- 1Department of Pharmaceutics, Faculty of Pharmaceutical Sciences, Government College University Faisalabad, Faisalabad, Pakistan
- 2Department of Pharmacy, The University of Faisalabad, Faisalabad, Pakistan
- 3Foundation University and Medical College Islamabad Department of Medicine, Islamabad, Pakistan
- 4Hamdard Institute of Pharmaceutical Sciences, Hamdard University Karachi, Islamabad Campus, Islamabad, Pakistan
- 5Center of Innovation in Personalized Medicine (CIPM), 3D Bioprinting Unit, King Abdulaziz University, Jeddah, Saudi Arabia
- 6Department of Pharmaceutics, Faculty of Pharmacy, King Abdulaziz University, Jeddah, Saudi Arabia
- 7Department of Chemical Laboratories, King Abdulaziz Medical City, Jeddah, Saudi Arabia
- 8Department of Medical Laboratory Sciences, College of Applied Medical Sciences, Prince Sattam Bin Abdulaziz University, Al-Kharj, Saudi Arabia
- 9Department of Pharmaceutics, College of Pharmacy, Jazan University, Jazan, Saudi Arabia
Hydrogels are crosslinked three-dimensional networks, and their properties can be easily tuned to target the various segments of the gastrointestinal tract (GIT). Cetirizine HCl (CTZ HCl) is an antihistaminic drug, which when given orally can upset the stomach. Moreover, this molecule has shown maximum absorption in the intestine. To address these issues, we developed a pH-responsive semi-interpenetrating polymer network (semi-IPN) for the delivery of CTZ HCl to the lower part of the GIT. Initially, 10 different formulations of itaconic acid-grafted-poly (acrylamide)/aloe vera [IA-g-poly (AAm)/aloe vera] semi-IPN were developed by varying the concentration of IA and aloe vera using the free radical polymerization technique. Based on swelling and sol-gel analysis, formulation F5 containing 0.3%w/w aloe vera and 6%w/w IA was chosen as the optimum formulation. The solid-state characterization of the optimized formulation (F5) revealed a successful incorporation of CTZ HCl in semi-IPN without any drug-destabilizing interaction. The in vitro drug release from F5 showed limited release in acidic media followed by a controlled release in the intestinal environment for over 72 h. Furthermore, during the in vivo evaluation, formulation F5 did not affect the hematological parameters, kidney, and liver functions. Clinical observations did not reveal any signs of illness in rabbits treated with hydrogels. Histopathological images of vital organs of treated animals showed normal cellular architecture. Thus, the results suggest a non-toxic nature and overall potential of the developed formulation as a targeted drug carrier.
1 Introduction
Drug carriers are intended for the safe and effective delivery of therapeutically active molecules to enhance their solubility, chemical stability, bioavailability, targetability, and pharmacological activity, as well as to reduce their side effects. In recent years, crosslinked polymeric carriers such as hydrogels have gained remarkable attention for the controlled and targeted delivery of the drug (Damiri et al., 2020). Hydrogels are three-dimensional networks where polymers are crosslinked via covalent bonding or are fastened together by intermolecular interactions. They possess a tremendous potential to hold or absorb large amounts of fluids within their interstitial spaces. However, homopolymer-based hydrogels have shown poor mechanical strength that leads to compromised structural integrity and burst release of entrapped molecules. Since 2000, scientists have been trying to overcome these limitations which can be easily overcome by using semi-IPN (Gong et al., 2003; Sedlačík et al., 2020). These networks are composed of two polymers, where the linear polymer penetrates the crosslinked network.
Polysaccharide-based hydrogels are widely engaged as drug carriers. According to existing literature, many natural polysaccharides (Bera et al., 2015) like aloe vera, starch, chitosan (Shalviri et al., 2010; Bajer et al., 2020), guar gum (George and Abraham, 2007), and Gum ghatti (Sharma et al., 2014a) have been used for the development of hydrogels. Aloe vera is a natural polymer that was used in the current study owing to ease of availability, biodegradability, economy, and biocompatibility. Several authors have highlighted the importance of aloe vera in different areas of the biomedical field owing to the presence of biologically active components. In tissue engineering, aloe vera has received considerable attention due to its biodegradability, biocompatibility, and low toxicity (Rahman et al., 2017). Aloe vera contains several amino acids, vitamins C and E, and zinc which play an important role in stimulating accelerated wound healing (Alven et al., 2021; Khan et al., 2023). It has also been investigated for the development of various drug carriers such as gels (Rompicherla et al., 2022), film (Hajian et al., 2017), sustained-release tablets (Laux et al., 2019), and microparticles (Pereira et al., 2014). It has been observed that blending aloe vera with other polymers not only increases their biocompatibility but also substantially improves the mechanical integrity of hydrogel-based matrices and films (Dey et al., 2015). Aloe vera is preferred over other polysaccharides owing to its high water-absorbing capacity and ease of modification/grafting due to the large number of functional groups in its backbone. Studies have demonstrated that the fluid-absorption capacity and mechanical strength of aloe vera can be further improved by grafting other monomers (Kumar et al., 2018).
Itaconic acid (IA) is an unsaturated dicarbonic acid where one carboxyl (COOH-) is linked with the ethylene group (Kumar et al., 2017). Acrylamide (Aam) is a white, colorless, and odorless monomer with good aqueous solubility (Faroon et al., 2009). Poly (AAm)-based hydrogels have been extensively studied owing to their excellent swelling capabilities (Jayaramudu et al., 2019), thermal stability (Zhang et al., 2022), and biocompatibility (Subramani et al., 2020). These properties are further improved by combining them with other polymers. The properties of polymeric blends or copolymers rely on the interactions between their components (Torres-Figueroa et al., 2020). IA was used to develop pH-sensitive hydrogels with many other monomers, e.g., poly (acrylic acid-co-IA) (Katime and Rodriguez, 2001) and poly (N-vinyl caprolactam-co-IA) (Cavus and Cakal, 2012). We came across other studies where AAm, IA, and other monomers were used in various combinations to develop pH-responsive hydrogels (Bera et al., 2015; Quintana et al., 2002; Katime et al., 2001; Caykara et al., 2004). Therefore, we aimed to formulate pH-sensitive semi-IPN hydrogels based on aloe vera, Aam, and IA for the site-specific delivery of Cetirizine HCl (CTZ HCl). Moreover, developed networks were extensively characterized by various in vitro and in vivo tests.
2 Materials and method
Aloe vera and acrylamide were purchased from Daejung reagents chemicals and metals Co., Ltd., Korea. Itaconic acid and cetirizine HCl were purchased from Shenyang polychemicals co., Ltd., China, and Remington Pharmaceutical Industries (Pvt) Ltd., Lahore, Pakistan, respectively. Ammonium persulfate and ethylene glycol dimethacrylate were procured from Sigma Aldrich. All other chemicals used were of analytical grade.
2.1 Development of IA-g-poly (AAm)/aloe vera
Different formulations of pH-sensitive semi-IPN hydrogels were prepared by using IA and aloe vera with a fixed concentration of crosslinkers (Table 1) via the free radical polymerization technique. In Brief, separate solutions of IA, Aam, and ammonium persulfate (APS) were prepared in deionized water with constant stirring at room temperature. To create free radicals, the initiator solution was added to the solution of aloe vera, while ethylene glycol dimethacrylate (EGDMA) was added to the AAm solution. Afterward, these two solutions were mixed, and IA was added dropwise at room temperature with continuous stirring to obtain a uniform solution. Subsequently, the resultant solution was sonicated (JP030S; Skymen Cleaning Equipment Shenzhen Co. Ltd., China) for 5 min to remove air bubbles. Then, the transparent solution was transferred to glass test tubes and deoxygenated with nitrogen gas. Tightly covered test tubes were placed in a pre-heated water bath maintained at 45°C to avoid bubble formation with a heating sequence at 45°C (2 hours), at 50°C (2 hours), at 55°C (2 hours), at 60°C (overnight), and further at 65°C (2 hours). Finally, the test tubes were removed from the water bath and were cooled at room temperature. Firm and cylindrical hydrogels were removed from the test tubes and cut into 8 mm discs with a sharp blade (Abbasi et al., 2019). Obtained hydrogel discs were washed with water and ethanol mixture (50:50). Finally, these discs were dried at room temperature and stored in a desiccator until further testing.
2.2 Swelling
For the optimization and determination of the pH-sensitive behavior of developed semi-IPN hydrogels, the swelling of blank and drug-loaded hydrogels was assessed by immersing the pre-weighed hydrogels in buffer solutions (pH 7.4 and pH 1.2). Hydrogels were removed at different time intervals, and the weights of swollen hydrogel discs were measured. The swelling index was calculated using the following formula (n = 3),
“Wt” refers to the final weight of hydrogels at specified time intervals, and “Wo” refers to the initial weight of dried hydrogels (Wei et al., 2021).
2.3 Sol-gel fraction
To evaluate non-crosslinked polymeric content, sol-gel analysis was performed. Pre-weighed dried blank IA-g-poly (AAm)/aloe vera semi-IPN hydrogels were soaked in deionized water for 7 days with frequent shaking. Distilled water was replaced with fresh distilled water after 2 days. After 7 days, the swollen hydrogels were removed and dried at room temperature. The weight of the dried hydrogels was noted (Sohail et al., 2014). The following equations were employed to estimate the sol-gel percentage.
Where “Wo” and “Wd” are the weights of dried hydrogels before and after polymer extraction, respectively.
2.4 Solid state characterization
Fourier transform infrared spectrometry (FTIR) analysis was employed to identify functional groups and possible chemical interactions between drugs and hydrogels. All samples were scanned from 4,000 to 500 cm−1 on Bruker FTIR. Scanning electron microscopy (SEM) was used to analyze the surface morphology of blank, pure drug, and drug-loaded semi-IPN hydrogels. To analyze the thermal properties and stabilities of blank, pure drug, and drug-loaded hydrogels, differential scanning calorimetry (DSC) and thermogravimetric analysis (TGA) were simultaneously performed on the SDT Q600 Series Thermal Analysis system at 15°C–400 C with a heating rate of 10 C/min in the presence of nitrogen stream. XRD was employed for the analysis of the physical state of CTZ HCl and hydrogels. All samples were scanned over a range of 5°C–90°C (Ahmad et al., 2018; Zahra et al., 2021; Shoukat et al., 2022).
2.5 Drug loading
CTZ HCl was loaded in an optimized formulation by employing the disc diffusion method. In brief, hydrogel discs were soaked in 100 mL of 1% w/v solution of CTZ HCl in a USP phosphate buffer of pH 7.4. These hydrogel discs were removed from the buffer solution once equilibrium was achieved. Excess drug solution from the hydrogel surface was removed with blotting paper, and the hydrogel was dried to attain a constant weight.
2.6 Drug content
Different methods were adopted to evaluate drug loading in the optimized semi-IPN hydrogels. Firstly, using the weight variation method, drug content was estimated as follows (Ranjha et al., 2016).
Where “Wo” and Wd are the initial and final weights of dried discs before and after drug loading, respectively. Secondly, by the extraction procedure, drug content was estimated by putting the weighed quantity of CTZ HCl-loaded discs in PBS pH 7.4. Every time, 25 mL of fresh PBS was added until no drug was detected in the pooled buffer solution. The amount of drug was estimated from the calibration curve, which was previously constructed on the UV spectrophotometer at λmax of 231 nm (Afzal et al., 2018).
2.7 Drug release
In vitro CTZ HCl release was monitored for 2 h at pH 1.2 (simulated gastric residence time) and in the USP buffer of pH 7.4 for 72 h in the USP type II apparatus at 37°C ± 0.5°C and 50 rpm (Afzal et al., 2018). At different time intervals, 5 mL of the sample was collected and replaced with a fresh buffer of a specified pH to maintain sink conditions. Then, the samples were analyzed at 231 nm on the UV spectrophotometer to estimate drug concentration (Khan et al., 2011). Afterward, cumulative drug release (Eq. 6) was evaluated, and the Korsmeyer Peppas model (Eq. 7) was applied to understand the mechanism of drug release.
Here, “Mt” refers to the amount of drug release at time “t”, “Mo” refers to the quantity of drug release at infinity, “KKP” is the release rate constant, and “n” is the diffusional coefficient.
2.8 Toxicity testing
We used the fixed-dose guideline number 420 for toxicity testing as set by The Organization for Economic Co-Operation and Development (OECD). All the protocols were approved by the Ethical Committee of GCUF, vide letter number GCUF/ERC/2153. Rabbits in the control group only received water and food, while the treated group received 2 g/kg of the optimized formulation (F5). All the physical changes, mortality rate, variation in body weight, and water and food consumption were monitored for 14 days. Blood samples were assessed for any changes in hematological and biochemical parameters. Afterward, the animals were sacrificed; their vital organs were weighed and fixed in a 10% v/v phosphate-buffered neutral formalin. Later, thin slices of their organs were stained with hematoxylin and eosin (H&E) for tissue analysis.
3 Results and discussion
Initially, 10 different formulations were developed by varying the concentrations of aloe vera, followed by changing the concentrations of IA. The possible structure of a semi-IPN hydrogel is shown in Figure 1. Subsequently, swelling behavior and sol-gel contents were investigated to select an optimum formulation.
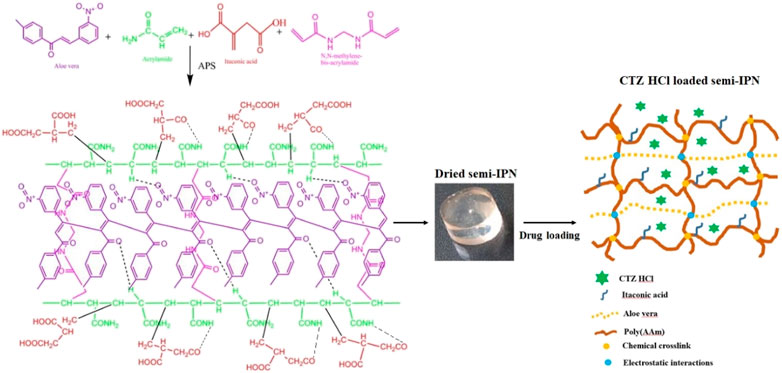
FIGURE 1. The graphical presentation of the initial ingredients, possible structure, and loading of CTZ HCl in IA-g-poly (AAm)/aloe vera semi-IPN hydrogel.
3.1 Swelling behavior
To explore the influence of the pH of the media on the water absorption and swelling behavior, prepared semi-IPN hydrogels were immersed in 0.1 M HCl (pH 1.2) and a buffer solution of pH 7.4. Their appearance in the dry form and media of different pH are shown in Figure 2.
3.1.1 Effect of aloe vera
Hydrogels immersed in acidic media presented the lowest water absorption owing to the protonation of the carboxylic groups. Here, the pH of the media was below the pKa of the ionizable groups of the aloe vera, resulting in a low swelling behavior. Contrarily, hydrogels immersed in a buffer of pH 7.4 exhibited higher water absorption and swelling, as shown in Figure 3A. In this solution, the carboxylic groups of the aloe vera are ionized (COO−) to increase the electrostatic repulsion between adjacent polymeric chains of the network and thus improve the swelling of the hydrogel.
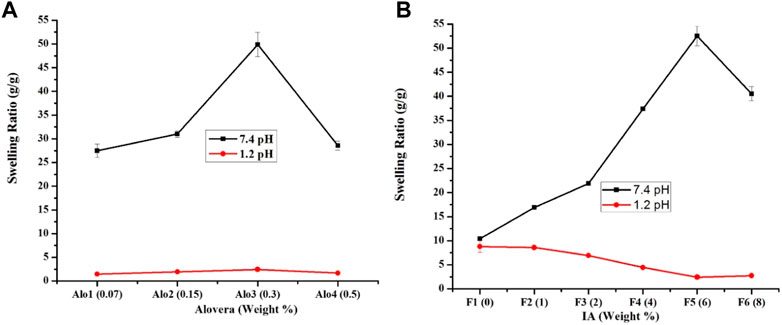
FIGURE 3. The effect of increasing concentrations of aloe vera (A) and IA (B) on the swelling ratio (g/g) of semi-IPN hydrogels.
Furthermore, increasing the concentration of aloe vera in various formulations significantly increased the swelling index of hydrogels. This behavior was attributed to the hydrophilic character of aloe vera. In this study, the hydrophilicity of the network increased proportionally with the concentration of aloe vera (Pereira et al., 2013). According to existing literature, hydrogels with higher aloe vera contents exhibited rapid absorption of water when immersed in a buffer solution of pH 7.4. The authors reported that the degree of swelling was enhanced with higher contents of aloe vera (Park and Nho, 2003). It is a well-known fact that highly crosslinked structures are not able to accommodate a high quantity of fluid within their network. However, the addition of aloe vera loosens the polymer network and increases the fluid uptake capacity (Bialik-Wąs et al., 2020). We further observed that on increasing the aloe vera contents above 0.3% w/w, swelling decreased (Figure 3A). It could be due to (a) increased crosslinking density and (b) a higher concentration; aloe vera might have occupied interstitial spaces and limited the interaction of crosslinked network with the media (Ajaz et al., 2022). After analysis, the formulation with 0.3 %w/w aloe vera (Alo3) was chosen for further testing.
3.1.2 Effect of IA
The formulation with the optimum concentration of aloe vera was further modified with various concentrations of IA (0–8%w/w). Prepared hydrogels showed similar swelling behavior as observed for aloe vera, i.e., a low swelling at pH 1.2 and a higher swelling at pH 7.4 (Figure 3B). At a low pH, the swelling of all formulations decreased, while at a high pH, swelling increased with an increase in the concentration of IA up to 6%w/w. At a higher pH, IA might have contributed to higher concentrations of–COO− ions along with free H+ ions within the hydrogel network. This could have increased the electrostatic repulsion between adjacent polymeric chains and osmotic pressure within the hydrogel to increase the swelling. Afterward, swelling decreased, as shown in Figure 3B, which may be due to the development of a dense polymeric network where swelling and penetration of dissolution media were difficult. Our observations are consistent with previous studies where swelling of hydrogel increased with an increase in the concentration of pH-sensitive monomers up to a certain concentration and then decreased afterward (Ajaz et al., 2021; Rehman et al., 2021).
3.2 Sol-gel fraction
The sol-gel fraction of hydrogels was used to estimate the non-crosslinked fraction of monomers or polymers. On increasing the IA concentrations, gel% increased while sol% decreased, as shown in Table 2. Here, upon increasing the concentration of the monomer, there was a higher crosslinking that ultimately enhanced the gel percent of hydrogels. Previous studies presented similar observations where IA-g-poly (acrylamide)/sterculia gum semi-IPN showed higher gel % with increasing content of monomers (Rehman et al., 2021). Based on swelling and gel content, F5 was chosen as the optimum formulation for further testing and evaluation.
3.3 FTIR
FTIR analysis was used to evaluate any possible drug excipient interactions and to elucidate the network structure. We obtained and analyzed the FTIR spectra of blank, drug-loaded semi-IPN hydrogels, and CTZ HCl (Figure 4). In literature, aloe vera showed absorption bands at 3,390, 2,931, 1719, 1,407, and 1,254 cm−1 that represent -OH group, -CH vibration, -CO linkages, -CH3 vibration, and -C O C groups, respectively (Pereira et al., 2013). IA exhibited hydroxyl group (-OH) peak at 3,432 cm−1, -carbonyl (-C=O) stretch of -COOH group at 1,702 cm−1, and -C=C stretching at 1,631 cm-1. Whereas, AAm demonstrated -CH group stretching at 3,102, 3,034, and 2,813 cm−1, -HN stretching at 3,347 and 3,191 cm−1, and -C = C peak at 1,648 cm−1 (Rehman et al., 2021).
The blank hydrogel spectrum showed characteristic bands at 3,300 cm−1 due to the presence of amino and hydroxyl groups of aloe vera. A shift of peak from 1,425 to 1,417 cm−1 could be due to the incorporation of aloe vera as observed previously (Pereira et al., 2013). Absorption bands in the range of 3,427 to 1,636 cm−1 were attributed to the -OH stretching and -COO. These results for absorption bands are in agreement with previous reports (Pereira et al., 2011). Moreover, the absence of -C = C peaks of Aam and IA at 1,648 and 1,631 cm−1, respectively, indicated the involvement of monomers in the polymerization and grafting of IA onto the polymeric network.
FTIR spectrum of the pure drug showed intense peaks at 757, 1,024, 1,191, and 1,317 cm−1 corresponding to aliphatic chloro compound, alkyl substituted ether, the tertiary amine of piperazine ring of CTZ HCl, and–COOH groups, respectively (Sharma et al., 2014b; Sharma et al., 2015). CTZ HCl peaks remained intact in F5, which indicates the compatibility of the drug with the developed hydrogel.
3.4 SEM
Surface morphology CTZ HCl and drug-loaded and blank semi-IPN hydrogels were analyzed by SEM micrographs. CTZ HCl showed an acicular shape with a smooth surface, partially agglomerated in bundles (Figure 5A). These findings are in agreement with previous observations about the crystalline nature of CTZ HCl (Douroumis et al., 2011). The SEM image of blank hydrogels depicted slightly rough and porous surfaces (Figure 5B), which might be due to the collapse of the polymeric network during drying. These surface pores of the hydrogel network could serve as the entry point for dissolution media. Furthermore, the porous assembly also contributed to a higher swelling ratio of hydrogels. Similar porous surfaces were observed in previous studies (Khalid et al., 2018). On the other hand, F5 showed a slightly rough surface with blocked pores and surface-adhered white particles (Figure 5C). These particles could be drug molecules that migrated to the surface along with dissolution media during drying after drug loading. This also indicates that the drug was uniformly distributed in the hydrogels. Furthermore, the presence of drug particles on the hydrogel surface could contribute to early drug release as observed by other researchers (Jabbar et al., 2021).
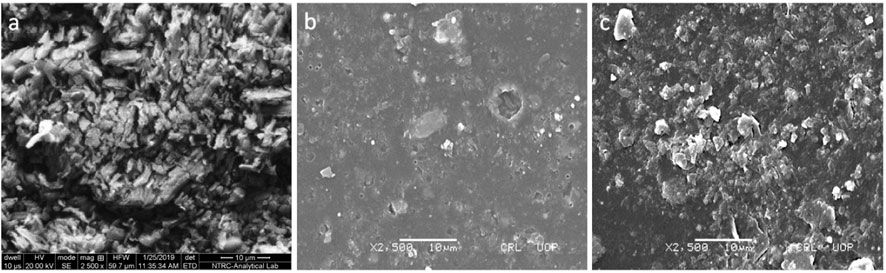
FIGURE 5. The SEM micrograph of (A) CTZ HCl, (B) blank, and (C) drug-loaded semi-IPN hydrogels (F5).
3.5 DSC
The DSC thermogram of blank hydrogels showed a melting peak of IA at approximately 160°C (Figure 6) as reported previously (Kirimura et al., 2011) and poly (acrylamide) above 300°C. Pure CTZ HCl exhibited a melting peak at approximately 201.3°C, followed by a degradation peak at approximately 220°C and is in agreement with previous studies (Katewongsa et al., 2017; Afzal et al., 2018). CTZ HCl peaks diminished in drug-loaded hydrogels (F5) indicating dispersion of the drug in an amorphous form. These results were further confirmed by XRD analysis.
3.6 TGA
TGA thermograms of CTZ HCl and blank and drug-loaded hydrogels are shown in Figure 7, which depicts the impact of thermal variation. The thermal degradation of blank hydrogel was accomplished in two steps. First, weight loss (8%) was observed up to 165°C which could be due to the loss of water molecules. Second, weight loss (42%) was detected between 165°C–00°C due to the degradation of IA chains of the polymer. Similar findings were observed elsewhere (Aghamohamadi et al., 2019) for aloe vera poly (vinylpyrrolidone) or aloe vera acetate poly (vinylpyrrolidone) electrospun fibers. In another study, IA-grafted-poly (AAm)/carbopol semi-IPN networks showed degradation of IA side chains from 165 °C onwards (Ajaz et al., 2021). Pure CTZ HCl showed ∼ 5% weight loss up to 165°C. This weight loss increased (∼92.8%) with the increase in temperature between 247°C–400°C, which corresponds to the thermal degradation of the drug (Sovizi and Hosseini, 2013). In comparison, the drug-loaded hydrogel presented slightly higher thermal stability when compared to the blank hydrogel. First, weight loss (11%) was observed up to 165°C, while 42% loss of weight was observed from 165°C–400°C. Thus, we can conclude that the prepared formulation was stable over a wide range of temperatures. Moreover, the incorporation of the drug did not affect the stability.
3.7 XRD
XRD analysis of CTZ HCl, blank, and drug-loaded hydrogel was carried out to establish the crystalline or amorphous nature. CTZ HCl presented sharp peaks at 8.3°C, 14.8°C, 18.29°C, 18.79°C, 23.97°C, 24.5°C, and 25°C (Figure 8), which indicate crystalline nature. In this literature, it is mentioned that IA is of a crystalline nature with peaks at 18°C, 22°C, 24°C, 30°C, 31°C, 36°C, 39°C, 44°C, 55°C, and 56°C (Sabaa et al., 2017), while poly (AAm) (Kumar et al., 2019) and aloe vera (Gontijo et al., 2013) are amorphous in nature. The intense peaks of CTZ HCl were absent in drug-loaded hydrogels, which indicates the dispersion of the drug at a molecular level. Furthermore, blank and optimized formulations (F5) were comparable. Both displayed broad and dull peaks, indicating the amorphous nature of hydrogels. Such amorphous networks also facilitate water uptake, swelling, and release of API.
3.8 Drug content
CTZ HCl was loaded in the optimum formulation (F5) that displayed maximum swelling at pH 7.4. Drug loading was determined by weight variation and the extraction process (Table 3). Similar patterns of CTZ HCl loading were also observed for gelatin/sodium alginate-based hydrogels (Ranjha et al., 2016).
3.9 Drug release
For developed hydrogels, cumulative % CTZ HCl release was very poor at pH 1.2, which was due to limited swelling of hydrogel in an acidic environment as mentioned in swelling results above. In acidic media, burst release of CTZ HCl (∼32%) was observed during the first 2 hours of the study. This could be due to the presence of CTZ HCl molecules on the surface of hydrogels as verified by SEM images. Moreover, this initial burst release could also be due to high concentration gradients of the drug between the hydrogel exterior and release media, which could be the driving force for CTZ HCl diffusion. Thus, initial burst release was usually established as soon as the hydrogel was placed in the release media (Zhang et al., 2004). Afterward, as the pH of the media was switched to basic, the drug was released in a controlled manner (∼86%) owing to the swelling of the three-dimensional network, which released the deeply-seated drug (Figure 9). At this pH, -COOH groups of the semi-IPN network were ionized, thus causing the electrostatic repulsion of network chains and ingression of dissolution media that not only caused swelling of the network but also caused the dissolution of the drug for constant release at the desired location (Fallon et al., 2019; Ajaz et al., 2021). Hydrogels are generally designed to respond to various physiological changes and, accordingly, alter the release pattern of entrapped drugs. In literature, we have successfully demonstrated that our developed semi-IPN exhibited changes in its swelling behavior, network structure, and permeability with variations in pH to release the drug at the desired location. Existing literature shows that CTZ HCl from IA-g-poly (AAm)/sterculia gum (Rehman et al., 2021) and sulfasalazine loaded in pectin-g-(PEG-co-MAA) pH-sensitive semi-IPN network released trapped molecules at a colonic pH (Abbasi et al., 2019).
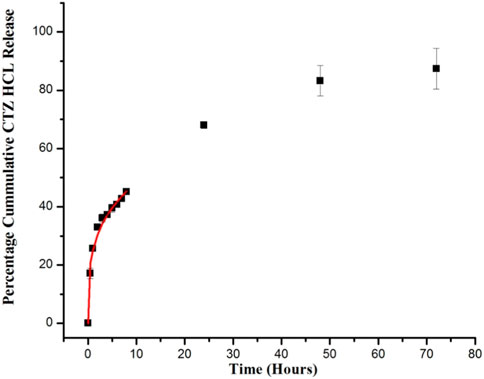
FIGURE 9. In vitro CTZ HCl release from semi-IPN hydrogel (F5). The release curve shows the Korsmeyer Peppas model on the first 60% release (Shown by the red line).
3.10 Drug release kinetics
The Korsmeyer Peppas model was used to elucidate the mechanism of CTZ HCl release from semi-IPN hydrogels. The correlation coefficient (R2) value that was near “1” explains the suitability of the model. For the optimized formulation (F5), the “n” value was below 0.45, indicating the involvement of the Fickian diffusion mechanism (Table 4).
3.11 Toxicity testing
3.11.1 The general condition of animals
During the course of the study, all animals in the control and treated groups were tested for different parameters. We did not observe any abnormal clinical findings (Tables 5, 6), indicating the non-toxic nature of the developed formulation.
3.11.2 Blood analysis
Blood samples of control and tested animals were analyzed to evaluate hematological and biochemical parameters, as shown in Tables 7, 8, respectively. These parameters show that the optimized formulation did not affect the composition of blood as all the values were within the normal range. Moreover, the livers and kidneys were also working normally. This suggests the nontoxicity of the developed formulation.
3.11.3 Histology
After H&E staining of micro sections of vital organs of both groups, slices were observed under the microscope to detect any possible changes in the cellular structure. In comparison, we did not observe any significant changes in the histology between the treated and control groups (Figure 10).
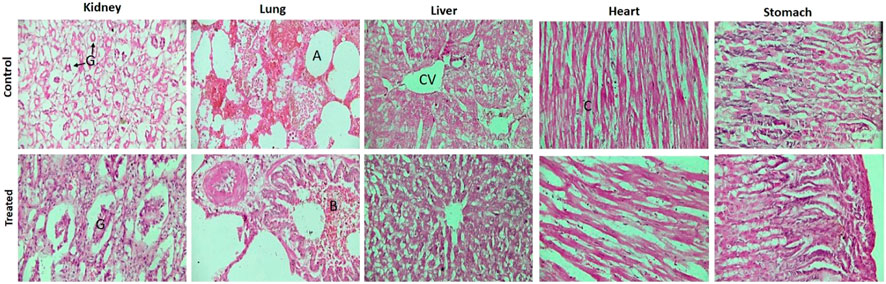
FIGURE 10. Histopathological images of vital organs. All images were acquired at 40x on a Tinocular microscope, Accu-Scope 3,001 fitted with a five-megapixel camera. Here, the alphabets represent, G: glomerulus, B: bronchiole, A: alveolus, CV: central vein, and C: cardiac muscles.
The kidneys of both groups showed intact glomeruli without any signs of necrosis and bleeding. The lungs also presented a normal structure, i.e., bronchiole, alveolus, and blood vessels without any signs of infiltrations, edema, and inflammation. In the liver, hepatocytes were arranged around the central vein. The heart displayed branched-striated myofibers arranged normally without any signs of hemorrhage. The histology of the stomach also presented normal cellular structure, intact mucosa without any signs of ulcer, and any other gastropathy. These findings are consistent with previous findings (Rehman et al., 2021; Ajaz et al., 2022). These findings suggest the biocompatibility and non-toxicity of semi-IPN hydrogels, identifying them as a suitable carrier for use in humans.
4 Conclusion
IA-g-poly (AAm)/aloe vera semi-IPN hydrogels were prepared and characterized by various in vitro and in vivo tests. The swelling of hydrogels was low at acidic pH but increased at higher pH (7.4). Formulation F5 with 0.3% w/w aloe vera, 6% w/w IA, and 20% w/w AAm was considered optimum, owing to better swelling characteristics. The solid-state characterization of the optimized hydrogel confirmed the presence of CTZ HCl in an amorphous form without any interaction with prepared semi-IPN hydrogel. The optimized formulation released limited amounts of the drug at a low pH, while it released maximum amounts of the entrapped drug in a controlled manner at pH 7.4. Hydrogels did not show any toxic effects on vital organs and blood components, and the function of the kidney and liver remained normal. Thus, prepared semi-IPN hydrogels can be deemed as a safe and effective carrier for the targeted delivery of CTZ HCl.
Data availability statement
The raw data supporting the conclusion of this article will be made available by the authors, without undue reservation.
Ethics statement
The animal study was reviewed and approved by Ethical Committee (ERC) of GCUF (Reference no. GCUF/ERC/2153).
Author contributions
All authors listed have made a substantial, direct, and intellectual contribution to the work and approved it for publication.
Funding
The Deanship of Scientific Research (DSR) at King Abdulaziz University, Jeddah, Saudi Arabia, funded this project under grant no (RG-2-166-43).
Conflict of interest
The authors declare that the research was conducted in the absence of any commercial or financial relationships that could be construed as a potential conflict of interest.
Publisher’s note
All claims expressed in this article are solely those of the authors and do not necessarily represent those of their affiliated organizations, or those of the publisher, the editors and the reviewers. Any product that may be evaluated in this article, or claim that may be made by its manufacturer, is not guaranteed or endorsed by the publisher.
References
Abbasi, M., Sohail, M., Minhas, M. U., Khan, S., Hussain, Z., Mahmood, A., et al. (2019). Novel biodegradable pH-sensitive hydrogels: An efficient controlled release system to manage ulcerative colitis. Int. J. Biol. Macromol. 136, 83–96. doi:10.1016/j.ijbiomac.2019.06.046
Afzal, S., Khan, S., Ranjha, N. M., Jalil, A., Riaz, A., Haider, M. S., et al. (2018). The structural, crystallinity, and thermal properties of pH-responsive interpenetrating gelatin/sodium alginate-based polymeric composites for the controlled delivery of cetirizine HCl. Turkish J. Pharm. Sci. 15 (1), 63–76. doi:10.4274/tjps.64326
Aghamohamadi, N., Sanjani, N. S., Majidi, R. F., and Nasrollahi, S. A. (2019). Preparation and characterization of Aloe vera acetate and electrospinning fibers as promising antibacterial properties materials. Mater. Sci. Eng. C 94, 445–452. doi:10.1016/j.msec.2018.09.058
Ahmad, S., Usman Minhas, M., Ahmad, M., Sohail, M., Abdullah, O., and Khan, K. U. (2018). Topical hydrogel patches of vinyl monomers containing mupirocin for skin injuries: Synthesis and evaluation. Adv. Polym. Technol. 37, 3401–3411. doi:10.1002/adv.22124
Ajaz, N., Khan, I. U., Asghar, S., Khalid, S. H., Irfan, M., Asif, M., et al. (2021). Assessing the pH responsive and mucoadhesive behavior of dexamethasone sodium phosphate loaded itaconic acid-grafted-poly(acrylamide)/carbopol semi-interpenetrating networks. J. Polym. Res. 28 (8), 278. doi:10.1007/s10965-021-02643-6
Ajaz, N., Khan, I. U., Irfan, M., Khalid, S. H., Asghar, S., and Mehmood, Y. (2022). In vitro and biological characterization of dexamethasone sodium phosphate laden pH-sensitive and mucoadhesive hydroxy propyl β-Cyclodextrin-g-poly(acrylic acid)/gelatin semi-interpenetrating networks. Gels 8 (5), 290. doi:10.3390/gels8050290
Alven, S., Khwaza, V., Oyedeji, O. O., and Aderibigbe, B. A. (2021). Polymer-based scaffolds loaded with aloe vera extract for the treatment of wounds. Pharmaceutics 13 (7), 961. doi:10.3390/pharmaceutics13070961
Bajer, D., Janczak, K., and Bajer, K. (2020). Novel starch/chitosan/aloe vera composites as promising biopackaging materials. J. Polym. Environ. 28 (3), 1021–1039. doi:10.1007/s10924-020-01661-7
Bera, R., Dey, A., and Chakrabarty, D. (2015). Synthesis, Characterization, and drug release study of acrylamide-co-itaconic acid based smart hydrogel. Polym. Eng. Sci. 55 (1), 113–122. doi:10.1002/pen.23874
Bialik-Wąs, K., Pluta, K., Malina, D., Barczewski, M., Malarz, K., and Mrozek-Wilczkiewicz, A. (2020). Advanced SA/PVA-based hydrogel matrices with prolonged release of Aloe vera as promising wound dressings. Mater. Sci. Eng. C 120, 111667. doi:10.1016/j.msec.2020.111667
Cavus, S., and Cakal, E. (2012). Synthesis and characterization of novel poly (N-vinylcaprolactam-co-itaconic acid) gels and analysis of pH and temperature sensitivity. Industrial Eng. Chem. Res. 51 (3), 1218–1226. doi:10.1021/ie2008746
Caykara, T., Doǧmuş, M., and Kantoǧlu, Ö. (2004). Network structure and swelling–shrinking behaviors of pH-sensitive poly (acrylamide-co-itaconic acid) hydrogels. J. Polym. Sci. Part B Polym. Phys. 42 (13), 2586–2594. doi:10.1002/polb.20129
Damiri, F., Bachra, Y., Bounacir, C., Laaraibi, A., and Berrada, M. (2020). Synthesis and characterization of lyophilized chitosan-based hydrogels cross-linked with benzaldehyde for controlled drug release. J. Chem. 2020, 1–10. doi:10.1155/2020/8747639
Dey, A., Bera, R., and Chakrabarty, D. (2015). Influence of Aloe vera on the properties of N-vinylpyrrolidone-Acrylamide copolymer hydrogel. Mater. Chem. Phys. 168, 168–179. doi:10.1016/j.matchemphys.2015.11.017
Douroumis, D. D., Gryczke, A., and Schminke, S. (2011). Development and evaluation of cetirizine HCl taste-masked oral disintegrating tablets. Aaps Pharmscitech 12 (1), 141–151. doi:10.1208/s12249-010-9569-7
Fallon, M., Halligan, S., Pezzoli, R., Geever, L., and Higginbotham, C. (2019). Synthesis and characterisation of novel temperature and pH sensitive physically cross-linked poly(N-vinylcaprolactam-co-itaconic acid) hydrogels for drug delivery. Gels 5 (3), 41. doi:10.3390/gels5030041
Faroon, O., Mumtaz, M., Ruiz, P., and Wohlers, D. (2009). Draft toxicological profile for acrylamide. CDC stacks 104 (1), 191–238.
George, M., and Abraham, T. (2007). pH sensitive alginate–guar gum hydrogel for the controlled delivery of protein drugs. Int. J. Pharm. 335 (1-2), 123–129. doi:10.1016/j.ijpharm.2006.11.009
Gong, J. P., Katsuyama, Y., Kurokawa, T., and Osada, Y. (2003). Double-network hydrogels with extremely high mechanical strength. Adv. Mater. 15 (14), 1155–1158. doi:10.1002/adma.200304907
Gontijo, S. MdL., Gomes, A. D. M., Gala-García, A., Sinisterra, R. D., and Cortés, M. E. (2013). Evaluation of antimicrobial activity and cell viability of Aloe vera sponges. Electron. J. Biotechnol. 16 (1), 2. doi:10.2225/vol16-issue1-fulltext-2
Hajian, M., Mahmoodi, M., and Imani, R. (2017). In vitro assessment of poly (vinyl alcohol) film incorporating aloe vera for potential application as a wound dressing. J. Macromol. Sci. Part B. 56 (7), 435–450. doi:10.1080/00222348.2017.1330183
Jabbar, F., Abbas, G., Ameer, N., Akhtar, M. F., Shah, S., and Hanif, M. (2021). Preparation and characterization of halloysite nanotubes containing hydrogels for controlled release drug delivery of cetirizine dihydrochloride. Polym. Bull. 79, 5417–5435. doi:10.1007/s00289-021-03750-6
Jayaramudu, T., Ko, H. U., Kim, H. C., Kim, J. W., and Kim, J. (2019). Swelling behavior of polyacrylamide-cellulose nanocrystal hydrogels: Swelling kinetics, temperature, and pH effects. Mater. (Basel) 12 (13), 2080. doi:10.3390/ma12132080
Katewongsa, P., Lertsuphotvanit, N., and Phaechamud, T. (2017). Cetirizine dihydrochloride, beta-cyclodextrin inclusion complex by ethanol kneading for taste masking. Indian J. Pharm. Sci. 79 (5), 758–767. doi:10.4172/pharmaceutical-sciences.1000289
Katime, I., and Rodriguez, E. (2001). Absorption of metal ions and swelling properties of poly (acrylic acid-co-itaconic acid) hydrogels. J. Macromol. Sci. Part A. 38 (5-6), 543–558. doi:10.1081/ma-100103366
Katime, I., Valderruten, N., and Quintana, J. R. (2001). Controlled release of aminophylline from poly (N-isopropylacrylamide-co-itaconic acid) hydrogels. Polym. Int. 50 (8), 869–879. doi:10.1002/pi.707
Khalid, I., Ahmad, M., Minhas, M. U., and Barkat, K. (2018). Synthesis and evaluation of chondroitin sulfate based hydrogels of loxoprofen with adjustable properties as controlled release carriers. Carbohydr. Polym. 181, 1169–1179. doi:10.1016/j.carbpol.2017.10.092
Khan, A., Andleeb, A., Azam, M., Tehseen, S., Mehmood, A., and Yar, M. (2023). Aloe vera and ofloxacin incorporated chitosan hydrogels show antibacterial activity, stimulate angiogenesis and accelerate wound healing in full thickness rat model. J. Biomed. Mater. Res. 111 (2), 331–342. doi:10.1002/jbm.b.35153
Khan, M. I., Murtaza, G., Sher, M., Iqbal, M., Waqas, M. K., Rasool, A., et al. (2011). Development and validation of stability indicating assay method of cetirizine hydrochloride by HPLC. Afr. J. Pharm. Pharmacol. 5 (2), 143–149. doi:10.5897/ajpp10.283
Kirimura, K., Honda, Y., and Hattori, T. (2011). “3.14 - gluconic and itaconic acids,” in Comprehensive Biotechnology. Editor M. Moo-Young Second Edition (Burlington: Academic Press), 143–147.
Kumar, A., Sharma, G., Thakur, M., and Pathania, D. (2019). Sol–gel synthesis of polyacrylamide-stannic arsenate nanocomposite ion exchanger: Binary separations and enhanced photo-catalytic activity. SN Appl. Sci. 1 (8), 862. doi:10.1007/s42452-019-0905-6
Kumar, S., Krishnan, S., Samal, S. K., Mohanty, S., and Nayak, S. K. (2017). Itaconic acid used as a versatile building block for the synthesis of renewable resource-based resins and polyesters for future prospective: A review. Polym. Int. 66 (10), 1349–1363. doi:10.1002/pi.5399
Kumar, V., Rehani, V., and Kaith, B. (2018). Microwave-assisted synthesis of biodegradable interpenetrating polymer network of aloe vera–poly (acrylic acid-co-acrylamide) for removal of malachite green dye: Equilibrium, kinetics and thermodynamic studies. Iran. Polym. J. 27 (11), 913–926. doi:10.1007/s13726-018-0665-y
Laux, A., Gouws, C., and Hamman, J. H. (2019). Aloe vera gel and whole leaf extract: Functional and versatile excipients for drug delivery? Expert Opin. drug Deliv. 16 (12), 1283–1285. doi:10.1080/17425247.2019.1675633
Park, K. R., and Nho, Y. C. (2003). Preparation and characterization by radiation of poly (vinyl alcohol) and poly (N-vinylpyrrolidone) hydrogels containing aloe vera. J. Appl. Polym. Sci. 90 (6), 1477–1485. doi:10.1002/app.12656
Pereira, G. G., Santos-Oliveira, R., Albernaz, M. S., Canema, D., Weismüller, G., Barros, E. B., et al. (2014). Microparticles of Aloe vera/vitamin E/chitosan: Microscopic, a nuclear imaging and an in vivo test analysis for burn treatment. Eur. J. Pharm. Biopharm. 86 (2), 292–300. doi:10.1016/j.ejpb.2013.10.011
Pereira, R., Carvalho, A., Vaz, D. C., Gil, M., Mendes, A., and Bártolo, P. (2013). Development of novel alginate based hydrogel films for wound healing applications. Int. J. Biol. Macromol. 52, 221–230. doi:10.1016/j.ijbiomac.2012.09.031
Pereira, R., Tojeira, A., Vaz, D. C., Mendes, A., and Bártolo, P. (2011). Preparation and characterization of films based on alginate and aloe vera. Int. J. Polym. Analysis Charact. 16 (7), 449–464. doi:10.1080/1023666x.2011.599923
Quintana, J. R., Valderruten, N. E., and Katime, I. (2002). Mechanical properties of poly (N-isopropylacrylamide-co-itaconic acid) hydrogels. J. Appl. Polym. Sci. 85 (12), 2540–2545. doi:10.1002/app.10852
Rahman, S., Carter, P., and Bhattarai, N. (2017). Aloe vera for tissue engineering applications. J. Funct. biomaterials 8 (1), 6. doi:10.3390/jfb8010006
Ranjha, N. M., Hanif, M., Naz, A., Shah, M. S., Abbas, G., and Afzal, Z. (2016). Synthesis and characterization of cetirizine−containing, pH−sensitive acrylic acid/poly (vinyl alcohol) hydrogels. J. Appl. Polym. Sci. 133 (19), 1–8. doi:10.1002/app.43407
Rehman, F., Khan, I. U., Khalid, S. H., Asghar, S., Irfan, M., Khalid, I., et al. (2021). Optimization, in vitro release and toxicity evaluation of novel pH sensitive itaconic acid-g-poly (acrylamide)/sterculia gum semi-interpenetrating networks. DARU J. Pharm. Sci. 29 (1), 171–184. doi:10.1007/s40199-021-00395-8
Rompicherla, N. C., Joshi, P., Shetty, A., Sudhakar, K., Amin, H. I. M., Mishra, Y., et al. (2022). Design, formulation, and evaluation of aloe vera gel-based capsaicin transemulgel for osteoarthritis. Pharmaceutics 14 (9), 1812. doi:10.3390/pharmaceutics14091812
Sabaa, M., Magid, E., and Mohamed, R. (2017). Maize starch-g-poly (Itaconic acid) and its application in sewage water treatment and as antimicrobial agent. Sci. Rev. Chem. Commun. 7 (1), 1–17.
Sedlačík, T., Nonoyama, T., Guo, H., Kiyama, R., Nakajima, T., Takeda, Y., et al. (2020). Preparation of tough double- and triple-network supermacroporous hydrogels through repeated cryogelation. Chem. Mater. 32 (19), 8576–8586. doi:10.1021/acs.chemmater.0c02911
Shalviri, A., Liu, Q., Abdekhodaie, M. J., and Wu, X. Y. (2010). Novel modified starch–xanthan gum hydrogels for controlled drug delivery: Synthesis and characterization. Carbohydr. Polym. 79 (4), 898–907. doi:10.1016/j.carbpol.2009.10.016
Sharma, D., Singh, G., Kumar, D., and Singh, M. (2015). Formulation development and evaluation of fast disintegrating tablets of salbutamol sulphate, cetirizine hydrochloride in combined pharmaceutical dosage form: A new era in novel drug delivery for pediatrics and geriatrics. J. drug Deliv. 2015, 1–10. doi:10.1155/2015/640529
Sharma, D., Singh, M., Kumar, D., and Singh, G. (2014). Formulation development and evaluation of fast disintegrating tablet of cetirizine hydrochloride: A novel drug delivery for pediatrics and geriatrics. J. Pharm. 2014, 1–8. doi:10.1155/2014/808167
Sharma, K., Kumar, V., Kaith, B., Kumar, V., Som, S., Kalia, S., et al. (2014). A study of the biodegradation behaviour of poly (methacrylic acid/aniline)-grafted gum ghatti by a soil burial method. RSC Adv. 4 (49), 25637–25649. doi:10.1039/c4ra03765k
Shoukat, H., Pervaiz, F., Khan, M., Rehman, S., Akram, F., Abid, U., et al. (2022). Development of β-cyclodextrin/polyvinypyrrolidone-co-poly (2-acrylamide-2-methylpropane sulphonic acid) hybrid nanogels as nano-drug delivery carriers to enhance the solubility of Rosuvastatin: An in vitro and in vivo evaluation. Plos one 17 (1), e0263026. doi:10.1371/journal.pone.0263026
Sohail, K., Khan, I. U., Shahzad, Y., Hussain, T., and Ranjha, N. M. (2014). pH-sensitive polyvinylpyrrolidone-acrylic acid hydrogels: Impact of material parameters on swelling and drug release. Braz. J. Pharm. Sci. 50 (1), 173–184. doi:10.1590/s1984-82502011000100018
Sovizi, M. R., and Hosseini, S. G. (2013). Studies on the thermal behavior and decomposition kinetic of drugs cetirizine and simvastatin. J. Therm. analysis Calorim. 111 (3), 2143–2148. doi:10.1007/s10973-012-2651-5
Subramani, R., Izquierdo-Alvarez, A., Bhattacharya, P., Meerts, M., Moldenaers, P., Ramon, H., et al. (2020). The influence of swelling on elastic properties of polyacrylamide hydrogels. Front. Mater. 7, 212. doi:10.3389/fmats.2020.00212
Torres-Figueroa, A. V., Pérez-Martínez, C. J., Castillo-Castro, T., Bolado-Martínez, E., Corella-Madueño, M. A., García-Alegría, A. M., et al. (2020). Composite hydrogel of poly (acrylamide) and starch as potential system for controlled release of amoxicillin and inhibition of bacterial growth. J. Chem. 2020, 1–14. doi:10.1155/2020/5860487
Wei, S., Chen, W., Tong, Z., Jiang, N., and Zhu, M. (2021). Synthesis of a functional biomass lignin-based hydrogel with high swelling and adsorption capability towards Acid Red 73. Environ. Sci. Pollut. Res. 28 (37), 51306–51320. doi:10.1007/s11356-021-14324-4
Zahra, Q., Minhas, M. U., Khan, S., Wu, P-C., Suhail, M., Iqbal, R., et al. (2021). Fabrication of polyethylene glycol hydrogels with enhanced swelling; loading capacity and release kinetics. Polym. Bull. 79, 5389–5415. doi:10.1007/s00289-021-03740-8
Zhang, X., Han, M., Xu, L., and AlSofi, A. M. (2022). Long-term stability prediction of polyacrylamide-type polymers at harsh conditions via thermogravimetric analysis. Chem. Phys. Lett. 795, 139538. doi:10.1016/j.cplett.2022.139538
Keywords: semi-interpenetrating network, sustainability of natural resources, aloe vera, itaconic acid, pH sensitive
Citation: Ajaz N, Bukhsh M, Kamal Y, Rehman F, Irfan M, Khalid SH, Asghar S, Rizg WY, Bukhary SM, Hosny KM, Alissa M, Safhi AY, Sabei FY and Khan IU (2023) Development and evaluation of pH sensitive semi-interpenetrating networks: assessing the impact of itaconic acid and aloe vera on network swelling and cetirizine release. Front. Bioeng. Biotechnol. 11:1173883. doi: 10.3389/fbioe.2023.1173883
Received: 25 February 2023; Accepted: 24 April 2023;
Published: 09 May 2023.
Edited by:
Shuai Liang, Saint-Gobain Research North America, United StatesReviewed by:
Zichao Wei, Saint-Gobain Research North America, United StatesYan Cong, Rogers Corporation, United States
Copyright © 2023 Ajaz, Bukhsh, Kamal, Rehman, Irfan, Khalid, Asghar, Rizg, Bukhary, Hosny, Alissa, Safhi, Sabei and Khan. This is an open-access article distributed under the terms of the Creative Commons Attribution License (CC BY). The use, distribution or reproduction in other forums is permitted, provided the original author(s) and the copyright owner(s) are credited and that the original publication in this journal is cited, in accordance with accepted academic practice. No use, distribution or reproduction is permitted which does not comply with these terms.
*Correspondence: Ikram Ullah Khan, aWtyYW1nbHRAZ21haWwuY29t