- 1Department of Orthopedics, Affiliated Zhongshan Hospital of Dalian University, Dalian, China
- 2Department of Engineering Mechanics, Dalian University of Technology, Dalian, China
- 3Department of anesthesiology, Affiliated Zhongshan Hospital of Dalian University, Dalian, China
Objective: To select the most appropriate internal fixation method based on the Pauwels angle, in order to provide a new concept for clinical accurate treatment of femoral neck fractures (FNFs).
Methods: FNFs models of Pauwels
Results: At Pauwels
Conclusion: Pauwels type Ⅰ (<
1 Introduction
FNFs account for 3.6% of the total fractures and 53% of hip fractures (Thorngren et al., 2002). Due to its special anatomical structure and biomechanical complexity, the selection of internal fixation should be as small as possible and have little interference with the blood supply, and more importantly, it should provide sufficient stability. At present, the mainstream internal fixation methods in the clinical applications include: cannulated compression screws (CCS) and dynamic hip screw (DHS) (Figures 1A,B) (Li et al., 2018). However, the current conventional fixation method interferes greatly with the blood supply in the femoral head (Zhao et al., 2017), and shows insufficient shear force for Pauwels type Ⅲ fracture (Li et al., 2019b). The rate of bone non-union after internal fixation is as high as 10%–34%, and the rate of osteonecrosis is as high as 35%–48%. Therefore, it has always been a difficult problem in orthopedic treatment (Davidovitch et al., 2010; Nauth et al., 2017; Medda et al., 2019).
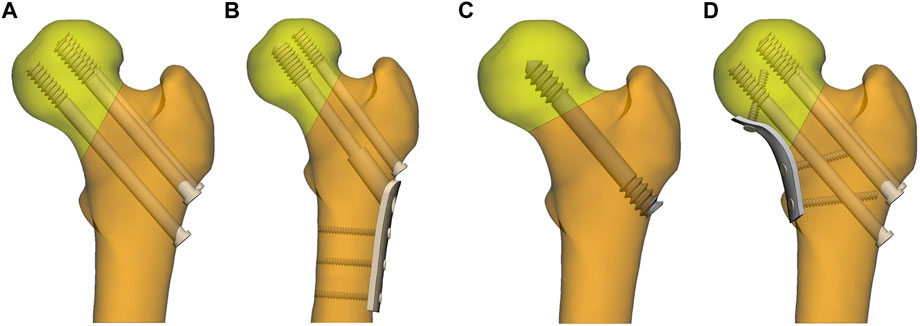
FIGURE 1. Internal fixation of femoral neck fractures. (A) three parallel cannulated compression screws (CCS); (B) dynamic hip screws and anti-rotation screws; (C) porous tantalum screws (PTS); (D) three parallel cannulated compression screws and medial buttress plate (MBP).
The Pauwels classification, which is vital for the biomechanical assessment of fracture healing, is based on the angle between the fracture line (C, D, E, F) and horizontal line B parallel to the iliac crest line on both sides in Figure 2. Specifically, it can be divided into three types: Pauwels Ⅰ type (<
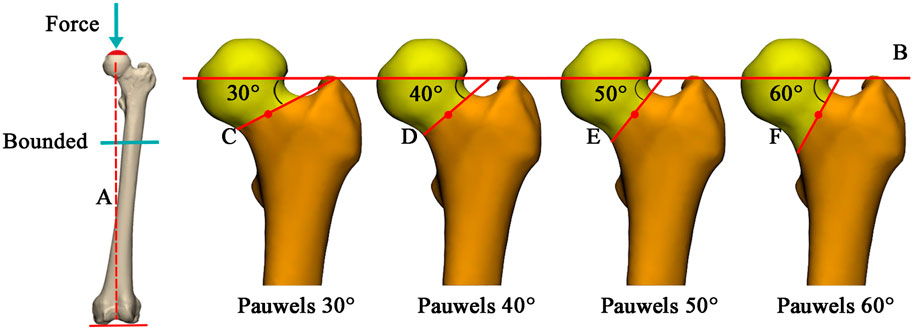
FIGURE 2. Illustration of the loading and femoral neck fracture model. (A) mechanical axis of the femur; (B) parallel lines of horizontal lines connecting the iliac crest on both sides and passing through the center of the femoral head; (C–F): fracture lines of Pauwels 30°, 40°, 50°, and 60°, respectively, passing through the center of the femoral neck.
As for Pauwels type III fractures, also known as vertical femoral neck fractures (vFNFs), due to the high vertical shear force and the fact that the tension provided by the internal fixator along the axis of the femoral neck has a component parallel to the fracture rim, an increase in the shear force at the fracture surface was observed. Thus the tension generated by the screw increased the vertical shear force on the fracture surface when CCS were used to fix the fracture, resulting in a high complication and fixation failure rate (Liporace et al., 2008). To tackle this problem, the scholars (Mir and Collinge, 2015) conceptualized incorporating a MBP into the treatment of displaced FNFs (Figure 1D). The MBP not only improved stability, but also converted the vertical shear force at the fracture surface into a compressive stress that promoted fracture healing and improved cure rates.
The healing time of FNFs and the incidence of osteonecrosis are closely related to the integrity of the internal vascular preservation of the femoral head. Therefore, ensuring tough internal fixation and protecting the remaining blood supply of the femoral head are important to avoid postoperative complications (Zhao et al., 2022). In addition, the biomechanical stability of Pauwels Ⅰ and Ⅱ fractures is better, and the stability of Pauwels Ⅲ fractures can also be improved to a certain extent after the fixation of the MBP. Therefore, we proposed that the number of internal fixations can be reduced to decrease the disruption of blood supply and the occurrence of complications, and ensure the tough fixation of the fracture. In this study, FNFs models for Pauwels
2 Materials and methods
2.1 Establishment of three-dimensional femur model
A 27-year-old healthy male volunteer (175cm, 70 kg) with no history of hip or systemic disease was recruited. A Siemens 64-row spiral CT scanner was used to scan the entire femur with a thickness of 0.5 mm. The CT image was stored in the standard format of Digital Imaging and Communications in Medicine (DICOM) in Mimics 21 (The Materialise Group, Leuven, Belgium), a medical 3D reconstruction software. Appropriate gray values were selected to distinguish bone and tissue, and the three-dimensional model of the original femur was established. Then the reconstructed model was imported into 3-Matic (The Materialise Group, Leuven, Belgium) software for surface optimization processing, such as model surface defect repair, smoothing and accurate surface function.
2.2 Establishment of femur neck fractures model
The fracture model was established in 3-Matic software. Mechanical axis A of the femur (the line between the center point of the femoral head and the midpoint of the medial and lateral condyles of the femur on the coronal plane) was first made, then the horizontal line B, iliac crest on both sides parallel line, was made passing through the head center. The straight line C (Pauwels
2.3 Establishment of the internal fixation model
In this study, 16 fixation methods were used for 4 FNFs models. As the main purpose of this study is to investigate and compare fractures of different Pauwels classifications to select the best internal fixation mode. Experimental tests on cadaveric and on synthetic bones could provide useful information, and using full-field experimental techniques such as digital image correlation strain measurement technique (Dickinson et al., 2011) or differential thermography (Zanetti and Audenino, 2012) is highly recommended. The FE analysis method was used in this study, and it is worth noting that the focus of this study is unrelated to the thread. Therefore, to establish a FE model and facilitate calculation, all the thread portions were simplified into smooth and thick solid cylinders. The length of the thread portion was 18.0 mm, the diameter was 7.5 mm, and the diameter of the screw was 6.5 mm. The appropriate length of internal fixation was selected according to the fracture model. Firstly, in 1CCS, the screw was placed in the center of the femoral neck, and the length was 88.0 mm. Secondly, in 2CCS, the screws were fixed vertically up and down, where in the antero-posterior view, the two screws were close to the superior and inferior cortex, and where in the lateral view, the two screws were on the midline of the femoral neck. The length of the upper and lower screws were 81.0 and 90.0 mm respectively. Thirdly, in 3CCS, the screws were arranged in an inverted triangle. According to the maximum width of the three screws, the upper two screws were close to the cortex, the lower one was close to the femoral calcar. The three screws were 2.5 mm from the cortical bone and 5.0 mm from the distal subchondral bone of the femoral head, and the length of the three screws were 81, 81 and 90 mm. Fourthly, the PTS specifications were designed according to previous study (Zhao et al., 2022), similarly, the head and tail threads were simplified to a smooth and thick solid cylinder. Fifth, the thinner steel plate may have less stimulation to the internal structure of the femoral neck, so the four-hole to six-hole buttress plate is commonly used in clinic. Therefore, a MBP of 2.7 mm thickness was created in 3-Matic to fit the bone surface with reference to Stryker (Mahwah, NJ, United States) four-hole locking plate. The diameter of the plate locking screw was 3.5 mm, and the lengths were 25.0, 30.0, and 35.0 mm. The above model was Non-Fluid assembled in 3-Matic, and the effect diagram after assembly is shown in Figure 3.
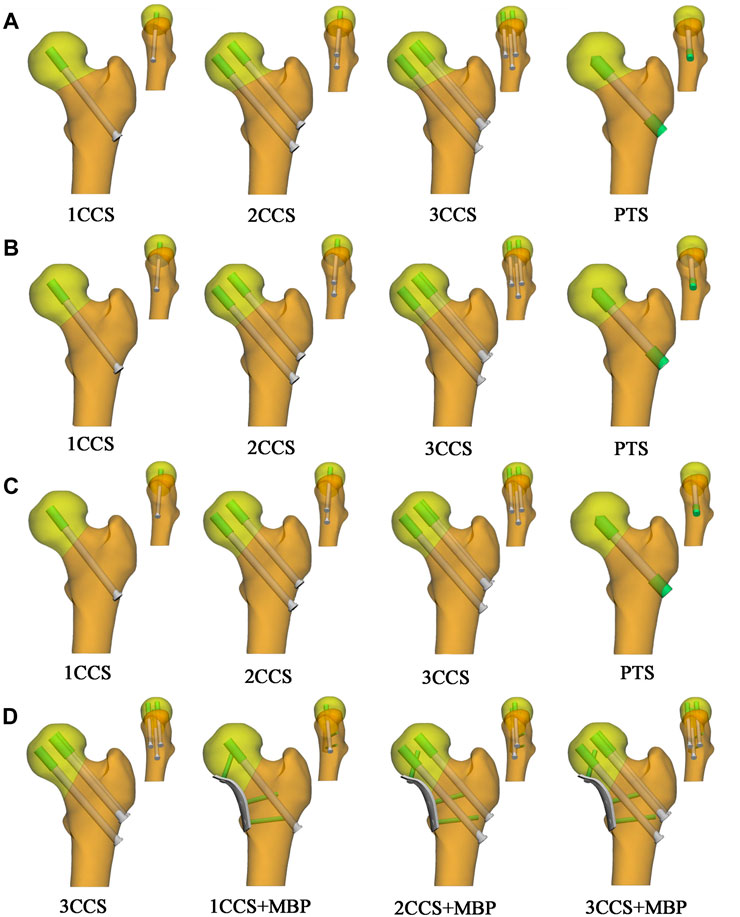
FIGURE 3. Illustration of the Non-manifold assembly FE model. (A) Pauwels 30°; (B) Pauwels 40°; (C) Pauwels 50°; (D) Pauwels 60°.
2.4 Establishment of the FE model
FE model meshed with tetrahedral 4-nodes elements (C3D4). The average size of the proximal femur mesh was controlled to 2.0 mm, and the minimum size of the mesh was controlled to more than 1.0 mm at the bone-screw contact surface and the fracture surface of the femoral neck. To obtain both the actual structure and the calculated scale of the models, the average size of the mesh was 1.0 mm, and the average size of the body mesh of the medial buttress plate was 1.5 mm. All models being analyzed were assumed to be continuous, isotropic, and with homogeneous linear elastic materials (Sitthiseripratip et al., 2003; Taheri et al., 2011). The models were re-imported into Mimics 21 and assigned material properties according to the corresponding regions of cortical bone and cancellous bone obtained by CT scanning. This study mainly evaluated various internal fixation methods from the perspective of structure. Titanium alloy (Ti-6Al-4V) was used in all internal fixation devices to eliminate the bias caused by different materials. The parameters of various materials are listed in Table 1 (Fu et al., 2022).
2.5 Setting of the model parameters
The above assembled models were imported into Abaqus 2021 (Simulia Corp, Providence, RI, United States), and the frictional contact interactions were set according to previous studies: the screws, MBP and bone was 0.3, and the interaction between fracture surface was 0.46 (Eberle et al., 2010). Besides, the binding relationship between thread, plate locking screw and bone was set. No pre-strain given by the screws between the two bone fragments was set. All nodes on the distal femur surface were constrained with 0 degrees of freedom to prevent rigid body movement during analysis. From the previous studies, when walking or running on the ground, the combined force exerted by human gravity and the muscles and ligaments around the hip joint can reach 3 to 5 times of the body weight (Cha et al., 2019). Therefore, in the present analysis, a load of 2100N corresponding to 3 times the body weight was uniformly applied to the main weight-bearing area of the femoral head along the mechanical axis of the femur as shown in Figure 2.
2.6 Evaluation criteria
Firstly, the evaluation parameters of MBS, MIS, MBD were measured. Secondly, since stiffness may not accurately reflect the stability around the fracture site, we did not use it as an evaluation parameter in the analysis of experimental results. Instead, interfragmentary motion (IFM) at the fracture end was used as an evaluation parameter. It can reflect the real “stability” of the model, which was the ultimate embodiment of stiffness and directly affects the healing effect. Previous studies have suggested that the optimal micromovement amplitude to accelerate fracture healing was within 0.5 mm (Kenwright and Goodship, 1989; Wolf et al., 1998).
3 Result
3.1 Validation of the developed FE model
Pauwels
3.2 The von mises stress distribution
In the models with different Pauwels angles, it was observed that the femoral stress was mainly concentrated at the fracture end and the medial cortex of the femur (Figure 4), and the internal fixation stress was mainly concentrated at the screw near the fracture surface and the junction between the plate and the fixation screws (Figure 5). In the same angle fracture, the stress became more distributed and balanced with the increase of the number of internal fixations. In the same internal fixation group, with the increase of Pauwels angle, the maximum stress on the femur and the internal fixation also increased.
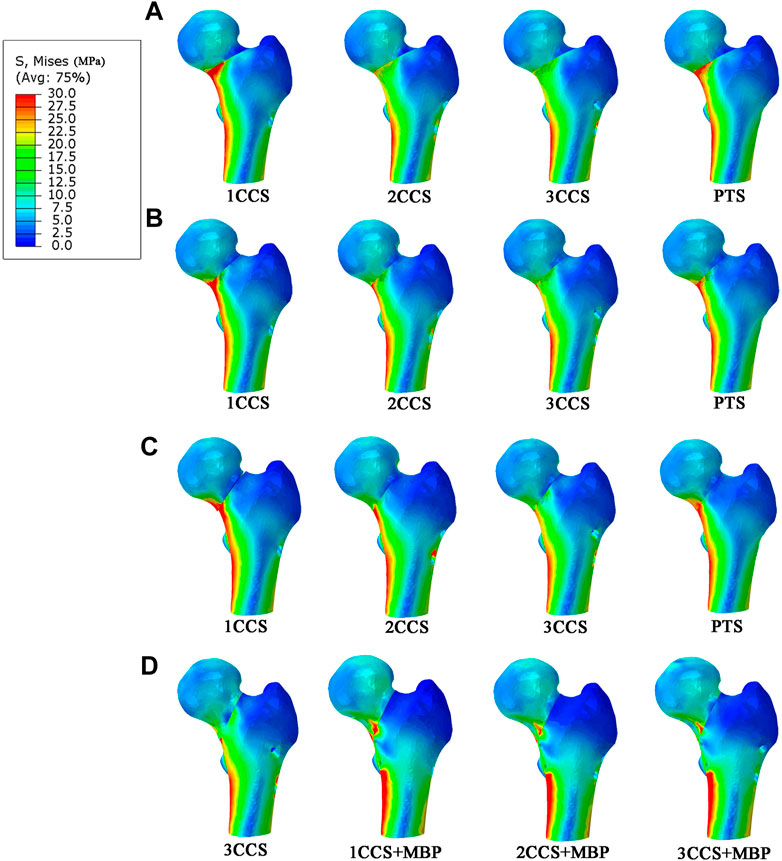
FIGURE 4. Stress distribution in different femur models.(A) Pauwels 30°; (B) Pauwels 40°; (C) Pauwels 50°; (D) Pauwels 60°.
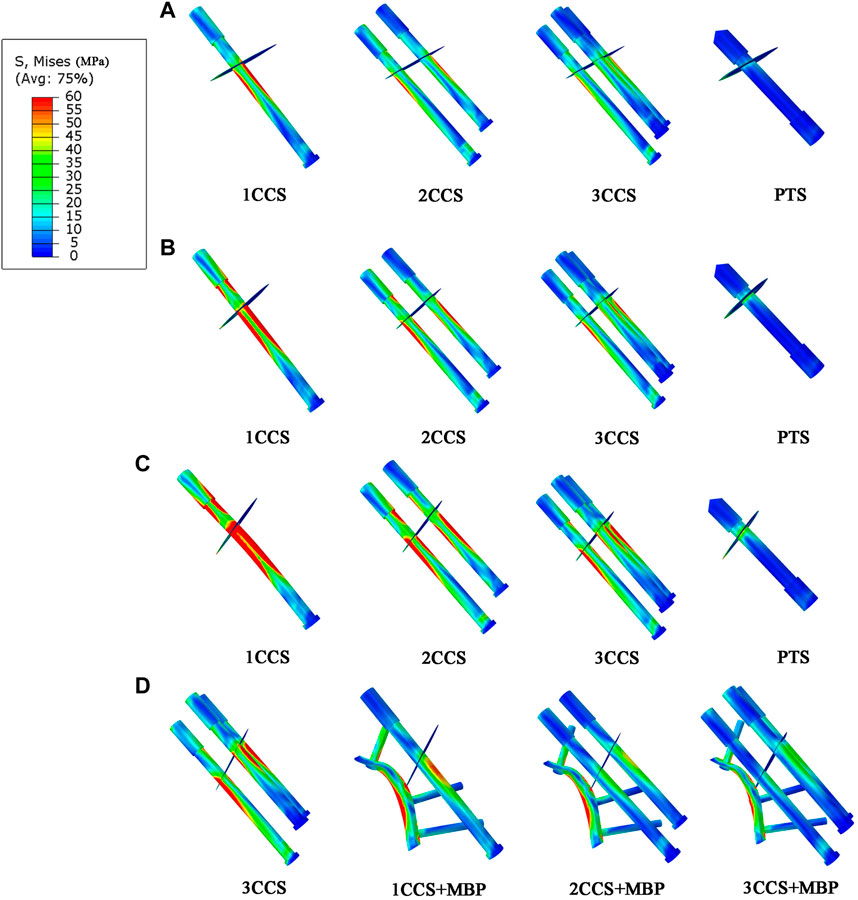
FIGURE 5. Stress distribution in different internal fixation models. (A) Pauwels 30°; (B) Pauwels 40°; (C) Pauwels 50°; (D) Pauwels 60°.
At Pauwels
At Pauwels
3.3 The displacement distribution of different femur models
The displacement distribution of the femur was shown in Figure 6. It can be observed from the figure that the displacement gradually increased from the distal end of the femoral model to the femoral head, and the largest displacements were located at the femoral head where the load was applied. It is worth noting that a discontinuity in displacement pattern is a clear sign of the fracture undergoing tensile stresses. Besides, the femoral displacement of the 1CCS group was the largest, and the maximum displacement decreased with the increase in the number of CCS. When using the same internal fixation, the MBD increased with the increase of the Pauwels angle. In the PTS group, the MBD was between 2CCS and 3CCS, which was close to the 2CCS fixation effect.
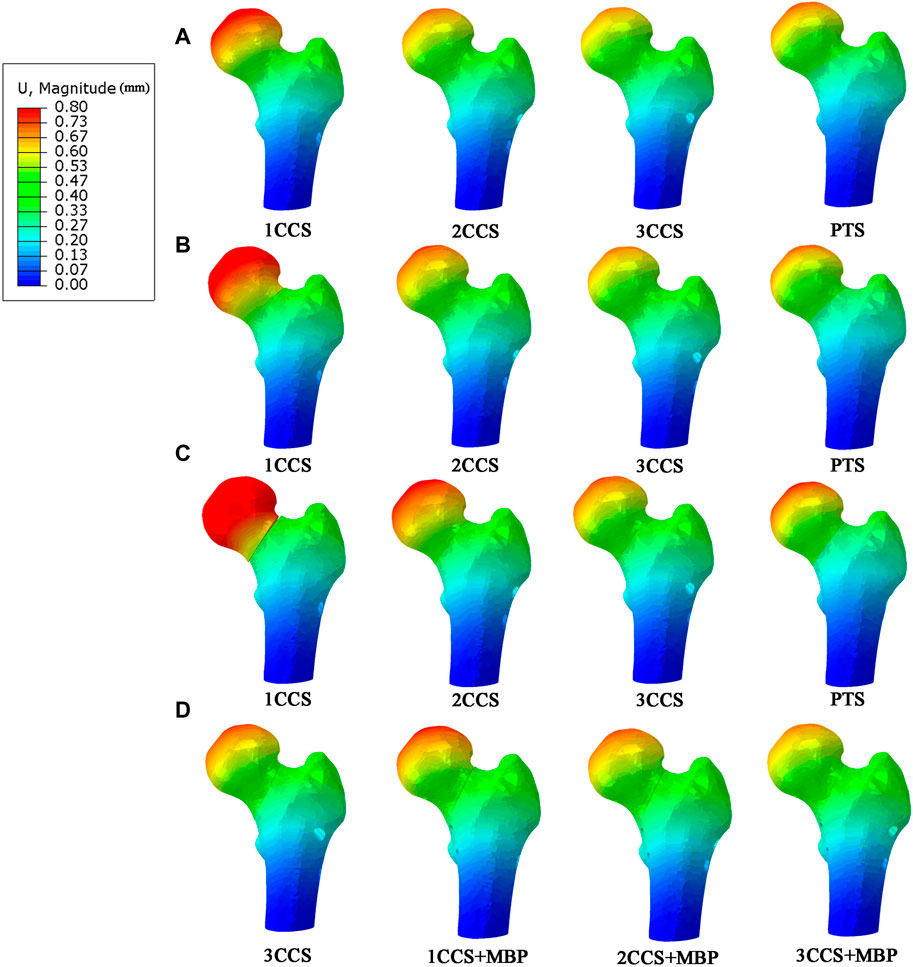
FIGURE 6. Displacement distribution in different femur models. (A) Pauwels 30°; (B) Pauwels 40°; (C) Pauwels 50°; (D) Pauwels 60°.
At Pauwels
3.4 Interfragmentary motion of femur neck fracture
The interfragmentary motion diagram was shown in Figure 7. At Pauwels
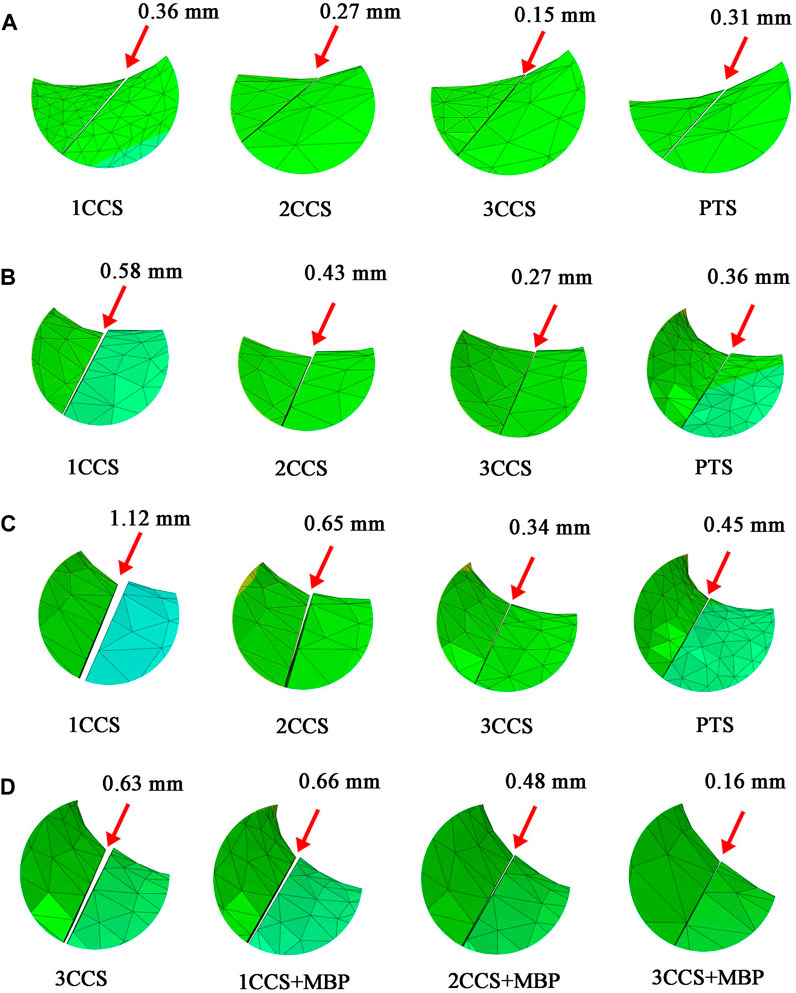
FIGURE 7. Interfragmentary motion of femur neck fracture. (A) Pauwels 30°; (B) Pauwels 40°; (C) Pauwels 50°; (D) Pauwels 60°.
At Pauwels
4 Discussion
FNFs in non-elderly patients are usually treated with hip preservation, but the treatment method is still controversial. With an improved understanding of fracture biomechanics, clinicians have been able to better understand and prevent these complications. Especially, FE analysis was the most widely used method for stress analysis in biomechanical research, which can provide a reliable basis for the selection of internal fixation methods for FNFs.
In recent years, the innovation of internal fixation methods for FNFs has become a research hotspot. There are many innovative and improved methods of internal fixation for Pauwels type Ⅲ fractures. However, the excessive pursuit of the stability of internal fixation leads to the aggravation of the damage to the blood supply of the femoral head. In fact, the biomechanics of fractures with different Pauwels angles are completely different. In this study, we selected appropriate internal fixation methods based on different Pauwels angles and verified their fixation effects. Thus, the difference of the different models could be investigated and the results could provide guidance for the selection of clinical implantation scheme.
From the results of this study, it can be observed that the fracture of Pauwels type Ⅰ (<
For the fracture of Pauwels type Ⅱ (
For Pauwels type Ⅲ fractures (>
In this study, the MBS in the 3CCS group was close to its yield strength. Besides, the stress was concentrated in the contact part of the femur and the lower screw tail, which had the risk of nail withdrawal. The IFM of this group was 0.63 mm, indicating that the fracture fragments were relatively displaced too much, and the bone was prone to non-union in the long term. However, CCS was combined with MBP, which distributed stress on CCS by providing an additional route to transfer stress between fracture fragments. In the 2CCS + MBP group, the IFM was less than 0.5mm, and the other parameters were significantly better than those of the 3CCS group. Furthermore, it can be observed in Figure 4 that the maximum stress was concentrated below the fracture surface of the femoral neck in contact with the plate. The reason for this phenomenon was that the fracture of the neck changed the mechanical conduction in the proximal femur, and the load in the proximal femur was mainly transmitted by these screws and plates. After adding the MBP, the stress of CCS and the medial femoral neck region was significantly reduced, indicating that the plate played a crucial role in supporting the fracture and establishing a better biomechanical environment for fracture healing.
Although we have comprehensively evaluated the different implantation approaches, there are still some limitations in this study. First, all models were developed using linear elastic materials, and the relationship between thread and bone was set as a binding relationship, without bone plastic deformation or screw loosening process. This study only focused on the initial stability, rather than the stability during bone healing. On the other hand, considering that the initial stability is crucial (Aminian et al., 2007) for fracture healing, the results of this study are still meaningful. Second, the intact fracture surface and theoretical anatomical reduction in our model, as well as a null pre-strain given by the screws between the two bone fragments, which may affect the accuracy of the results. Third, the thread of the implant was simplified in this study, but it proved to have little effect on the results (Inzana et al., 2016). Fourth, we only applied forces along the mechanical axis of the femur and did not simulate the muscles attached to the femur (such as action of abduction muscles), which may not accurately reflect the proximal femoral movement in the physiological loading mode and may affect the internal fixation stress distribution. In general, further biomechanical experiments research in cadavers is still necessary in the future. Despite these limitations, our results may help orthopedic surgeons to select the most appropriate fixation strategy in clinical practice.
5 Conclusion
When the Pauwels angle is small, the fracture position has a compressive effect, which is conducive to fracture healing. Therefore, Pauwels type Ⅰ (<
Data availability statement
The original contributions presented in the study are included in the article/Supplementary Material, further inquiries can be directed to the corresponding authors.
Author contributions
FW conducted experiments and edited the entire manuscript. YL and YH wrote the first draft and finalized the manuscript. ZW, JZ, MX, KM and LW was involved in the establishing and analysing the finite element models. YL reviewed and finalized the manuscript. LC and DZ was involved in the supervision and resources.
Funding
This research was funded by Dalian Key Medical Specialties “Peak Climbing” Program (No. 2021 (243)).
Conflict of interest
The authors declare that the research was conducted in the absence of any commercial or financial relationships that could be construed as a potential conflict of interest.
Publisher’s note
All claims expressed in this article are solely those of the authors and do not necessarily represent those of their affiliated organizations, or those of the publisher, the editors and the reviewers. Any product that may be evaluated in this article, or claim that may be made by its manufacturer, is not guaranteed or endorsed by the publisher.
References
Aminian, A., Gao, F., Fedoriw, W. W., Zhang, L. Q., Kalainov, D. M., and Merk, B. R. (2007). Vertically oriented femoral neck fractures: Mechanical analysis of four fixation techniques. J. Orthop. Trauma 21 (8), 544–548. doi:10.1097/BOT.0b013e31814b822e
Bartoníček, J. (2001). Pauwels' classification of femoral neck fractures: Correct interpretation of the original. J. Orthop. Trauma 15 (5), 358–360. doi:10.1097/00005131-200106000-00009
Cha, Y. H., Yoo, J. I., Hwang, S. Y., Kim, K. J., Kim, H. Y., Choy, W. S., et al. (2019). Biomechanical evaluation of internal fixation of Pauwels type III femoral neck fractures: A systematic review of various fixation methods. Clin. Orthop. Surg. 11 (1), 1–14. doi:10.4055/cios.2019.11.1.1
Davidovitch, R. I., Jordan, C. J., Egol, K. A., and Vrahas, M. S. (2010). Challenges in the treatment of femoral neck fractures in the nonelderly adult. J. Trauma 68 (1), 236–242. doi:10.1097/TA.0b013e3181c428ce
Dickinson, A. S., Taylor, A. C., Ozturk, H., and Browne, M. (2011). Experimental validation of a finite element model of the proximal femur using digital image correlation and a composite bone model. J. Biomech. Eng. 133 (1), 014504. doi:10.1115/1.4003129
Eberle, S., Gerber, C., von Oldenburg, G., Högel, F., and Augat, P. (2010). A biomechanical evaluation of orthopaedic implants for hip fractures by finite element analysis and in-vitro tests. Proc. Inst. Mech. Eng. H. 224 (10), 1141–1152. doi:10.1243/09544119jeim799
Fu, G., Zhong, G., Yang, Z., Cheng, S., Ma, L., and Zhang, Y. (2022). Two cannulated screws provide sufficient biomechanical strength for prophylactic fixation in adult patients with an aggressive benign femoral neck lesion. Front. Bioeng. Biotechnol. 10, 891338. doi:10.3389/fbioe.2022.891338
Inzana, J. A., Varga, P., and Windolf, M. (2016). Implicit modeling of screw threads for efficient finite element analysis of complex bone-implant systems. J. Biomech. 49 (9), 1836–1844. doi:10.1016/j.jbiomech.2016.04.021
Kenwright, J., and Goodship, A. E. (1989). Controlled mechanical stimulation in the treatment of tibial fractures. Clin. Orthop. Relat. Res. 241, 36–47. doi:10.1097/00003086-198904000-00006
Krastman, P., van den Bent, R. P., Krijnen, P., and Schipper, I. B. (2006). Two cannulated hip screws for femoral neck fractures: Treatment of choice or asking for trouble? Arch. Orthop. Trauma Surg. 126 (5), 297–303. doi:10.1007/s00402-006-0143-4
Lazaro, L. E., Sculco, P. K., Pardee, N. C., Klinger, C. E., Dyke, J. P., Helfet, D. L., et al. (2013). Assessment of femoral head and head-neck junction perfusion following surgical hip dislocation using gadolinium-enhanced magnetic resonance imaging: A cadaveric study. J. Bone Jt. Surg. Am. 95 (23), e182–e1828. doi:10.2106/jbjs.L.01185
Li, J., Wang, M., Zhou, J., Han, L., Zhang, H., Li, C., et al. (2018). Optimum configuration of cannulated compression screws for the fixation of unstable femoral neck fractures: Finite element analysis evaluation. Biomed. Res. Int. 2018, 1–10. doi:10.1155/2018/1271762
Li, J., Yin, P., Zhang, L., Chen, H., and Tang, P. (2019a). Medial anatomical buttress plate in treating displaced femoral neck fracture a finite element analysis. Injury 50 (11), 1895–1900. doi:10.1016/j.injury.2019.08.024
Li, J., Zhao, Z., Yin, P., Zhang, L., and Tang, P. (2019b). Comparison of three different internal fixation implants in treatment of femoral neck fracture-a finite element analysis. J. Orthop. Surg. Res. 14 (1), 76. doi:10.1186/s13018-019-1097-x
Liporace, F., Gaines, R., Collinge, C., and Haidukewych, G. J. (2008). Results of internal fixation of Pauwels type-3 vertical femoral neck fractures. J. Bone Jt. Surg. Am. 90 (8), 1654–1659. doi:10.2106/JBJS.G.01353
Ma, J. X., Kuang, M. J., Xing, F., Zhao, Y. L., Chen, H. T., Zhang, L. K., et al. (2018). Sliding hip screw versus cannulated cancellous screws for fixation of femoral neck fracture in adults: A systematic review. Int. J. Surg. 52, 89–97. doi:10.1016/j.ijsu.2018.01.050
Maurer, S. G., Wright, K. E., Kummer, F. J., Zuckerman, J. D., and Koval, K. J. (2003). Two or three screws for fixation of femoral neck fractures? Am. J. Orthop. (Belle Mead NJ) 32 (9), 438–442.
Medda, S., Snoap, T., and Carroll, E. A. (2019). Treatment of young femoral neck fractures. J. Orthop. Trauma 33 (1), S1–S6. doi:10.1097/bot.0000000000001369
Mir, H., and Collinge, C. (2015). Application of a medial buttress plate may prevent many treatment failures seen after fixation of vertical femoral neck fractures in young adults. Med. Hypotheses 84 (5), 429–433. doi:10.1016/j.mehy.2015.01.029
Nauth, A., Creek, A. T., Zellar, A., Lawendy, A.-R., Dowrick, A., Gupta, A., et al. (2017). Fracture fixation in the operative management of hip fractures (FAITH): An international, multicentre, randomised controlled trial. Lancet 389 (10078), 1519–1527. doi:10.1016/S0140-6736(17)30066-1
Parker, M. J. (2009). Results of internal fixation of Pauwels type-3 vertical femoral neck fractures. J. Bone Jt. Surg. Am. 91 (2), 490–491. author reply 491.
Sitthiseripratip, K., Van Oosterwyck, H., Vander Sloten, J., Mahaisavariya, B., Bohez, E. L., Suwanprateeb, J., et al. (2003). Finite element study of trochanteric gamma nail for trochanteric fracture. Med. Eng. Phys. 25 (2), 99–106. doi:10.1016/s1350-4533(02)00185-6
Taheri, N. S., Blicblau, A. S., and Singh, M. (2011). Comparative study of two materials for dynamic hip screw during fall and gait loading: Titanium alloy and stainless steel. J. Orthop. Sci. 16 (6), 805–813. doi:10.1007/s00776-011-0145-0
Thorngren, K. G., Hommel, A., Norrman, P. O., Thorngren, J., and Wingstrand, H. (2002). Epidemiology of femoral neck fractures. Injury 33 (3), C1–C7. doi:10.1016/s0020-1383(02)00324-8
Tianye, L., Peng, Y., Jingli, X., QiuShi, W., GuangQuan, Z., Wei, H., et al. (2019). Finite element analysis of different internal fixation methods for the treatment of Pauwels type III femoral neck fracture. Biomed. Pharmacother. 112, 108658. doi:10.1016/j.biopha.2019.108658
Wolf, S., Janousek, A., Pfeil, J., Veith, W., Haas, F., Duda, G., et al. (1998). The effects of external mechanical stimulation on the healing of diaphyseal osteotomies fixed by flexible external fixation. Clin. Biomech. (Bristol, Avon) 13 (4-5), 359–364. doi:10.1016/s0268-0033(98)00097-7
Zanetti, E. M., and Audenino, A. L. (2012). Differential thermography for experimental, full-field stress analysis of hip arthroplasty. J. Mech. Med. Biol. 10 (03), 515–529. doi:10.1142/s0219519410003496
Zhan, S., Jiang, D., Xu, J., Ling, M., Yang, K., Li, Y., et al. (2020). Influence of the proximal screws of buttress plates on the stability of vertical femoral neck fractures: A finite element analysis. BMC Musculoskelet. Disord. 21 (1), 842. doi:10.1186/s12891-020-03853-7
Zhao, D., Ma, Z., Liu, B., Yang, L., Qiu, X., Tian, S., et al. (2022). A new method to protect blood supply in the treatment of femoral neck fractures: Bidirectional compression porous tantalum screws. Orthop. Surg. 14 (9), 1964–1971. doi:10.1111/os.13285
Keywords: femoral neck fractures, finite element analysis, biomechanics, medial buttress plate, cannulated compression screws, porous tantalum screws
Citation: Wang F, Liu Y, Huo Y, Wang Z, Zhang J, Xu M, Ma K, Wang L, Lu Y, Cheng L and Zhao D (2023) Biomechanical study of internal fixation methods for femoral neck fractures based on Pauwels angle. Front. Bioeng. Biotechnol. 11:1143575. doi: 10.3389/fbioe.2023.1143575
Received: 13 January 2023; Accepted: 20 February 2023;
Published: 03 March 2023.
Edited by:
Fuhao Mo, Hunan University, ChinaReviewed by:
Fengyuan Zhao, Peking University Third Hospital, ChinaElisabetta M. Zanetti, University of Perugia, Italy
Copyright © 2023 Wang, Liu, Huo, Wang, Zhang, Xu, Ma, Wang, Lu, Cheng and Zhao. This is an open-access article distributed under the terms of the Creative Commons Attribution License (CC BY). The use, distribution or reproduction in other forums is permitted, provided the original author(s) and the copyright owner(s) are credited and that the original publication in this journal is cited, in accordance with accepted academic practice. No use, distribution or reproduction is permitted which does not comply with these terms.
*Correspondence: Dewei Zhao, zhaodewei2016@163.com; Liangliang Cheng, liangliang30766@163.com