery and opens an innovative pathway to reach the cytoplasm of cells (Iturrioz-Rodriguez et al., 2017).
Finally, MSNs have also ideal properties to become a platform for vaccine development. Montalvo-Quirós et al. engineered MSNs loaded with immunomodulatory proteins that showed to have protective effects against infection of tuberculosis, (Montalvo-Quiros et al., 2020), and that could be used for dual delivery of immunomodulatory proteins and antitubercular drugs.
Magnetic NPs (MNPs) are an exceptional tool for biomedical treatment. They can deeply internalize in tissues, and have magnetic-heating capability. The most explored MNPs for nanomedicine are, by far, superparamagnetic iron oxide NPs (SPIONs) and related ferrites (Roca et al., 2019), (Pardo et al., 2020), (Mazario et al., 2012), (Garcia-Soriano et al., 2020), (Rubia-Rodriguez et al., 2021a). In clinical practice and biomedical research, they are used as iron supplements, contrast agents, and magnetic hyperthermia therapeutics (Polo et al., 2018), (de la Presa et al., 2012). Through the later application, localized heat is generated, which increases gene expression (Moros et al., 2019), particularly of the heat shock protein family, and the formation of reactive oxygen species (ROS), while inducing apoptosis and several cellular stresses such as endoplasmic reticular stress or mitochondrial damage. Interestingly, cancer cells are more sensitive to heat than healthy ones, and therefore, this approach can present reduced toxicity in healthy cells and tissues. On the other hand, the requirement to use this therapy is the application of an alternating magnetic field (AMF), which has a high penetration and negligible effect on the tissues, compared with other antitumoral techniques that present toxic side effects for nearby healthy cells and tissues. Finally, magnetic hyperthermia can be used to promote drug release and therefore it can be used alone or in combination with drugs, for synergistic combinatory therapy, or with specific molecules for active targeting (Table 2). For example, Fe3O4 NPs were synthesized by a seed growth method with defined shapes and sizes and were functionalized with an arginine-glycine-aspartate (RGD)-type peptide to target αvβ3 integrin receptors over-expressed in angiogenic cancer cells. NPs showed a good heating response, lower toxicity and better biocompatibility with improved magnetic properties (Arriortua et al., 2018). Sanz et al. extensively studied and compared conventional hyperthermia and the therapeutic advantage of using MNPs, confirming the improved effectiveness of the nanoheaters (Sanz et al., 2017). In case of other type of hyperthermia, Lozano-Pedraza et al. explored the optical heat losses using iron oxide NPs and identified the parameters that influence the NIR-heating effects for therapeutic purposes (Lozano-Pedraza et al., 2021).
Remarkably, iron oxide and other NPs can be obtained in a wide variety of morphologies and anisometric properties (Figure 3) (Roca et al., 2019) including, rods, cubes, stars, rings or as flower-shaped NPs among others (Gavilán et al., 2017). It is worth mentioning that the biological properties (e.g., internalization, toxicity) and response to AMF vary upon specific morphology (Ovejero et al., 2021a), (Simeonidis et al., 2016).
FIGURE 3. Different shapes and morphologies of iron oxide and other NPs. Scanning and transmission electron microscopy images on the upper part and morphology representation on the lower part. (A) nanorod, (B) nanohusk, (C) distorted cubes, (D) nanosheets, (E) distorted cubes, (F) porous spheres, (G) self-oriented flowers, (H) prismatic IONPs, (I) nanostar, (J) nanodiscs, (K) nanorings and (L) nanotubes. (A, B, C, E, F, G) adapted from Sayed and Polshettiwar (2015), (D) adapted from Chin et al. (2007), (I) adapted from Becerril-Castro et al. (2022), (J) adapted from Qu et al. (2021), (H) adapted from Ramirez-Jimenez et al. (2020), (K) adapted from Jia et al. (2008), (L) adapted from Zhang et al. (2016). Licensed under a Creative Commons Attribution.
MNPs can be also combined with other nanostructures to yield nanostructures with additional properties. In this regard, Paterson et al. engineered self-assembled gold suprashells around dextran-coated SPION cores, which allowed them to obtain nanostructures with plasmonic and magnetic properties. The use of magnetic fields can be used to promote the accumulation of these nanostructures in cancer cells, and then exploit the plasmonic properties to induce heat by a light source, leading to the death of the tumoral cells (Paterson et al., 2017). In another work, MNPs were functionalized with dextran and crocin, which has antiproliferative properties. Crocin-coated dextran-MNPs showed greater anti-tumor effects and a higher rate of early apoptosis on MCF-7 breast tumor cells than free crocin was obtained, suggesting an effective alternative to traditional cancer treatments (Saravani et al., 2020).
Mejias et al., have worked in hyperthermia that improves tumor antigen presentation, activation of dendritic cells and natural killer cells, and leukocyte trafficking through endothelium. Probably due to NP aggregation, the contact of MNPs with cells could affect the heating capacity, highlighting the importance of NP coating to avoid cell-induced aggregation (Mejias et al., 2019). Similarly, Cabrera et al. studied intracellular MNP clustering, that led to a reduction of the magnetic hyperthermia ability. This work allowed to predict the magnetic thermal response of several NPs sizes in the cellular media (Cabrera et al., 2018).
Beola et al. investigated MNPs activity in 3D cell cultures, showing that magnetic hyperthermia can trigger necrosis or disruption of the extracellular matrix depending if the MNPs are inside or outside the cells (Beola et al., 2018). They studied the cell death mechanisms and the influence of the number of internalized particles to the cytotoxic effect testing several concentrations up to 7.5 pg Fe/cell. They showed that different apoptotic routes are triggered depending on the number of internalized NPs (Beola et al., 2020). In another work, they selected the conditions that caused the largest effect in cell viability for testing the NPs in animal models (Beola et al., 2021). AMF promoted MNPs migration into the tumor and confirmed that NP biodistribution is essential for hyperthermia effectiveness, and is affected by surface coating, playing the protein corona a significant role (Stepien et al., 2018).
Also employing 3D cell cultures, the group of V. Salgueiriño and co-workers described the assembly of magnetic microswimmers, composed of 500 nm polystyrene particles containing ferrite NPs. The motion of the self-propelled microswimmers was triggered by calcium, and they were able to penetrate spheroid models for heat delivery under AMF (Ramos-Docampo et al., 2019).
Luengo et al. synthesized maghemite NPs with different coatings to determine the best properties to use in clinical applications. The NPs were injected into animals with pancreatic cancer, and the results determined that modulating the field intensity can control the temperature rise during magnetic hyperthermia protocols in animal models (Luengo et al., 2022).
The combination of experimental and simulation approaches might be a useful tool for better engineering NPs. In this regard, a model has provided quantitative predictions to fit the properties of iron NPs, including a targeting agent and a drug. Particularly, it allowed the design of NPs with a pseudopeptide Nucant-6L, which induced a significant accumulation in tumors. The studies revealed the synergy of Nucant-6L, the chemotherapeutic drug gemcitabine, and the NPs, together with the importance of fine tuning the functionalization (Aires et al., 2017).
Christou et al. developed a seed-assisted methodology for the synthesis of gold and iron oxide nanoflowers. The particles were functionalized with PEG, greatly enhancing the colloidal stability of the conjugates. The nanoflowers performed highly as contrast agents and exhibited a considerable conversion of energy to heat, having ideal properties to be used as theragnostic agents (Christou et al., 2022).
Del Sol-Fernandez et al. also developed flowerlike manganese iron oxide cRGD-functionalized NPs that, when exposed to the appropriate AMF conditions, induced intracellular magnetic hyperthermia resulting in hsp70 transcription and strong ROS production leading to cell death in a glioblastoma cell line (Del Sol-Fernandez et al., 2019).
Espinosa et al. developed Janus magneto-plasmonic NPs, using gold nanostars and iron oxide nanospheres subjected to an external magnetic field and NIR light. With this strategy, a synergistic cytotoxic effect on cancer cells was achieved based on the combination of the two thermal effects into a magneto-photothermal modality. Moreover, experiments in animal models confirmed the high efficiency of magnetically enhanced photothermal therapy (PTT) that led to tumor growth inhibition, and the delivery was highly improved by magnetic targeting (Espinosa et al., 2020). Another type of magneto-plasmonic materials that display magneto- and photothermal anisotropic transductions for cancer ablation has been proposed by Rincon- Iglesias et al., that incorporated Fe3O4@Au nanorods in an agarose hydrogel, resulting in free-standing anisotropic materials (Rincon-Iglesias et al., 2022).
Mulens-Arias et al., investigated the modulation of angiogenesis as an antitumor therapy. They used MNPs and a magnetic field for this approach. PEI-SPIONs, (SPIONs coated with polyethylenimine) showed anti-angiogenic and antitumoral effects as these NPs were able to reduce tumor vessel numbers and promoted intratumor macrophage infiltration in a tumor model after administration and application of magnetic field (Mulens-Arias et al., 2019). As another strategy against cancer, Sanz Ortega et al. developed NPs-based drug delivery systems to increase immunotherapy effectiveness. They showed that MNPs and the use of AMF can guide and retain T lymphocytes to a target region of interest and can be magnetically retained there (Sanz-Ortega et al., 2019a), (Sanz-Ortega et al., 2019b). In addition, they took advantage of the role of natural killer cells in antitumor immunity by binding MNPs coated with 3-aminopropyl triethoxysilane (APTES) to the surface of natural killer cells. They reported the retention of the cells at the specific target site by using external magnetic fields as the magnetic guiding effect (Sanz-Ortega et al., 2019c).
While hyperthermia has been exploited using several conjugates, an unsolved problem in this field is the lack of real time information on the temperature achieved locally, which complicates a fine control of therapeutic parameters in situ. Ximendes et al. combined in a recent work MNPs with infrared nanothermometers of Ag2S NPs that provided an efficient solution to this problem by monitoring the subcutaneous temperatures in real time, to build 2D thermal maps, which were used to accurately assess the therapeutic effect of the MNPs (Ximendes et al., 2021). Alternatively, the temperature at the surface of AMF-activated MNPs was also obtained with fluorescence probes by J. Ovejero et al. (Ovejero et al., 2021b). Moreover, to guarantee an efficient thermal treatment in tumors in a safe window of applicability in the clinical practice, a modelling of the heat distribution in tissues (in silico studies) is crucial. Rubia-Rodriguez et al. have explored collateral heating effects on prostheses that can affect the safety and efficiency of magnetic hyperthermia treatments of localized tumors (Rubia-Rodriguez et al., 2021b).
Luengo et al. enhanced the antibacterial properties of silver NPs in combination with iron oxide and its magnetic hyperthermia properties. The authors showed how the introduction of silver in the iron oxide particles had bactericidal activity against Staphylococcus aureus and Escherichia coli, and in addition, how the external magnetic fields enhanced this activity, demonstrating the synergistic properties of both materials when used in the same composite (Luengo et al., 2020).
In a recent study, MNPs were coated by a sonochemical method with a mesoporous silica surface in which the drug, DOX, could be loaded. The release of the drug used was dependent on pH, which showed effectiveness at acidic pH, proving the ultrasound synthesis as successful (Fuentes-García et al., 2021). In another strategy for DOX delivery, Lazaro-Carrillo et al. engineered a release mechanism based on iron oxide MNPs controlled by pH. The reductive environment of the cell was critical to diminish the side effects of the chemotherapy, increasing the effect against cancer cells (Lazaro-Carrillo et al., 2020).
Trabulo et al. developed a nanoformulation of MNPs with gemcitabine (chemotherapeutic drug) and anti-CD47 (adjuvant). The anti-CD47 antibody formulation showed efficient induction of apoptosis in cancer cells compared to free antibodies. In addition, the NPs were covered with BSA and polyethylene glycol (PEG) avoiding their rapid clearance and leading to a better efficacy (Trabulo et al., 2017).
MNPs can also be employed as smart delivery system for miRNAs. Lafuente-Gómez et al. developed maghemite core NPs loaded with immunomodulatory miRNA that induced a pro-inflammatory response in macrophages due to their load and specific coating (Lafuente-Gomez et al., 2022).
A promising strategy against cancer delivered by NPs is heterogeneous catalysis, which aims to target key chemical species of the tumor and generate in situ harmful biomolecules. Bonet-Aleta et al. engineered copper-iron oxide spinel NPs that effectively reduced glutathione levels and increased ROS and apoptotic pathways in cancer cells (Bonet-Aleta et al., 2022a). Furthermore, they investigated in depth the selective homo- and heterogenous catalytic processes undergoing in the tumor microenvironment, in which the higher glutathione levels are the main driving factor (Bonet-Aleta et al., 2022b). Glutathione is in a much higher concentration inside the cells than on the outside and it is largely responsible of the redox environment of the intracellular medium. This has also been exploited using iron oxide-MnO2 core-satellite shell NPs that undergo a chemical dissolution of the manganese dioxide shell when they are internalized by cells (Garcia-Soriano et al., 2022). This stimuli-responsive behavior changes the MRI contrast mode of the NPs and, at the same time, the iron oxide cores preserve their ability to kill cells through magnetic hyperthermia.
Another interesting property of MNPs is their antiviral activity, de Diego et al. showed that iron oxide NPs impair SARS-CoV-2 infection, highlighting their repurposing value as prophylactic agents against this viral infection (DeDiego et al., 2022).
Finally, the ultimate goal of research in nanomedical development is to reach clinical trials and improve current therapies. In this regard, it is worth highlighting the work done within the European project NoCanTher, where several Spanish institutions were involved. The consortium has been able to test the magnetic hyperthermia approach at Vall d’Hebron hospital (Barcelona) for the treatment of locally advanced pancreatic cancer. These types of studies are essential to make nanomaterial-based treatments a reality in the near future (Nanoscience, 2020).
Gold NPs (AuNPs) are especially relevant due to their ease of preparation, surface reactivity and unique optical properties (Garcia, 2011), (Goesmann and Feldmann, 2010; Wolfram and Ferrari, 2019). The small size of AuNPs, their biocompatibility, low toxicity and the possibility of simultaneous assembly of different molecular functionalities are attractive for biomedical use in therapy and sensing (Giner-Casares et al., 2016), (Saha et al., 2012), (Fabrizio et al., 2016), (Amendola et al., 2017), (Sperling et al., 2008), (Soenen et al., 2015). They are excellent candidates for PTT, biological imaging and optical sensing applications based on the localized surface plasmon resonance (LSPR) phenomenon, in terms of intrinsic properties as well as loading of different molecules, and they can also serve as contrast agents in computed tomography. Here we give an overview of the field given some examples of different AuNPs types but focusing on spherical colloids (Table 3).
The group of Liz-Marzan is a recognized reference in the synthesis of gold-based nanomaterials for multiple biomedical applications, including sensing, photothermia, and preparation of 3D scaffolds (Garcia-Lojo et al., 2019). For instance, they systematically investigated the synthesis of gold-branched nanostructures, such as nanostars with interesting optical properties related to LSPR and surface-enhanced spectroscopies, as excellent candidates for biomedical purposes (Barbosa et al., 2010). They are considered state-of-the-art NPs to be used as efficient agents for photothermal treatment at the NIR range employed as a single modality or combination with other therapeutic functionalities (Quintanilla et al., 2019), (Espinosa et al., 2016), (Villaverde et al., 2018).
In order to enhance the cellular adherence of AuNPs, some strategies have been explored. For instance Artiga et al. encapsulated AuNPs inside a mucoadhesive chitosan hydrogel using polyoxometalates and phosphotungstic acid, showing that these containers can adhere to the cytoplasmic membrane of cells, enabling the thermoablating effect of the AuNPs without the need of cellular internalization (Artiga et al., 2018) (Figure 4). Gonzalez-Pastor et al. explored an interesting modification of Adenoviral vectors (Ad) for improving their uptake in resistant cells and their biodistribution. The authors proposed a strategy based on the modification of the Ad surface with 14 nm PEGylated AuNPs with quaternary ammonium groups and arginine-glycine-aspartic acid peptide motifs (or RGD-motif (Alipour et al., 2020)) to promote the attachment to cells via alternative cellular surface receptors, helped by the increase in positive charges. Modified vectors were tested in cellular models and in mice demonstrating their biocompatibility, high transduction efficiency, and antitumor activity (Gonzalez-Pastor et al., 2021).
FIGURE 4. Synthesis of AuNR@CS hydrogel. Reprinted from Artiga et al. (2018)and licensed under the Creative Commons Attribution.
As highlighted in MNPs, the shape of AuNPs also affects their biological behavior and therapeutic properties, and therefore a controlled synthesis with optimized purification methodologies is critical to obtain homogeneous NPs. Ramírez-Martínez et al. developed an efficient method to synthesize gold nanoprisms that showed reduced non-specific interactions with cells (Ramirez-Jimenez et al., 2020).
Enzyme prodrug therapy consists of selectively delivering an enzyme that activates a nontoxic prodrug into an active agent. Vivo-Llorca et al. functionalized the NPs with horseradish peroxidase, able to oxidize indole-3-acetic acid into toxic agents, and showed that this strategy presented high activity in 3D tumor models in which the three components on their own exhibit no therapeutic action (Vivo-Llorca et al., 2022).
Garcia-Garrido et al. studied drug delivery systems based on gold NPs tailored with low molecular weight polymers branched polyethylenimine and PEG. The system was able to deliver Gapmers targeting p53, reducing the chemoresistance to gemcitabine in mutant p53 cancer cells (Garcia-Garrido et al., 2021).
As is the case with every NP, the protein corona formed around NPs in biological media, modulates several key properties, including cellular internalization or clearance. Therefore, it is essential to study its formation, stability, and composition to understand NPs dynamics in cell and animal experiments. In this regard, Barbero et al. have studied the impact of common cell culture media elements in the formation of protein corona, and the mechanisms behind cellular penetration (Barbero et al., 2019), (Barbero et al., 2017), (Barbero et al., 2022). In this same subject, Mosquera et al. developed a strategy to control protein corona formation. The authors used AuNPs covered by an anionic dye (pyranine), which disrupts protein binding when a positively charged macromolecular cage is present. Zwitterionic surface ligands containing positive and negative charges will favor the formation of a protective hydration layer around the NPs. The authors demonstrate the reversibility of the system, which allows the control of corona formation through external additives. Applying this strategy the authors also increased (30-fold) the cellular uptake due to a synergistic effect between corona suppression and the charge switch from negative to positive at the NP surface. Finally, they explored its use in PTT, exploiting the conditional and enhanced cellular uptake of the system, this time using gold nanorods, with promising results (Mosquera et al., 2020).
Given the advantages of hybrid NPs, Encinas-Basurto et al. used DOX with gold NPs for PTT using human serum albumin NPs. When HeLa cells were treated with HSA-AuNR-DOX NPs, the cell viability was lower than the nanoplatform without DOX decreasing even further when the cells were irradiated (Encinas-Basurto et al., 2018a). Villar-Alvarez et al. also developed an hybrid nanocarrier based on human serum albumin/chitosan NPs that encapsulated free docetaxel and DOX-modified gold nanorods (DOX-GNRs) aiming to combine the chemotherapeutic properties of docetaxel and DOX with the plasmonic optical properties of GNRs for plasmonic-based PTT. This nanoformulation produced high cytotoxicity in breast cancer cells, and PTT enhances the cytostatic efficacy, with apoptosis being the main activated pathway (Villar-Alvarez et al., 2019). In a similar approach, Paris et al. engineered gold nanorods coated with a mesoporous silica shell to deliver two antivascular drugs with different mechanisms of action. The NPs also released heat and ROS through photothermal and PDT upon NIR light irradiation yielding remarkable results in a chicken embryo xenograft model (Paris et al., 2020).
In order to combine therapeutic plasmonic hyperthermia and DOX chemoaction, nanotransporters consisting of gold nanorods coated with poly(sodium-4-styrenesulfonate) (PSS)/DOX/hydrolyzed polylysine (PLL)/hyaluronic acid and (PSS/DOX/PLL)2/hyaluronic acid were developed by Villar-Alvarez et al. Hyaluronic acid targets CD44 receptors, which are overexpressed in some cancers. PTT induced cell necrosis, and apoptosis was promoted by DOX, resulting in a significant synergistic effect provided by a nano-based platform of targeted and multimodal controlled delivery (Villar-Alvarez et al., 2018). Astorga-Gamaza et al. also developed the synthesis of multivalent bispecific AuNPs to enhance the immune response towards HIV-expressing cells. They developed a cooperative adsorption methodology that allows the production of NPs with a 50:50 conjugation with two different antibodies that recognize the HIV gp120 protein and the CD16 receptor of natural killer cells. They performed a thorough characterization of the particles, which were able to promote specific cell-to-cell contact and induce a potent cytotoxic response (Astorga-Gamaza et al., 2021).
Martin-Saavedra et al. designed an hydrogel to incorporate AuNPs and thermosensitivie liposomes loaded with DOX. Upon NIR irradiation, the temperature rose locally releasing active DOX, whose delivery was dependent on the hydrogel composition and irradiation characteristics. Finally, the authors refined the system by incorporating copper sulfide NPs to create an easily biodegradable composite (Martin-Saavedra et al., 2017).
With the aim of controlling and monitoring the temperature of NPs reached during photothermal procedures in the tumor environment and, therefore, minimizing collateral effects during thermal treatments, plasmonic-mediated intracellular hyperthermia generated by Au nanomaterials has been tracked by nanothermometry methods. For instance, Rocha et al. have used Nd-doped Infrared-emitting NPs to monitor the light-to-heat conversion of Au nanorods during PTT (Rocha et al., 2016). Quintanilla et al. designed a hybrid probe for simultaneous plasmonic heating and NIR nanothermometry in glioma cells (Quintanilla et al., 2019). Finally, X-rays were also used to probe the local temperature of photoexcitated Au-based nanomaterials under NIR light for PTT, revealing significant nanothermal gradients (Espinosa et al., 2021).
Guasch et al., also investigated adoptive T cell therapy as a treatment for cancer, in an attempt to overcome the challenge of activating and expanding primary human T cells in vitro. They performed a method for activating primary human CD4+ T cells in vitro functionalizing nanostructured surfaces. These surfaces consist of covalently functionalized RGD on rigid TiO2 surfaces decorated with arrays of AuNPs cell-linked to the stimulating antibody anti-CD3. They demonstrated that the combination of prestimulatory steps, nanostructure surfaces, and costimulatory compounds has an effect on the activation and proliferation of cells (Guasch et al., 2018).
Multidrug resistance is one of the problems of chemotherapy that reduces the efficacy of treatment, and nanocarriers can be used to enhance permeability and retention effect at the target site. Latorre et al. selected two chemotherapeutic drugs, DOX and the camptothecin analogue (CPT) SN38, for the functionalization of albumin-stabilized gold nanoclusters (AuNCs) using tailored linkers. The drugs were released when exposed to different stimuli, such as glutathione and acid pH, leading to a potent antitumor activity. Furthermore, this system showed antineoplastic activity against cancer stem cells (Latorre et al., 2019).
Recently, there has been wide interest in the nanomaterials community to synthesize NPs in a sustainable manner, reducing the use of toxic chemicals and solvents. In this regard, Gomez-Graña et al. explored the possibility of synthesizing gold and silver NPs using a lipopeptide biosurfactant extracted from corn steep liquor. The silver NPs showed antimicrobial properties against Escherichia coli that was greater than similar citrate-stabilized NPs, enhancing the application of sustainable methodologies in NP synthesis (Gomez-Grana et al., 2017). Gonzalez-Ballesteros et al. also synthesized gold and silver NPs in bionanofactories, aiming at developing environmentally friendly processes for NPs synthesis. They characterized the NPs obtained and demonstrated that the particles showed antiproliferative properties and could also serve as immunostimulant agents (Gonzalez-Ballesteros et al., 2021a). In another related work, Gonzalez-Ballesteros and colleagues performed a green synthesis of AuNPs that were decorated with carrageenan extracted from red seaweed. The NPs showed relevant antioxidant and antitumoral properties, highlighting the beneficial effect of NP loading of the active compound (Gonzalez-Ballesteros et al., 2021b).
Regarding delivery, AuNPs have demonstrated to be effective carriers for a wide variety of oligonucleotides. For example, siRNAs are interesting molecules capable of modulating gene expression. One of the critical factors for this strategy to be effective is choosing an optimal strategy to link the siRNA to the AuNPs, and several chemistries have been developed to form stable complexes with proven activity in cellular and animal models (Tortiglione and de la Fuente, 2019). Sánchez-Arribas et al. developed a strategy combining plasmonic gold nanostars and gemini cationic lipoplexes to release plasmid DNA upon irradiation (Sanchez-Arribas et al., 2021). Interestingly, this strategy exploits the release of a DNA plasmid “on demand” upon external stimuli.
Similarly, Milan-Rois et al., developed a strategy for the delivery of four miRNA downregulated in uveal melanoma, and other cancers, in combination with SN38, a topoisomerase I inhibitor. The study showed a synergistic effect between the four oligonucleotides and chemotherapeutic drug conjugated to the AuNPs (Milan Rois et al., 2018). In the same pathology, Ahijado-Guzman et al. explored the potential of gold nanostars as efficient plasmonic PTT using a non-harmful laser irradiation (Ahijado-Guzman et al., 2020). Their results show how these cancer cells can release and uptake the NPs achieving effective PTT even in non-preloaded cells.
Dosil et al. have demonstrated how AuNP-based delivery of specific NK-extracellular vesicles-miRNAs regulates immune responses related to Th1 and recapitulated this phenomenon in animal models. Th1 cells directly killed tumor cells via the release of cytokines that activate death receptors on the tumor cell surface, representing a potential immunomodulatory strategy against diseases (Dosil et al., 2022).
Endosomal escape of the transported cargo is another important feature of NP delivery, to guarantee active functionality in the cytosol. In a recent work, Plaza Ga et al. (Plaza-Ga et al., 2019), explored a mechanism used by the bacterial pathogen Listeria monocytogenes through a toxin called Listeriolysin O. They conjugated this protein to the surface of AuNPs and observed how upon endosomal acidification, the protein disassembles from the NPs to form a pore in the endosomal lipid bilayer enabling the escape of NPs. Within the same focus, and to improve the delivery of proteins, Garcia et al. developed gold nanorods modified with a cell-penetrating peptide that, upon NIR irradiation in the safe second biological window, releases the protein cargo in a controlled spatial and temporal manner (Garcia et al., 2021).
Smart delivery or delivery after external stimuli offers exciting methodologies for delivering cargo into the cytosol on demand. Soprano et al. developed cell-derived NPs that contained gold nanorods in their structure that enabled the release of non-permeant antibodies into the cytosol of cells. The nanocarriers were responsive to NIR irradiation, which proved safe for cells at the conditions needed for cytosolic delivery (Soprano et al., 2020).
Escudero-Duch and collaborators developed a NIR responsive hydrogel based on fibrin, and hollow poly-L-lysine covered gold NPs. The in-situ polymerization of fibrin upon NIR irradiation yields a hydrogel potentially suitable for its use as a scaffold in regenerative medicine. This hydrogel tested in cellular and animal models showed good biocompatibility and allowed the spatial patterning of transgene expression triggered by heat (Escudero-Duch et al., 2019).
Hernández-Montoto et al. prepared Janus gold nanostars MSNs loaded with DOX, and equipped with a cyclodextrin supramolecular gatekeeper. NIR light triggered the release of succinic acid that enabled gate opening and cargo delivery. This strategy enables to use AuNPs as photochemical transducers able to release a chemical messenger upon NIR irradiation. The Janus NPs showed a reduction in cell viability, proving their potential as smart delivery materials (Hernandez Montoto et al., 2019).
In an attempt to improve the available treatments for melanoma, which is highly resistant to cytotoxic agents after metastasis, Villaverde et al. engineered gold nanorods coated with silica and a thermosensitive polymer conjugated to NAPamide, a selective targeting agent for alpha melanocytes. Thus, these NPs exerted a synergistic effect of the cytotoxic DOX and PTT (Villaverde et al., 2018).
Carrillo-Carrión et al. investigated the combination of gold nanostars with metal organic frameworks based on zeolitic imidazole and an amphiphilic polymer to afford thermoresponsive nanocomposites. They demonstrated their stability and the release of cargo inside cells (Carrillo-Carrion et al., 2019).
Zamora-Pérez et al. employed Hyperspectral-Enhanced Dark Field Microscopy (HEDFM) to test the dynamics of Au/CuS NPs directly, based on the changes in the scattering of the nanomaterial in different physiological conditions. Changes in the scattering profiles of NPs could be used as indicators of their performance as photothermal probes. The authors demonstrated how the combination of plasmonic NPs with HEDFM informs of the behavior of intracellular NPs to optimize their functionality for nanomedical applications (Zamora-Perez et al., 2021).
Aguilera-Correa et al. also developed gold nanostars and tested their antimicrobial properties alone and loaded with a potent and widely used antibiotic. The gold nanostars per se did not show antimicrobial activity, however in combination with amikacin inhibited the growth of bacterial biofilm of carbapenem-resistant Klebsiella pneumoniae strain, suggesting that the NPs facilitate the entrance of the therapeutic agent into the biofilm (Aguilera-Correa et al., 2022b).
Finally, Peña-Gonzalez et al. engineered AuNPs and AgNPs with a cationic carbosilane dendrone coating that improved their delivering capabilities, and characterized their interactions with erythrocytes, platelets, and peripheral blood mononuclear cells. The NPs showed to have a safe profile in these systems and proved successful in cell delivery of siRNA against HIV (Pena-Gonzalez et al., 2017).
Similar to gold NPs, silver NPs (AgNPs) have unique and useful properties and are being used in several consumer products such as textiles or home appliances (Ahamed et al., 2010). The antibacterial properties of silver have been known for centuries (McGillicuddy et al., 2017), and colloidal silver has been used by humans for more than 150 years for the treatment of wounds and infections (Reidy et al., 2013). Interestingly, this biological activity is gaining significant interest among researchers due to the resistance developed by different pathogens to current antibiotics. In this regard, some developments carried out by Spanish scientists are mentioned below (Table 4).
Silvan et al. designed AgNPs stabilized with glutathione and evaluated their efficacy against multidrug-resistant Campylobacter strains that were extracted from chicken samples. While the NPs were able to inhibit bacterial growth, the mean minimal inhibitory concentration resulted cytotoxic for three different human intestinal cell lines tested. This effect highlights the importance of further safety experiments to assess the practical potential of AgNPs therapeutic effects (Silvan et al., 2018).
Sabio et al. designed a two-sided material combining AgNPs on one side and living probiotics on the other, with antibacterial capacity against Pseudomonas aeruginosa (Sabio et al., 2021). AgNPs have also been explored for their anti-amoebotic properties against pathogens responsible for keratitis. In this regard, Hendiger et al. evaluated the activity, cytotoxicity, and anti-adhesive properties of AgNPs included in contact lens solutions against the Acanthamoeba castellanii Neff strain (Hendiger et al., 2021), (Hendiger et al., 2020). The presence of the AgNPs showed a significant increase in anti-amoebic activity, without increasing the overall cytotoxicity, decreasing the risk of Acanthamoeba keratitis infection. Padzik et al. also employed AgNPs conjugated with tannic acid as potential agents against Acanthamoeba spp (Padzik et al., 2018).
In the case of peri-implantitis due to biofilm deposits, Pérez-Tanoira et al. immobilized AgNPs in titanium, demonstrating its beneficial effect in reducing the biofilms stablished by S. aureus and by mixed oral bacterial flora (Perez-Tanoira et al., 2022). Other anti-biofilm strategies involve the use of silver-containing nanoscaled Metal Organic Frameworks (MOFs) against S. aureus biofilm. Arenas-Vivo et al. demonstrated the use of AgNPs functionalized with DNase I decreasing the S. aureus biofilm viability more than using the antibiotics alone (Arenas-Vivo et al., 2019). Rubio-Canalejas et al. pointed out that clinical treatment combining antibiofilm enzymes and antibiotics may be essential to eliminating chronic wound infections (Rubio-Canalejas et al., 2022). Finally, González-Fernández et al. demonstrated how silver nanorings are capable of totally inhibiting the germination of A. castellanii cysts (Gonzalez-Fernandez et al., 2022).
In regards to drug delivery, the formation of hybrid Ag2S NPs with poly(lactic-co-glycolic acid) (PLGA) by electrospray allows for the encapsulation of drugs such as maslinic acid (MA). The anticancer drug showed an efficient encapsulation and controlled release in cellular models (Alvear-Jimenez et al., 2022).
Morey et al., modified silver and gold NPs using cysteine to bind raltitrexed to the surface of NPs and tested them in A549 and HTC-116 cells lines. Silver raltitrexed NPs inhibited cancer cell viability (Morey et al., 2021). Other modifications, such as the functionalization of AgNPs with Annona muricata plant antitumoral extracts, have been prepared and tested by González-Pedroza et al. as promising antitumoral nanoformulations (Gonzalez-Pedroza et al., 2021).
Finally, AgNPs can be used as luminescent biofluids capable of acting as photothermal agents and nanothermometers. Mendez-Gonzalez et al. showed how a nanofluid containing AgNPs had improved properties compared to a combination with magnetic nanoflowers and showcases their use for hyperthermia in brain tumors (Mendez-Gonzalez et al., 2022).
Organic NPs have synthetic or natural organic components such as carbohydrates, proteins, peptides, or lipids (Romero et al., 2012). Their biodegradable composition, together with the relatively simple encapsulation of drugs, make them the preferred drug delivery systems. The current advances in the use of protein, polysaccharide, polymeric and lipid NPs carried out in Spanish institutions are discussed below, and summarized in tables at the end of each section.
Protein NPs are of great interest in nanomedicine due to the intrinsic properties of proteins, such as their biocompatibility and biodegradability. Proteins possess different functional groups located in the side chains of amino acids that can be exploited for chemical conjugation. They also contain hydrophilic and hydrophobic regions that can be used to interact with hydrosoluble or insoluble compounds. Finally, they have tunable structures that can be obtained by protein engineering, expanding their applications (Table 5).
Several proteins have been used to create NPs for their use in nanomedicine, being albumin one of the most successful ones. It is naturally present in the blood, so it can avoid immunogenic reactions, increasing the circulation time of their cargoes. It is a natural vehicle especially suited for the interaction with hydrophobic molecules. Furthermore, human cells present albumin receptors, namely, gp60 and SPARC (Prajapati and Somoza, 2021). As these receptors are overexpressed in cancer cells, albumin NPs can be used to target tumor sites. This strategy has been successfully used in the formulation of Abraxane, which has been approved for the treatment of several tumors (Trabulo et al., 2017). Albumin is a monomeric protein unable to form NPs by itself, therefore several methods have been used to form albumin nanostructures, being desolvation the most common one. For example, Prajapati et al. used this method to encapsulate DOX, a drug limited by its toxicity. Its encapsulation inside of NPs enabled to target tumor cells specifically, increased the drug efficacy, and decreased its toxicity. Furthermore, they stabilized the NPs employing several cross-linkers, showing that a redox-dependent crosslinker (SPDP, N-succinimidyl 3-(2-pyridyldithio) propionate) increased drug release in cancer cells, due to their enhanced redox environment compared to non-cancer cells. Their nanoformulation showed toxicity in breast cancer cells and a negligible effect in non-tumoral cells, presenting a potential use for the treatment of breast cancer (Prajapati et al., 2021) (Figure 5). The same group also used albumin-based nanostructures for uveal melanoma treatment. In another article, they studied the use of albumin to deliver AZD8055, a potent inhibitor of the mTOR pathway that is overexpressed in the pathology and is critical in tumorgenesis. They produced gold nanoclusters stabilized by albumin, while the drug was conjugated externally using disulphide bonds. The lately thiol-dependent conjugation of the drug allowed its specific release in the cytoplasm of cancer cells. The authors showed that their nanostructures had anti-tumoral activity in mice models, using a dose 23-fold lower than previously reported (Latorre et al., 2021). The conjugation of folate to NPs based on BSA and alginate as an active targeting strategy for the delivery of PTX was showed by Martinez-Relimpio et al. which resulted in an increased uptake of the NPs by cancer cell lines, as there is an overexpression of folate receptors (Martinez-Relimpio et al., 2021). To exploit BSA NPs delivery possibilities Gerke et al. developed a simple methodology with clickable anti-PD-L1 antibodies, showcasing the versatility of this bioorthogonal design (Gerke et al., 2022).
FIGURE 5. Albumin NPs synthesis. Reprinted from Prajapati et al. (2021) and licensed under the Creative Commons Attribution.
Hydrophilic proteins with multiple arginine residues, such as protamine and polyarginine, are widely used to form NPs as their positive charge confers membrane-translocation properties (Thwala et al., 2018). Similarly to albumin, it does not form NPs by itself, so a solvent displacement method must be used to create protamine nanocapsules. NPs contain an oily core and a protamine coating. Thwala et al. used them for the delivery of insulin, a highly used protein that cannot be administered orally. The authors used a protamine/PSA shell that controls the release of insulin from the moment the NPs are administered orally until reaching the intestine (Thwala et al., 2018). Furthermore, the insulin transport across mucus layers can be increased by incorporating in the formulation penetration enhancers such as oleic acid (Niu et al., 2017). The same group used protamine nanocapsules loaded with antigens as an alternative to vaccine adjuvants. This system can load multiple antigens, be lyophilized, and trigger the immune response. These properties have been shown in particles loaded with influenza hemagglutinin antigen, and particles containing hepatitis B virus surface antigen (Gonzalez-Aramundiz et al., 2017; Gonzalez-Aramundiz et al., 2018). According to the results, the protamine nanocapsules showed the ability to enter macrophages without toxicity and produced an important immune response against influenza (Gonzalez-Aramundiz et al., 2017).
Zein is a small hydrophobic protein that, in contrast to albumin and protamine, can easily self-assemble, forming colloidal NPs in the aqueous phase. Zein NPs coated with PEG and Gantrez AN-thiamine have been used to deliver insulin. They have an enhanced permeation within the mucus and intestinal absorption, which decreases the glucose level in blood (Inchaurraga et al., 2020; Reboredo et al., 2021). In particular, the Gantrez conjugate reduced the accumulated fat in Caenorhabditis elegans (Martinez-Lopez et al., 2021). Given this promising results a double blind clinical trial has been designed by Clínica Universidad de Navarra to determine whether this particles are able to provide glycemic control in patients (NT: 05560412) (Clinica Universidad de Navarra UdN, 2022b).
Another interesting kind of proteins are the ones considered self-delivered nanoscale drugs that, at the same time, can self-assemble in NPs. With this in mind, Sanchez-García et al. fused bacterial toxin peptides to a N-terminal cationic T22 peptide and a C-terminal region with 6 histidines. These engineered peptides self-assemble in nanostructures by the interaction of the N- and C-terminal regions. T22 peptide can recognize CXCR4, a receptor overexpressed in cancer cells, providing specific tumor targeting, while the toxin peptide promotes general cell death (Sanchez-Garcia et al., 2018). The bacterial toxins can be exchanged for human pro-apoptotic peptides with similar results (Sanchez-Garcia et al., 2020). These nanostructures were successfully applied to several cancer models in mice, such as colon, (Sanchez-Garcia et al., 2018), lymphoma, (Falgas et al., 2020), and leukemia (Pallares et al., 2021). Volta-Duran et al. (Volta-Duran et al., 2021) designed a method to deliver anticancer drug pairs that consist of a tumor-targeted protein NP based on two microbial toxins, exotoxin A and diphtheria toxin, chemically coupled with oligo-floxuridine and monomethyl auristatin E respectively. These nanoformulations were able to internalize into target cells and had a biological impact. Unfortunately, the chemical conjugation annulled the activity of the toxins. Pallares et al. synthesized a nanoconjugate that contained GFP instead of a bacterial toxin, covalently labelled with auristatin, a potent antimitotic agent. With this treatment, they were able to significantly reduce and control myeloid leukemia dissemination (Pallares et al., 2020).
Polysaccharides are biomacromolecules formed by sugars found in every living organism. They are non-toxic or immunogenic and are a better biocompatible alternative to synthetic polymers. In addition, they are highly versatile, varying in molecular weight, branch degree, and functional groups, and can be tuned to deliver different cargo (Serrano-Sevilla et al., 2019; Shokrani et al., 2022). In Spain, many polysaccharides have been used to form nanostructures with biomedical applications, such as chitosan, hyaluronic acid or cellulose as reviewed here (Table 6).
Chitosan is a derivative of the natural polysaccharide chitin, which is the second most abundant polysaccharide in the world after cellulose. Chitosan has many interesting properties, including biocompatibility, biodegradability, antibacterial effect, and muco-adhesion, and it is widely used in food, cosmetics, and biomedical applications. Chitosan has a high concentration of reactive amino groups along its backbone, conferring a high positive charge that promotes its interaction with biological tissues (Frigaard et al., 2022). The excellent properties of chitosan NPs for intracellular delivery have been exploited by Ambrossone et al. They showed that oily core chitosan nanocapsules synthesized by nanoemulsion efficiently delivered alsterpaullone, a Wnt signaling agonist, into the model organism Hydra vulgaris. They also studied the characteristics of the intracellular delivery with Nile red-loaded NPs. Their methodology resulted in a more efficient manner of activating Wnt pathway than free alsterpaullone at the same concentration (Ambrosone et al., 2020). Similarly, Montero et al. developed chitosan-BSA NPs and studied their potential as vehicles with different combinations, demonstrating their potential for drug delivery (Montero et al., 2019).
Chitosan NPs also enable the transportation of agents into the brain, given the difficulty to target this organ. As a proof of concept, Casadomé-Perales et al. demonstrated the inhibition of p38 MAPK, an enzyme that is commonly dysregulated in several neurodegenerative diseases. They encapsulated PH797804, an inhibitor of this enzyme, in NPs with a nanoemulsion core. The intranasal delivery of the NPs enabled the inhibition in different parts of the brain in animal models, showing that it could be an efficient strategy for brain delivery (Casadome-Perales et al., 2019).
Marciello et al. used chitosan NPs to deliver a model peptide through vaginal mucosa, given the interest of microbicides delivery for the prevention and treatment of sexually transmitted diseases. The NPs contained chitosan and ascorbate, with insulin as the model cargo. Then, the NPs were incorporated in sponge-like cylinders made of mannitol, sucrose, and gelatin B to control their release in the vaginal environment (Marciello et al., 2017).
The fact that chitosan NPs can encapsulate peptides opens the possibility of their use as nanovaccines. As mentioned previously, using NPs as vaccines improves both the administration and the activation of the targeted immune cells, which results in greater efficacy. The role of chitosan in these formulations has shown to enhance the activation of the adaptive immune response (Moran et al., 2018).
The generation of an effective HIV vaccine is still an important health challenge, to which several nanotechnological approaches are being developed. According to previous works, the peptide sequences around the protease cleavage sites have been proposed as a target for HIV vaccines. Dacoba et al. developed different chitosan NPs loaded with the HIV peptide PCS5. The NPs were formed by ionic complexation, mixing the positive polysaccharide chitosan with negative ones like Hyaluronic acid or dextran. Furthermore, they tested if the presence of poly (I:C), an immunomodulatory molecule, had any effect. All the NPs were able to induce humoral responses against the antigen (Dacoba et al., 2019). However, they showed that the binding of the antigen, the presence of poly (I:C), and the nature of the polysaccharides influence the type of immune response, such as the kinetics of the effector T cell responses. The results suggest the possibility of developing a nanovaccine against HIV and its translation into clinical trials (Dacoba et al., 2020a). The same group showed that NPs made of chitosan and the anionic carboxymethyl-β-glucan, which accumulate in the lymph nodes, promoted the accumulation of the NPs in draining lymph nodes and exerted an immune response. The NPs were formed using the ionic complexation method, and loaded with ovalbumin (Frigaard et al., 2022).
Polysaccharide NPs loaded with antigens can also be used to induce tolerance for allergens. Rodriguez et al. explored the capabilities of several mannose nanostructures to serve as an efficient platform to generate specific recognition without the need for additional adjuvants. In this particular case the treatment developed prolonged protection against allergen exposure without any sign of anaphylaxis (Rodriguez et al., 2019).
Hyaluronic acid is an anionic polysaccharide, a glycosaminoglycan, consisting of disaccharide repeating units of β-1,4-D-glucuronic acid-β-1,3-N-acetyl-D-glucosamine. It has a high binding affinity towards the CD44 receptor, highly expressed in cancer cells (Liu and Huang, 2022). It can be used as shell in oil-based NPs, by the solvent displacement technique. Teijeiro-Valino et al. used this kind of NPs for the encapsulation of the anti-cancer drug docetaxel. Furthermore, they decorated the hyaluronic acid shell with the tumor homing peptide tLyp1. Their formulation increased penetration in the tumor and anti-cancer activity in lung and pancreatic cancer mice models (Teijeiro-Valino et al., 2019).
Cadete et al. used a modification of hyaluronic acid consisting of the addition of hydrophobic side chains, like dodecyl, to promote the self-assembly of NPs without the use of surfactants. This strategy decreased the cytotoxicity of the NPs, while showing an improved intracellular drug delivery (Cadete et al., 2019).
Delivery of drugs on the eye surface can be achieved by flexible electrospun nanofibers, which are able to adapt and persist on the eye surface whilst the drug is released. Grimaudo et al. overcame the incapability of hyaluronic acid to be electrospunned, by using PVP as an excipient. This resulted in creating hyaluronan nanofibers, capable of delivering the antioxidant ferulic acid and the antimicrobial peptide ε-polylysine at the same time (Grimaudo et al., 2020).
In another study, Storozhylova et al. engineered hydrogels formed by Hyaluronic acid and fibrin, with hyaluronic acid nanocapsules loaded with anti-inflammatory drugs. They were used to improve intra-articular administration, showing a rapid efflux of the administered drugs. This system could relieve the inflammatory conditions of large joints (Storozhylova et al., 2020).
Qaiser et al. developed three types of nanomicelles formulations to synthesize a targeted, mucoadhesive and mucopenetrating drug delivery system. The goal was to encapsulate clarithromycin, an antibacterial drug, to improve its residence time at the Helicobacter pylori infection site. They concluded that clarithromycin-loaded papain-modified ureido-conjugated thiolated hyaluronic acid-co-oleic acid (CLR-PAP-Ur-thHA-co-OA) nanomicelles could be used as nanocarriers for the treatment of H. pylori infection, due to their mucopenetration, mucoadhesion properties, stability, and extended drug release (Qaiser et al., 2022).
Cellulose is the world’s most abundant polysaccharide. It is a linear polymer composed of repeating units of two anhydroglucose rings. Its abundance and biocompatibility make nanocellulose a good candidate for biomedical applications (Nicu et al., 2021). Recently, Do et al. developed a modified cellulose nanocrystal (CNC) for the delivery of anti-cancer drugs. They engineered nanoplatforms based in modified CNCs with APTES to improve their dispersibility. These CNCs were covalently functionalized with folic acid (FA), followed by the incorporation of Carbon dots and the drug DOX, by electrostatic interaction. These CNCs may be promising nanoplatforms to be used both in chemotherapy and PTT against cancer (Do et al., 2021).
Gallego-Yerga et al. prepared DNA-cyclodextrin NPs to improve gene therapy. They controlled the morphology of the complexes to study how the shape affects the transfection properties. They found several complexes that exhibited highly efficient transgene expression and were able to deliver DNA to the spleen in a tissue-specific manner in animal models (Gallego-Yerga et al., 2018).
Finally, inulin is a fructan that has high structural flexibility and good biodegradability, which allows for its use as a drug delivery system. In a study comparing chitosan with inulin for drug delivery, the small inulin NPs showed less toxicity and a higher accumulation in the lymphatic nodes (Crecente-Campo et al., 2019).
The potential of polymeric NPs relies on their highly versatile structure, which can be altered depending on the therapeutic application, cargo, or type of administration. Moreover, the chemical reactivity of the polymers can be exploited for the controlled release of drugs at different pH or thermal environments. These properties in addition to good biocompatibility, make polymeric NPs great candidates in the biomedicine field (Table 7) (Elsabahy and Wooley, 2012; Sartaj et al., 2021).
Given their versatility, several anti-cancer strategies have been accomplished. Aznar et al. have studied the immunotherapeutic profile of BO-112, a nanoplexed form of Poly I:C coupled to polyethylemine that prevents its degradation from proteases. Poly I:C is a synthetic analog of double stranded RNA that activates innate immune receptors and several formulations with different polymers have been tested in the clinic. BO-112 was locally injected, leading to the death of tumoral cells. Also, this nanoplexed Poly I:C showed an antitumoral activity through the induction of type I Interferon and CD8 T-cell infiltrates in the tumor (Aznar et al., 2019). These promising results motivated the development of two clinical trials of BO-112 in combination with antiPD-1 therapies or with pembrolizumab showing encouraging clinical benefits in cancer patients. Dacoba et al. developed an arginine-based poly (I:C) nanocomplex that induces the accumulation of endosomal toll-like receptor 3 agonists, which affect the polarization of the profile M2 to M1 (pro-inflammatory and antitumoral) in endosomal compartments. This strategy has been explored in cancer, where the polarization to M1 profile of tumor-associated macrophages could be a promising approach against tumors (Dacoba et al., 2020b). Another study was based on the study of intratumoral immunotherapy.
Poly amino acid nanogels are also an interesting strategy in the delivery of cancer treatment. Interestingly, these agents can be developed to release the cargo specifically in the tumor microenvironment as pH responsive particles (Arroyo-Crespo et al., 2018). Duro-Castano et al. engineered polyglutamic acid nanogels loaded with DOX as an effective strategy for the treatment of triple-negative breast cancer metastases, which effectively reduced lung and lymph node metastases (Duro-Castano et al., 2021a). In another strategy, Fernández et al. developed a tert-Ser polyacetal loaded with PTX forming NPs of 10–70 nm, which showed a controlled release of drug dependent on pH. The nanomedicine thus inhibited an early release and reduced primary tumors in animal models while also inhibiting metastasis (Fernandez et al., 2022).
Staka et al. developed a low molecular weight hydrogel formed by a nucleoside (N4-octanoyl-2-deoxycytidine). The gel accommodated multiple polymeric NPs loaded with gemcitabine, a chemotherapeutic drug. The gel released the encapsulated drug for a month, which could be used as a treatment for unresectable cancer (Staka et al., 2019).
Myeloid-derived suppressor cells are a target in adoptive T cells transfer. Ledo et al. developed multilayer polymer nanocapsules to co-deliver two drugs: RNAi polynucleotides and chemokine CCL2. These NPs may help modulate the activity of myeloid derived suppressor cells (Ledo et al., 2019).
Etchenausia et al. studied poly(N-vinyl caprolactam) (PVCL)-based thermoresponsive microgels with polymer brushes as potential drug delivery nanocarriers. These microgels were biocompatible on HeLa and RAW cells. They tested DOX-loaded microgels and determined a sustained release of DOX from microgels as well as increased cell viability compared to free DOX, confirming the suitability of these microgels as safe drug delivery nanocarriers (Etchenausia et al., 2019).
Cordeiro et al. designed synthetic and natural polymer NPs and nanocapsules for antigen delivery. They observed that small-size cationic nanoclusters showed high accumulation in the lymph nodes and concluded that by modifying the physicochemical properties and composition of the nanocapsules, modulation of lymphatic uptake and biodistribution would be possible (Cordeiro et al., 2019).
Relano-Rodríguez et al. worked on human cytomegalovirus (HCMV), which infects and replicates in a wide variety of cells. They focused on the study of polyanionic carbosilane dendrimers (PCD). They tested two PCDs, G2-S16 and G2-S24P, which, alone or with current treatments, seemed to be a good tool against HCMV (Relano-Rodriguez et al., 2021) (Figure 6).
FIGURE 6. Dendrimer strategy as antiviral therapy. Reprinted from Relano-Rodriguez et al. (2021) and licensed under the Creative Commons Attribution.
Polymeric nanocapsules are promising carriers for various antigens against different pathogens whose immunogenicity can be improved by including immunostimulatory molecules, improving current vaccines. Imiquimod (IMQ) has been described as a good modulator of innate immunity and activator of the Th1 immune response, which is the target of most vaccines. Previous work showed that encapsulation of IMQ in chitosan (CS) NC induced protective antibody levels against recombinant hepatitis B surface antigen (HB) in mice immunized intranasally. In this work, two different IMQ-loaded NPs with a CS or inulin/pArg polymeric shell were synthesized for the development of a model vaccine containing a recombinant fusion protein (RFP) derived from the ESAT-6 antigen and CFP-10 from Mtb. The vaccine containing INU:pArg:Ag nanocapsules was the most immunogenic prototype against the ECH fusion protein (Diego-Gonzalez et al., 2020). The use of mRNA in vaccination has achieved great success in preventing the acute effects of COVID-19 after SARS-Cov-2 infection. Fornaguera et al. developed and described a protocol for the preparation of poly(β-amino esters) and their conjugation to mRNA and studies in cellular models (Fornaguera et al., 2021).
Poly amino acid particles are also excellent carriers able to cross the BBB when conjugated to brain penetrant peptides, demonstrating interesting properties in treating central nervous system disorders such as Alzheimer’s disease (Duro-Castano et al., 2021b). Octaarginine conjugated with lauric acid forms a hydrophobic complex that can protect labile molecules by electrostatic and hydrophobic interactions, improving miRNA delivery in the hippocampus (Samaridou et al., 2020). Poly-I-glutamic acid complexes are also an efficient and non-toxic strategy to deliver oligonucleotides or DNA. Niño-Pariente et al. developed nanocomplexes with a poly-I-glutamic acid backbone that was derivatized with oligoaminoamide residues to efficiently assemble and deliver plasmid DNA (Nino-Pariente et al., 2017). Using PLGA-based polymeric NPs, Nozal et al., enhanced BBB permeation of NPs loaded with S14, a phosphodiesterase 7 (PDE7) inhibitor with great potential to treat Parkinson’s disease. According to the studies, S14-loaded PLGA-NPs showed improved pharmacokinetic properties of S14 in animal models, as well as enhanced safety of this inhibitor (Nozal et al., 2021). The same group also encapsulated PHA-767491, a potent cell division cycle 7 (CDC7) kinase inhibitor in PLGA-NPs improving its permeability and CNS delivery properties (Rojas-Prats et al., 2021).
Grayston et al. tested biocompatible PLGA nanocapsules functionalized with superparamagnetic iron oxide NPs and Cy7.5 to improve brain targeting of drugs after stroke. According to the results, the intra-arterial route for the cerebral administration of new treatments showed an extraordinary advantage (Grayston et al., 2022).
Another active area of research belongs to anti-inflammatory agents. Interleukin-12 and interleukin-23 have a high interest as therapeutic targets to treat autoimmune/inflammatory diseases and chronic inflammatory diseases where T cells are the primary cells that are dysfunctional. Espinosa-Cano et al. prepared anti-inflammatory polymeric NPs that combine ketoprofen and dexamethasone (14Dx-KT), which are one of the most efficient cyclooxygenase-inhibitors. According to the results, a long-term treatment with 14Dx-KT NPs reduced the expression of Il12b and IL23a to normal cellular levels. The results suggest that the ketoprofen-based systems present an anti-inflammatory activity reducing the basal levels of pro-inflammatory markers and increasing the gene expression of anti-inflammatory cytokines (Espinosa-Cano et al., 2020a). Recent studies demonstrate the anti-inflammatory capacity of dexamethasone and naproxen. However, their use is limited by the rapid clearance of free drug. Espinosa-Cano et al. used polymeric NPs to administer these drugs in order to accumulate in pathological tissue, increasing the drugs’ activity and reducing their adverse effects. The prepared naproxen-containing polymeric NPs loaded with dexamethasone were able to repress Il12b transcript levels, which would be an interesting treatment of autoimmune/anti-inflammatory diseases in which IL12 and IL23 are overexpressed (Espinosa-Cano et al., 2020b).
Oral delivery of insulin is also an attractive application for this type of NPs, since the acidity of intestinal fluids increases the risk of degradation. For instance, coating polyarginine insulin NPs with PEGylated polyaminoacids, protects insulin until it reaches the intestinal mucus (Niu et al., 2018), achieving the highest insulin uptake ever recorded in cellular models. Despite not resulting in a significantly increased systemic insulin uptake, the system showed a great potential for the delivery of peptides through the intestine mucosa. Santalices et al. also aimed at orally delivering hydrophobically modified insulin. They developed and extensively characterized nanoemulsions with selected components with improved properties on permeation, stability and mucodiffusion, such as miglyol, PEGylated phospholipids and poloxamer. The nanocomplexes showed promising results in cellular models and a moderate but significant hypoglycemic response in animals, highlighting key steps to take into consideration to overcome intestinal barriers (Santalices et al., 2021).
Similarly, Ariza-Saenz et al. used polymeric NPs of PLGA particles coated with glycol-chitosan (Ariza-Saenz et al., 2017) to enhance the delivery of a peptide inhibitor of the HIV-1 fusion protein that demonstrated to have enhanced permeability and efficacy of the peptide alone (Ariza-Saenz et al., 2018; Sanchez-Lopez et al., 2021).
As already showcased, polymeric NPs have been widely studied for the delivery of active compounds with poor pharmacokinetic properties. Here the delivery of licochalcone A was investigated using PLGA NPs and cell-penetrating peptides Tet and B6. Galindo et al. showed how the nanoconjugates with B6 showed increased activity for the treatment of ocular inflammation (Galindo et al., 2022).
Since combining drugs with miRNAs within a nanocarrier is a promising treatment for atherosclerosis, Leal et al., have developed polymeric PLGA NPs that simultaneously encapsulate and deliver miRNA-124a and the statin atorvastatin (ATOR). This combination reduced levels of proinflammatory cytokines and ROS. In addition, the dual-loaded NPs proved to be non-toxic to cells and prevent the accumulation of low-density lipoproteins inside macrophages and morphological changes, showing promise as a treatment for these types of diseases (Leal et al., 2022).
Encinas-Basurto et al., have developed allyl-isothiocyanate (AITC)-loaded PLGA NPs that target epithelial carcinoma cells due to anti-EGFR antibody binding to the surface. These NPs showed better anti-cancer properties compared to the free drug, suggesting that receptor-ligand binding could be used to target the NPs to tumor cells for improved drug delivery (Encinas-Basurto et al., 2018b).
In another attempt to generate smart-delivery NPs, Correa-Paz et al. engineered polymeric templated CaCO3 NPs with a layer-by-layer structure. The NPs were loaded with a fragile thrombolytic serine protease, labelled fluorescently and tagged with iron oxide NPs. The particles proved to efficiently release their protease cargo upon ultrasound application in cellular and animal models and maintained the activity after its delivery (Correa-Paz et al., 2019).
In another example of smart drug delivery using nanomaterials, Xuan et al. developed nanobottles formed from polyethylene glycol and ribose with a soft-template-based polymerization methodology. Using NIR light, the inner fluid of the bottle can be heated, resulting in its propulsion. The authors studied the trajectories, velocity and explosion events of the motors that could be controlled by modulating the NIR source and analyzed them through finite element analysis. This work is paving the road for the discovery and synthesis of new fuel-free based nanomotors (Xuan et al., 2018).
Lipid NPs (LNPs) range from liposomes to solid LNPs (SLNs) and quatsomes (QS). The potential of these formulations relies on their stable structure and ability to cross biological barriers. LNPs constitute a good vehicle to transport both hydrophobic and hydrophilic drugs, providing protection for its cargo. For example, the complexation of lipids with nucleic acids allows their transport and prevents their degradation. They can be divided into four major types: liposomes, niosomes, SLNs, and nanostructured lipid carriers (NLCs) (Dhiman et al., 2021). SLNs and NLCs overcome liposomes and niosome due to their better performance under pH- and enzyme-dependent degradation. All of these formulations have been of research interest in Spain (Table 8) (Vargas-Nadal et al., 2020), (Tenchov et al., 2021)
LNPs are vastly used to treat cancer. Mitxelena-Iribarren et al. tested the effectiveness of nanoencapsulated methotrexate against human bone osteosarcoma cells U2OS using microfluidic platforms that allow cell culture and incubation under highly controlled dynamic conditions (Mitxelena-Iribarren et al., 2017; Mitxelena-Iribarren et al., 2021). Cacicedo et al. combined bacterial cellulose hydrogel (BC) and NLC, including cationic or neutral DOX as a drug model and tested in human breast adenocarcinoma MDA-MB-231 cells and orthotopic breast cancer mouse model. These carriers showed a significant reduction in tumor growth, metastasis incidence and local drug toxicities (Cacicedo et al., 2018). González-Fernández et al. tested the oral administration of edelfosine encapsulated SLNs in osteosarcoma cancer cell lines and animal models. They found that oral administration had a better effect against primary osteosarcoma tumors and successfully prevented the metastatic spread of cancer cells from the primary tumor to the lungs. In addition, Lasa-Saracíbar et al. labelled these SLNs with Tc and studied their biodistribution in mice after intraperitoneal and intravenous administration. Results showed that the drug could reach circulation and provide a more constant blood concentration after intraperitoneal administration (Gonzalez-Fernandez et al., 2018; Lasa-Saracibar et al., 2022) (Figure 7). Aguilera-Garrido et al. tested the delivery maslinic acid using SLNs with three different shell compositions: Poloxamer 407 (PMA), dicarboxylic acid-Poloxamer 407 (PCMA), and Hyaluronic acid-coated PCMA (PCMA-HA) in Caco-2/HT29-MTX co-cultures. Interestingly, they found that the SLNPs improved the solubility of MA up to 7.5 mg/mL, stable in a wide range of pH, and increased the bioaccessibility of MA after gastrointestinal digestion in a cellular model (Aguilera-Garrido et al., 2022). Arana et al. studied how different amounts of phosphatidylethanolamine polyethylene glycol (PE⁻PEG) influence SLNs composed of stearic acid, Epikuron 200, and sodium taurodeoxycholate. They observed that the presence of PE⁻PEG improved active cell internalization of the NPs in an oral adenocarcinoma cell line, reducing non-specific internalization mechanisms. Furthermore, they also tested the effect of surface coating on the efficiency of incorporated drugs finding that PE⁻PEG coated SLN increases its chemotoxic effect compared to non-coated SL (Arana et al., 2019). Gundogdu et al. rigorously studied the physical characteristics of imatinib-containing NLC for the treatment of gastrointestinal stroma tumors. They found that these NPs revealed a Korsmeyer-Peppas drug release model of 53% at 8 h with above 90% of cell viability. They also found an IC50 of 23.61 μM and induction of apoptosis in CRL-1739 cell lines (Gundogdu et al., 2022). Leiva et al. tested glyceryl tripalmitate NLCs loaded with PTX. The NPs-PTX significantly enhanced PTX antitumor activity in human breast (MCF7, MDAMB231, SKBR3, and T47D) and lung (A549, NCI-H520, and NCI-H460) cancer cells. They also decreased the volume of breast and lung multicellular tumor spheroids (Leiva et al., 2017).
FIGURE 7. Distribution of LNPs loaded with edelfosine. Reprinted from Lasa-Saracibar et al. (2022), and licensed under the CReative Commons Attribution.
To induce apoptosis in cancer cells, Gallego-Leyda et al. employed SLNs decorated with TNF-related apoptosis-inducing ligand (TRAIL). They found that the decorated NPs were more cytotoxic than soluble TRAIL in A673 cells, RD cells and HT-1080 cells (Gallego-Lleyda et al., 2018). Similarly, Solar-Agesta et al. developed LNPs whose surfaces can bind granulysin as an antitumoral treatment, increasing the concentration at the site of contact with the target cell. Granulysin binding to the liposomes significantly increased the cytotoxic potency, produced mainly by apoptosis (Soler-Agesta et al., 2022).
NLC can overcome the toxicity of some antibiotics with a current limited use. Vairo et al. encapsulated antibiotics in different NLC formulations through high pressure homogenization, and showed that negatively charged SCM-NLC, with trehalose as cryoprotectant, had the best efficacy in several bacteria strains (Vairo et al., 2020). With a similar aim, Vinuesa et al. explored different lipid nanoformulation options for benznidazole, a commercially available drug currently used for the treatment of Chagas disease with a high toxicity, finding balanced conjugates of activity/toxicity (Vinuesa et al., 2017).
LNPs are also used to destroy biofilms. Sans-Serramitjana et al. studied the viability of P. aeruginosa biofilms treated with both free and nanoencapsulated colistin finding a more rapid killing of P. aeruginosa bacterial biofilms by nanostructure lipid carrier-colistin than by free colistin (Sans-Serramitjana et al., 2017a). The same group investigated the encapsulation of Tobramycin and its effect on planktonic and biofilm forms of Pseudomonas. They found that the nanoencapsulation of tobramycin did not improve its efficacy against planktonic P. aeruginosa but did improve its ability to eradicate P. aeruginosa biofilms (Sans-Serramitjana et al., 2017b).
Machine learning algorithms can be used to unravel hidden patterns in NP. In this work by Basso et al., the effects of two novel glycerol-based lipids, GLY1 and GLY2, on the architecture and performance of NLC were evaluated. Results showed that GLY1 circumvents the intrinsic cytotoxicity, is effective at increasing glioblastoma uptake, and exhibits encouraging anticancer activity (Basso et al., 2021).
Diselenide loaded in NLCs were developed by Etxebeste-Mitxeltorena et al. to treat visceral leishmaniasis. In this work, Diselenide (2m), a trypanothione reductase inhibitor, was loaded in glyceryl palmitostearate and diethylene glycol monoethyl ether-based NLCs. They found that diselenide 2m-NLCs drastically enhanced its intestinal permeability and provided plasmatic levels higher than its effective concentration (IC50). In Leishmania infantum-infected BALB/c mice, 2m-NLC reduced the parasite burden in the spleen, liver, and bone marrow by at least 95% after 5 doses (Etxebeste-Mitxeltorena et al., 2021).
SLNs can also be included in microspheres of appropriate size through a spray-drying technique. Gaspar et al. encapsulated SLNs containing rifabutin (pulmonary antibiotic) and tested their antimycobacterial activity in a murine model of infection with Mycobacterium tuberculosis (Gaspar et al., 2017). Moreover, tuberculosis was also treated using Bedaquiline encapsulation in SLNs and chitosan nanocapsules in a work performed by Matteis et al. The authors encapsulated this drug and found no cytotoxicity at the concentration needed to kill bacteria, (De Matteis et al., 2018), highlighting the great potential of this approach (Baranyai et al., 2021).
Another interesting application of SLNs was developed by Cervantes et al. to protect auditory cells from cisplatin-induced ototoxicity. SLNs were loaded with glucocorticoids (dexamethasone and hydrocortisone) and they were efficiently incorporated by auditory HEI-OC1. Their results showed that the encapsulation in SLNs increased the protective effect of low-doses of hydrocortisone and lengthened the survival of HEI-OC1 cells treated with cisplatin (Cervantes et al., 2019).
NLCs were also used to treat inflammatory bowel disease. Huguet-Casquero et al. loaded NLCs with polyphenol (antioxidant/antiinflammatory) oleuropein (OLE). NLC-OLE showed to be more effective in decreasing the TNF-α secretion and intracellular ROS by activated macrophages (J774) compared to the conventional form of OLE in a murine model (Huguet-Casquero et al., 2020)
Fernandez et al. developed a SLN to deliver N-iodomethyl-N, N-Dimethyl-N-(6,6-diphenylhex-5-en-1-yl) ammonium iodide (C6I). The SLNs were obtained by emulsion–solvent evaporation method. They were made of glycerol tripalmitate and glyceryl tristearate. They compared their performance with PLGA NPs. Although SLNs performed better than the free C6I, the SLNs were not capable of overcoming the performance of PLGA (Fernández et al., 2021).
Oligonucleotides are one of the biomolecules that reach their site of action less effectively. Thus, Fabregas et al. characterized the main physicochemical characteristics and binding capabilities of SLNs to oligonucleotides. They optimized the formulation of the NPs to efficiently transfect circular DNA and linear RNA molecules into cells (Fabregas et al., 2017). Gomez-Aguado et al. developed different SLNs by the combination of cationic and ionizable lipids, to deliver mRNA and pDNA. They evaluated their performance in human retinal pigment epithelial cells (ARPE-19) and human embryonic kidney cells (HEK-293). The results showed that SLNs containing only DOTAP (1,2-dioleoyl-3-trimethylammonium-propane) were the most promising formulations for nucleic acid delivery (Gomez-Aguado et al., 2020).
Similarly, Sanchez-Arribas et al. developed a strategy for siRNA delivery using cationic lipoplexes (Sanchez-Arribas et al., 2020a) enabling moderate to high gene lockdown levels. The authors also characterized the protein corona formed around these lipoplexes, that could have an influence on the silencing activity of these agents (Sanchez-Arribas et al., 2020b). Delivery of siRNAs was also performed by Suñé-Pou et al. The authors incorporated the cholesterol derivative cholesteryl oleate to produce SLN–nucleic acid complexes with reduced cytotoxicity and more efficient cellular uptake. They found that intermediate concentrations of cholesteryl oleate exhibited good stability and spherical structures with no aggregation (Sune-Pou et al., 2018). Latorre J. et al. generated a LNP containing a siRNA with a chemically modified lipopolysaccharide-binding protein in order to reduce the levels of fat accumulated in the liver. The NPs showed to be effective in vivo, stressing the potential of this therapy against fatty liver disease (Latorre et al., 2022). In another work, Torrecillas et al. developed a SLN-based shRNA delivery system. This system was designed to downregulate metalloproteinase 9 (MMP-9), a proangiogenic factor, in corneal cells for the treatment of corneal neovascularization associated with inflammation. The non-viral vectors based on SLNs were able to downregulate the MMP-9 expression in HCE-2 cells via gene silencing, and, consequently, to inhibit cell migration and tube formation (Torrecilla et al., 2019).
Martinez-Negro et al. developed a multidisciplinary approach using a nanocarrier built with gemini-bolaamphiphilic hybrid lipids. This strategy resulted in a non-cytotoxic delivery of DNA plasmids in cellular models (Martinez-Negro et al., 2018). With the same goal, nanovesicles have proven to be effective carriers of miRNA in a recent work from Bloix et al. They engineered quatsomes, for the delivery of miRNAs and other small RNAs into the cytosol of tumor cells. The miRNAs delivered by this methodology were able to reach their target destination and thus providing a potential platform for the delivery of these molecules (Boloix et al., 2022).
LNPs have also been employed to cross the BBB to deliver drugs to the brain. Hernando et al. developed polyunsaturated fatty acids (PUFA)-based NLCs, namely, DHAH-NLC. The carriers were modulated with BBB-permeating compounds such as CS and trans-activating transcriptional activator (TAT) from HIV-1. They quantitatively assessed the permeability of DHAH-NLCs in endothelial cells (BMECs). Successfully, they reported that TAT-functionalized DHAH-NLCs successfully crossed the BBB in a cellular model (Hernando et al., 2022).
Improving ocular drug delivery is a milestone that can greatly impact the treatment of eye disorders. Varela-Fernandez et al. designed, developed and performed the physicochemical characterization of lactoferrin-loaded NLCs as a new therapeutic alternative for the keratoconus treatment. Based on the preclinical base obtained, they concluded that NLCs were stable, non-toxic and showed mucoadhesive properties (Varela-Fernandez et al., 2022). Vicente-Pascal et al. designed SLN-based eye drops as gene delivery system to induce the expression of interleukin 10 (IL-10). Two kinds of SLNs combined with different ligands (protamine, dextran, or hyaluronic acid) and formulated with polyvinyl alcohol (PVA) were prepared and tested in cellular and animal models. SLN-based vectors were capable of transfecting corneal epithelial cells. Animal model experiments show that IL-10 could reach even the endothelial layer (Vicente-Pascual et al., 2020; Gomez-Aguado et al., 2021).
To generate human keratinocyte primary cell cultures with potential in tissue engineering Chato-Astrain et al. developed EGF-loaded NLCs. After testing in skin keratinocytes and cornea epithelial cells, and in two epithelial cancer cell lines, gene expression analysis showed that the NLCs were able to increase EGFR gene expression (Chato-Astrain et al., 2021). Tomsen-Melero et al. investigated the potential of nanovesicles as carriers of α-galactosidase A, specifically quatsomes and hybrid liposomes. These structures provided improved colloidal stability in comparison to nanoliposomes and the conditions to preserve α-galactosidase A activity were also characterized, thus resulting in promising nanostructures for the delivery of this enzyme (Tomsen-Melero et al., 2021). In another study, MKC-Quatsomes formed by cholesterol and myristalkonium chloride were highly stable in different media and remained unaltered in human plasma for 24 h. After studying their biodistribution in a xenograft colorectal model they accumulated in tumors, liver, spleen, and kidneys (Vargas-Nadal et al., 2020). In fact, given their stability, they have also been used to efficiently incorporate different fluorophores (Ardizzone et al., 2018a), including diketopyrrolopyrroles, which promoted their photophysical properties and were used for imaging experiments in Saos-2 osteosarcoma cell line (Ardizzone et al., 2018b).
Pasto et al. prepared sodium colistimethate-loaded NLCs to treat respiratory infections (multiresistant P. aeroginosa). Biodistribution assessments showed a mild systemic absorption after nebulization and a notorious absorption after intramuscular route (Pastor et al., 2019).
In this review, we aimed to provide an overview of the nanomedical research currently being performed in Spain. We organized the literature based on NP type and the most common materials employed in their preparation. There is a large number of researchers devoted to engineering NPs with nanomedical applications, and although here we have only focused on NPs with therapeutic applications, there is also a substantial amount of scientists engineering NPs for diagnostic purposes (Ortgies et al., 2016; Jornet-Martinez et al., 2019; Portela et al., 2020). Similarly, the thorough study of NPs and their properties is fundamental to future applications of these therapeutic agents. Here, we have reviewed a fraction of the work, due to space limitations, on the more popular nanoformulations and their therapeutic applications (Table 9). It is important to highlight the diverse applications of every type of NPs, that showcase the versatility of these nanomedical materials and their potential (Alfranca et al., 2016; Perez-Hernandez et al., 2017; Vallet-Regi, 2022).
Indubitably, one of the most explored abilities of NPs is their role as nanocarriers. In fact, in the last years the delivery function continues to improve, reaching a finely tuned controlled release that can be achieved through nanoformulation, and be sensitive to changes in the NPs’ environment, i.e., temperature light, ultrasounds or pH (Xuan et al., 2018; Diez et al., 2021; Mayol et al., 2021; Aguilera-Correa et al., 2022a). Exquisitely controlled layer-by-layer design of NPs enables to control and achieve drug release with spatiotemporal resolution, improving currently available therapeutic options (Correa-Paz et al., 2019). In addition, targeting key pathological cells present in several diseases such as senescent cells through their high β-galactosidase activity was achieved through galacto-oligosaccharides encapsulated drugs, yielding a versatile strategy potentially effective for multiple pathologies (Munoz-Espin et al., 2018). This delivery function can be further improved with the combination of self-propelled materials, (Ramos-Docampo et al., 2019), or synergistic delivery of therapeutic agents as demonstrated by the delivery of siRNA and a peptidic drug with remarkable potential against osteoporosis (Mora-Raimundo et al., 2019). The field however still shows important challenges ahead as highlighted in the aimed insulin oral delivery (Niu et al., 2018) that did not translate into an increased systemic absorption of insulin despite achieving a high retention in enterocytes.
The delivery of therapeutic agents and oligonucleotides based on polymeric nanocomplexes that stabilize and shield RNA analogs enabled the delivery of poly(I:C) to effectively treat several cancers. The preclinical data motivated clinical trials coordinated from Spain in which BO-112 was administered alone or in combinations treatments and performed in several hospitals with positive clinical benefits for some patients (Márquez-Rodas et al., 2022), (NCT: 02828098) (Therapeutics, 2016), (NCT: 05265650) (Clinica Universidad de Navarra UdN, 2022a), (NCT: 04570332) (Therapeutics, 2020). Interestingly, the same poly(I:C) agent in combination with polysaccharide based NPs has been employed for the development of another challenging therapeutic area: the creation of the HIV vaccine. Hyaluronic and chitosan NPs decorated with conserved HIV peptides as antigens and loaded with poly(I:C) yielded in a strong activation af antigen presenting cells (Dacoba et al., 2019).
Another clinical trial has its aim at evaluating antibiotic-containing alginate NPs for the treatment of Pseudomonas aeruginosa infection and biofilm formation in bronchiectasis patients. These type of NPs have shown to be efficient in treating pulmonary infections (Dhand, 2018).
Protein NPs also show promising characteristics for the delivery of insulin which yielded in clinical translation. Specifically the preclinical results obtained with Zein NPs has motivated the design of a clinical trial to verify in humans whether they could provide an efficient glycemic control in diabetic and obese patients through and orally administered therapeutic insulin derivative (NCT: 05560412) (Clinica Universidad de Navarra UdN, 2022b).
Regarding clinical applications, organic NPs and biodegradable MSNs have a competitive advantage over other metallic NPs. However, the remarkable properties that the metallic NPs have motivate their research for fighting complex diseases (Thompson et al., 2017). Thus, the combination of several cores in Janus–type NPs yields highly efficient conjugates that can not only combine complementary drugs or targeting agents but also synergistic effects simultaneously whith great potential to improve the treatment of several diseases (Lopez et al., 2017; Espinosa et al., 2020).
One of the hardest and more significant step in nanomedical development entails reaching clinical trials. This normally challenging road can have additional hurdles to achieve suitable nanoformulations. To translate nanomedicines into the clinic, multiple challenges should be taken into account to manufacture them under quality guidelines regulations (Foulkes et al., 2020). First, the results observed in research settings must be contextualized and standardized to prepare them for clinical translation. While small molecules are commonly evaluated for their efficacy, toxicity, and side effects, nanotherapies must also prove the biocompatibility for each of the components within the formulation. The use of specific nanomaterials for the development of personalized treatment requires in depth understanding of disease biology and the interaction between nanomaterials and the body. The potential of inorganic NPs in biomedicine is still in the preliminary stages of the clinic. Some important challenges in terms of biodistribution, pharmacokinetics, metabolism, biological barriers, safety, large-scale synthesis, patient heterogeneity or overall cost must be taken into account and optimized (Perez-Hernandez et al., 2017). In addition, several key parameters of the intrinsic NP-derived effects such as magnetic hyperthermia must be extensively studied and characterized to better understand its therapeutic operability and improve clinical translation (Beola et al., 2021; Luengo et al., 2022). On the objectives of the Spanish Scientific Network HIPERNANO stablished among different national groups was to consolidate the current scientific knowledge on hyperthermal therapies to enhance their practical implementation in clinical development (Spanish Scientific Network HiperNano, 2018). In addition, a key milestone was reached in 2022, where the first patient with pancreatic cancer was treated with magnetic hyperthermia in combination with the standard of care at Vall d’Hebron hospital (NoCanTher Project, 2022). This pilot study will guide future interventions and shed light on the steps needed to bring nanomedicines to the clinic. From this experience, it is worth highlighting that it is essential to involve multiple stakeholders, from scientists to clinicians, at every level (e.g., nanotherapy and clinical study design) from the beginning of the project to increase the success of this kind of study (Liz-Marzán et al., 2022). Furthermore collaborative nets and workshops such as NanomedSpain, Nanbiosis, Ciber-bbn and NanoSpain enable to promote collaborative studies with this goal.
Finally, while in this review we have only focused on the therapeutic role of NPs, there is another substantial part of nanomedicine that involves the diagnosis of diseases and in which an extensive amount of research groups in Spain are engaged. Both methods to diagnose in vivo (Ximendes et al., 2021), (Ximendes et al., 2017), (Pellico et al., 2021), (Santos et al., 2020) and ex vivo (Pelaez et al., 2018), (Litti et al., 2021), (Enshaei et al., 2021) enabled by several nanoformulations have revolutionized current medical practices and will continue to improve the crucial ability to diagnose diseases efficiently. In addition, the continuous effort of researchers to understand and exquisitely characterize the fate of NPs in biological system is a crucial foundation for all this work (Carregal-Romero et al., 2021; Areny-Balaguero et al., 2022).
VP, PF-G, CPA, and MC contributed to the concept and design of the manuscript. VP, PF-G, CPA, JAB, and CT-B contributed to the writing. BS, AS, ST, AE, GS, and MC critically revised the manuscript and contributed to the writing. All authors contributed to the article and approved the submitted version.
This work has been partially supported by MCIN/AEI /10.13039/501100011033 and European Union NextGenerationEU/PRTR (PID2021-128340OA-I00, PID2020-119352RB-I00, PID2021-127033OB-C21 and RTI2018-101050-J-I00), Comunidad de Madrid (S2022/BMD-7403 RENIM-CM and Talento program 2018-T1/IND-1005), European Union’s Horizon 2020 research and innovation programme (grant agreement No 685795), la Caixa Foundation LCF/PR/HA21/52350003, Asociación Española Contra el Cáncer IDEAS21989THOM, MCIN/AEI/10.13039/501100011033 and “ESF investing in your future” (RYC2019-027489-I, RYC2020-029282-I). CTB thanks Ministerio de Educación (FPU18/06310) for the predoctoral fellowship. IMDEA Nanociencia acknowledges support from the ‘Severo Ochoa’ Programme for Centers of Excellence in R&D (MINECO, CEX2020-001039-S).
The authors want to thank Prof. Dr. S. Ortega-Gutierrez and Prof. Dr. M. L. López-Rodríguez for the inspiration to start this review. Due to space restrictions, we made a selection of publications to be discussed in this review; we apologize to all of our colleagues whose work has not been cited here.
The authors declare that the research was conducted in the absence of any commercial or financial relationships that could be construed as a potential conflict of interest.
All claims expressed in this article are solely those of the authors and do not necessarily represent those of their affiliated organizations, or those of the publisher, the editors and the reviewers. Any product that may be evaluated in this article, or claim that may be made by its manufacturer, is not guaranteed or endorsed by the publisher.
1Hybrid here refers to nanostructures combining two types of inorganic materials in which both are clearly distinguishable (e.g., core-shell, aggregates/encapsulates of individual NPs of both materials) and both provide a relevant function for the application (i.e., are not used just as a coating or as a platform).
Aguilera-Correa, J. J., Garcia-Alvarez, R., Mediero, A., Esteban, J., and Vallet-Regi, M. (2022). Effect of gold nanostars plus amikacin against carbapenem-resistant Klebsiella pneumoniae biofilms. Biol. (Basel). 11 (2), 162. doi:10.3390/biology11020162
Aguilera-Correa, J. J., Gisbert-Garzaran, M., Mediero, A., Fernandez-Acenero, M. J., de-Pablo-Velasco, D., Lozano, D., et al. (2022). Antibiotic delivery from bone-targeted mesoporous silica nanoparticles for the treatment of osteomyelitis caused by methicillin-resistant Staphylococcus aureus. Acta Biomater. 154, 608–625. doi:10.1016/j.actbio.2022.10.039
Aguilera-Garrido, A., Arranz, E., Galvez-Ruiz, M. J., Marchal, J. A., Galisteo-Gonzalez, F., and Giblin, L. (2022). Solid lipid nanoparticles to improve bioaccessibility and permeability of orally administered maslinic acid. Drug Deliv. 29 (1), 1971–1982. doi:10.1080/10717544.2022.2086937
Ahamed, M., Alsalhi, M. S., and Siddiqui, M. K. (2010). Silver nanoparticle applications and human health. Clin. Chim. Acta 411 (23-24), 1841–1848. doi:10.1016/j.cca.2010.08.016
Ahijado-Guzman, R., Sanchez-Arribas, N., Martinez-Negro, M., Gonzalez-Rubio, G., Santiago-Varela, M., Pardo, M., et al. (2020). Intercellular trafficking of gold nanostars in uveal melanoma cells for plasmonic photothermal therapy. Nanomater. (Basel). 10 (3), 590. doi:10.3390/nano10030590
Aires, A., Cadenas, J. F., Guantes, R., and Cortajarena, A. L. (2017). An experimental and computational framework for engineering multifunctional nanoparticles: Designing selective anticancer therapies. Nanoscale 9 (36), 13760–13771. doi:10.1039/c7nr04475e
Alfranca, G., Artiga, A., Stepien, G., Moros, M., Mitchell, S. G., and de la Fuente, J. M. (2016). Gold nanoprism-nanorod face off: Comparing the heating efficiency, cellular internalization and thermoablation capacity. Nanomedicine (Lond). 11 (22), 2903–2916. doi:10.2217/nnm-2016-0257
Alfranca, G., Beola, L., Liu, Y., Gutierrez, L., Zhang, A., Artiga, A., et al. (2019). In vivo comparison of the biodistribution and long-term fate of colloids - gold nanoprisms and nanorods - with minimum surface modification. Nanomedicine (Lond). 14 (23), 3035–3055. doi:10.2217/nnm-2019-0253
Alipour, M., Baneshi, M., Hosseinkhani, S., Mahmoudi, R., Jabari Arabzadeh, A., Akrami, M., et al. (2020). Recent progress in biomedical applications of RGD-based ligand: From precise cancer theranostics to biomaterial engineering: A systematic review. J. Biomed. Mater Res. A 108 (4), 839–850. doi:10.1002/jbm.a.36862
Alvear-Jimenez, A., Zabala Gutierrez, I., Shen, Y., Villaverde, G., Lozano-Chamizo, L., Guardia, P., et al. (2022). Electrospraying as a technique for the controlled synthesis of biocompatible PLGA@Ag2S and PLGA@Ag2S@SPION nanocarriers with drug release capability. Pharmaceutics 14 (1), 214. doi:10.3390/pharmaceutics14010214
Ambrosone, A., Matteis, L., Serrano-Sevilla, I., Tortiglione, C., and De La Fuente, J. M. (2020). Glycogen synthase kinase 3β inhibitor delivered by chitosan nanocapsules promotes safe, fast, and efficient activation of Wnt signaling in vivo. ACS Biomater. Sci. Eng. 6 (5), 2893–2903. doi:10.1021/acsbiomaterials.9b01820
Amendola, V., Pilot, R., Frasconi, M., Marago, O. M., and Iati, M. A. (2017). Surface plasmon resonance in gold nanoparticles: A review. J. Phys. Condens Matter 29 (20), 203002. doi:10.1088/1361-648x/aa60f3
Arana, L., Bayon-Cordero, L., Sarasola, L. I., Berasategi, M., Ruiz, S., and Alkorta, I. (2019). Solid lipid nanoparticles surface modification modulates cell internalization and improves chemotoxic treatment in an oral carcinoma cell line. Nanomater. (Basel) 9 (3), 464. doi:10.3390/nano9030464
Arcos, D., Gomez-Cerezo, N., Saiz-Pardo, M., de Pablo, D., Ortega, L., Enciso, S., et al. (2022). Injectable mesoporous bioactive nanoparticles regenerate bone tissue under osteoporosis conditions. Acta Biomater. 151, 501–511. doi:10.1016/j.actbio.2022.07.067
Ardizzone, A., Blasi, D., Vona, D., Rosspeintner, A., Punzi, A., Altamura, E., et al. (2018). Highly stable and red-emitting nanovesicles incorporating lipophilic diketopyrrolopyrroles for cell imaging. Chemistry 24 (44), 11386–11392. doi:10.1002/chem.201801444
Ardizzone, A., Kurhuzenkau, S., Illa-Tuset, S., Faraudo, J., Bondar, M., Hagan, D., et al. (2018). Nanostructuring lipophilic dyes in water using stable Vesicles, quatsomes, as scaffolds and their use as probes for bioimaging. Small 14 (16), e1703851. doi:10.1002/smll.201703851
Arenas-Vivo, A., Amariei, G., Aguado, S., Rosal, R., and Horcajada, P. (2019). An Ag-loaded photoactive nano-metal organic framework as a promising biofilm treatment. Acta Biomater. 97, 490–500. doi:10.1016/j.actbio.2019.08.011
Areny-Balaguero, A., Mekseriwattana, W., Camprubi-Rimblas, M., Stephany, A., Roldan, A., Sole-Porta, A., et al. (2022). Fluorescent PLGA nanocarriers for pulmonary administration: Influence of the surface charge. Pharmaceutics 14 (7), 1447. doi:10.3390/pharmaceutics14071447
Ariza-Saenz, M., Espina, M., Bolanos, N., Calpena, A. C., Gomara, M. J., Haro, I., et al. (2017). Penetration of polymeric nanoparticles loaded with an HIV-1 inhibitor peptide derived from GB virus C in a vaginal mucosa model. Eur. J. Pharm. Biopharm. 120, 98–106. doi:10.1016/j.ejpb.2017.08.008
Ariza-Saenz, M., Espina, M., Calpena, A., Gomara, M. J., Perez-Pomeda, I., Haro, I., et al. (2018). Design, characterization, and biopharmaceutical behavior of nanoparticles loaded with an HIV-1 fusion inhibitor peptide. Mol. Pharm. 15 (11), 5005–5018. doi:10.1021/acs.molpharmaceut.8b00609
Arriortua, O. K., Insausti, M., Lezama, L., Gil de Muro, I., Garaio, E., de la Fuente, J. M., et al. (2018). RGD-Functionalized Fe3O4 nanoparticles for magnetic hyperthermia. Colloids Surf. B Biointerfaces 165, 315–324. doi:10.1016/j.colsurfb.2018.02.031
Arroyo-Crespo, J. J., Arminan, A., Charbonnier, D., Balzano-Nogueira, L., Huertas-Lopez, F., Marti, C., et al. (2018). Tumor microenvironment-targeted poly-L-glutamic acid-based combination conjugate for enhanced triple negative breast cancer treatment. Biomaterials 186, 8–21. doi:10.1016/j.biomaterials.2018.09.023
Artiga, A., Garcia-Embid, S., De Matteis, L., Mitchell, S. G., and de la Fuente, J. M. (2018). Effective in vitro photokilling by cell-adhesive gold nanorods. Front. Chem. 6, 234. doi:10.3389/fchem.2018.00234
Astorga-Gamaza, A., Vitali, M., Borrajo, M. L., Suarez-Lopez, R., Jaime, C., Bastus, N., et al. (2021). Antibody cooperative adsorption onto AuNPs and its exploitation to force natural killer cells to kill HIV-infected T cells. Nano Today 36, 101056. doi:10.1016/j.nantod.2020.101056
Aznar, M. A., Planelles, L., Perez-Olivares, M., Molina, C., Garasa, S., Etxeberria, I., et al. (2019). Immunotherapeutic effects of intratumoral nanoplexed poly I:C. J. Immunother. Cancer 7 (1), 116. doi:10.1186/s40425-019-0568-2
Baranyai, Z., Soria-Carrera, H., Alleva, M., Millán-Placer, A. C., Lucía, A., Martín-Rapún, R., et al. (2021). Nanotechnology-based targeted drug delivery: An emerging tool to overcome tuberculosis. Adv. Ther. 4 (1), 2000113. doi:10.1002/adtp.202000113
Barbero, F., Michelini, S., Moriones, O. H., Patarroyo, J., Rosell, J., Gusta, M. F., et al. (2022). Role of common cell culture media supplements on citrate-stabilized gold nanoparticle protein corona formation, aggregation state, and the consequent impact on cellular uptake. Bioconjug Chem. 33 (8), 1505–1514. doi:10.1021/acs.bioconjchem.2c00232
Barbero, F., Moriones, O. H., Bastus, N. G., and Puntes, V. (2019). Dynamic equilibrium in the cetyltrimethylammonium bromide-Au nanoparticle bilayer, and the consequent impact on the formation of the nanoparticle protein corona. Bioconjug Chem. 30 (11), 2917–2930. doi:10.1021/acs.bioconjchem.9b00624
Barbero, F., Russo, L., Vitali, M., Piella, J., Salvo, I., Borrajo, M. L., et al. (2017). formation of the protein corona: The interface between nanoparticles and the immune system. Semin. Immunol. 34, 52–60. doi:10.1016/j.smim.2017.10.001
Barbosa, S., Agrawal, A., Rodriguez-Lorenzo, L., Pastoriza-Santos, I., Alvarez-Puebla, R. A., Kornowski, A., et al. (2010). Tuning size and sensing properties in colloidal gold nanostars. Langmuir 26 (18), 14943–14950. doi:10.1021/la102559e
Bass, H., Moore, J. L., and Coakley, W. T. (1978). Lethality in mammalian cells due to hyperthermia under oxic and hypoxic conditions. Int. J. Radiat. Biol. Relat. Stud. Phys. Chem. Med. 33 (1), 57–67. doi:10.1080/09553007714551491
Basso, J., Mendes, M., Silva, J., Cova, T., Luque-Michel, E., Jorge, A. F., et al. (2021). Sorting hidden patterns in nanoparticle performance for glioblastoma using machine learning algorithms. Int. J. Pharm. 592, 120095. doi:10.1016/j.ijpharm.2020.120095
Becerril-Castro, I. B., Calderon, I., Pazos-Perez, L., Guerrini, F., Schulz, N., Feliu, I., et al. (2022). Gold nanostars: Synthesis, optical and SERS analytical properties. Anal. Sens. 2, e202200005. doi:10.1002/anse.202200005
Beola, L., Asin, L., Fratila, R. M., Herrero, V., de la Fuente, J. M., Grazu, V., et al. (2018). Dual role of magnetic nanoparticles as intracellular hotspots and extracellular matrix disruptors triggered by magnetic hyperthermia in 3D cell culture models. ACS Appl. Mater Interfaces 10 (51), 44301–44313. doi:10.1021/acsami.8b18270
Beola, L., Asin, L., Roma-Rodrigues, C., Fernandez-Afonso, Y., Fratila, R. M., Serantes, D., et al. (2020). The intracellular number of magnetic nanoparticles modulates the apoptotic death pathway after magnetic hyperthermia treatment. ACS Appl. Mater Interfaces 12 (39), 43474–43487. doi:10.1021/acsami.0c12900
Beola, L., Grazu, V., Fernandez-Afonso, Y., Fratila, R. M., de Las Heras, M., de la Fuente, J. M., et al. (2021). Critical parameters to improve pancreatic cancer treatment using magnetic hyperthermia: Field conditions, immune response, and particle biodistribution. ACS Appl. Mater Interfaces 13 (11), 12982–12996. doi:10.1021/acsami.1c02338
Bernardos, A., Piacenza, E., Sancenon, F., Hamidi, M., Maleki, A., Turner, R. J., et al. (2019). Mesoporous silica-based materials with bactericidal properties. Small 15 (24), e1900669. doi:10.1002/smll.201900669
Boloix, A., Feiner-Gracia, N., Kober, M., Repetto, J., Pascarella, R., Soriano, A., et al. (2022). Engineering pH-sensitive stable nanovesicles for delivery of MicroRNA therapeutics. Small 18 (3), e2101959. doi:10.1002/smll.202101959
Bonet-Aleta, J., Encinas-Gimenez, M., Urriolabeitia, E., Martin-Duque, P., Hueso, J. L., and Santamaria, J. (2022). Unveiling the interplay between homogeneous and heterogeneous catalytic mechanisms in copper-iron nanoparticles working under chemically relevant tumour conditions. Chem. Sci. 13 (28), 8307–8320. doi:10.1039/d2sc01379g
Bonet-Aleta, J., Sancho-Albero, M., Calzada-Funes, J., Irusta, S., Martin-Duque, P., Hueso, J. L., et al. (2022). Glutathione-Triggered catalytic response of Copper-Iron mixed oxide Nanoparticles. Leveraging tumor microenvironment conditions for chemodynamic therapy. J. Colloid Interface Sci. 617, 704–717. doi:10.1016/j.jcis.2022.03.036
Borzenkov, M., Pallavicini, P., and Chirico, G. (2019). Photothermally active inorganic nanoparticles: From colloidal solutions to photothermally active printed surfaces and polymeric nanocomposite materials. Eur. J. Inorg. Chem. 2019 (41), 4397–4404. doi:10.1002/ejic.201900836
Cabrera, D., Coene, A., Leliaert, J., Artes-Ibanez, E. J., Dupre, L., Telling, N. D., et al. (2018). Dynamical magnetic response of iron oxide nanoparticles inside live cells. ACS Nano 12 (3), 2741–2752. doi:10.1021/acsnano.7b08995
Cacicedo, M. L., Islan, G. A., Leon, I. E., Alvarez, V. A., Chourpa, I., Allard-Vannier, E., et al. (2018). Bacterial cellulose hydrogel loaded with lipid nanoparticles for localized cancer treatment. Colloids Surf. B Biointerfaces 170, 596–608. doi:10.1016/j.colsurfb.2018.06.056
Cadete, A., Olivera, A., Besev, M., Dhal, P. K., Goncalves, L., Almeida, A. J., et al. (2019). Self-assembled hyaluronan nanocapsules for the intracellular delivery of anticancer drugs. Sci. Rep. 9 (1), 11565. doi:10.1038/s41598-019-47995-8
Carrillo-Carrion, C., Martinez, R., Navarro Poupard, M. F., Pelaz, B., Polo, E., Arenas-Vivo, A., et al. (2019). Aqueous stable gold nanostar/ZIF-8 nanocomposites for light-triggered release of active cargo inside living cells. Angew. Chem. Int. Ed. Engl. 58 (21), 7078–7082. doi:10.1002/anie.201902817
Casadome-Perales, A., Matteis, L., Alleva, M., Infantes-Rodriguez, C., Palomares-Perez, I., Saito, T., et al. (2019). Inhibition of p38 MAPK in the brain through nasal administration of p38 inhibitor loaded in chitosan nanocapsules. Nanomedicine (Lond) 14 (18), 2409–2422. doi:10.2217/nnm-2018-0496
Casarrubios, L., Gomez-Cerezo, N., Feito, M. J., Vallet-Regi, M., Arcos, D., and Portoles, M. T. (2020). Ipriflavone-loaded mesoporous nanospheres with potential applications for periodontal treatment. Nanomater. (Basel) 10 (12), 2573. doi:10.3390/nano10122573
Casarrubios, L., Polo-Montalvo, A., Serrano, M. C., Feito, M. J., Vallet-Regi, M., Arcos, D., et al. (2021). Effects of ipriflavone-loaded mesoporous nanospheres on the differentiation of endothelial progenitor cells and their modulation by macrophages. Nanomater. (Basel) 11 (5), 1102. doi:10.3390/nano11051102
Cervantes, B., Arana, L., Murillo-Cuesta, S., Bruno, M., Alkorta, I., and Varela-Nieto, I. (2019). Solid lipid nanoparticles loaded with glucocorticoids protect auditory cells from cisplatin-induced ototoxicity. J. Clin. Med. 8 (9), 1464. doi:10.3390/jcm8091464
Chato-Astrain, J., Sanchez-Porras, D., Garcia-Garcia, O. D., Vairo, C., Villar-Vidal, M., Villullas, S., et al. (2021). Improvement of cell culture methods for the successful generation of human keratinocyte primary cell cultures using EGF-loaded nanostructured lipid carriers. Biomedicines 9 (11), 1634. doi:10.3390/biomedicines9111634
Chin, K. C., Chong, G. L., Poh, C. K., Van, L. H., Sow, C. H., Lin, J., et al. (2007). Large-scale synthesis of Fe3O4 nanosheets at low temperature. J. Phys. Chem. C 111 (26), 9136–9141. doi:10.1021/jp070873g
Christou, E., Pearson, J. R., Beltran, A. M., Fernandez-Afonso, Y., Gutierrez, L., de la Fuente, J. M., et al. (2022). Iron-gold nanoflowers: A promising tool for multimodal imaging and hyperthermia therapy. Pharmaceutics 14 (3), 636. doi:10.3390/pharmaceutics14030636
Clinica Universidad de Navarra UdN (2022a). Study of BO-112 with radiotherapy and nivolumab for metastatic refractory NSCLC (noelia) clinicaltrials.gov2022. Available from: https://clinicaltrials.gov/ct2/show/NCT05265650?term=BO-112&draw=2&rank=1.
Clinica Universidad de Navarra UdN (2022b). Zein nanoparticles for glycemic control (GLUCOCAPS) clinicaltrials.gov2022. Available from: https://clinicaltrials.gov/ct2/show/NCT05560412?term=zein&cntry=ES&draw=2&rank=1.
Colilla, M., and Vallet-Regi, M. (2020). Targeted stimuli-responsive mesoporous silica nanoparticles for bacterial infection treatment. Int. J. Mol. Sci. 21 (22), 8605. doi:10.3390/ijms21228605
Cordeiro, A. S., Crecente-Campo, J., Bouzo, B. L., Gonzalez, S. F., de la Fuente, M., and Alonso, M. J. (2019). Engineering polymeric nanocapsules for an efficient drainage and biodistribution in the lymphatic system. J. Drug Target 27 (5-6), 646–658. doi:10.1080/1061186x.2018.1561886
Correa-Paz, C., Navarro Poupard, M. F., Polo, E., Rodriguez-Perez, M., Taboada, P., Iglesias-Rey, R., et al. (2019). In vivo ultrasound-activated delivery of recombinant tissue plasminogen activator from the cavity of sub-micrometric capsules. J. Control Release 308, 162–171. doi:10.1016/j.jconrel.2019.07.017
Crecente-Campo, J., Virgilio, T., Morone, D., Calvino-Sampedro, C., Fernandez-Marino, I., Olivera, A., et al. (2019). Design of polymeric nanocapsules to improve their lympho-targeting capacity. Nanomedicine (Lond). 14 (23), 3013–3033. doi:10.2217/nnm-2019-0206
Dacoba, T. G., Anfray, C., Mainini, F., Allavena, P., Alonso, M. J., Torres Andon, F., et al. (2020). Arginine-based poly(I:C)-Loaded nanocomplexes for the polarization of macrophages toward M1-antitumoral effectors. Front. Immunol. 11, 1412. doi:10.3389/fimmu.2020.01412
Dacoba, T. G., Olivera, A., Torres, D., Crecente-Campo, J., and Alonso, M. J. (2017). Modulating the immune system through nanotechnology. Semin. Immunol. 34, 78–102. doi:10.1016/j.smim.2017.09.007
Dacoba, T. G., Omange, R. W., Li, H., Crecente-Campo, J., Luo, M., and Alonso, M. J. (2019). Polysaccharide nanoparticles can efficiently modulate the immune response against an HIV peptide antigen. ACS Nano 13 (5), 4947–4959. doi:10.1021/acsnano.8b07662
Dacoba, T. G., Ruiz-Gaton, L., Benito, A., Klein, M., Dupin, D., Luo, M., et al. (2020). Technological challenges in the preclinical development of an HIV nanovaccine candidate. Drug Deliv. Transl. Res. 10 (3), 621–634. doi:10.1007/s13346-020-00721-8
de la Presa, P., Luengo, Y., Multigner, M., Costo, R., Morales, M. P., Rivero, G., et al. (2012). Study of heating efficiency as a function of concentration, size, and applied field in γ-Fe2O3 nanoparticles. J. Phys. Chem. C 116 (48), 25602–25610. doi:10.1021/jp310771p
De Luca, R., Profita, G., and Cicero, G. (2019). <p>Nab-paclitaxel in pretreated metastatic breast cancer: Evaluation of activity, safety, and quality of life</p>. Onco Targets Ther. 12, 1621–1627. doi:10.2147/ott.s191519
De Matteis, L., Jary, D., Lucía, A., García-Embid, S., Serrano-Sevilla, I., Pérez, D., et al. (2018). New active formulations against M. tuberculosis: Bedaquiline encapsulation in lipid nanoparticles and chitosan nanocapsules. Chem. Eng. J. 340, 181–191. doi:10.1016/j.cej.2017.12.110
DeDiego, M. L., Portilla, Y., Daviu, N., Lopez-Garcia, D., Villamayor, L., Mulens-Arias, V., et al. (2022). Iron oxide and iron oxyhydroxide nanoparticles impair SARS-CoV-2 infection of cultured cells. J. Nanobiotechnology 20 (1), 352. doi:10.1186/s12951-022-01542-2
Del Sol-Fernandez, S., Portilla-Tundidor, Y., Gutierrez, L., Odio, O. F., Reguera, E., Barber, D. F., et al. (2019). Flower-like Mn-doped magnetic nanoparticles functionalized with αvβ3-integrin-ligand to efficiently induce intracellular heat after alternating magnetic field exposition, triggering glioma cell death. ACS Appl. Mater Interfaces 11 (30), 26648–26663. doi:10.1021/acsami.9b08318
Dhand, R. (2018). The rationale and evidence for use of inhaled antibiotics to control Pseudomonas aeruginosa infection in non-cystic fibrosis Bronchiectasis. J. Aerosol Med. Pulm. Drug Deliv. 31 (3), 121–138. doi:10.1089/jamp.2017.1415
Dhiman, N., Awasthi, R., Sharma, B., Kharkwal, H., and Kulkarni, G. T. (2021). Lipid nanoparticles as carriers for bioactive delivery. Front. Chem. 9, 580118. doi:10.3389/fchem.2021.580118
Diego-Gonzalez, L., Crecente-Campo, J., Paul, M. J., Singh, M., Reljic, R., Alonso, M. J., et al. (2020). Design of polymeric nanocapsules for intranasal vaccination against Mycobacterium tuberculosis: Influence of the polymeric shell and antigen positioning. Pharmaceutics 12 (6), 489. doi:10.3390/pharmaceutics12060489
Diez, P., Lucena-Sanchez, E., Escudero, A., Llopis-Lorente, A., Villalonga, R., and Martinez-Manez, R. (2021). Ultrafast directional Janus Pt-mesoporous silica nanomotors for smart drug delivery. ACS Nano 15 (3), 4467–4480. doi:10.1021/acsnano.0c08404
Di Silvio, D., Silvestri, A., Lay, L., Polito, L., and Moya, S. E. (2018). Impact of concanavalinA affinity in the intracellular fate of protein corona on Glucosamine Au nanoparticles. Scientific Reports 8 (1), 9046. doi:10.1038/s41598-018-27418-w
Do, T. T. A., Grijalvo, S., Imae, T., Garcia-Celma, M. J., and Rodriguez-Abreu, C. (2021). A nanocellulose-based platform towards targeted chemo-photodynamic/photothermal cancer therapy. Carbohydr. Polym. 270, 118366. doi:10.1016/j.carbpol.2021.118366
Dolai, J., Mandal, K., and Jana, N. R. (2021). Nanoparticle size effects in biomedical applications. ACS Appl. Nano Mater. 4 (7), 6471–6496. doi:10.1021/acsanm.1c00987
Dosil, S. G., Lopez-Cobo, S., Rodriguez-Galan, A., Fernandez-Delgado, I., et al. (2022). Natural killer (NK) cell-derived extracellular-vesicle shuttled microRNAs control T cell responses. Elife 11, e76319. doi:10.7554/elife.76319
Duro-Castano, A., Borras, C., Herranz-Perez, V., Blanco-Gandia, M. C., Conejos-Sanchez, I., Arminan, A., et al. (2021). Targeting Alzheimer's disease with multimodal polypeptide-based nanoconjugates. Sci. Adv. 7 (13), eabf9180. doi:10.1126/sciadv.abf9180
Duro-Castano, A., Sousa-Herves, A., Arminan, A., Charbonnier, D., Arroyo-Crespo, J. J., Wedepohl, S., et al. (2021). Polyglutamic acid-based crosslinked doxorubicin nanogels as an anti-metastatic treatment for triple negative breast cancer. J. Control Release 332, 10–20. doi:10.1016/j.jconrel.2021.02.005
Elming, P. B., Sorensen, B. S., Oei, A. L., Franken, N. A. P., Crezee, J., Overgaard, J., et al. (2019). Hyperthermia: The optimal treatment to overcome radiation resistant hypoxia. Cancers (Basel) 11 (1), 60. doi:10.3390/cancers11010060
Elsabahy, M., and Wooley, K. L. (2012). Design of polymeric nanoparticles for biomedical delivery applications. Chem. Soc. Rev. 41 (7), 2545–2561. doi:10.1039/c2cs15327k
Encinas-Basurto, D., Ibarra, J., Juarez, J., Pardo, A., Barbosa, S., Taboada, P., et al. (2018). Hybrid folic acid-conjugated gold nanorods-loaded human serum albumin nanoparticles for simultaneous photothermal and chemotherapeutic therapy. Mater Sci. Eng. C Mater Biol. Appl. 91, 669–678. doi:10.1016/j.msec.2018.06.002
Encinas-Basurto, D., Juarez, J., Valdez, M. A., Burboa, M. G., Barbosa, S., and Taboada, P. (2018). Targeted drug delivery via human epidermal growth factor receptor for sustained release of allyl isothiocyanate. Curr. Top. Med. Chem. 18 (14), 1252–1260. doi:10.2174/1568026618666180810150113
Enshaei, H., Puiggali-Jou, A., Del Valle, L. J., Turon, P., Saperas, N., and Aleman, C. (2021). Nanotheranostic interface based on antibiotic-loaded conducting polymer nanoparticles for real-time monitoring of bacterial growth inhibition. Adv. Healthc. Mater 10 (7), e2001636. doi:10.1002/adhm.202001636
Escudero-Duch, C., Martin-Saavedra, F., Prieto, M., Sanchez-Casanova, S., Lopez, D., Sebastian, V., et al. (2019). Gold nanoparticles for the in situ polymerization of near-infrared responsive hydrogels based on fibrin. Acta Biomater. 100, 306–315. doi:10.1016/j.actbio.2019.09.040
Espinosa, A., Castro, G. R., Reguera, J., Castellano, C., Castillo, J., Camarero, J., et al. (2021). Photoactivated nanoscale temperature gradient detection using X-ray absorption spectroscopy as a direct nanothermometry method. Nano Lett. 21 (1), 769–777. doi:10.1021/acs.nanolett.0c04477
Espinosa, A., Reguera, J., Curcio, A., Munoz-Noval, A., Kuttner, C., Van de Walle, A., et al. (2020). Janus magnetic-plasmonic nanoparticles for magnetically guided and thermally activated cancer therapy. Small 16 (11), e1904960. doi:10.1002/smll.201904960
Espinosa, A., Silva, A. K., Sanchez-Iglesias, A., Grzelczak, M., Pechoux, C., Desboeufs, K., et al. (2016). Cancer cell internalization of gold nanostars impacts their photothermal efficiency in vitro and in vivo: Toward a plasmonic thermal fingerprint in tumoral environment. Adv. Healthc. Mater 5 (9), 1040–1048. doi:10.1002/adhm.201501035
Espinosa-Cano, E., Aguilar, M. R., Portilla, Y., Barber, D. F., and San Roman, J. (2020). Anti-inflammatory polymeric nanoparticles based on ketoprofen and dexamethasone. Pharmaceutics 12 (8), 723. doi:10.3390/pharmaceutics12080723
Espinosa-Cano, E., Aguilar, M. R., Portilla, Y., Barber, D. F., and San Roman, J. (2020). Polymeric nanoparticles that combine dexamethasone and naproxen for the synergistic inhibition of Il12b transcription in macrophages. Macromol. Biosci. 20 (7), e2000002. doi:10.1002/mabi.202000002
Etchenausia, L., Villar-Alvarez, E., Forcada, J., Save, M., and Taboada, P. (2019). Evaluation of cationic core-shell thermoresponsive poly(N-vinylcaprolactam)-based microgels as potential drug delivery nanocarriers. Mater Sci. Eng. C Mater Biol. Appl. 104, 109871. doi:10.1016/j.msec.2019.109871
Etxebeste-Mitxeltorena, M., Moreno, E., Carvalheiro, M., Calvo, A., Navarro-Blasco, I., Gonzalez-Penas, E., et al. (2021). Oral efficacy of a diselenide compound loaded in nanostructured lipid carriers in a murine model of visceral leishmaniasis. ACS Infect. Dis. 7 (12), 3197–3209. doi:10.1021/acsinfecdis.1c00394
Fabregas, A., Prieto-Sanchez, S., Sune-Pou, M., Boyero-Corral, S., Tico, J. R., Garcia-Montoya, E., et al. (2017). Improved formulation of cationic solid lipid nanoparticles displays cellular uptake and biological activity of nucleic acids. Int. J. Pharm. 516 (1-2), 39–44. doi:10.1016/j.ijpharm.2016.11.026
Fabrizio, E. D., Schlücker, S., Wenger, J., Regmi, R., Rigneault, H., Calafiore, G., et al. (2016). Roadmap on biosensing and photonics with advanced nano-optical methods. J. Opt. 18 (6), 063003. doi:10.1088/2040-8978/18/6/063003
Falgas, A., Pallares, V., Serna, N., Sanchez-Garcia, L., Sierra, J., Gallardo, A., et al. (2020). Selective delivery of T22-PE24-H6 to CXCR4(+) diffuse large B-cell lymphoma cells leads to wide therapeutic index in a disseminated mouse model. Theranostics 10 (12), 5169–5180. doi:10.7150/thno.43231
Fernández, M., Holgado, M. Á., Cayero-Otero, M. D., Pineda, T., Yepes, L. M., Gaspar, D. P., et al. (2021). Improved antileishmanial activity and cytotoxicity of a novel nanotherapy for N-iodomethyl-N,N-dimethyl-N-(6,6-diphenylhex-5-en-1-yl)ammonium iodide. J. Drug Deliv. Sci. Technol. 61, 101988. doi:10.1016/j.jddst.2020.101988
Fernandez, Y., Movellan, J., Foradada, L., Gimenez, V., Garcia-Aranda, N., Mancilla, S., et al. (2022). In vivo antitumor and antimetastatic efficacy of a polyacetal-based paclitaxel conjugate for prostate cancer therapy. Adv. Healthc. Mater 11 (7), e2101544. doi:10.1002/adhm.202101544
Fornaguera, C., Diaz-Caballero, M., Garcia-Fernandez, C., Olmo, L., Stampa-Lopez Pinto, M., Navalon-Lopez, M., et al. (2021). Synthesis and characterization of mRNA-loaded poly(beta aminoesters) nanoparticles for vaccination purposes. J. Vis. Exp. 2021, 174.
Foulkes, R., Man, E., Thind, J., Yeung, S., Joy, A., and Hoskins, C. (2020). The regulation of nanomaterials and nanomedicines for clinical application: Current and future perspectives. Biomater. Sci. 8 (17), 4653–4664. doi:10.1039/d0bm00558d
Qu, N., Luo, Z., Zhao, S., and Liu, B. (2021). Frame-guided synthesis of polymeric colloidal discs. J. Am. Chem. Soc. 143 (4), 1790–1797. doi:10.1021/jacs.0c08627
Fleury, J. B., Werner, M., Guével, X. L., and Baulin, V. A. (2021). Protein corona modulates interaction of spiky nanoparticles with lipid bilayers. Journal of Colloid and Interface Science 603, 550–558. doi:10.1016/j.jcis.2021.06.047
Fratila, R. M., and de la Fuente, J. M. (2019). “Introduction to hyperthermia,” in Nanomaterials for magnetic and optical hyperthermia applications. Editors R. M. Fratila, and J. M. De La Fuente (Elsevier), 1–10.
Frigaard, J., Jensen, J. L., Galtung, H. K., and Hiorth, M. (2022). The potential of chitosan in nanomedicine: An overview of the cytotoxicity of chitosan based nanoparticles. Front. Pharmacol. 13, 880377. doi:10.3389/fphar.2022.880377
Fuentes-García, J. A. C. A, A., de Castro, C. E., Giacomelli, F. C., Ibarra, M. R., Bonvent, J., Fabián Goya, G., et al. (2021). Sonochemical route for mesoporous silica-coated magnetic nanoparticles towards pH-triggered drug delivery system. J. Mater Res. Technol. 15, 52–67. doi:10.1016/j.jmrt.2021.08.014
Galindo, R., Sanchez-Lopez, E., Gomara, M. J., Espina, M., Ettcheto, M., Cano, A., et al. (2022). Development of peptide targeted PLGA-PEGylated nanoparticles loading licochalcone-A for ocular inflammation. Pharmaceutics 14 (2), 285. doi:10.3390/pharmaceutics14020285
Gallego-Lleyda, A., De Miguel, D., Anel, A., and Martinez-Lostao, L. (2018). Lipid nanoparticles decorated with TNF-related aptosis-inducing ligand (TRAIL) are more cytotoxic than soluble recombinant TRAIL in sarcoma. Int. J. Mol. Sci. 19 (5), 1449. doi:10.3390/ijms19051449
Gallego-Yerga, L., Benito, J. M., Blanco-Fernandez, L., Martinez-Negro, M., Velaz, I., Aicart, E., et al. (2018). Plasmid-templated control of DNA-cyclodextrin nanoparticle morphology through molecular vector design for effective gene delivery. Chemistry 24 (15), 3825–3835. doi:10.1002/chem.201705723
García, A., González, B., Harvey, C., Izquierdo-Barba, I., and Vallet-Regí, M. (2021). Effective reduction of biofilm through photothermal therapy by gold core@shell based mesoporous silica nanoparticles. Microporous Mesoporous Mater. 328, 111489. doi:10.1016/j.micromeso.2021.111489
Garcia, J., Fernandez-Pradas, J. M., Llado, A., Serra, P., Zalvidea, D., Kogan, M. J., et al. (2021). The combined use of gold nanoparticles and infrared radiation enables cytosolic protein delivery. Chemistry 27 (14), 4670–4675. doi:10.1002/chem.202005000
Garcia, M. A. (2011). Surface plasmons in metallic nanoparticles: Fundamentals and applications. J. Phys. D Appl. Phys. 44 (28), 283001. doi:10.1088/0022-3727/44/28/283001
Garcia-Alvarez, R., and Vallet-Regi, M. (2021). Hard and soft protein corona of nanomaterials: Analysis and relevance. Nanomater. (Basel). 11 (4), 888. doi:10.3390/nano11040888
Garcia-Fernandez, A., Sancenon, F., and Martinez-Manez, R. (2021). Mesoporous silica nanoparticles for pulmonary drug delivery. Adv. Drug Deliv. Rev. 177, 113953. doi:10.1016/j.addr.2021.113953
Garcia-Fernandez, A., Sancho, M., Bisbal, V., Amoros, P., Marcos, M. D., Orzaez, M., et al. (2021). Targeted-lung delivery of dexamethasone using gated mesoporous silica nanoparticles. A new therapeutic approach for acute lung injury treatment. J. Control Release 337, 14–26. doi:10.1016/j.jconrel.2021.07.010
Garcia-Garrido, E., Cordani, M., and Somoza, A. (2021). Modified gold nanoparticles to overcome the chemoresistance to gemcitabine in mutant p53 cancer cells. Pharmaceutics 13 (12), 2067. doi:10.3390/pharmaceutics13122067
Garcia-Lojo, D., Nunez-Sanchez, S., Gomez-Grana, S., Grzelczak, M., Pastoriza-Santos, I., Perez-Juste, J., et al. (2019). Plasmonic supercrystals. Acc. Chem. Res. 52 (7), 1855–1864. doi:10.1021/acs.accounts.9b00213
Garcia-Soriano, D., Amaro, R., Lafuente-Gomez, N., Milan-Rois, P., Somoza, A., Navio, C., et al. (2020). The influence of cation incorporation and leaching in the properties of Mn-doped nanoparticles for biomedical applications. J. Colloid Interface Sci. 578, 510–521. doi:10.1016/j.jcis.2020.06.011
Garcia-Soriano, D., Milan-Rois, P., Lafuente-Gomez, N., Navio, C., Gutierrez, L., Cusso, L., et al. (2022). Iron oxide-manganese oxide nanoparticles with tunable morphology and switchable MRI contrast mode triggered by intracellular conditions. J. Colloid Interface Sci. 613, 447–460. doi:10.1016/j.jcis.2022.01.070
Gaspar, D. P., Gaspar, M. M., Eleuterio, C. V., Grenha, A., Blanco, M., Goncalves, L. M. D., et al. (2017). Microencapsulated solid lipid nanoparticles as a hybrid platform for pulmonary antibiotic delivery. Mol. Pharm. 14 (9), 2977–2990. doi:10.1021/acs.molpharmaceut.7b00169
Gatoo, M. A., Naseem, S., Arfat, M. Y., Dar, A. M., Qasim, K., and Zubair, S. (2014). Physicochemical properties of nanomaterials: Implication in associated toxic manifestations. Biomed. Res. Int. 2014, 1–8. doi:10.1155/2014/498420
Gavilán, H., Kowalski, A., Heinke, D., Sugunan, A., Sommertune, J., Varón, M., et al. (2017). Colloidal flower-shaped iron oxide nanoparticles: Synthesis strategies and coatings. Part. Part. Syst. Charact. 34 (7), 1700094. doi:10.1002/ppsc.201700094
Gerke, C., Zabala Gutierrez, I., Méndez-González, D., Cruz, M. C. I., Mulero, F., Jaque, D., et al. (2022). Clickable albumin nanoparticles for pretargeted drug delivery toward PD-L1 overexpressing tumors in combination immunotherapy. Bioconjugate Chemistry 33 (5), 821–828. doi:10.1021/acs.bioconjchem.2c00087
Giner-Casares, J. J., Henriksen-Lacey, M., Coronado-Puchau, M., and Liz-Marzán, L. M. (2016). Inorganic nanoparticles for biomedicine: Where materials scientists meet medical research. Mater. Today 19 (1), 19–28. doi:10.1016/j.mattod.2015.07.004
Gisbert-Garzaran, M., Lozano, D., and Vallet-Regi, M. (2020). Mesoporous silica nanoparticles for targeting subcellular organelles. Int. J. Mol. Sci. 21 (24), 9696. doi:10.3390/ijms21249696
Goesmann, H., and Feldmann, C. (2010). Cover picture: Nanoparticulate functional materials (angew. Chem. Int. Ed. 8/2010). Angew. Chem. Int. Ed. 49 (8), 1333. doi:10.1002/anie.200907226
Gomez-Aguado, I., Rodriguez-Castejon, J., Beraza-Millor, M., Vicente-Pascual, M., Rodriguez-Gascon, A., Garelli, S., et al. (2021). mRNA-based nanomedicinal products to address corneal inflammation by interleukin-10 supplementation. Pharmaceutics 13 (9), 1472. doi:10.3390/pharmaceutics13091472
Gomez-Aguado, I., Rodriguez-Castejon, J., Vicente-Pascual, M., Rodriguez-Gascon, A., Pozo-Rodriguez, A. D., and Solinis Aspiazu, M. A. (2020). Nucleic acid delivery by solid lipid nanoparticles containing switchable lipids: Plasmid DNA vs. Messenger RNA. Mol. 25 (24), 5995. doi:10.3390/molecules25245995
Gomez-Grana, S., Perez-Ameneiro, M., Vecino, X., Pastoriza-Santos, I., Perez-Juste, J., Cruz, J. M., et al. (2017). Biogenic synthesis of metal nanoparticles using a biosurfactant extracted from corn and their antimicrobial properties. Nanomater. (Basel) 7 (6), 139. doi:10.3390/nano7060139
Gonzalez-Aramundiz, J. V., Peleteiro, M., Gonzalez-Fernandez, A., Alonso, M. J., and Csaba, N. S. (2018). Protamine nanocapsules for the development of thermostable adjuvanted nanovaccines. Mol. Pharm. 15 (12), 5653–5664. doi:10.1021/acs.molpharmaceut.8b00852
Gonzalez-Aramundiz, J. V., Presas, E., Dalmau-Mena, I., Martinez-Pulgarin, S., Alonso, C., Escribano, J. M., et al. (2017). Rational design of protamine nanocapsules as antigen delivery carriers. J. Control Release 245, 62–69. doi:10.1016/j.jconrel.2016.11.012
Gonzalez-Ballesteros, N., Diego-Gonzalez, L., Lastra-Valdor, M., Grimaldi, M., Cavazza, A., Bigi, F., et al. (2021). Saccorhiza polyschides used to synthesize gold and silver nanoparticles with enhanced antiproliferative and immunostimulant activity. Mater Sci. Eng. C Mater Biol. Appl. 123, 111960. doi:10.1016/j.msec.2021.111960
Gonzalez-Ballesteros, N., Torres, M. D., Florez-Fernandez, N., Diego-Gonzalez, L., Simon-Vazquez, R., Rodriguez-Arguelles, M. C., et al. (2021). Eco-friendly extraction of Mastocarpus stellatus carrageenan for the synthesis of gold nanoparticles with improved biological activity. Int. J. Biol. Macromol. 183, 1436–1449. doi:10.1016/j.ijbiomac.2021.05.115
Gonzalez-Fernandez, S., Lozano-Iturbe, V., Menendez, M. F., Ordiales, H., Fernandez-Vega, I., Merayo, J., et al. (2022). A promising antifungal and antiamoebic effect of silver nanorings, a novel type of AgNP. Antibiot. (Basel) 11 (8), 1054. doi:10.3390/antibiotics11081054
Gonzalez-Fernandez, Y., Brown, H. K., Patino-Garcia, A., Heymann, D., and Blanco-Prieto, M. J. (2018). Oral administration of edelfosine encapsulated lipid nanoparticles causes regression of lung metastases in pre-clinical models of osteosarcoma. Cancer Lett. 430, 193–200. doi:10.1016/j.canlet.2018.05.030
Gonzalez-Pastor, R., Hernandez, Y., Gimeno, M., de Martino, A., Man, Y. K. S., Hallden, G., et al. (2021). Coating an adenovirus with functionalized gold nanoparticles favors uptake, intracellular trafficking and anti-cancer therapeutic efficacy. Acta Biomater. 134, 593–604. doi:10.1016/j.actbio.2021.07.047
Gonzalez-Pedroza, M. G., Argueta-Figueroa, L., Garcia-Contreras, R., Jimenez-Martinez, Y., Martinez-Martinez, E., Navarro-Marchal, S. A., et al. (2021). Silver nanoparticles from Annona muricata peel and leaf extracts as a potential potent, biocompatible and low cost antitumor tool. Biocompatible Low Cost Antitumor Tool. Nanomater. (Basel). 11 (5), 1273. doi:10.3390/nano11051273
Grayston, A., Zhang, Y., Garcia-Gabilondo, M., Arrue, M., Martin, A., Kopcansky, P., et al. (2022). Endovascular administration of magnetized nanocarriers targeting brain delivery after stroke. J. Cereb. Blood Flow. Metab. 42 (2), 237–252. doi:10.1177/0271678x211028816
Grimaudo, M. A., Concheiro, A., and Alvarez-Lorenzo, C. (2020). Crosslinked hyaluronan electrospun nanofibers for ferulic acid ocular delivery. Pharmaceutics 12 (3), 274. doi:10.3390/pharmaceutics12030274
Grzelak, J., Teles, M., Roher, N., Grayston, A., Rosell, A., Gich, M., et al. (2022). Bioevaluation of magnetic mesoporous silica rods: Cytotoxicity, cell uptake and biodistribution in zebrafish and rodents. RSC Adv. 12 (49), 31878–31888. doi:10.1039/d2ra05750f
Guasch, J., Hoffmann, M., Diemer, J., Riahinezhad, H., Neubauer, S., Kessler, H., et al. (2018). Combining adhesive nanostructured surfaces and costimulatory signals to increase T cell activation. Nano Lett. 18 (9), 5899–5904. doi:10.1021/acs.nanolett.8b02588
Guisasola, E., Asin, L., Beola, L., de la Fuente, J. M., Baeza, A., and Vallet-Regi, M. (2018). Beyond traditional hyperthermia: In vivo cancer treatment with magnetic-responsive mesoporous silica nanocarriers. ACS Appl. Mater Interfaces 10 (15), 12518–12525. doi:10.1021/acsami.8b02398
Guisasola, E., Baeza, A., Asín, L., de la Fuente, J. M., and Vallet-Regí, M. (2018). Heating at the nanoscale through drug-delivery devices: Fabrication and synergic effects in cancer treatment with nanoparticles. Small Methods 2 (9), 1800007. doi:10.1002/smtd.201800007
Gundogdu, E., Demir, E. S., Ekinci, M., Ozgenc, E., Ilem-Ozdemir, D., Senyigit, Z., et al. (2022). An innovative formulation based on nanostructured lipid carriers for imatinib delivery: Pre-formulation, cellular uptake and cytotoxicity studies. Nanomater. (Basel). 12 (2), 250. doi:10.3390/nano12020250
Hendiger, E. B., Padzik, M., Sifaoui, I., Reyes-Batlle, M., Lopez-Arencibia, A., Rizo-Liendo, A., et al. (2020). Silver nanoparticles as a novel potential preventive agent against Acanthamoeba keratitis. Pathogens 9 (5), 350. doi:10.3390/pathogens9050350
Hendiger, E. B., Padzik, M., Sifaoui, I., Reyes-Batlle, M., Lopez-Arencibia, A., Zyskowska, D., et al. (2021). Silver nanoparticles conjugated with contact lens solutions may reduce the risk of Acanthamoeba keratitis. Pathogens 10 (5), 583. doi:10.3390/pathogens10050583
Hernandez Montoto, A., Llopis-Lorente, A., Gorbe, M., Terrés, J. M., Cao-Milan, R., Diaz de Grenu, B., et al. (2019). Janus gold nanostars-mesoporous silica nanoparticles for NIR-light-triggered drug delivery. Chemistry 25 (36), 8471–8478. doi:10.1002/chem.201900750
Hernando, S., Nikolakopoulou, P., Voulgaris, D., Hernandez, R. M., Igartua, M., and Herland, A. (2022). Dual effect of TAT functionalized DHAH lipid nanoparticles with neurotrophic factors in human BBB and microglia cultures. Fluids Barriers CNS 19 (1), 22. doi:10.1186/s12987-022-00315-1
Huguet-Casquero, A., Xu, Y., Gainza, E., Pedraz, J. L., and Beloqui, A. (2020). Oral delivery of oleuropein-loaded lipid nanocarriers alleviates inflammation and oxidative stress in acute colitis. Int. J. Pharm. 586, 119515. doi:10.1016/j.ijpharm.2020.119515
Inchaurraga, L., Martinez-Lopez, A. L., Martin-Arbella, N., and Irache, J. M. (2020). Zein-based nanoparticles for the oral delivery of insulin. Drug Deliv. Transl. Res. 10 (6), 1601–1611. doi:10.1007/s13346-020-00796-3
Iturrioz-Rodriguez, N., Correa-Duarte, M. A., and Fanarraga, M. L. (2019). <p>Controlled drug delivery systems for cancer based on mesoporous silica nanoparticles</p>. Int. J. Nanomedicine 14, 3389–3401. doi:10.2147/ijn.s198848
Iturrioz-Rodriguez, N., Gonzalez-Dominguez, E., Gonzalez-Lavado, E., Marin-Caba, L., Vaz, B., Perez-Lorenzo, M., et al. (2017). A biomimetic escape strategy for cytoplasm invasion by synthetic particles. Angew. Chem. Int. Ed. Engl. 56 (44), 13924–13928. doi:10.1002/ange.201707769
Jaque, D., Martinez Maestro, L., del Rosal, B., Haro-Gonzalez, P., Benayas, A., Plaza, J. L., et al. (2014). Nanoparticles for photothermal therapies. Nanoscale 6 (16), 9494–9530. doi:10.1039/c4nr00708e
Jia, C. J., Sun, L. D., Luo, F., Han, X. D., Heyderman, L. J., Yan, Z. G., et al. (2008). Large-scale synthesis of single-crystalline iron oxide magnetic nanorings. J. Am. Chem. Soc. 130 (50), 16968–16977. doi:10.1021/ja805152t
Jimenez-Falcao, S., de Luis, B., Garcia-Fernandez, A., Llopis-Lorente, A., Diez, P., Sanchez, A., et al. (2019). Glucose-responsive enzyme-controlled mesoporous nanomachine with a layer-by-layer supramolecular architecture. ACS Appl. Bio Mater 2 (8), 3321–3328. doi:10.1021/acsabm.9b00338
Jindal, A. B. (2017). The effect of particle shape on cellular interaction and drug delivery applications of micro- and nanoparticles. Int. J. Pharm. 532 (1), 450–465. doi:10.1016/j.ijpharm.2017.09.028
Jornet-Martinez, N., Hakobyan, L., Argente-Garcia, A. I., Molins-Legua, C., and Campins-Falco, P. (2019). Nylon-supported plasmonic assay based on the aggregation of silver nanoparticles: In situ determination of hydrogen sulfide-like compounds in breath samples as a proof of concept. ACS Sens. 4 (8), 2164–2172. doi:10.1021/acssensors.9b01019
Khan, I., Saeed, K., and Khan, I. (2019). Nanoparticles: Properties, applications and toxicities. Arabian J. Chem. 12 (7), 908–931. doi:10.1016/j.arabjc.2017.05.011
Kim, D., Kim, J., Park, Y. I., Lee, N., and Hyeon, T. (2018). Recent development of inorganic nanoparticles for biomedical imaging. ACS Cent. Sci. 4 (3), 324–336. doi:10.1021/acscentsci.7b00574
Kim, W. J., Kwon, Y. J., Cho, C. H., Ye, S. K., and Kim, K. O. (2021). Insulin smart drug delivery nanoparticles of aminophenylboronic acid-POSS molecule at neutral pH. Sci. Rep. 11 (1), 21894. doi:10.1038/s41598-021-01216-3
Kolosnjaj-Tabi, J., Marangon, I., Nicolas-Boluda, A., Silva, A. K. A., and Gazeau, F. (2017). Nanoparticle-based hyperthermia, a local treatment modulating the tumor extracellular matrix. Pharmacol. Res. 126, 123–137. doi:10.1016/j.phrs.2017.07.010
Lafuente-Gomez, N., Wang, S., Fontana, F., Dhanjani, M., Garcia-Soriano, D., Correia, A., et al. (2022). Synergistic immunomodulatory effect in macrophages mediated by magnetic nanoparticles modified with miRNAs. Nanoscale 14 (31), 11129–11138. doi:10.1039/d2nr01767a
Lasa-Saracibar, B., El Moukhtari, S. H., Tsotakos, T., Xanthopoulos, S., Loudos, G., Bouziotis, P., et al. (2022). In vivo biodistribution of edelfosine-loaded lipid nanoparticles radiolabeled with Technetium-99 m: Comparison of administration routes in mice. Eur. J. Pharm. Biopharm. 175, 1–6. doi:10.1016/j.ejpb.2022.04.007
Latorre, A., Latorre, A., Castellanos, M., Lafuente-Gomez, N., Diaz, C. R., Crespo-Barreda, A., et al. (2021). Albumin-based nanostructures for uveal melanoma treatment. Nanomedicine 35, 102391. doi:10.1016/j.nano.2021.102391
Latorre, A., Latorre, A., Castellanos, M., Rodriguez Diaz, C., Lazaro-Carrillo, A., Aguado, T., et al. (2019). Multifunctional albumin-stabilized gold nanoclusters for the reduction of cancer stem cells. Cancers (Basel) 11 (7), 969. doi:10.3390/cancers11070969
Latorre, J., Diaz-Trelles, R., Comas, F., Gavalda-Navarro, A., Milbank, E., Dragano, N., et al. (2022). Downregulation of hepatic lipopolysaccharide binding protein improves lipogenesis-induced liver lipid accumulation. Mol. Ther. Nucleic Acids 29, 599–613. doi:10.1016/j.omtn.2022.08.003
Lazaro-Carrillo, A., Calero, M., Aires, A., Simoes, B. M., Latorre, A., et al. (2020). Tailored functionalized magnetic nanoparticles to target breast cancer cells including cancer stem-like cells. Cancers (Basel) 12 (6), 1397. doi:10.3390/cancers12061397
Leal, B. H., Velasco, B., Cambon, A., Pardo, A., Fernandez-Vega, J., Arellano, L., et al. (2022). Combined therapeutics for atherosclerosis treatment using polymeric nanovectors. Pharmaceutics 14 (2), 258. doi:10.3390/pharmaceutics14020258
Ledo, A. M., Sasso, M. S., Bronte, V., Marigo, I., Boyd, B. J., Garcia-Fuentes, M., et al. (2019). Co-delivery of RNAi and chemokine by polyarginine nanocapsules enables the modulation of myeloid-derived suppressor cells. J. Control Release 295, 60–73. doi:10.1016/j.jconrel.2018.12.041
Lee, S., Son, B., Park, G., Kim, H., Kang, H., Jeon, J., et al. (2018). Immunogenic effect of hyperthermia on enhancing radiotherapeutic efficacy. Int. J. Mol. Sci. 19 (9), 2795. doi:10.3390/ijms19092795
Leiva, M. C., Ortiz, R., Contreras-Caceres, R., Perazzoli, G., Mayevych, I., Lopez-Romero, J. M., et al. (2017). Tripalmitin nanoparticle formulations significantly enhance paclitaxel antitumor activity against breast and lung cancer cells in vitro. Sci. Rep. 7 (1), 13506. doi:10.1038/s41598-017-13816-z
Litti, L., Trivini, S., Ferraro, D., and Reguera, J. (2021). 3D printed microfluidic device for magnetic trapping and SERS quantitative evaluation of environmental and biomedical analytes. ACS Appl. Mater Interfaces 13 (29), 34752–34761. doi:10.1021/acsami.1c09771
Liu, K., and Huang, X. (2022). Synthesis of self-assembled hyaluronan based nanoparticles and their applications in targeted imaging and therapy. Carbohydr. Res. 511, 108500. doi:10.1016/j.carres.2022.108500
Liz-Marzán, L. M., Nel, A. E., Brinker, C. J., Chan, W. C. W., Chen, C., Chen, X., et al. (2022). What do we mean when we say nanomedicine? ACS Nano 16 (9), 13257–13259. doi:10.1021/acsnano.2c08675
Llinas, M. C., Martinez-Edo, G., Cascante, A., Porcar, I., Borros, S., and Sanchez-Garcia, D. (2018). Preparation of a mesoporous silica-based nano-vehicle for dual DOX/CPT pH-triggered delivery. Drug Deliv. 25 (1), 1137–1146. doi:10.1080/10717544.2018.1472678
Lopez, V., Villegas, M. R., Rodriguez, V., Villaverde, G., Lozano, D., Baeza, A., et al. (2017). Janus mesoporous silica nanoparticles for dual targeting of tumor cells and mitochondria. ACS Appl. Mater Interfaces 9 (32), 26697–26706. doi:10.1021/acsami.7b06906
Lozano-Pedraza, C., Plaza-Mayoral, E., Espinosa, A., Sot, B., Serrano, A., Salas, G., et al. (2021). Assessing the parameters modulating optical losses of iron oxide nanoparticles under near infrared irradiation. Nanoscale Adv. 3 (22), 6490–6502. doi:10.1039/d1na00601k
Luengo, Y., Diaz-Riascos, Z. V., Garcia-Soriano, D., Teran, F. J., Artes-Ibanez, E. J., Ibarrola, O., et al. (2022). Fine control of in vivo magnetic hyperthermia using iron oxide nanoparticles with different coatings and degree of aggregation. Pharmaceutics 14 (8), 1526. doi:10.3390/pharmaceutics14081526
Luengo, Y., Sot, B., and Salas, G. (2020). Combining Ag and gamma-Fe2O3 properties to produce effective antibacterial nanocomposites. Colloids Surf. B Biointerfaces 194, 111178. doi:10.1016/j.colsurfb.2020.111178
Manzano, M., and Vallet-Regi, M. (2018). Mesoporous silica nanoparticles in nanomedicine applications. J. Mater Sci. Mater Med. 29 (5), 65. doi:10.1007/s10856-018-6069-x
Manzano, M., and Vallet-Regi, M. (2019). Ultrasound responsive mesoporous silica nanoparticles for biomedical applications. Chem. Commun. (Camb) 55 (19), 2731–2740. doi:10.1039/c8cc09389j
Marciello, M., Rossi, S., Caramella, C., and Remunan-Lopez, C. (2017). Freeze-dried cylinders carrying chitosan nanoparticles for vaginal peptide delivery. Carbohydr. Polym. 170, 43–51. doi:10.1016/j.carbpol.2017.04.051
Márquez-Rodas, I., Dutriaux, C., Saiag, P., de la Cruz Merino, L., Castanon Álvarez, E., Robert, C., et al. (2022). Abstract CT014: Efficacy of intratumoral BO-112 with systemic pembrolizumab in patients with advanced melanoma refractory to anti-PD-1-based therapy: Final results of SPOTLIGHT203 phase 2 study. Cancer Res. 82, CT014. doi:10.1158/1538-7445.am2022-ct014
Martin-Rapun, R., De Matteis, L., Ambrosone, A., Garcia-Embid, S., Gutierrez, L., and de la Fuente, J. M. (2017). Targeted nanoparticles for the treatment of alzheimer's disease. Curr. Pharm. Des. 23 (13), 1927–1952. doi:10.2174/1381612822666161226151011
Martin-Saavedra, F., Ruiz-Hernandez, E., Escudero-Duch, C., Prieto, M., Arruebo, M., Sadeghi, N., et al. (2017). Lipogels responsive to near-infrared light for the triggered release of therapeutic agents. Acta Biomater. 61, 54–65. doi:10.1016/j.actbio.2017.08.010
Martinez-Carmona, M., Izquierdo-Barba, I., Colilla, M., and Vallet-Regi, M. (2019). Concanavalin A-targeted mesoporous silica nanoparticles for infection treatment. Acta Biomater. 96, 547–556. doi:10.1016/j.actbio.2019.07.001
Martinez-Carmona, M., Lozano, D., Colilla, M., and Vallet-Regi, M. (2018). Lectin-conjugated pH-responsive mesoporous silica nanoparticles for targeted bone cancer treatment. Acta Biomater. 65, 393–404. doi:10.1016/j.actbio.2017.11.007
Martinez-Edo, G., Xue, E. Y., Ha, S. Y. Y., Ponton, I., Gonzalez-Delgado, J. A., Borros, S., et al. (2021). Nanoparticles for triple drug release for combined chemo- and photodynamic therapy. Chemistry 27 (59), 14610–14618. doi:10.1002/chem.202101842
Martinez-Lopez, A. L., Gonzalez-Navarro, C. J., Vizmanos, J. L., and Irache, J. M. (2021). Zein-based nanocarriers for the oral delivery of insulin. in vivo evaluation in Caenorhabditis elegans. Drug Deliv. Transl. Res. 11 (2), 647–658. doi:10.1007/s13346-021-00919-4
Martinez-Negro, M., Guerrero-Martinez, A., Garcia-Rio, L., Domenech, O., Aicart, E., Tros de Ilarduya, C., et al. (2018). Multidisciplinary approach to the transfection of plasmid DNA by a nonviral nanocarrier based on a gemini-bolaamphiphilic hybrid lipid. ACS Omega 3 (1), 208–217. doi:10.1021/acsomega.7b01657
Martinez-Relimpio, A. M., Benito, M., Perez-Izquierdo, E., Teijon, C., Olmo, R. M., and Blanco, M. D. (2021). Paclitaxel-loaded folate-targeted albumin-alginate nanoparticles crosslinked with ethylenediamine. Synthesis and in vitro characterization. Polym. (Basel) 13 (13), 2083. doi:10.3390/polym13132083
Mayol, B., Diez, P., Sanchez, A., de la Torre, C., Villalonga, A., Lucena-Sanchez, E., et al. (2021). A glutathione disulfide-sensitive Janus nanomachine controlled by an enzymatic AND logic gate for smart delivery. Nanoscale 13 (44), 18616–18625. doi:10.1039/d0nr08282a
Mazario, E., Herrasti, P., Morales, M. P., and Menendez, N. (2012). Synthesis and characterization of CoFe2O4 ferrite nanoparticles obtained by an electrochemical method. Nanotechnology 23 (35), 355708. doi:10.1088/0957-4484/23/35/355708
McGillicuddy, E., Murray, I., Kavanagh, S., Morrison, L., Fogarty, A., Cormican, M., et al. (2017). Silver nanoparticles in the environment: Sources, detection and ecotoxicology. Sci. Total Environ. 575, 231–246. doi:10.1016/j.scitotenv.2016.10.041
Mejias, R., Hernandez Flores, P., Talelli, M., Tajada-Herraiz, J. L., Brollo, M. E. F., Portilla, Y., et al. (2019). Cell-promoted nanoparticle aggregation decreases nanoparticle-induced hyperthermia under an alternating magnetic field independently of nanoparticle coating, core size, and subcellular localization. ACS Appl. Mater Interfaces 11 (1), 340–355. doi:10.1021/acsami.8b18451
Mendez-Gonzalez, D., Lifante, J., Zabala Gutierrez, J., Marin, R., Ximendes, E., Sanz-de Diego, E., et al. (2022). Optomagnetic nanofluids for controlled brain hyperthermia: a critical study. Nanoscale 14 (43), 16208–16219. doi:10.1039/d2nr03413a
Milan Rois, P., Latorre, A., Rodriguez Diaz, C., Del Moral, A., and Somoza, A. (2018). Reprogramming cells for synergistic combination therapy with nanotherapeutics against uveal melanoma. Biomimetics (Basel) 3 (4), 28. doi:10.3390/biomimetics3040028
Miron-Barroso, S., Domenech, E. B., and Trigueros, S. (2021). Nanotechnology-based strategies to overcome current barriers in gene delivery. Int. J. Mol. Sci. 22 (16), 8537. doi:10.3390/ijms22168537
Mitxelena-Iribarren, O., Hisey, C. L., Errazquin-Irigoyen, M., Gonzalez-Fernandez, Y., Imbuluzqueta, E., Mujika, M., et al. (2017). Effectiveness of nanoencapsulated methotrexate against osteosarcoma cells: In vitro cytotoxicity under dynamic conditions. Biomed. Microdevices 19 (2), 35. doi:10.1007/s10544-017-0177-0
Mitxelena-Iribarren, O., Lizarbe-Sancha, S., Campisi, J., Arana, S., and Mujika, M. (2021). Different microfluidic environments for in vitro testing of lipid nanoparticles against osteosarcoma. Bioeng. (Basel) 8 (6), 77. doi:10.3390/bioengineering8060077
Montalvo-Quiros, S., Gomez-Grana, S., Vallet-Regi, M., Prados-Rosales, R. C., Gonzalez, B., and Luque-Garcia, J. L. (2021). Mesoporous silica nanoparticles containing silver as novel antimycobacterial agents against Mycobacterium tuberculosis. Colloids Surf. B Biointerfaces 197, 111405. doi:10.1016/j.colsurfb.2020.111405
Montalvo-Quiros, S., Vallet-Regi, M., Palacios, A., Anguita, J., Prados-Rosales, R. C., Gonzalez, B., et al. (2020). Mesoporous silica nanoparticles as a potential platform for vaccine development against tuberculosis. Tuberc. Pharm. 12 (12), 1218. doi:10.3390/pharmaceutics12121218
Montero, N., Pérez, E., Benito, M., Teijón, C., Teijón, J. M., Olmo, R., et al. (2019). Biocompatibility studies of intravenously administered ionic-crosslinked chitosan-BSA nanoparticles as vehicles for antitumour drugs. International Journal of Pharmaceutics 554, 337–351. doi:10.1016/j.ijpharm.2018.11.027
Mora-Raimundo, P., Lozano, D., Manzano, M., and Vallet-Regi, M. (2019). Nanoparticles to knockdown osteoporosis-related gene and promote osteogenic marker expression for osteoporosis treatment. ACS Nano 13 (5), 5451–5464. doi:10.1021/acsnano.9b00241
Moran, H. B. T., Turley, J. L., Andersson, M., and Lavelle, E. C. (2018). Immunomodulatory properties of chitosan polymers. Biomaterials 184, 1–9. doi:10.1016/j.biomaterials.2018.08.054
Morey, J., Llinas, P., Bueno-Costa, A., Leon, A. J., and Pina, M. N. (2021). Raltitrexed-modified gold and silver nanoparticles for targeted cancer therapy: Cytotoxicity behavior in vitro on A549 and HCT-116 human cancer cells. Mater. (Basel) 14 (3), 534. doi:10.3390/ma14030534
Moros, M., Idiago-Lopez, J., Asin, L., Moreno-Antolin, E., Beola, L., Grazu, V., et al. (2019). Triggering antitumoural drug release and gene expression by magnetic hyperthermia. Adv. Drug Deliv. Rev. 138, 326–343. doi:10.1016/j.addr.2018.10.004
Mosquera, J., Garcia, I., Henriksen-Lacey, M., Martinez-Calvo, M., Dhanjani, M., Mascarenas, J. L., et al. (2020). Reversible control of protein corona formation on gold nanoparticles using host-guest interactions. ACS Nano 14 (5), 5382–5391. doi:10.1021/acsnano.9b08752
Mulens-Arias, V., Rojas, J. M., Sanz-Ortega, L., Portilla, Y., Perez-Yague, S., and Barber, D. F. (2019). Polyethylenimine-coated superparamagnetic iron oxide nanoparticles impair in vitro and in vivo angiogenesis. Nanomedicine 21, 102063. doi:10.1016/j.nano.2019.102063
Mulvihill, J. J., Cunnane, E. M., Ross, A. M., Duskey, J. T., Tosi, G., and Grabrucker, A. M. (2020). Drug delivery across the blood-brain barrier: Recent advances in the use of nanocarriers. Nanomedicine (Lond). 15 (2), 205–214. doi:10.2217/nnm-2019-0367
Munoz-Espin, D., Rovira, M., Galiana, I., Gimenez, C., Lozano-Torres, B., Paez-Ribes, M., et al. (2018). A versatile drug delivery system targeting senescent cells. EMBO Mol. Med. 10 (9), e9355. doi:10.15252/emmm.201809355
Nanoscience, I. (2020). NoCanTher project 2020. Available from: http://www.nocanther-project.eu/.
Navarro-Palomares, E., Garcia-Hevia, L., Padin-Gonzalez, E., Banobre-Lopez, M., Villegas, J. C., Valiente, R., et al. (2021). Targeting nanomaterials to head and neck cancer cells using a fragment of the Shiga toxin as a potent natural ligand. Cancers (Basel). 13 (19), 4920. doi:10.3390/cancers13194920
Nicu, R., Ciolacu, F., and Ciolacu, D. E. (2021). Advanced functional materials based on nanocellulose for pharmaceutical/medical applications. Pharmaceutics 13 (8), 1125. doi:10.3390/pharmaceutics13081125
Nino-Pariente, A., Arminan, A., Reinhard, S., Scholz, C., Kos, P., Wagner, E., et al. (2017). Design of poly-l-glutamate-based complexes for pDNA delivery. Macromol. Biosci. 17 (10). doi:10.1002/mabi.201700245
Niu, Z., Samaridou, E., Jaumain, E., Coene, J., Ullio, G., Shrestha, N., et al. (2018). PEG-PGA enveloped octaarginine-peptide nanocomplexes: An oral peptide delivery strategy. J. Control Release 276, 125–139. doi:10.1016/j.jconrel.2018.03.004
Niu, Z., Tedesco, E., Benetti, F., Mabondzo, A., Montagner, I. M., Marigo, I., et al. (2017). Rational design of polyarginine nanocapsules intended to help peptides overcoming intestinal barriers. J. Control Release 263, 4–17. doi:10.1016/j.jconrel.2017.02.024
NoCanTher Project (2022). First patient is enrolled in the NoCanTher’s clinical study 2022. Available from: http://www.nocanther-project.eu/index.php/news/70-first-patient-is-enrolled-in-the-nocanther-s-clinical-study.
Nozal, V., Rojas-Prats, E., Maestro, I., Gil, C., Perez, D. I., and Martinez, A. (2021). Improved controlled release and brain penetration of the small molecule S14 using PLGA nanoparticles. Int. J. Mol. Sci. 22 (6), 3206. doi:10.3390/ijms22063206
Ortgies, D. H., de la Cueva, L., Del Rosal, B., Sanz-Rodriguez, F., Fernandez, N., Iglesias-de la Cruz, M. C., et al. (2016). In vivo deep tissue fluorescence and magnetic imaging employing hybrid nanostructures. ACS Appl. Mater Interfaces 8 (2), 1406–1414. doi:10.1021/acsami.5b10617
Ovejero, J. G., Armenia, I., Serantes, D., Veintemillas-Verdaguer, S., Zeballos, N., Lopez-Gallego, F., et al. (2021). Selective magnetic nanoheating: Combining iron oxide nanoparticles for multi-hot-spot induction and sequential regulation. Nano Lett. 21 (17), 7213–7220. doi:10.1021/acs.nanolett.1c02178
Ovejero, J. G., Spizzo, F., Morales, M. P., and Del Bianco, L. (2021). Mixing iron oxide nanoparticles with different shape and size for tunable magneto-heating performance. Nanoscale 13 (11), 5714–5729. doi:10.1039/d0nr09121a
Padzik, M., Hendiger, E. B., Chomicz, L., Grodzik, M., Szmidt, M., Grobelny, J., et al. (2018). Tannic acid-modified silver nanoparticles as a novel therapeutic agent against Acanthamoeba. Parasitol. Res. 117 (11), 3519–3525. doi:10.1007/s00436-018-6049-6
Pallares, V., Nunez, Y., Sanchez-Garcia, L., Falgas, A., Serna, N., Unzueta, U., et al. (2021). Antineoplastic effect of a diphtheria toxin-based nanoparticle targeting acute myeloid leukemia cells overexpressing CXCR4. J. Control Release 335, 117–129. doi:10.1016/j.jconrel.2021.05.014
Pallares, V., Unzueta, U., Falgas, A., Sanchez-Garcia, L., Serna, N., Gallardo, A., et al. (2020). An Auristatin nanoconjugate targeting CXCR4+ leukemic cells blocks acute myeloid leukemia dissemination. J. Hematol. Oncol. 13 (1), 36. doi:10.1186/s13045-020-00863-9
Pardo, A., Pelaz, B., Gallo, J., Bañobre-López, M., Parak, W. J., Barbosa, S., et al. (2020). Synthesis, characterization, and evaluation of superparamagnetic doped ferrites as potential therapeutic nanotools. Chem. Mater. 32 (6), 2220–2231. doi:10.1021/acs.chemmater.9b04848
Paris, J. L., Villaverde, G., Gomez-Grana, S., and Vallet-Regi, M. (2020). Nanoparticles for multimodal antivascular therapeutics: Dual drug release, photothermal and photodynamic therapy. Acta Biomater. 101, 459–468. doi:10.1016/j.actbio.2019.11.004
Pastor, M., Basas, J., Vairo, C., Gainza, G., Moreno-Sastre, M., Gomis, X., et al. (2019). Safety and effectiveness of sodium colistimethate-loaded nanostructured lipid carriers (SCM-NLC) against P. aeruginosa: In vitro and in vivo studies following pulmonary and intramuscular administration. Nanomedicine 18, 101–111. doi:10.1016/j.nano.2019.02.014
Paterson, S., Thompson, S. A., Wark, A. W., and de la RicaGold, R. S. (2017). Gold suprashells: Enhanced photothermal nanoheaters with multiple localized surface plasmon resonances for broadband surface-enhanced Raman scattering. J. Phys. Chem. C 121 (13), 7404–7411. doi:10.1021/acs.jpcc.6b12792
Paul, W., and Sharma, C. P. (2020). “13 - inorganic nanoparticles for targeted drug delivery,” in Biointegration of medical implant materials. Editor C. P. Sharma Second Edition (Woodhead Publishing), 333–373.
Pelaez, E. C., Estevez, M. C., Portela, A., Salvador, J. P., Marco, M. P., and Lechuga, L. M. (2018). Nanoplasmonic biosensor device for the monitoring of acenocoumarol therapeutic drug in plasma. Biosens. Bioelectron. 119, 149–155. doi:10.1016/j.bios.2018.08.011
Pellico, J., Fernandez-Barahona, I., Ruiz-Cabello, J., Gutierrez, L., Munoz-Hernando, M., Sanchez-Guisado, M. J., et al. (2021). HAP-multitag, a PET and positive MRI contrast nanotracer for the longitudinal characterization of vascular calcifications in atherosclerosis. ACS Appl. Mater Interfaces 13 (38), 45279–45290. doi:10.1021/acsami.1c13417
Pena-Gonzalez, C. E., Pedziwiatr-Werbicka, E., Shcharbin, D., Guerrero-Beltran, C., Abashkin, V., Loznikova, S., et al. (2017). Gold nanoparticles stabilized by cationic carbosilane dendrons: Synthesis and biological properties. Dalton Trans. 46 (27), 8736–8745. doi:10.1039/c6dt03791g
Perez-Hernandez, M., Moros, M., Stepien, G., Del Pino, P., Menao, S., de Las Heras, M., et al. (2017). Multiparametric analysis of anti-proliferative and apoptotic effects of gold nanoprisms on mouse and human primary and transformed cells, biodistribution and toxicity in vivo. Part Fibre Toxicol. 14 (1), 41. doi:10.1186/s12989-017-0222-4
Perez-Tanoira, R., Fernandez-Arias, M., Potel, C., Carballo-Fernandez, R., Perez-Castro, S., Boutinguiza, M., et al. (2022). Silver nanoparticles produced by laser ablation and Re-irradiation are effective preventing peri-implantitis multispecies biofilm formation. Int. J. Mol. Sci. 23 (19), 12027. doi:10.3390/ijms231912027
Plaza-Ga, I., Manzaneda-Gonzalez, V., Kisovec, M., Almendro-Vedia, V., Munoz-Ubeda, M., Anderluh, G., et al. (2019). pH-triggered endosomal escape of pore-forming Listeriolysin O toxin-coated gold nanoparticles. J. Nanobiotechnology 17 (1), 108. doi:10.1186/s12951-019-0543-6
Polo, E., del Pino, P., Pardo, A., Taboada, P., and Pelaz, B. (2018). “Magnetic nanoparticles for cancer therapy and bioimaging,” in Nanooncology: Engineering nanomaterials for cancer therapy and diagnosis. Editors G. Gonçalves, and G. Tobias (Cham: Springer International Publishing), 239–279.
Portela, A., Calvo-Lozano, O., Estevez, M. C., Medina Escuela, A., and Lechuga, L. M. (2020). Optical nanogap antennas as plasmonic biosensors for the detection of miRNA biomarkers. J. Mater Chem. B 8 (19), 4310–4317. doi:10.1039/d0tb00307g
Poyatos-Racionero, E., Gonzalez-Alvarez, I., Sanchez-Moreno, P., Sitia, L., Gatto, F., Pompa, P. P., et al. (2021). Lactose-Gated mesoporous silica particles for intestinal controlled delivery of essential oil components: An in vitro and in vivo study. Pharmaceutics 13 (7), 982. doi:10.3390/pharmaceutics13070982
Prajapati, R., Garcia-Garrido, E., and Somoza, A. (2021). Albumin-based nanoparticles for the delivery of doxorubicin in breast cancer. Cancers (Basel) 13 (12), 3011. doi:10.3390/cancers13123011
Prajapati, R., and Somoza, A. (2021). Albumin nanostructures for nucleic acid delivery in cancer: Current trend, emerging issues, and possible solutions. Cancers (Basel). 13 (14), 3454. doi:10.3390/cancers13143454
Qaiser, A., Kiani, M. H., Parveen, R., Sarfraz, M., Shahnaz, G., Rahdar, A., et al. (2022). Design and synthesis of multifunctional polymeric micelles for targeted delivery in Helicobacter pylori infection. J. Mol. Liq. 363, 119802. doi:10.1016/j.molliq.2022.119802
Quintanilla, M., Garcia, I., de Lazaro, I., Garcia-Alvarez, R., Henriksen-Lacey, M., Vranic, S., et al. (2019). Thermal monitoring during photothermia: Hybrid probes for simultaneous plasmonic heating and near-infrared optical nanothermometry. Theranostics 9 (24), 7298–7312. doi:10.7150/thno.38091
Ramirez-Jimenez, R., Artiga, A., Mitchell, S. G., Martin-Rapun, R., and de la Fuente, J. M. (2020). Surfactant-free synthesis and scalable purification of triangular gold nanoprisms with low non-specific cellular uptake. Nanomater. (Basel) 10 (3), 539. doi:10.3390/nano10030539
Ramos-Docampo, M. A., Fernandez-Medina, M., Taipaleenmaki, E., Hovorka, O., Salgueirino, V., and Stadler, B. (2019). Microswimmers with heat delivery capacity for 3D cell spheroid penetration. ACS Nano 13 (10), 12192–12205. doi:10.1021/acsnano.9b06869
Reboredo, C., Gonzalez-Navarro, C. J., Martinez-Oharriz, C., Martinez-Lopez, A. L., and Irache, J. M. (2021). Preparation and evaluation of PEG-coated zein nanoparticles for oral drug delivery purposes. Int. J. Pharm. 597, 120287. doi:10.1016/j.ijpharm.2021.120287
Redolfi Riva, E., Pastoriza-Santos, I., Lak, A., Pellegrino, T., Perez-Juste, J., and Mattoli, V. (2017). Plasmonic/magnetic nanocomposites: Gold nanorods-functionalized silica coated magnetic nanoparticles. J. Colloid Interface Sci. 502, 201–209. doi:10.1016/j.jcis.2017.04.089
Reidy, B., Haase, A., Luch, A., Dawson, K. A., and Lynch, I. (2013). Mechanisms of silver nanoparticle release, transformation and toxicity: A critical review of current knowledge and recommendations for future studies and applications. Mater. (Basel) 6 (6), 2295–2350. doi:10.3390/ma6062295
Relano-Rodriguez, I., Espinar-Buitrago, M. S., Martin-Canadilla, V., Gomez-Ramirez, R., Jimenez, J. L., and Munoz-Fernandez, M. A. (2021). Nanotechnology against human cytomegalovirus in vitro: Polyanionic carbosilane dendrimers as antiviral agents. J. Nanobiotechnology 19 (1), 65. doi:10.1186/s12951-021-00809-4
Rincon-Iglesias, M., Rodrigo, I. L. B. B., Serea, E. S. A., Plazaola, F., Lanceros-Mendez, S., et al. (2022). Core-shell Fe(3)O(4)@Au nanorod-loaded gels for tunable and anisotropic magneto- and photothermia. ACS Appl. Mater Interfaces 14 (5), 7130–7140. doi:10.1021/acsami.1c20990
Ritz, S., Schottler, S., Kotman, N., Baier, G., Musyanovych, A., Kuharev, J., et al. (2015). Protein corona of nanoparticles: Distinct proteins regulate the cellular uptake. Biomacromolecules 16 (4), 1311–1321. doi:10.1021/acs.biomac.5b00108
Roca, A. G., Gutierrez, L., Gavilan, H., Fortes Brollo, M. E., Veintemillas-Verdaguer, S., and Morales, M. D. P. (2019). Design strategies for shape-controlled magnetic iron oxide nanoparticles. Adv. Drug Deliv. Rev. 138, 68–104. doi:10.1016/j.addr.2018.12.008
Rocha, U., Hu, J., Rodriguez, E. M., Vanetsev, A. S., Rahn, M., Sammelselg, V., et al. (2016). Subtissue imaging and thermal monitoring of gold nanorods through joined encapsulation with Nd-doped infrared-emitting nanoparticles. Small 12 (39), 5394–5400. doi:10.1002/smll.201600866
Rodriguez, M. J., Ramos-Soriano, J., Perkins, J. R., Mascaraque, A., Torres, M. J., Gomez, F., et al. (2019). Glycosylated nanostructures in sublingual immunotherapy induce long-lasting tolerance in LTP allergy mouse model. Sci. Rep. 9 (1), 4043. doi:10.1038/s41598-019-40114-7
Rojas-Prats, E., Tosat-Bitrian, C., Martinez-Gonzalez, L., Nozal, V., Perez, D. I., and Martinez, A. (2021). Increasing brain permeability of PHA-767491, a cell division cycle 7 kinase inhibitor, with biodegradable polymeric nanoparticles. Pharmaceutics 13 (2), 180. doi:10.3390/pharmaceutics13020180
Romero, G., and Moya, S. E. (2012). “Chapter 4 - synthesis of organic nanoparticles,” in Frontiers of nanoscience. Editors J. M. de la Fuente, and V. Grazu (Elsevier), 4, 115–141.
Rubia-Rodriguez, I., Santana-Otero, A., Spassov, S., Tombacz, E., Johansson, C., De La Presa, P., et al. (2021). Whither magnetic hyperthermia? A Tentat. Roadmap. Mater. (Basel) 14 (4), 706. doi:10.3390/ma14040706
Rubia-Rodriguez, I., Zilberti, L., Arduino, A., Bottauscio, O., Chiampi, M., and Ortega, D. (2021). In silico assessment of collateral eddy current heating in biocompatible implants subjected to magnetic hyperthermia treatments. Int. J. Hyperth. 38 (1), 846–861. doi:10.1080/02656736.2021.1909758
Rubio-Canalejas, A., Baelo, A., Herbera, S., Blanco-Cabra, N., Vukomanovic, M., and Torrents, E. (2022). 3D spatial organization and improved antibiotic treatment of a Pseudomonas aeruginosa-Staphylococcus aureus wound biofilm by nanoparticle enzyme delivery. Front. Microbiol. 13, 959156. doi:10.3389/fmicb.2022.959156
Sabio, L., Sosa, A., Delgado-Lopez, J. M., and Dominguez-Vera, J. M. (2021). Two-sided antibacterial cellulose combining probiotics and silver nanoparticles. Molecules 26 (10), 2848. doi:10.3390/molecules26102848
Saha, K., Agasti, S. S., Kim, C., Li, X., and Rotello, V. M. (2012). Gold nanoparticles in chemical and biological sensing. Chem. Rev. 112 (5), 2739–2779. doi:10.1021/cr2001178
Samaridou, E., Walgrave, H., Salta, E., Alvarez, D. M., Castro-Lopez, V., Loza, M., et al. (2020). Nose-to-brain delivery of enveloped RNA - cell permeating peptide nanocomplexes for the treatment of neurodegenerative diseases. Biomaterials 230, 119657. doi:10.1016/j.biomaterials.2019.119657
Sanchez-Arribas, N., Diaz-Nunez, P., Osio Barcina, J., Aicart, E., Junquera, E., and Guerrero-Martinez, A. (2021). Controlled pDNA release in gemini cationic lipoplexes by femtosecond laser irradiation of gold nanostars. Nanomater. (Basel) 11 (6), 1498. doi:10.3390/nano11061498
Sanchez-Arribas, N., Martinez-Negro, M., Villar, E. M., Perez, L., Aicart, E., Taboada, P., et al. (2020). Biocompatible nanovector of siRNA consisting of arginine-based cationic lipid for gene knockdown in cancer cells. ACS Appl. Mater Interfaces 12 (31), 34536–34547. doi:10.1021/acsami.0c06273
Sanchez-Arribas, N., Martinez-Negro, M., Villar, E. M., Perez, L., Osio Barcina, J., Aicart, E., et al. (2020). Protein expression knockdown in cancer cells induced by a gemini cationic lipid nanovector with histidine-based polar heads. Pharmaceutics 12 (9), 791. doi:10.3390/pharmaceutics12090791
Sanchez-Garcia, L., Sala, R., Serna, N., Alamo, P., Parlade, E., Alba-Castellon, L., et al. (2020). A refined cocktailing of pro-apoptotic nanoparticles boosts anti-tumor activity. Acta Biomater. 113, 584–596. doi:10.1016/j.actbio.2020.06.033
Sanchez-Garcia, L., Serna, N., Alamo, P., Sala, R., Cespedes, M. V., Roldan, M., et al. (2018). Self-assembling toxin-based nanoparticles as self-delivered antitumoral drugs. J. Control Release 274, 81–92. doi:10.1016/j.jconrel.2018.01.031
Sanchez-Lopez, E., Gomara, M. J., and Haro, I. (2021). Nanotechnology-based platforms for vaginal delivery of peptide microbicides. Curr. Med. Chem. 28 (22), 4356–4379. doi:10.2174/0929867328666201209095753
Sans-Serramitjana, E., Jorba, M., Fuste, E., Pedraz, J. L., Vinuesa, T., and Vinas, M. (2017). Free and nanoencapsulated tobramycin: Effects on planktonic and biofilm forms of Pseudomonas. Microorganisms 5 (3), 35. doi:10.3390/microorganisms5030035
Sans-Serramitjana, E., Jorba, M., Pedraz, J. L., Vinuesa, T., and Vinas, M. (2017). Determination of the spatiotemporal dependence of <em>Pseudomonas aeruginosa</em> biofilm viability after treatment with NLC-colistin. Int. J. Nanomedicine 12, 4409–4413. doi:10.2147/ijn.s138763
Santalices, I., Vazquez-Vazquez, C., Santander-Ortega, M. J., Lozano, V., Araujo, F., Sarmento, B., et al. (2021). A nanoemulsion/micelles mixed nanosystem for the oral administration of hydrophobically modified insulin. Drug Deliv. Transl. Res. 11 (2), 524–545. doi:10.1007/s13346-021-00920-x
Santos, H. D. A., Zabala Gutierrez, I., Shen, Y., Lifante, J., Ximendes, E., Laurenti, M., et al. (2020). Ultrafast photochemistry produces superbright short-wave infrared dots for low-dose in vivo imaging. Nat. Commun. 11 (1), 2933. doi:10.1038/s41467-020-16333-2
Sanz, B., Calatayud, M. P., Torres, T. E., Fanarraga, M. L., Ibarra, M. R., and Goya, G. F. (2017). Magnetic hyperthermia enhances cell toxicity with respect to exogenous heating. Biomaterials 114, 62–70. doi:10.1016/j.biomaterials.2016.11.008
Sanz-Ortega, L., Portilla, Y., Perez-Yague, S., and Barber, D. F. (2019). Magnetic targeting of adoptively transferred tumour-specific nanoparticle-loaded CD8(+) T cells does not improve their tumour infiltration in a mouse model of cancer but promotes the retention of these cells in tumour-draining lymph nodes. J. Nanobiotechnology 17 (1), 87. doi:10.1186/s12951-019-0520-0
Sanz-Ortega, L., Rojas, J. M., Marcos, A., Portilla, Y., Stein, J. V., and Barber, D. F. (2019). T cells loaded with magnetic nanoparticles are retained in peripheral lymph nodes by the application of a magnetic field. J. Nanobiotechnology 17 (1), 14. doi:10.1186/s12951-019-0440-z
Sanz-Ortega, L., Rojas, J. M., Portilla, Y., Perez-Yague, S., and Barber, D. F. (2019). Magnetic nanoparticles attached to the NK cell surface for tumor targeting in adoptive transfer therapies does not affect cellular effector functions. Front. Immunol. 10, 2073. doi:10.3389/fimmu.2019.02073
Sapareto, S. A., and Dewey, W. C. (1984). Thermal dose determination in cancer therapy. Int. J. Radiat. Oncol. Biol. Phys. 10 (6), 787–800. doi:10.1016/0360-3016(84)90379-1
Saravani, R., Sargazi, S., Saravani, R., Rabbani, M., Rahdar, A., and Taboada, P. (2020). Newly crocin-coated magnetite nanoparticles induce apoptosis and decrease VEGF expression in breast carcinoma cells. J. Drug Deliv. Sci. Technol. 60, 101987. doi:10.1016/j.jddst.2020.101987
Sartaj, A., Qamar, Z., Qizilbash, F. F., Annu, Md S., Alhakamy, N. A., et al. (2021). Polymeric nanoparticles: Exploring the current drug development and therapeutic insight of breast cancer treatment and recommendations. Polym. (Basel) 13 (24), 4400. doi:10.3390/polym13244400
Sayed, F. N., and Polshettiwar, V. (2015). Facile and sustainable synthesis of shaped iron oxide nanoparticles: Effect of iron precursor salts on the shapes of iron oxides. Sci. Rep. 5, 9733. doi:10.1038/srep09733
Serrano-Sevilla, I., Artiga, A., Mitchell, S. G., De Matteis, L., and de la Fuente, J. M. (2019). Natural polysaccharides for siRNA delivery: Nanocarriers based on chitosan, hyaluronic acid, and their derivatives. Molecules 24 (14), 2570. doi:10.3390/molecules24142570
Sharma, G., and Jeevanandam, P. (2013). Synthesis of self-assembled prismatic iron oxide nanoparticles by a novel thermal decomposition route. RSC Adv. 3 (1), 189–200. doi:10.1039/c2ra22004k
Shi, L., Zhang, J., Zhao, M., Tang, S., Cheng, X., Zhang, W., et al. (2021). Effects of polyethylene glycol on the surface of nanoparticles for targeted drug delivery. Nanoscale 13 (24), 10748–10764. doi:10.1039/d1nr02065j
Shokrani, H., Shokrani, A., Sajadi, S. M., Khodadadi Yazdi, M., Seidi, F., Jouyandeh, M., et al. (2022). Polysaccharide-based nanocomposites for biomedical applications: A critical review. Nanoscale Horiz. 7, 1136–1160. doi:10.1039/d2nh00214k
Silvan, J. M., Zorraquin-Pena, I., Gonzalez de Llano, D., Moreno-Arribas, M. V., and Martinez-Rodriguez, A. J. (2018). Antibacterial activity of glutathione-stabilized silver nanoparticles against Campylobacter multidrug-resistant strains. Front. Microbiol. 9, 458. doi:10.3389/fmicb.2018.00458
Simeonidis, K., Morales, M. P., Marciello, M., Angelakeris, M., de la Presa, P., Lazaro-Carrillo, A., et al. (2016). In-situ particles reorientation during magnetic hyperthermia application: Shape matters twice. Sci. Rep. 6, 38382. doi:10.1038/srep38382
Soenen, S. J., Parak, W. J., Rejman, J., and Manshian, B. (2015). (Intra)cellular stability of inorganic nanoparticles: Effects on cytotoxicity, particle functionality, and biomedical applications. Chem. Rev. 115 (5), 2109–2135. doi:10.1021/cr400714j
Soler-Agesta, R., Guerrero-Ochoa, P., Marco-Brualla, J., Ibanez-Perez, R., Marzo, I., Martinez-Lostao, L., et al. (2022). Conjugation of the 9-kDa isoform of granulysin with liposomes potentiates its cytotoxicity. Int. J. Mol. Sci. 23 (15), 8705. doi:10.3390/ijms23158705
Soprano, E., Alvarez, A., Pelaz, B., Del Pino, P., and Polo, E. (2020). Plasmonic cell-derived nanocomposites for light-controlled cargo release inside living cells. Adv. Biosyst. 4 (3), e1900260. doi:10.1002/adbi.201900260
Spanish Scientific Network HiperNano (2018). Spanish scientific Network HiperNano (RED2018-102626-T).
Sperling, R. A., Rivera Gil, P., Zhang, F., Zanella, M., and Parak, W. J. (2008). Biological applications of gold nanoparticles. Chem. Soc. Rev. 37 (9), 1896–1908. doi:10.1039/b712170a
Spilde, M. N., Northup, D. E., Boston, P. J., Schelble, R. T., Dano, K. E., Crossey, L. J., et al. (2005). Geomicrobiology of cave ferromanganese deposits: A field and laboratory investigation. Geomicrobiol. J. 22 (3-4), 99–116. doi:10.1080/01490450590945889
Staka, I., Cadete, A., Surikutchi, B. T., Abuzaid, H., Bradshaw, T. D., Alonso, M. J., et al. (2019). A novel low molecular weight nanocomposite hydrogel formulation for intra-tumoural delivery of anti-cancer drugs. Int. J. Pharm. 565, 151–161. doi:10.1016/j.ijpharm.2019.04.070
Stepien, G., Moros, M., Perez-Hernandez, M., Monge, M., Gutierrez, L., Fratila, R. M., et al. (2018). Effect of surface chemistry and associated protein corona on the long-term biodegradation of iron oxide nanoparticles in vivo. ACS Appl. Mater Interfaces 10 (5), 4548–4560. doi:10.1021/acsami.7b18648
Storozhylova, N., Crecente-Campo, J., Cabaleiro, D., Lugo, L., Dussouy, C., Simões, S., et al. (2020). An in situ hyaluronic acid-fibrin hydrogel containing drug-loaded nanocapsules for intra-articular treatment of inflammatory joint diseases. Regen. Eng. Transl. Med. 6 (2), 201–216. doi:10.1007/s40883-020-00154-2
Sune-Pou, M., Prieto-Sanchez, S., El Yousfi, Y., Boyero-Corral, S., Nardi-Ricart, A., Nofrerias-Roig, I., et al. (2018). Cholesteryl oleate-loaded cationic solid lipid nanoparticles as carriers for efficient gene-silencing therapy. Int. J. Nanomedicine 13, 3223–3233. doi:10.2147/ijn.s158884
Carregal-Romero, S., Groult, H., Cañadas, O., A-Gonzalez, N., LechugaVieco, A. V., García-Fojeda, B., et al. (2021). Delayed alveolar clearance of nanoparticles through control of coating composition and interaction with lung surfactant protein A. Materials Science and Engineering: C 134, 112551. doi:10.1016/j.msec.2021.112551
Teijeiro-Valino, C., Novoa-Carballal, R., Borrajo, E., Vidal, A., Alonso-Nocelo, M., de la Fuente Freire, M., et al. (2019). A multifunctional drug nanocarrier for efficient anticancer therapy. J. Control Release 294, 154–164. doi:10.1016/j.jconrel.2018.12.002
Tenchov, R., Bird, R., Curtze, A. E., and Zhou, Q. (2021). Lipid nanoparticles-from liposomes to mRNA vaccine delivery, a landscape of research diversity and advancement. ACS Nano 15, 16982–17015. doi:10.1021/acsnano.1c04996
Therapeutics, H. (2020). BO-112 with pembrolizumab in unresectable malignant melanoma (SPOTLIGHT203) clinicaltrials.gov2020. Available from: https://clinicaltrials.gov/ct2/show/NCT04570332.
Therapeutics, H. (2016). Exploratory study of BO-112 in adult patients with aggressive solid tumors clinicaltrials gov. [Available from: https://clinicaltrials.gov/ct2/show/NCT02828098.
Thompson, S. A., Paterson, S., Azab, M. M., Wark, A. W., and de la Rica, R. (2017). Light-triggered inactivation of enzymes with photothermal nanoheaters. Small 13 (15), 1603195. doi:10.1002/smll.201603195
Thwala, L. N., Delgado, D. P., Leone, K., Marigo, I., Benetti, F., Chenlo, M., et al. (2018). Protamine nanocapsules as carriers for oral peptide delivery. J. Control Release 291, 157–168. doi:10.1016/j.jconrel.2018.10.022
Tomsen-Melero, J., Passemard, S., Garcia-Aranda, N., Diaz-Riascos, Z. V., Gonzalez-Rioja, R., Nedergaard Pedersen, J., et al. (2021). Impact of chemical composition on the nanostructure and biological activity of alpha-galactosidase-loaded nanovesicles for fabry disease treatment. ACS Appl. Mater Interfaces 13 (7), 7825–7838. doi:10.1021/acsami.0c16871
Torrecilla, J., Gomez-Aguado, I., Vicente-Pascual, M., Del Pozo-Rodriguez, A., Solinis, M. A., and Rodriguez-Gascon, A. (2019). MMP-9 downregulation with lipid nanoparticles for inhibiting corneal neovascularization by gene silencing. Nanomater. (Basel) 9 (4), 631. doi:10.3390/nano9040631
Tortiglione, C., and de la Fuente, J. M. (2019). Synthesis of gold nanoparticles for gene silencing. Methods Mol. Biol. 1974, 203–214. doi:10.1007/978-1-4939-9220-1_15
Trabulo, S., Aires, A., Aicher, A., Heeschen, C., and Cortajarena, A. L. (2017). Multifunctionalized iron oxide nanoparticles for selective targeting of pancreatic cancer cells. Biochim. Biophys. Acta Gen. Subj. 1861 (6), 1597–1605. doi:10.1016/j.bbagen.2017.01.035
Vairo, C., Basas, J., Pastor, M., Palau, M., Gomis, X., Almirante, B., et al. (2020). In vitro and in vivo antimicrobial activity of sodium colistimethate and amikacin-loaded nanostructured lipid carriers (NLC). Nanomedicine 29, 102259. doi:10.1016/j.nano.2020.102259
Vallet-Regi, M., Colilla, M., Izquierdo-Barba, I., and Manzano, M. (2017). Mesoporous silica nanoparticles for drug delivery: Current insights. Molecules 23 (1), 47. doi:10.3390/molecules23010047
Vallet-Regi, M., Gonzalez, B., and Izquierdo-Barba, I. (2019). Nanomaterials as promising alternative in the infection treatment. Int. J. Mol. Sci. 20 (15), 3806. doi:10.3390/ijms20153806
Vallet-Regi, M. (2022). Our contributions to applications of mesoporous silica nanoparticles. Acta Biomater. 137, 44–52. doi:10.1016/j.actbio.2021.10.011
Vallet-Regi, M., Rámila, A., del Real, R. P., and Pérez-Pariente, J. (2001). A new property of MCM-41: Drug delivery system. Chem. Mater. 13 (2), 308–311. doi:10.1021/cm0011559
Vallet-Regi, M., Schuth, F., Lozano, D., Colilla, M., and Manzano, M. (2022). Engineering mesoporous silica nanoparticles for drug delivery: Where are we after two decades? Chem. Soc. Rev. 51 (13), 5365–5451. doi:10.1039/d1cs00659b
Varela-Fernandez, R., Garcia-Otero, X., Diaz-Tome, V., Regueiro, U., Lopez-Lopez, M., Gonzalez-Barcia, M., et al. (2022). Lactoferrin-loaded nanostructured lipid carriers (NLCs) as a new formulation for optimized ocular drug delivery. Eur. J. Pharm. Biopharm. 172, 144–156. doi:10.1016/j.ejpb.2022.02.010
Vargas-Nadal, G., Munoz-Ubeda, M., Alamo, P., Mitjans, M., Cespedes, V., Kober, M., et al. (2020). MKC-quatsomes: A stable nanovesicle platform for bio-imaging and drug-delivery applications. Nanomedicine 24, 102136. doi:10.1016/j.nano.2019.102136
Vicente-Pascual, M., Gomez-Aguado, I., Rodriguez-Castejon, J., Rodriguez-Gascon, A., Muntoni, E., Battaglia, L., et al. (2020). Topical administration of SLN-based gene therapy for the treatment of corneal inflammation by de novo IL-10 production. Pharmaceutics 12 (6), 584. doi:10.3390/pharmaceutics12060584
Vilaboa, N., Bore, A., Martin-Saavedra, F., Bayford, M., Winfield, N., Firth-Clark, S., et al. (2017). New inhibitor targeting human transcription factor HSF1: Effects on the heat shock response and tumor cell survival. Nucleic Acids Res. 45 (10), 5797–5817. doi:10.1093/nar/gkx194
Villar-Alvarez, E., Cambon, A., Pardo, A., Arellano, L., Marcos, A. V., Pelaz, B., et al. (2019). Combination of light-driven co-delivery of chemodrugs and plasmonic-induced heat for cancer therapeutics using hybrid protein nanocapsules. J. Nanobiotechnology 17 (1), 106. doi:10.1186/s12951-019-0538-3
Villar-Alvarez, E., Cambon, A., Pardo, A., Mosquera, V. X., Bouzas-Mosquera, A., Topete, A., et al. (2018). Gold nanorod-based nanohybrids for combinatorial therapeutics. ACS Omega 3 (10), 12633–12647. doi:10.1021/acsomega.8b01591
Villaverde, G., Gómez-Graña, S., Guisasola, E., García, I., Hanske, C., Liz-Marzán, L. M., et al. (2018). Targeted chemo-photothermal therapy: A nanomedicine approximation to selective melanoma treatment. Part. Part. Syst. Charact. 35 (7), 1800148. doi:10.1002/ppsc.201800148
Villegas, M. R., Baeza, A., and Vallet-Regi, M. (2018). Nanotechnological strategies for protein delivery. Molecules 23 (5), 1008. doi:10.3390/molecules23051008
Vinuesa, T., Herraez, R., Oliver, L., Elizondo, E., Acarregui, A., Esquisabel, A., et al. (2017). Benznidazole nanoformulates: A chance to improve therapeutics for Chagas disease. Am. J. Trop. Med. Hyg. 97 (5), 1469–1476. doi:10.4269/ajtmh.17-0044
Vivo-Llorca, G., Morella-Aucejo, A., Garcia-Fernandez, A., Diez, P., Llopis-Lorente, A., Orzaez, M., et al. (2022). Horseradish peroxidase-functionalized gold nanoconjugates for breast cancer treatment based on enzyme prodrug therapy. Int. J. Nanomedicine 17, 409–422. doi:10.2147/ijn.s323802
Volta-Duran, E., Serna, N., Sanchez-Garcia, L., Avino, A., Sanchez, J. M., Lopez-Laguna, H., et al. (2021). Design and engineering of tumor-targeted, dual-acting cytotoxic nanoparticles. Acta Biomater. 119, 312–322. doi:10.1016/j.actbio.2020.11.018
Wolfram, J., and Ferrari, M. (2019). Clinical cancer nanomedicine. Nano Today 25, 85–98. doi:10.1016/j.nantod.2019.02.005
Ximendes, E., Marin, R., Shen, Y., Ruiz, D., Gomez-Cerezo, D., Rodriguez-Sevilla, P., et al. (2021). Infrared-emitting multimodal nanostructures for controlled in vivo magnetic hyperthermia. Adv. Mater 33 (30), e2100077. doi:10.1002/adma.202100077
Ximendes, E. C., Rocha, U., Sales, T. O., Fernández, N., Sanz-Rodríguez, F., Martín, I. R., et al. (2017). In vivo subcutaneous thermal video recording by supersensitive infrared nanothermometers. Adv. Funct. Mater. 27 (38), 1702249. doi:10.1002/adfm.201702249
Xuan, M., Mestre, R., Gao, C., Zhou, C., He, Q., and Sanchez, S. (2018). Noncontinuous super-diffusive dynamics of a light-activated nanobottle motor. Angew. Chem. Int. Ed. Engl. 57 (23), 6954–6958. doi:10.1002/ange.201801910
Zamora-Perez, P., Pelaz, B., Tsoutsi, D., Soliman, M. G., Parak, W. J., and Rivera-Gil, P. (2021). Hyperspectral-enhanced dark field analysis of individual and collective photo-responsive gold-copper sulfide nanoparticles. Nanoscale 13 (31), 13256–13272. doi:10.1039/d0nr08256b
Keywords: nanoparticles, therapy, Spain, nanotechnology, nanomedicine
Citation: Fernández-Gómez P, Pérez de la Lastra Aranda C, Tosat-Bitrián C, Bueso de Barrio JA, Thompson S, Sot B, Salas G, Somoza Á, Espinosa A, Castellanos M and Palomo V (2023) Nanomedical research and development in Spain: improving the treatment of diseases from the nanoscale. Front. Bioeng. Biotechnol. 11:1191327. doi: 10.3389/fbioe.2023.1191327
Received: 21 March 2023; Accepted: 23 May 2023;
Published: 21 July 2023.
Edited by:
Yuce Li, Sungkyunkwan University, Republic of KoreaReviewed by:
Leopoldo Sitia, University of Milan, ItalyCopyright © 2023 Fernández-Gómez, Pérez de la Lastra Aranda, Tosat-Bitrián, Bueso de Barrio, Thompson, Sot, Salas, Somoza, Espinosa, Castellanos and Palomo. This is an open-access article distributed under the terms of the Creative Commons Attribution License (CC BY). The use, distribution or reproduction in other forums is permitted, provided the original author(s) and the copyright owner(s) are credited and that the original publication in this journal is cited, in accordance with accepted academic practice. No use, distribution or reproduction is permitted which does not comply with these terms.
*Correspondence: Valle Palomo, dmFsbGUucGFsb21vQGltZGVhLm9yZw==
†These authors share first authorship
Disclaimer: All claims expressed in this article are solely those of the authors and do not necessarily represent those of their affiliated organizations, or those of the publisher, the editors and the reviewers. Any product that may be evaluated in this article or claim that may be made by its manufacturer is not guaranteed or endorsed by the publisher.
Research integrity at Frontiers
Learn more about the work of our research integrity team to safeguard the quality of each article we publish.